DOI:
10.1039/D4QO01495B
(Research Article)
Org. Chem. Front., 2024,
11, 6735-6741
Ir/XuPhos-catalyzed direct asymmetric reductive amination of ketones with secondary amines†
Received
14th August 2024
, Accepted 29th September 2024
First published on 5th October 2024
Abstract
A diverse array of bidentate P-containing ligands has been developed for Ir-catalyzed asymmetric hydrogenation, but chiral monodentate phosphine ligands have remained comparatively underexplored. We herein report a novel iridium catalyst with XuPhos as a chiral monodentate phosphine ligand for the direct asymmetric reductive amination of ketones with secondary amines. This catalytic system tolerates a wide range of substrates, providing a series of chiral tertiary amines efficiently with high enantioselectivities. The high catalytic activity is attributed to the presence of the aliphatic cyclohexyl group on the P-atom and the relative configuration of the ligand, which is crucial for XuPhos to act as a monodentate P-ligand for iridium.
Introduction
Asymmetric hydrogenation catalyzed by chiral transition metal complexes contributes significantly to the synthesis of chiral compounds because of its perfect atom economy, high efficiency, and operational simplicity.1 Over the past several decades, an abundance of chiral ligands has been developed, owing to their essential functions in asymmetric hydrogenations.2 While there have been enormous efforts focused on the development of chiral P-containing bidentate and tridentate ligands, only a few chiral monodentate phosphorus ligands have been developed for asymmetric hydrogenation.2 Nonetheless, chiral monophosphorus ligands were the first ligands used for asymmetric hydrogenation. In 1972, Knowles reported the monophosphine ligand CAMP, which promoted Rh-catalyzed asymmetric hydrogenation, achieving up to 88% ee.3 Around 2000, BINOL-derived monophosphorus ligands were developed and immediately became a privileged class of chiral ligands (Scheme 1a).4 Different biphenolic skeletons and the Y groups have been introduced into these types of chiral ligands to promote different asymmetric reactions, including hydrogenation reactions.5 However, the development of CAMP-type monophosphine ligands for asymmetric hydrogenation has encountered considerable challenges, possibly due to the difficulty in the preparation of P-stereogenic compounds.6
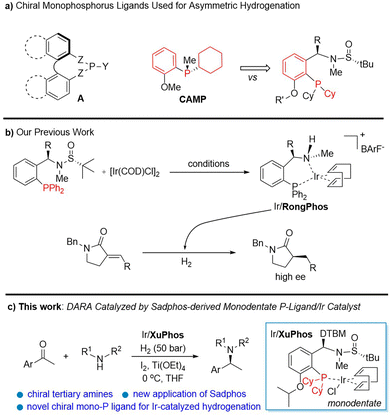 |
| Scheme 1 Sadphos ligands for Ir-catalyzed asymmetric hydrogenation. | |
On the other hand, the asymmetric hydrogenation of ketimines (including ketiminiums) is one of the most widely used methods for the preparation of chiral amines, owing to its high efficiency and good atom-economy.7 Among the methods for the asymmetric hydrogenation of ketimines, direct asymmetric reductive amination (DARA) is a step economical method that is highly attractive to academia and industry because it allows for one-pot synthesis that eliminates the need for ketimine isolation. Many protocols have been reported for the DARA reaction using ammonium salts or primary amines as the coupling partner,8 but the use of secondary amines that could give valuable chiral tertiary amines has been relatively sparse. It should be noted that a successful study has been reported by the Chang group, wherein a phosphoramidite ligand/Ir catalytic system was employed to realize the DARA reaction using secondary amines to afford chiral tertiary amines with good yields and enantioselectivites.9,10
Recently, we have developed the Sadphos family ligands, which feature a good chiral environment, ready accessibility, ease of modification, and relatively good stability.11 It has been revealed that Sadphos ligands can act as monodentate or bidentate P-ligands to promote a wide range of metal-catalyzed asymmetric catalyses.12 Following this success, we were also interested in the application of such ligands in asymmetric hydrogenations. As shown in Scheme 1b, upon the coordination of MingPhos (a Sadphos-derived ligand) with the iridium salt, P,N/Ir complexes could be generated selectively, catalyzing the asymmetric hydrogenation of α,β-unsaturated carbonyl compounds with excellent results.13 We noticed that one of the sub-classes of Sadphos, XuPhos, bears a part of the skeleton of CAMP (Scheme 1a). Therefore, we wondered whether XuPhos can be utilized as a monodentate phosphine ligand to promote asymmetric hydrogenations. Herein, we report for the first time that Sadphos can function as a monodentate phosphine ligand to coordinate with iridium. The resultant complex could catalyze the DARA reaction of secondary amines to give α-chiral tertiary amines with good yields and enantioselectivities (Scheme 1c).
Results and discussion
In the beginning, we tested different Sadphos ligands via in situ coordination with an Ir salt using 1a and 2a as the substrates (Scheme 2). Ti(OEt)4 was used to promote the in situ condensation between the amine and ketone and the additive I2 was used based on the proposed oxidation of Ir(I) by I2 to generate an active Ir(III) complex.9,14 Firstly, we tested ligands with different substituents on the P-atom (entries 1–3). The results revealed that the P–Cy group is important for the enantio-inducing ability and catalytic activity of the NMe-XuPhos ligand (NMe-Xu1) in this reaction, since the ligand NMe-XiangPhos with a P-adamantyl group showed almost no enantioselectivity and NMe-MingPhos showed no activity. Next, we tested NMe-XuPhos ligands bearing different substituents on the carbon stereocenter. However, no improvement of the ee was observed (as shown by the results using NMe-Xu2). The opposite configuration of the stereogenic carbon in NMe-Xu3 with respect to NMe-Xu1 led to a lower ee (entry 5). In our previous work, the side arm ortho to PR2 on the benzene ring plays an important role in the improvement of the ee in Pd-catalyzed cyclizations.15,16 Therefore, we next tested the effect of the side arm of NMe-XuPhos on this DARA reaction. An obvious influence on the chiral-inducing ability of the ligand based on the bulkiness of the side arm ortho to the PCy2 of NMe-XuPhos was realized (entries 6, 9 and 10). Moreover, the size of the aryl substituent on the stereogenic carbon also had a significant impact on the enantioselectivity (entries 6–8). NMe-Xu6 bearing the OiPr and DTBM groups gave the best collective impact and exhibited the best ee (94%). Finally, the N–Me group was essential for the catalytic activity of these ligands since the NH-XuPhos ligand did not catalyze the reaction at all (entries 11 and 12). The Me group might prevent the formation of the sulfinamidate–iridium complex.
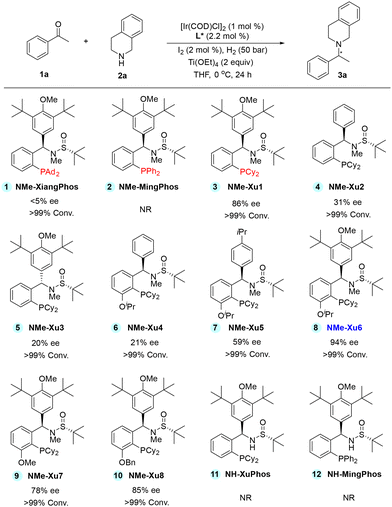 |
| Scheme 2 Screening of Sadphos family ligands. | |
Other conditions besides ligands were also screened (Table 1). Different solvents were tested using the in situ formed complex of the NMe-Xu6 ligand with [Ir(COD)Cl]2 (entries 1–7) at 60 °C. Hydrogenation in THF, EtOAc, and DCE could give 3a with full conversion and similar ee values (entries 1–3). However, aromatic solvents such as toluene and PhCF3 led to much lower enantioselectivities (entries 4 and 5). The reaction in methanol could also proceed smoothly, but no enantioselectivity was detected (entry 6). When the strong coordinating solvent MeCN was used, the reaction could also provide product 3a in a high yield with 51% ee (entry 7). The screening of different reaction temperatures (entries 1 & 8–10) revealed that 0 °C was the best temperature for this reaction (entry 9). There was no improvement of the ee when the reaction temperature was reduced further to −10 °C, but a lower conversion was observed (entry 10). Then we tested the reaction at 0 °C with 12 h reaction time, which generated the product with a lower conversion (entry 11). When NaBArF was added as an additive, it did not have any effect on this asymmetric hydrogenation.
Table 1 Optimization of reaction conditionsa,b
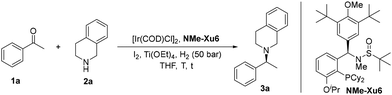
|
Entry |
Solvent |
T (°C) |
t (h) |
Conv. |
ee |
Unless otherwise noted, all reactions were carried out with 1a (0.20 mmol), 2a (0.22 mmol), [Ir(COD)Cl]2 (1.0 mol%), NMe-Xu6 (2.2 mol%), I2 (2 mol%), and Ti(OEt)4 (0.4 mmol) in solvent (2 mL) under H2 (50 bar).
The conversion was determined by 1H NMR and the ee was determined by HPLC using a chiral column.
NaBArF (2.4 mol%) was added as an additive.
|
1c |
THF |
60 |
24 |
>99% |
64 |
2 |
EtOAc |
60 |
24 |
>99% |
60 |
3 |
DCE |
60 |
24 |
>99% |
61 |
4 |
Toluene |
60 |
24 |
>99% |
30 |
5 |
PhCF3 |
60 |
24 |
>99% |
27 |
6 |
MeOH |
60 |
24 |
>99% |
∼0 |
7 |
MeCN |
60 |
24 |
>99% |
51 |
8 |
THF |
rt |
24 |
>99% |
85 |
9 |
THF |
0 |
24 |
>99% |
94 |
10 |
THF |
−10 |
24 |
78% |
94 |
11 |
THF |
0 |
12 |
66% |
94 |
12c |
THF |
0 |
24 |
>99% |
94 |
We wanted to gain insights into the key aspects that made the catalytic behaviour of NMe-XuPhos much different from that of NMe-MingPhos in this DARA reaction. Therefore, NMe-Xu1 and NMe-Xu3 were used to prepare the Ir-complex by mixing the ligand with [Ir(COD)Cl]2 in THF. Single crystal X-ray structure analysis of the NMe-Xu1/Ir complex indicated that NMe-Xu1 behaved as a chiral monodentate phosphine ligand (Scheme 3a and Fig. 1). Even in the presence of NaBArF, this complex was stable and no counterion exchange occurred. This is consistent with the experimental evidence that NaBArF had no effect on the catalytic activity and enantioselectivity of NMe-Xu1 (Table 1, entry 9 vs. 12). Interestingly, NMe-Xu3 with the opposite configuration of the stereogenic carbon formed a chiral P,O/Ir complex in the presence of NaBArF under similar conditions, where NMe-Xu3 acted as a bidentate ligand (Scheme 3b and Fig. 1). In contrast, our previous study showed that the NMe-MingPhos ligand coordinated with [Ir(COD)Cl]2 in the presence of NaBArF to generate the P,N/Ir complex by eliminating the tBuSO group (Scheme 3c).13 When these types of N-stereogenic P,N/Ir complexes (Ir-cat3 and Ir-cat4) were used in the DARA reaction (Scheme 3d), no desired tertiary amine product was formed, even at 60 °C, indicating that the P,N-coordination is substantially detrimental to the DARA reaction. On the other hand, the NMe-Xu1-Ir complex catalyzed the reaction smoothly (Scheme 3d), and the results were the same as those of the in situ formed complex (Scheme 2, entry 3). The NMe-Xu3-Ir-BArF complex was also tested for this reaction, giving the desired product with full conversion and −10% ee, which was different from the result obtained using the in situ formed complex without NaBArF (Scheme 2, entry 5, 20% ee). These results suggest that the three different coordination (P,N- vs. P,O- vs. monophosphine) behaviors of these two types of ligands (NMe-XuPhos and NMe-MingPhos) with iridium lead to very different catalytic activities for different reactions. The P–Cy group may prevent the formation of the P,N-complex by exerting specific electronic and steric effects. Together with the effect of the side arm (OiPr) vicinal to PCy2 and the aryl substituent (DTBM) on the carbon stereocenter, the NMe-Xu6 ligand provides a good chiral environment for this reaction.
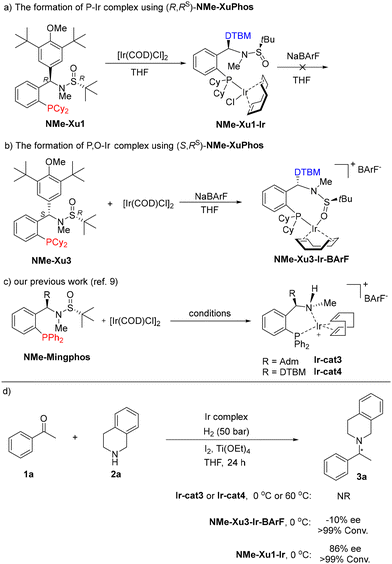 |
| Scheme 3 Different coordination and catalytic behaviours of Sadphos ligands with Ir. | |
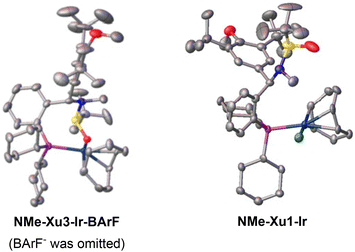 |
| Fig. 1 Single crystal X-ray diffraction structures of Ir-complexes. | |
With the optimized conditions in hand, we examined the substrate scope using NMe-Xu6 as the chiral ligand and I2 and Ti(OEt)4 as the additives, and running the reaction in THF at 0 °C for 24 h under 50 bar of H2 (Scheme 4). All the tested ketones and secondary amines underwent condensation and hydrogenation under our catalytic system, and most of the products were obtained with more than 90% ee. Electron-donating or electron-withdrawing groups on the benzene ring of the ketone substrates only had a slight impact on the enantioselectivity, while different positions of the substituent (3b–3q) only resulted in slight changes in the ee values. Notably, the ortho substituents did not show an obvious steric effect when compared with the results of the para or meta-substituted substrates (3n–3qvs.3b–3m). However, the dialkyl ketone 1r was not compatible with the chiral environment of the catalyst, giving 3r with low enantioselectivity. Next, different secondary amines were investigated. Cyclic and acyclic secondary amines were suitable coupling partners for this reaction, and the desired tertiary amines were prepared with excellent yields and good ee values (3s–3w).
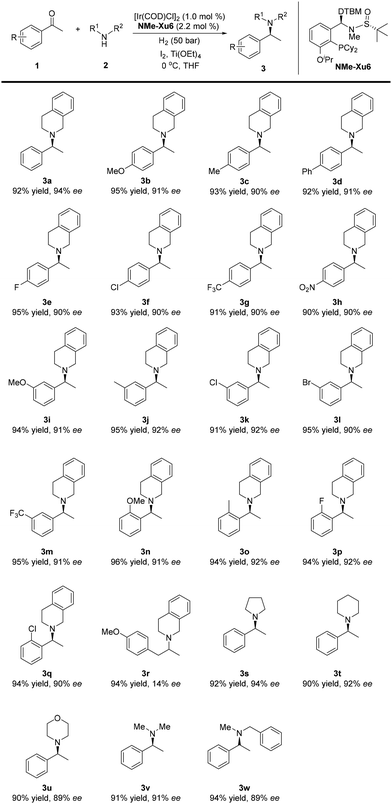 |
| Scheme 4 Substrate scope. | |
A gram-scale reaction was also carried out using the standard amine 1a and ketone 2a (Scheme 5a). Product 3a was prepared with good results, demonstrating the practical applicability of our method. To further establish the utility of this protocol, two transformations of the DARA products were carried out (Scheme 5b and c). The DARA reaction of substrate 1h with amine 2f smoothly gave the tertiary amine 3x, which was directly used for the next deprotection step to yield the chiral secondary amine 4 with good results. Rivastigmine 5, a drug used for the treatment of dementia associated with Alzheimer's and Parkinson's diseases and Lewy body dementia, was synthesized using 1t as the starting material. It reacted with dimethylamine under our conditions to yield 3y, which was directly used without purification for the next step. Thereafter, rivastigmine 5 was obtained in 85% yield with 90% ee over two steps.
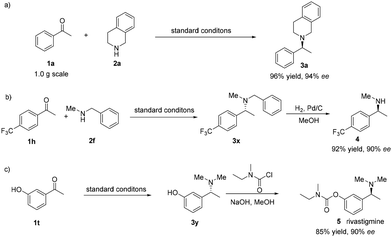 |
| Scheme 5 Synthetic applications of chiral tertiary amine products. | |
Conclusions
In summary, we have developed a new asymmetric iridium catalytic system with the chiral monodentate phosphine ligand NMe-XuPhos for the direct asymmetric reductive amination of ketones with secondary amines, yielding chiral tertiary amines in excellent yields with good ee values. The side arm (OiPr) and substituent (DTBM) on the carbon stereocenter enabled the NMe-Xu6 ligand to provide a good chiral environment for this reaction.
Data availability
The data supporting this article have been included as part of the ESI.†
Crystallographic data for NMe-Xu3-Ir-BArF and NMe-Xu1-Ir have been deposited at the CCDC under 2328618 (NMe-Xu3-Ir-BArF) and 2371593 (NMe-Xu1-Ir)† and can be obtained from https://www.ccdc.cam.ac.uk/deposit.
Conflicts of interest
There are no conflicts to declare.
Acknowledgements
We gratefully acknowledge the funding support from the National Key R&D Program of China (2021YFF0701600), the NSFC (22031004, 21871088, and 22371073), and the Shanghai Municipal Education Commission (20212308).
References
-
(a) A. Pfaltz, J. Blankenstein, R. Hilgraf, E. Hörmann, S. McIntyre, F. Menges, M. Schönleber, S. P. Smidt, B. Wüstenberg and N. Zimmermann, Iridium-Catalyzed Enantioselective Hydrogenation of Olefins, Adv. Synth. Catal., 2003, 345, 33–43 CrossRef CAS
;
(b) X. Cui and K. Burgess, Catalytic Homogeneous Asymmetric Hydrogenations of Largely Unfunctionalized Alkenes, Chem. Rev., 2005, 105, 3272–3296 CrossRef CAS PubMed
;
(c) J.-H. Xie and Q.-L. Zhou, New Progress and Prospects of Transition Metal-Catalyzed Asymmetric Hydrogenation, Acta Chim. Sin., 2012, 70, 1427–1438 CrossRef CAS
;
(d) D.-S. Wang, Q.-A. Chen, S.-M. Lu and Y.-G. Zhou, Asymmetric Hydrogenation of Heteroarenes and Arenes, Chem. Rev., 2012, 112, 2557–2590 CrossRef CAS PubMed
;
(e) J.-H. Xie, S.-F. Zhu and Q.-L. Zhou, Recent advances in transition metal-catalyzed enantioselective hydrogenation of unprotected enamines, Chem. Soc. Rev., 2012, 41, 4126–4139 RSC
;
(f) J. J. Verendel, O. Pamies, M. Dieguez and P. G. Andersson, Asymmetric Hydrogenation of Olefins Using Chiral Crabtree-type Catalysts: Scope and Limitations, Chem. Rev., 2014, 114, 2130–2169 CrossRef CAS PubMed
;
(g) Z. Zhang, N. A. Butt and W. Zhang, Asymmetric Hydrogenation of Nonaromatic Cyclic Substrates, Chem. Rev., 2016, 116, 14769–14827 CrossRef CAS
;
(h) T. Ayad, P. Phansavath and V. R. Vidal, Transition-Metal-Catalyzed Asymmetric Hydrogenation and Transfer Hydrogenation: Sustainable Chemistry to Access Bioactive Molecules, Chem. Rec., 2016, 16, 2754–2771 CrossRef
;
(i) S.-F. Zhu and Q.-L. Zhou, Iridium-Catalyzed Asymmetric Hydrogenation of Unsaturated Carboxylic Acids, Acc. Chem. Res., 2017, 50, 988–1001 CrossRef CAS PubMed
;
(j) H. Wang, J. Wen and X. Zhang, Chiral Tridentate Ligands in Transition Metal-Catalyzed Asymmetric Hydrogenation, Chem. Rev., 2021, 121, 7530–7567 CrossRef CAS PubMed
;
(k) Y.-M. He, Y.-Z. Cheng, Y. Duan, Y.-D. Zhang, Q.-H. Fan, S.-L. You, S. Luo, S.-F. Zhu, X.-F. Fu and Q.-L. Zhou, Recent Progress of Asymmetric Catalysis from a Chinese Perspective, CCS Chem., 2023, 5, 2685–2716 CrossRef CAS
.
- For reviews, see:
(a) G. Helmchen and A. Pfaltz, Phosphinooxazolines A New Class of Versatile, Modular P,N-Ligands for Asymmetric Catalysis, Acc. Chem. Res., 2000, 33, 336–345 CrossRef CAS PubMed
;
(b) W. Tang and X. Zhang, New Chiral Phosphorus Ligands for Enantioselective Hydrogenation, Chem. Rev., 2003, 103, 3029–3070 CrossRef CAS PubMed
;
(c) J.-H. Xie and Q.-L. Zhou, Chiral Diphosphine and Monodentate Phosphorus Ligands on a Spiro Scaffold for Transition-Metal-Catalyzed Asymmetric Reactions, Acc. Chem. Res., 2008, 41, 581–593 CrossRef CAS PubMed
;
(d) G. C. Hargaden and P. J. Guiry, Recent Applications of Oxazoline-Containing Ligands in Asymmetric Catalysis, Chem. Rev., 2009, 109, 2505–2550 CrossRef CAS
;
(e) Y.-N. Yu and M.-H. Xu, Design of a new series of chiral phosphite–olefin ligands and their application in asymmetric catalysis, Org. Chem. Front., 2014, 1, 738–741 RSC
;
(f) T. Imamoto, Searching for Practically Useful P-Chirogenic Phosphine Ligands, Chem. Rec., 2016, 16, 2659–2673 CrossRef PubMed
;
(g) Y. Liu, W. Li and J. Zhang, Chiral ligands designed in China, Natl. Sci. Rev., 2017, 4, 326–358 CrossRef CAS
;
(h) S. Otocka, M. Kwiatkowska, L. Madalińska and P. Kiełbasiński, Chiral Organosulfur Ligands/Catalysts with a Stereogenic Sulfur Atom: Applications in Asymmetric Synthesis, Chem. Rev., 2017, 117, 4147–4181 CrossRef CAS PubMed
;
(i) F. Yang, J.-H. Xie and Q.-L. Zhou, Highly Efficient Asymmetric Hydrogenation Catalyzed by Iridium Complexes with Tridentate Chiral Spiro Aminophosphine Ligands, Acc. Chem. Res., 2023, 56, 332–349 CrossRef CAS PubMed
;
(j) Y. Nie, Q. Yuan and W. Zhang, Axis-Unfixed Biphenylphosphine-Oxazoline Ligands: Design and Applications in Asymmetric Catalytic Reactions, Chem. Rec., 2023, 23, e202300133 CrossRef CAS PubMed
. For selected recent papers, see:
(k) X. Wu, H.-T. Yue, X.-D. Zuo, X.-H. Yang, P.-C. Yan, J.-H. Xie and Q.-L. Zhou, Kinetic Resolution of Racemic 4-Substituted Chroman-2-ones Through Asymmetric Lactone Hydrogenation, CCS Chem., 2024, 6, 2560–2576 CrossRef
;
(l) G.-Q. Chen, J.-M. Huang, B.-J. Lin, C. Shi, L.-Y. Zhao, B.-D. Ma, X.-B. Ding, Q. Yin and X. Zhang, Highly Enantioselective Hydrogenation of tetra- and tri-Substituted α,β-Unsaturated Carboxylic Acids with oxa-Spiro Diphosphine Ligands, CCS Chem., 2020, 2, 468–477 CrossRef CAS
;
(m) G. Dahiya, M. Pappoppula and A. Aponick, Configuration Sampling With Five-Membered Atropisomeric P,N-Ligands, Angew. Chem., Int. Ed., 2021, 60, 19604–19608 CrossRef CAS PubMed
;
(n) Q. Meng, Y. Meng, Q. Liu, B. Yu, Z.-J. Li, E.-Q. Li and J. Zhang, Enantioselective Synthesis of Oxazocines via MQ-Phos Enabled Palladium-Catalyzed Asymmetric Formal [4 + 4]-Cycloadditions, Adv. Sci., 2024, 2402170 CrossRef CAS
.
- W. S. Knowles, M. J. Sabacky and B. D. Vineyard, Catalytic asymmetric hydrogenation, J. Chem. Soc., Chem. Commun., 1972, 10–11 RSC
.
- B. L. Feringa, Phosphoramidites: Marvellous Ligands in Catalytic Asymmetric Conjugate Addition, Acc. Chem. Res., 2000, 33, 346–353 CrossRef CAS PubMed
.
-
(a) S. Gladiali, E. Alberico, K. Junge and M. Beller, BINEPINES: chiral binaphthalene-core monophosphepine ligands for multipurpose asymmetric catalysis, Chem. Soc. Rev., 2011, 40, 3744–3763 RSC
;
(b) W. Fu and W. Tang, Chiral Monophosphorus Ligands for Asymmetric Catalytic Reactions, ACS Catal., 2016, 6, 4814–4858 CrossRef CAS
.
- For some representative chiral monophosphine ligands, see:
(a) T. Hayashi, Chiral Monodentate Phosphine Ligand MOP for Transition-Metal-Catalyzed Asymmetric Reactions, Acc. Chem. Res., 2000, 33, 354–362 CrossRef CAS
;
(b) F. Lagasse and H. B. Kagan, Chiral Monophosphines as Ligands for Asymmetric Organometallic Catalysis, Chem. Pharm. Bull., 2000, 48, 315–324 CrossRef CAS
;
(c) G. Xu, C. H. Senanayake and W. Tang, P-Chiral, Phosphorus Ligands Based on a 2,3-Dihydrobenzo[d,][1,3]oxaphosphole Motif for Asymmetric Catalysis, Acc. Chem. Res., 2019, 52, 1101–1112 CrossRef CAS
;
(d) Also see ref. 6a.
-
(a) Z. Yu, W. Jin and Q. Jiang, Brønsted Acid Activation Strategy in Transition-Metal Catalyzed Asymmetric Hydrogenation of N-Unprotected Imines, Enamines, and N-Heteroaromatic Compounds, Angew. Chem., Int. Ed., 2012, 51, 6060–6072 CrossRef CAS
;
(b) T.-X. Fan and Y. Liu, Recent Advances in Synthesis of Chiral Tertiary Amines via Asymmetric Catalysis Involving Metal-Hydride Species, Chin. J. Org. Chem., 2022, 42, 3280–3294 CrossRef CAS
;
(c) J. B. Rivera, Y. Xu, M. Wills and V. Vyas, Recent Advances in the Enantioselective Synthesis of Chiral Amines via Transition Metal-Catalyzed Asymmetric Hydrogenation, Chem. Rev., 2022, 122, 269–339 CrossRef
;
(d) Z. Zhang, N. Butt, M. Zhou, D. Liu and W. Zhang, Asymmetric Transfer and Pressure Hydrogenation with Earth-Abundant Transition Metal Catalysts, Chin. J. Chem., 2018, 36, 443–454 CrossRef CAS
.
- For reviews, see:
(a) O. Afanasyev, E. Kuchuk, D. Usanov and D. Chusov, Reductive Amination in the Synthesis of Pharmaceuticals, Chem. Rev., 2019, 119, 11857–11911 CrossRef CAS
;
(b) Y. Tian, L. Hu, Y.-Z. Wang, X. Zhang and Q. Yin, Recent advances on transition-metal-catalysed asymmetric reductive amination, Org. Chem. Front., 2021, 8, 2328–2342 RSC
;
(c) T. Irrgang and R. Kempe, Transition-Metal-Catalyzed Reductive Amination Employing Hydrogen, Chem. Rev., 2020, 120, 9583–9674 CrossRef CAS PubMed
. For related papers, see:
(d) M. Chang, S. Liu, K. Huang and X. Zhang, Direct Catalytic Asymmetric Reductive Amination of Simple Aromatic Ketones, Org. Lett., 2013, 15, 4354–4357 CrossRef CAS PubMed
;
(e) R. Liu, J. Han, B. Li, X. Liu, Z. Wei, J. Wang, Q. Wang, R. Jiang, H. Nie and S. Zhang, Enantioselective synthesis of tetrahydroisoquinolines via catalytic intramolecular asymmetric reductive amination, Org. Chem. Front., 2021, 8, 1930–1934 RSC
;
(f) Y. Shi, J. Wang, F. Yang, C. Wang, X. Zhang, P. Chiu and Q. Yin, Direct asymmetric reductive amination of α-keto acetals: a platform for synthesizing diverse a-functionalized amines, Chem. Commun., 2022, 58, 513–516 RSC
;
(g) T. Ghosh, M. Ernst, A. Hashmi and T. Schaub, Ruthenium Catalyzed Direct Asymmetric Reductive Amination of Simple Aliphatic Ketones Using Ammonium Iodide and Hydrogen, Eur. J. Org. Chem., 2020, 4796–4800 CrossRef CAS
;
(h) H. Huang, Y. Zhao, Y. Yang, L. Zhou and M. Chang, Direct Catalytic Asymmetric Reductive Amination of Aliphatic Ketones Utilizing Diphenylmethanamine as Coupling Partner, Org. Lett., 2017, 19, 1942–1945 CrossRef CAS
;
(i) T. Kawada, K. Yabushita, T. Yasuda, T. Ohta, T. Yajima, K. Tanaka, N. Utsumi, M. Watanabe, K. Murata, Y. Kayaki, S. Kuwata and T. Katayama, Asymmetric Transfer Hydrogenative Amination of Benzylic Ketones Catalyzed by Cp*Ir(III) Complexes Bearing a Chiral N-(2- Picolyl)sulfonamidato Ligand, J. Org. Chem., 2022, 87, 8458–8468 CrossRef CAS
;
(j) S. Zhang, F. Chen, Y.-M. He and Q.-H. Fan, Asymmetric Hydrogenation of Dibenzo[c,e]azepine Derivatives with Chiral Cationic Ruthenium Diamine Catalysts, Org. Lett., 2019, 21, 5538–5541 CrossRef CAS PubMed
;
(k) N. Reshi, V. Saptal, M. Beller and J. Bera, Recent Progress in Transition-Metal-Catalyzed Asymmetric Reductive Amination, ACS Catal., 2021, 11, 13809–13837 CrossRef CAS
;
(l) S. Yuan, G. Gao, L. Wang, C. Liu, L. Wan, H. Huang, H. Geng and M. Chang, The combination of asymmetric hydrogenation of olefins and direct reductive amination, Nat. Commun., 2020, 11, 621 CrossRef CAS
;
(m) Z. Wu, W. Wang, H. Guo, G. Gao, H. Huang and M. Chang, Iridium-catalyzed direct asymmetric reductive amination utilizing primary alkyl amines as the N-sources, Nat. Commun., 2022, 13, 3344 CrossRef CAS PubMed
;
(n) T. Thorpe, J. Marshall, V. Harawa, R. Ruscoe, A. Cuetos, J. Finnigan, A. Angelastro, R. Heath, F. Parmeggiani, S. Charnock, R. Howard, R. Kumar, D. Daniels, G. Grogan and N. Turner, Multifunctional biocatalyst for conjugate reduction and reductive amination, Nature, 2022, 604, 86–91 CrossRef CAS
;
(o) T. Yasukawa, R. Masuda and S. Kobayashi, Development of heterogeneous catalyst systems for the continuous synthesis of chiral amines via asymmetric hydrogenation, Nat. Catal., 2019, 2, 1088–1092 CrossRef CAS
;
(p) M. Yamada, K. Azuma and M. Yamano, Highly Enantioselective Direct Asymmetric Reductive Amination of 2-Acetyl-6-Substituted Pyridines, Org. Lett., 2021, 23, 3364–3367 CrossRef CAS PubMed
;
(q) M. Yamada, K. Murai, K. Yamaguchi and M. Yamano, Ruthenium-Catalyzed Direct Asymmetric Reductive Amination for the Synthesis of a Chiral Primary Amine Intermediate EnRoute to a PDE2A Inhibitor, TAK-915, Org. Process Res. Dev., 2023, 27, 972–981 CrossRef CAS
;
(r) Y. Zhang, Y.-Q. Liu, L. Hu, X. Zhang and Q. Yin, Asymmetric Reductive
Amination/Ring-Closing Cascade: Direct Synthesis of Enantioenriched Biaryl-Bridged NH Lactams, Org. Lett., 2020, 22, 6479–6483 CrossRef CAS
;
(s) H. Huang, X. Liu, L. Zhou, M. Chang and X. Zhang, Direct Asymmetric Reductive Amination for the Synthesis of Chiral β-Arylamines, Angew. Chem., Int. Ed., 2016, 55, 5309–5312 CrossRef CAS
;
(t) Z. Gao, J. Liu, H. Huang, H. Geng and M. Chang, An Iridium Catalytic System Compatible with Inorganic and Organic Nitrogen Sources for Dual Asymmetric Reductive Amination Reactions, Angew. Chem., Int. Ed., 2021, 60, 27307–27311 CrossRef CAS PubMed
;
(u) W. Zhao, W. Wang, H. Zhou, Q. Liu, Z. Ma, H. Huang and M. Chang, An Asymmetric Hydrogenation/N-Alkylation Sequence for a Step-Economical Route to Indolizidines and Quinolizidines, Angew. Chem., Int. Ed., 2023, 62, e202308836 CrossRef CAS
;
(v) Y. Lou, Y. Hu, J. Lu, F. Guan, G. Gong, Q. Yin and X. Zhang, Dynamic Kinetic Asymmetric Reductive Amination: Synthesis of Chiral Primary β-Amino Lactams, Angew. Chem., Int. Ed., 2018, 57, 14193–14197 CrossRef CAS
;
(w) Z. Gao, J. Liu, H. Huang, H. Geng and M. Chang, An Iridium Catalytic System Compatible with Inorganic and Organic Nitrogen Sources for Dual Asymmetric Reductive Amination Reactions, Angew. Chem., 2021, 133, 27513–27517 CrossRef
;
(x) Y. Gao, Z. Wang, X. Zhang, M. Zhao, S. Zhang, C. Wang, L. Xu and P. Li, Additive-Free Transfer Hydrogenative Direct Asymmetric Reductive Amination Using a Chiral Pyridine-Derived Half-Sandwich Catalyst, Angew. Chem., Int. Ed., 2023, 62, e202303709 CrossRef
;
(y) Z. Wang, X.-Y. Yang, Y. Xu and Q.-L. Zhou, Iridium-Catalyzed Asymmetric Hydrogenation of Dialkyl Imines, CCS Chem., 2024, 6, 905–911 CrossRef CAS
;
(z) G. Liu, L. Zheng, K. Tian, H. Wang, L. W. Chung, X. Zhang and X.-Q. Dong, Ir-Catalyzed Asymmetric Hydrogenation of Unprotected Indoles: Scope Investigations and Mechanistic Studies, CCS Chem., 2023, 5, 1398–1410 CrossRef CAS
;
(a
a) Y. Chi, Y.-G. Zhou and X. Zhang, Highly Enantioselective Reductive Amination of Simple Aryl Ketones Catalyzed by Ir−f-Binaphane in the Presence of Titanium(IV) Isopropoxide and Iodine, J. Org. Chem., 2003, 68, 4120–4122 CrossRef CAS
;
(a
b) L.-C. Yang, Y.-N. Wang, Y. Zhang and Y. Zhao, Acid-Assisted Ru-Catalyzed Enantioselective Amination of 1,2-Diols through Borrowing Hydrogen, ACS Catal., 2017, 7, 93–97 CrossRef CAS
;
(a
c) Y. Zhang, C. S. Lim, D. S. B. Sim, H. J. Pan and Y. Zhao, Catalytic Enantioselective Amination of Alcohols by the Use of Borrowing Hydrogen Methodology: Cooperative Catalysis by Iridium and a Chiral Phosphoric Acid, Angew. Chem., Int. Ed., 2014, 53, 1399–1403 CrossRef CAS PubMed
.
- Z. Wu, S. Du, G. Gao, W. Yang, X. Yang, H. Huang and M. Chang, Secondary amines as coupling partners in direct catalytic asymmetric reductive amination, Chem. Sci., 2019, 10, 4509–4514 RSC
.
- For other reports using complex secondary amines or in situ generated secondary amines, see:
(a) Y. Chen, Y.-M. He, S. Zhang, T. Miao and Q.-H. Fan, Rapid Construction of Structurally Diverse Quinolizidines, Indolizidines, and Their Analogues via Ruthenium-Catalyzed Asymmetric Cascade Hydrogenation/Reductive Amination, Angew. Chem., Int. Ed., 2019, 58, 3809–3813 CrossRef CAS
;
(b) W. Zhao, W. Wang, H. Zhou, Q. Liu, Z. Ma, H. Huang and M. Chang, An Asymmetric Hydrogenation/N-Alkylation Sequence for a Step-Economical Route to Indolizidines and Quinolizidines, Angew. Chem., Int. Ed., 2023, 62, e202308836 CrossRef CAS
;
(c) W. Li, S. Zhang, X. Yu, X. Feng, Y. Yamamoto and M. Bao, Chiral Indolizidine Synthesis through the Ir-Catalyzed Asymmetric
Hydrogenation of Cyclic Pyridinium Salts, J. Org. Chem., 2021, 86, 10773–10781 CrossRef CAS
;
(d) L.-R. Wang, D. Chang, Y. Feng, Y.-M. He, G.-J. Deng and Q.-H. Fan, Highly Enantioselective Ruthenium-Catalyzed Cascade Double Reduction Strategy: Construction of Structurally Diverse Julolidines and Their Analogues, Org. Lett., 2020, 22, 2251–2255 CrossRef CAS
;
(e) R. Xie, H. Zhou, H. Lu, Y. Mu, G. Xu and M. Chang, Transition-Metal-Catalyzed Asymmetric Reductive Amination and Amidation Cascade Reaction for the Synthesis of Piperazinones, Org. Lett., 2022, 24, 9033–9037 CrossRef CAS
.
- W. Li and J. Zhang, Sadphos as Adaptive Ligands in Asymmetric Palladium Catalysis, Acc. Chem. Res., 2024, 57, 489–513 CAS
.
-
(a) Z.-M. Zhang, P. Chen, W. Li, Y. Niu, X.-L. Zhao and J. Zhang, A New Type of Chiral Sulfinamide Monophosphine Ligands: Stereodivergent Synthesis and Application in Enantioselective Gold(I)-Catalyzed Cycloaddition Reactions, Angew. Chem., Int. Ed., 2014, 53, 4350–4354 CrossRef CAS PubMed
;
(b) Z. M. Zhang, B. Xu, Y. Qian, L. Wu, Y. Wu, L. Zhou, Y. Liu and J. Zhang, Palladium-Catalyzed Enantioselective Reductive Heck Reactions: Convenient Access to 3,3-Disubstituted 2,3-Dihydrobenzofuran, Angew. Chem., Int. Ed., 2018, 57, 10373–10377 CrossRef CAS
;
(c) Y. Wang, L. Wang, M. Chen, Y. Tu, Y. Liu and J. Zhang, Palladium/Xu-Phos-catalyzed asymmetric carboamination towards isoxazolidines and pyrrolidines, Chem. Sci., 2021, 12, 8241–8245 RSC
;
(d) W. Li and J. Zhang, Sadphos as Adaptive Ligands in Asymmetric Palladium Catalysis, Acc. Chem. Res., 2024, 57, 489–513 CAS
.
- R. Zhang, S. Xu, Z. Luo, Y. Liu and J. Zhang, Enantiodivergent Hydrogenation of Exocyclic α,β-Unsaturated Lactams Enabled by Switching the N-Chirality of Iridium Catalyst, Angew. Chem., Int. Ed., 2023, 62, e202213600 CrossRef CAS
.
- D. Xiao and X. Zhang, Highly Enantioselective Hydrogenation of Acyclic Imines Catalyzed by Ir–f-Binaphane Complexes, Angew. Chem., Int. Ed., 2001, 40, 3425–3428 CrossRef CAS
.
-
(a) Y. Wang, L. Wang, M. Chen, Y. Tu, Y. Liu and J. Zhang, Palladium/Xu-Phos-catalyzed asymmetric carboamination towards isoxazolidines and pyrrolidines, Chem. Sci., 2021, 12, 8241–8245 RSC
;
(b) S. Zhu, Z. Ye, M. J. Chen, L. Wang, Y. Z. Wang, K. N. Zhang, W. B. Li, H. M. Ding, Z. Li and J. Zhang, Mechanistic study on the side arm effect in a palladium/Xu-Phos-catalyzed enantioselective alkoxyalkenylation of γ-hydroxyalkenes, Nat. Commun., 2023, 14, 7611 CrossRef CAS PubMed
;
(c) S. Zhu, M. Chen, H. Shen, H. Ding, W. Li and J. Zhang, Palladium/Xu-Phos-catalyzed enantioselective arylalkoxylation reaction of γ-hydroxyalkenes at room temperature, Chin. Chem. Lett., 2024, 109879 CrossRef CAS
.
- S. Liao, X.-L. Sun and Y. Tang, Side Arm Strategy for Catalyst Design: Modifying Bisoxazolines for Remote Control of Enantioselection and Related, Acc. Chem. Res., 2014, 47, 2260–2272 CrossRef CAS
.
Footnotes |
† Electronic supplementary information (ESI) available. CCDC 2328618 and 2371593. For ESI and crystallographic data in CIF or other electronic format see DOI: https://doi.org/10.1039/d4qo01495b |
‡ These authors contributed equally to this work. |
|
This journal is © the Partner Organisations 2024 |
Click here to see how this site uses Cookies. View our privacy policy here.