DOI:
10.1039/D4QO01522C
(Research Article)
Org. Chem. Front., 2024,
11, 7037-7043
δ-Amination of alkyl alcohols via energy transfer photocatalysis†
Received
16th August 2024
, Accepted 8th October 2024
First published on 10th October 2024
Abstract
Amino alcohols play a crucial role in the realm of biologically active compounds due to their functional diversity. In this study, we introduce a metal-free energy transfer photocatalytic method for the preparation of 1,4-aminoalcohols from readily available alcohol feedstocks. The key feature of this transformation is the simultaneous generation of a persistent iminyl radical and a transient carbon-centered radical through σ-homolytic cleavage of the O–N bond via energy transfer (EnT). The process involves fragmentation/decarboxylation/1,5-HAT (hydrogen atom transfer), leading to the formation of a C(sp3)-hybridized radical, which undergoes selective radical–radical cross-coupling or a radical chain event to yield the desired products. In addition, the protocol was also found to be suitable for N-tosyl amines, giving rise to 1,4-diamines. Our approach combines experimental mechanistic investigations with detailed computational studies using density functional theory (DFT) to provide insights into the reaction mechanism. This innovative method provides a new approach for preparing δ-amino alkyl alcohols and amines.
Introduction
The generation of radicals under mild visible light photocatalyzed conditions has emerged as a powerful tool that has expanded the repertoire of synthetic transformations, enabling new ways to access complex molecules.1 For instance, the functionalization of a carbon centered radical generated by 1,5-hydrogen atom transfer (HAT) from a heteroatom radical is exceptionally interesting since it allows remote selective functionalization of inert C–H bonds.2 Consequently, diverse approaches have been developed for this remote functionalization (Scheme 1A).3
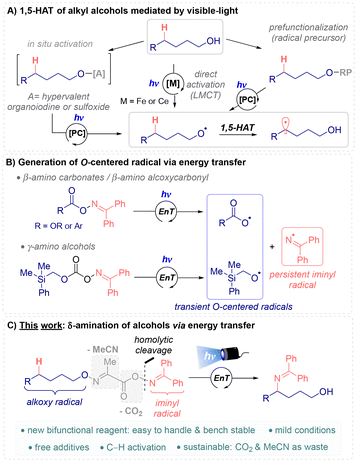 |
| Scheme 1 (A) Different visible-light-assisted strategies to generate δ- C-centered radical from alkyl alcohols. (B) Bifunctional reagents to achieve O-centered radicals by energy transfer photocatalysis. (C) Merging of alkoxy radical reactivity with energy transfer activation. | |
The most common strategy involves the preactivation of the O–H bond using a redox auxiliary (Scheme 1A, O-RP, RP = radical precursor) such as N-alkoxyphthalimides,4N-alkoxypyridinium salts5 or α-oxime acids or esters,6 among others. These radical precursors are easy to prepare and produce the desired alkoxy radical, and consequently the δ-C(sp3)-centered radical, under photoredox conditions. Strategies for the formation of alkoxy radicals from free alcohols have also been recently developed using stoichiometric amounts of organohypervalent iodine7a–c or sulfoxide reagents7d (Scheme 1A, O–[A]), coordination of the alcohol with metals by ligand-to-metal charge transfer (LMCT, Ce or Fe catalyst)8 or direct hydrogen atom transfer of the O–H bond by proton coupled electron transfer (PCET).9 However, to the best of our knowledge, methods to functionalize δ-C(sp3)–H bonds of aliphatic alcohols via energy transfer (EnT) mechanism have not yet been reported.
Recently, different research groups have described the simultaneous generation of a persistent iminyl radical and a transient O-centered radical via σ-homolytic bond cleavage through EnT activation (Scheme 1B).10 In 2021, the groups of Glorius11 and Han12 independently reported the generation of both alkoxycarbonyloxyl radicals from oxime carbonates to synthesize 1,2-amino carbonates (Scheme 1B, R = OR). Huo's group described the synthesis of 1,2-amino alkoxycarbonyl compounds via the formation of an alkoxycarbonyl radical intermediate from oxime esters.13 Recently, Glorius et al. reported the generation of an alkoxy radical to prepare 1,3-amino alcohols via N–O bond homolysis, followed by a radical Brook rearrangement (Scheme 1B).14
Amino alcohols are valuable compounds in organic synthesis, medicinal chemistry, and materials science. In particular, 1,4-amino alcohol subunits are present in many biologically active compounds, act as flexible building blocks in synthetic chemistry and have been used as ligands in asymmetric catalysis.15 Due to the great importance of this motif, and inspired by the inherent reactivity of the alkoxy radical and the advantages that the energy transfer presents, we envisaged a δ-imination reaction of alkyl alcohols via EnT photocatalysis (Scheme 1C). The selective insertion of the protected amino group at this remote position of an alkyl alcohol will be achieved by the use of a novel bifunctional reagent. This new reagent has to carry both the iminyl and the alkoxy fragments in its structure, must be bench stable and easy to handle, and produce minimal waste after its activation.
Results and discussion
To investigate the feasibility of the proposed δ-amination protocol from alcohols, the bifunctional reagent 1a was used as the model substrate.16 This starting material is bench stable and easily accessible from the corresponding alcohol after three synthetic steps. After screening several reaction conditions (different photocatalyst, solvent, catalyst loading, concentration and sources of light), the best results were obtained using 5CzBn (1 mol%) as the organo photocatalyst in acetone (0.1 M) under blue light irradiation (λmax = 427 nm), yielding the δ-amino alcohol 2a in 50% isolated yield (Scheme 2).16 Additionally, as part of the structural design of the new bifunctional reagent, different iminyl fragments were evaluated. The derivative from thioxanthone afforded the corresponding desired product 2b in a similar yield. However, the 9-fluorenone derivative was unreactive, with only a trace amount of 2c detected by 1H NMR analysis (Scheme 2). Given the advantages offered by reagent 1a, the synthesis of its oxime is well described and it is easy to prepare and cost effective (benzophenone ∼€0.02 per g vs. thioxanthone ∼ €0.80 per g),17 benzophenone was chosen as the optimal iminyl precursor. Control experiments performed in the absence of light or the photocatalyst confirmed that both conditions are essential to enable the δ-imination of aliphatic alcohols (Scheme 2).16 The reactions can be performed under open air conditions with similar yields being obtained.
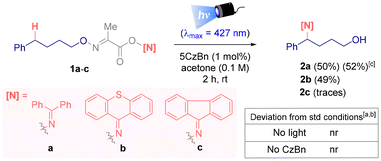 |
| Scheme 2 Structure optimization of 1 and control experiments. a Std conditions: 1 (0.10 mmol), 5CzBN (1 mol%), in dry degassed acetone (0.1 M) under blue Kessil irradiation (λmax = 427 nm) for 2 h at rt, inert atmosphere. b Starting material 1a. c Open-to-air conditions. Abbreviation: std, standard; nr, no reaction. | |
With the structure of the optimal bifunctional reagent established and the best reaction conditions in hand, the scope of this amination reaction was explored (Scheme 3A.1). The desired δ-imination product from primary alkyl alcohols, both non-functionalized (2d–g) and functionalized (2h–q), was achieved. Hence, the products resulting from a secondary C-centered radical intermediate (2e–f, 2q–s) and a non-stabilized primary C-centered radical (2d) were obtained, whereas product 2g derived from a stabilized tertiary C-centered radical intermediate was obtained in 6% yield. We hypothesized that the low yields observed in certain examples may be attributed to unfavourable pathways involving the iminyl and alkoxy radicals, as the starting alcohol was detected in most cases.18
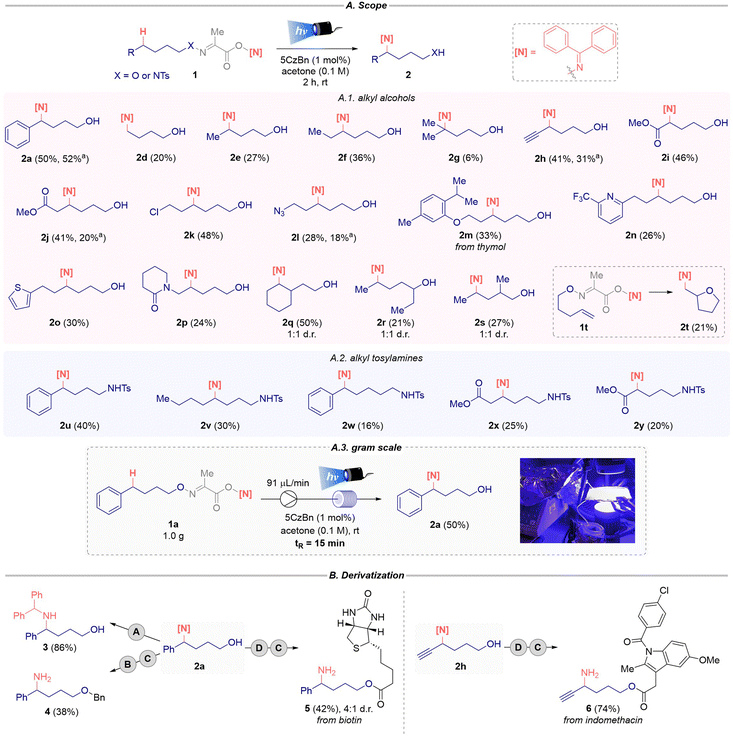 |
| Scheme 3
Substrate scope. Reactions were performed using 1 (0.20 mmol), 5CzBN (1 mol%), in dry degassed acetone (2.0 mL, 0.1 M) under blue Kessil irradiation (λmax = 427 nm) for 2 h at rt under an inert atmosphere. a Open-to-air conditions. b 35% 1H NMR yield calculated using trimethoxybenzene as internal standard. Diversification reactions.16 Conditions A: NaBH4 (25 equiv.). Conditions B: BnBr (1.1 equiv.) and NaH (1.5 equiv.). Conditions C: PPTS (1.2 equiv.). Conditions D: carboxylic acid (1.5 equiv.). | |
Terminal alkyne (2h), chlorine (2k) and azide (2l) functional groups were tolerated under the standard reaction conditions. This protocol also allowed the synthesis of α- and β-amino esters (2i and 2j) containing a free alcohol in moderate yields. The phenolic ether 2m (from thymol), electron-poor and electron-rich heterocycles (2n and 2o) and the δ-lactam moiety 2p were also suitable for this transformation (Scheme 3A.1). Using secondary alcohols, no β-fragmentation product was detected and the corresponding product 2r was obtained in 21% yield.
Interestingly, the reaction using the 4-pentenyloxy derivative 1t afforded the iminated tetrahydrofuran product 2t after an intramolecular 5-exo-trig cyclization event19 followed by imine insertion. In certain cases (2a, 2h, 2j and 2l), the reactions were conducted under open-to-air conditions, resulting in a decrease in yields, indicating that while an inert atmosphere is not strictly necessary, it is beneficial for achieving better yields. Additionally, some bifunctional reagents failed to yield the desired product 2 due to their rigid structure or the presence of bulky groups.16
Next, we investigated a Hoffman–Löffler–Freytag-type (HLF) reaction using alkyl N-tosyl amines 1 (X = NTos).20,21 The synthesis of these reagents was inspired by Yu's seminal work on the δ-halogenation of alkyl N-tosyl amines from hydrazonyl carboxylic acid precursors.22 To our delight, the desired 5-iminyl alkylamines (2u–y) were obtained under the standard conditions (Scheme 3A.2). Remarkably, the 1,6-HAT product 2w was obtained instead of the expected 1,5-HAT product. This is likely due to the easy activation of the benzylic C–H bond and the higher stability of the preceding benzylic radical intermediate. Additionally, the corresponding β- and α-amino esters 2x and 2y, bearing a pendant N-tosyl amine, were successfully prepared.
Furthermore, the scale-up of this transformation was achieved using a continuous-flow photoreactor.23 This technology enabled the complete conversion of 1.0 gram of 1a to the desired product 2a after only 5 h of irradiation (tR = 15 min), yielding 50% of the isolated product (Scheme 3A.3). This continuous flow process was optimized using 2a as the model substrate at 0.25 mmol scale, and the yield obtained under batch conditions was not improved.16
Some diversification reactions were performed using products 2a and 2h (Scheme 3B). The treatment of 2a with NaBH4 afforded the 1,4-amino alcohol 3 in good yield by reduction of the imine moiety. The 1,4-amino ether 4 was obtained via Williamson etherification followed by the hydrolysis of the imine group, while an esterification and deprotection reaction with PPTS yielded 1,4-amino esters 5 and 6 from biotin and indomethacin, respectively. These transformations showed the synthetic utility of the 1,4-imino alcohol motifs 2 in the construction of more complex molecules.
Some mechanistic investigations were carried out to shed light on the reaction mechanism. The addition of TEMPO (2,2,6,6-tetramethyl-1-piperidinyloxy) under the standard conditions completely inhibited the reaction, resulting in the complete recovery of the starting material 1a. Furthermore, the direct excitation of 1a in the absence of the photocatalyst, using a purple Kessil lamp (λmax = 390 nm), yielded the desired product 2a in 30% yield. Both results ruled out the possibility of a redox process and indicated that this transformation proceeded through an energy transfer event between the photocatalyst and substrate 1a.16
In addition, dispersion corrected density functional theory (DFT) calculations were performed. Fig. 1 shows the mechanistic energetic profile of 2a from 1a. The excited state 1a* (44.9 kcal mol−1) can be accessed by the triple-triplet state 5CzBn* (58.2 kcal mol−1). Then, the N–O homolytic cleavage happens from 1a* to TS1* with a small energy barrier (ΔΔG‡rel = 4.8 kcal mol−1), forming the persistent ambiphilic iminyl radical B and the transient O-centered radical A. The highly reactive alkoxy radical C (rel‡ 64.5 kcal mol−1 downhill in energy from 1a*) is generated after spontaneously extrusion of CO2 and MeCN via TSA. Then, C′ can undergo an irreversible 1,5-HAT event via TSC' to afford the transient δ-C(sp3)-centered radical D (downhill in energy by 14.5 kcal mol−1 from C′). Afterward, D can undergo a selective radical–radical cross coupling with the iminyl radical B to generate the 1,4-amino alcohol 2a. However, D can also follow a radical chain pathway to form 2a, as suggested by the experimentally measured quantum yield value (Φ ≈ 3) and the lower relative concentration of the iminyl radical Bversus the precursor 1a. D upon addition to reagent 1avia TSD-1a forms the radical intermediate E (rel‡ 2.3 kcal mol−1 uphill in energy from D). Finally, intermediate E affords the desired 1,4-amino alcohol 2avia TSE (energy barrier of 0.3 kcal mol−1) through the release of CO2, MeCN and C′, regenerating the cycle. These findings are in agreement with previous reports similar to this transformation14,24 and with the results of the light on/off experiment.16
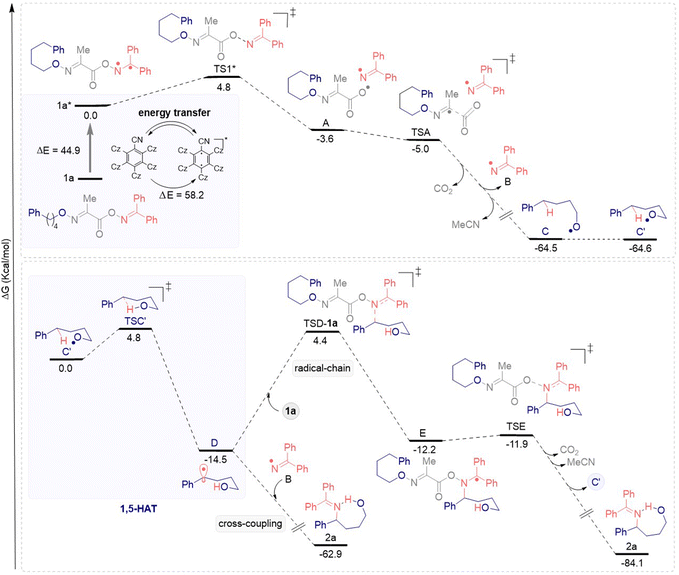 |
| Fig. 1 Proposed mechanism supported by computational studies to transform 1a into 2a. Calculated free Gibbs energy (G) values at 298 K (kcal mol−1) [CPCM(acetone) UB3LYP-D3/def2-svp].16 | |
Conclusions
In summary, an operationally simple δ-insertion of protected amines into the skeleton of aliphatic alcohols was developed from bifunctional reagents. These reagents were designed to carry both radical partners, a transient alkoxy radical and a persistent iminyl radical formed through an energy transfer (EnT) photocatalytic process. This protocol was used in the synthesis of tetrahydrofuran 2t and in the δ-amination of alkyl N-tosyl amines. The scale-up of this photoinduced transformation was conveniently realized via a continuous-flow system, maintaining reactivity and reducing reaction time compared to batch conditions. Furthermore, the synthetic potential of the constructed δ-imino alcohols was demonstrated through some derivatization reactions incorporating this motif into pharmaceuticals such as indomethacin. Although the photochemical quantum yield suggested a radical chain mechanism, its low value and computational studies do not entirely rule out the catalytic cycle pathway.
Author contributions
All authors have given approval to the final version of the manuscript.
Data availability
Experimental procedures and analytical data (NMR, MS and melting points) can be found in the ESI.† Copies of the NMR spectra are also provided.
Conflicts of interest
There are no conflicts to declare.
Acknowledgements
We thank Ministerio de Ciencia, Innovación y Universidades–Agencia Estatal de Investigación (Grants PID2023-146801NB-C32, PID2021-1248553NB-100 and PID2020-113059GB-C22), the European Union's Horizon 2020 Research and Innovation programme under Grant agreement N° 101034324 and Comunidad de Madrid (S2022/BMD-7403 RENIM−CM) for financial support. We also thanks the Centro de Computación Científica at the UAM for their generous allocation of computer time.
References
-
(a) Y. Chan, I. B. Perry, N. B. Bissonnette, B. F. Buksh, G. A. Edwards, L. I. Frye, O. L. Garry, M. N. Lavagnino, B. X. Li, Y. Liang, E. Mao, A. Millet, J. V. Oakley, N. L. Reed, H. A. Sakai, C. P. Seath and D. W. C. MacMillan, Metallaphotoredox: The Merger of Photoredox and Transition Metal Catalysis, Chem. Rev., 2022, 122, 1485–1542 CrossRef PubMed;
(b) L. Candish, K. D. Collins, G. C. Cook, J. J. Douglas, A. Gómez-Suárez, A. Jolit and S. Keess, Photocatalysis in the Life Science Industry, Chem. Rev., 2022, 122, 2907–2980 CrossRef;
(c) N. Holmberg-Douglas and D. A. Nicewicz, Photoredox-Catalyzed C–H Functionalization Reactions, Chem. Rev., 2022, 122, 1925–2016 CrossRef;
(d) J. D. Bell and J. A. Murphy, Recent advances in visible light-activated radical coupling reactions triggered by (i) ruthenium, (ii) iridium and (iii) organic photoredox agents, Chem. Soc. Rev., 2021, 50, 9540–9685 RSC;
(e) N. A. Romero and D. A. Nicewicz, Organic Photoredox Catalysis, Chem. Rev., 2016, 116, 10075–10166 CrossRef PubMed.
- Selective δ-C(sp3)–H bond functionalization of alkyl alcohols relies on the formation of a stronger O–H bond (BDE ≈105 kcal mol−1) versus an intramolecular 1,5-hydrogen atom transfer (HAT) of the weaker aliphatic C–H bond (BDE ≈98–102 kcal mol−1) by the alkoxy radical. See: L. Capaldo, D. Ravelli and M. Fagnoni, Direct Photocatalyzed Hydrogen Atom Transfer (HAT) for Aliphatic C–H Bonds Elaboration, Chem. Rev., 2022, 122, 1875–1924 CrossRef PubMed.
- Selected reviews:
(a) L. Chang, Q. An, L. Duan, K. Feng and Z. Zuo, Alkoxy Radicals See the Light: New Paradigms of Photochemical Synthesis, Chem. Rev., 2022, 122, 2429–2486 CrossRef PubMed;
(b) W. Guo, Q. Wang and J. Zhu, Visible light photoredox-catalysed remote C–H functionalisation enabled by 1,5-hydrogen atom transfer (1,5-HAT), Chem. Soc. Rev., 2021, 50, 7359–7377 RSC;
(c) E. Tsui, H. Wang and R. R. Knowles, Catalytic generation of alkoxy radicals from unfunctionalized alcohols, Chem. Sci., 2020, 11, 11124–11141 RSC.
-
(a) Y. Li, J. Zhang, D. Li and Y. Chen, Metal-Free C(sp3)–H Allylation via Aryl Carboxyl Radicals Enabled by Donor–Acceptor Complex, Org. Lett., 2018, 20, 3296–3299 CrossRef PubMed;
(b) C. Wang, K. Harms and E. Meggers, Catalytic Asymmetric Csp3–H Functionalization under Photoredox Conditions by Radical Translocation and Stereocontrolled Alkene Addition, Angew. Chem., 2016, 128, 13693–13696 CrossRef;
(c) J. Zhang, Y. Li, F. Zhang, C. Hu and Y. Chen, Generation of Alkoxyl Radicals by Photoredox Catalysis Enables Selective C(sp3)–H Functionalization under Mild Reaction Conditions, Angew. Chem., 2016, 128, 1904–1907 CrossRef.
- Some examples:
(a) X. Bao, Q. Wang and J. Zhu, Remote C(sp3)–H Arylation and Vinylation of N-Alkoxypyridinium Salts to δ-Aryl and δ-Vinyl Alcohols, Chem. – Eur. J., 2019, 25, 11630–11634 CrossRef;
(b) X. Bao, Q. Wang and J. Zhu, Dual Photoredox/Copper Catalysis for the Remote C(sp3)–H Functionalization of Alcohols and Alkyl Halides by N-Alkoxypyridinium Salts, Angew. Chem., Int. Ed., 2019, 58, 2139–2143 CrossRef;
(c) I. Kim, B. Park, G. Kang, J. Kim, H. Jung, H. Lee, M. Baik and S. Hong, Visible-Light-Induced Pyridylation of Remote C(sp3)–H Bonds by Radical Translocation of N-Alkoxypyridinium Salts, Angew. Chem., Int. Ed., 2018, 57, 15517–15522 CrossRef.
-
(a) A. N. Herron, D. Liu, G. Xia and J.-Q. Yu, δ-C–H Mono- and Dihalogenation of Alcohols, J. Am. Chem. Soc., 2020, 142, 2766–2770 CrossRef CAS;
(b) X.-L. Luo, S.-S. Li, Y.-S. Jiang, F. Liu, S.-H. Li and P.-J. Xia, Photocatalytic 1,2-Iminosulfonylation and Remote 1,6-Iminosulfonylation of Olefins, Org. Lett., 2023, 25, 1742–1747 CrossRef;
(c) Y. Zheng, Z. Liao, Z. Xie, H. Chen, K. Chen, H. Xiang and H. Yang, Photochemical Alkene Trifluoromethylimination Enabled by Trifluoromethylsulfonylamide as a Bifunctional Reagent, Org. Lett., 2023, 25, 2129–2133 CrossRef PubMed.
-
(a) Z. Liu, Y. Pan, P. Zou, H. Huang and Y. Chen, Hypervalent Iodine Reagents Enable C–H Alkynylation with Iminophenylacetic Acids via Alkoxyl Radicals, Org. Lett., 2022, 24, 5951–5956 CrossRef;
(b) G. Li, X. Hu, G. He and G. Chen, Photoredox-mediated remote C(sp3)–H heteroarylation of free alcohols, Chem. Sci., 2019, 10, 688–693 RSC;
(c) X. Wu, H. Zhang, N. Tang, Z. Wu, D. Wang, M. Ji, Y. Xu, M. Wang and C. Zhu, Metal-free alcohol-directed regioselective heteroarylation of remote unactivated C(sp3)–H bonds, Nat. Commun., 2018, 9, 3343 CrossRef PubMed;
(d) D. S. Finis and D. A. Nicewicz, Alkoxy Radical Generation Mediated by Sulfoxide Cation Radicals for Alcohol-Directed Aliphatic C–H Functionalization, J. Am. Chem. Soc., 2024, 146, 16830–16837 CrossRef PubMed.
- Some examples:
(a) Z. Jue, Y. Huang, J. Qian and P. Hu, Visible Light-Induced Unactivated δ-C(sp3)–H Amination of Alcohols Catalyzed by Iron, ChemSusChem, 2022, 15, e202201241 CrossRef PubMed;
(b) A. Hu, J. Guo, H. Pan, H. Tang, Z. Gao and Z. Zuo, δ-Selective Functionalization of Alkanols Enabled by Visible-Light-Induced Ligand-to-Metal Charge Transfer, J. Am. Chem. Soc., 2018, 140, 1612–1616 CrossRef CAS PubMed.
- Some examples:
(a) M. Wang, L. Huan and C. Zhu, Cyanohydrin-Mediated Cyanation of Remote Unactivated C(sp3)–H Bonds, Org. Lett., 2019, 21, 821–825 CrossRef CAS PubMed;
(b) X. Wu, M. Wang, L. Huan, D. Wang, J. Wang and C. Zhu, Tertiary-Alcohol-Directed Functionalization of Remote C(sp3)–H Bonds by Sequential Hydrogen Atom and Heteroaryl Migrations, Angew. Chem., Int. Ed., 2018, 57, 1640–1644 CrossRef CAS PubMed.
- Some reviews:
(a) S. Dutta, J. E. Erchinger, F. Strieth-Kalthoff, R. Kleinmans and F. Glorius, Energy transfer photocatalysis: exciting modes of reactivity, Chem. Soc. Rev., 2024, 53, 1068–1089 RSC;
(b) F. Strieth-Kalthoff, M. J. James, M. Teders, L. Pitzer and F. Glorius, Energy transfer catalysis mediated by visible light: principles, applications, directions, Chem. Soc. Rev., 2018, 47, 7190–7202 RSC.
- T. Patra, M. Das, C. G. Daniliuc and F. Glorius, Metal-free photosensitized oxyimination of unactivated alkenes with bifunctional oxime carbonates, Nat. Catal., 2021, 4, 54–61 CrossRef CAS.
- S. Lai, B. Wei, J. Wang, W. Yu and B. Han, Photocatalytic Anti-Markovnikov Radical Hydro- and Aminooxygenation of Unactivated Alkenes Tuned by Ketoxime Carbonates, Angew. Chem., Int. Ed., 2021, 60, 21997–22003 CrossRef.
- J. Li, Y. Yuan, X. Bao, T. Sang, J. Yang and C. Huo, Visible-Light-Induced Intermolecular Oxyimination of Alkenes, Org. Lett., 2021, 23, 3712–3717 CrossRef.
- R. Laskar, S. Dutta, J. C. Spies, P. Mukherjee, A. Rentería-Gómez, R. E. Thuelemann, C. G. Daniliuc, O. Gutierrez and F. Glorius, γ-Amino Alcohols via Energy Transfer Enabled Brook Rearrangement, J. Am. Chem. Soc., 2024, 146, 10899–10907 CrossRef PubMed.
- Some examples:
(a) D. J. Diaz, K.-G. Hylton and L. McElwee-White, Selective Catalytic Oxidative Carbonylation of Amino Alcohols to Ureas, J. Org. Chem., 2006, 71, 734–738 Search PubMed;
(b) D. Scarpi, F. Lo Galbo and A. Guarna, Synthesis of a new 1,4-aminoalcohol and its use as catalyst in the enantioselective addition of organozinc to aldehydes, Tetrahedron: Asymmetry, 2006, 17, 1409–1414 CrossRef;
(c) J.-C. Zhong, M.-G. Wang, H.-C. Guo, M.-M. Yin, Q.-H. Bian and M. Wang, Design and Synthesis of 1,4-Amino Alcohol Ligands with a Chiral Cyclopropane Backbone for Asymmetric Diethylzinc Addition to Aromatic Aldehydes, Synlett, 2006, 1667–1670 Search PubMed;
(d) M. Sugiura, H. Hagio, R. Hirabayashi and S. Kobayashi, Lewis Acid-Catalyzed Ring-Opening Reactions of Semicyclic N,O-Acetals Possessing an Exocyclic Nitrogen Atom: Mechanistic Aspect and Application to Piperidine Alkaloid Synthesis, J. Am. Chem. Soc., 2001, 123, 12510–12517 CrossRef PubMed;
(e) N. Hanyu, T. Mino, M. Sakamoto and T. Fujita, Facile synthesis of amino bicyclo[2.2.1]heptyl alcohol and its application for enantioselective additions of diethylzinc to aldehydes, Tetrahedron Lett., 2000, 41, 4587–4590 CrossRef.
- See the ESI† for further details.
- Information obtained from ChemoSapiens chemical suppliers’ platform.
- 1,4-Imino alcohol 2g was formed in 35% 1H NMR yield; the crude mixture was analysed using trimethoxybenzene as the internal standard.
- Y. Kim, K. Lee, G. R. Mathi, I. Kimab and S. Hong, Visible-light-induced cascade radical ring-closure and pyridylation for the synthesis of tetrahydrofurans, Green Chem., 2019, 21, 2082–2087 RSC.
- Some reviews:
(a) X. Teng, T. Yu, J. Shi, H. Huang, R. Wang, W. Peng, K. Sun, S. Yang and X. Wang, Recent Advances in the Functionalization of Remote C–H Bonds by Hofmann–Löffler–Freytag-type Reactions, Adv. Synth. Catal., 2023, 365, 3211–3226 CrossRef;
(b) N. Goswami and D. Maiti, An Update on Distal C(sp3)–H Functionalization Involving 1,5-HAT Emerging from Nitrogen Radicals, Isr. J. Chem., 2020, 60, 303–312 CrossRef;
(c) C. Pratley, S. Fenner and J. A. Murphy, Nitrogen-Centered Radicals in Functionalization of sp2 Systems: Generation, Reactivity, and Applications in Synthesis, Chem. Rev., 2022, 122, 8181–8260 CrossRef;
(d) M. D. Kärkäs, Photochemical Generation of Nitrogen-Centered Amidyl, Hydrazonyl, and Imidyl Radicals: Methodology Developments and Catalytic Applications, ACS Catal., 2017, 7, 4999–5022 CrossRef.
-
(a) S.-P. Hu, C.-H. Gao, T.-M. Liu, B.-Y. Miao, H.-C. Wang, W. Yu and B. Han, Integrating Olefin Carboamination and Hofmann-Löffler-Freytag Reaction by Radical Deconstruction of Hydrazonyl N–N Bond, Angew. Chem., Int. Ed., 2024, 63, e202400168 CrossRef PubMed;
(b) S.-S. Li, Y.-S. Jiang, X.-L. Luo, C.-X. Pan and P.-J. Xia, Photoinduced Remote C(sp3)–H Imination Enabled by Vinyl Radical-Mediated 1,5-Hydrogen Atom Transfer, Org. Lett., 2023, 9, 1595–1599 CrossRef PubMed;
(c) Q. Guo, X. Ren and Z. Lu, Controllable Intramolecular Unactivated C(sp3)–H Amination and Oxygenation of Carbamates, Org. Lett., 2019, 21(4), 880–884 CrossRef CAS PubMed;
(d) X. Ren, Q. Guo, J. Chen, H. Xie, Q. Xu and Z. Lu, Visible-Light Promoted Distereodivergent Intramolecular Oxyamidation of Alkenes, Chem. – Eur. J., 2016, 22, 18695–18699 CrossRef CAS PubMed.
- A. N. Herron, C. Hsu and J.-Q. Yu, δ-C–H Halogenation Reactions Enabled by a Nitrogen-Centered Radical Precursor, Org. Lett., 2022, 24, 3652–3656 CrossRef CAS.
- R. I. Rodríguez, M. Sicignano, M. J. García, R. G. Enríquez, S. Cabrera and J. Alemán, Taming photocatalysis in flow: easy and speedy preparation of α-aminoamide derivatives, Green Chem., 2022, 24, 6613–6618 RSC.
- J. Majhi, R. K. Dhungana, Á. Rentería-Gómez, M. Sharique, L. Li, W. Dong, O. Gutierrez and G. A. Molander, Metal-Free Photochemical Imino-Alkylation of Alkenes with Bifunctional Oxime Esters, J. Am. Chem. Soc., 2022, 144, 15871–15878 CrossRef CAS PubMed.
|
This journal is © the Partner Organisations 2024 |
Click here to see how this site uses Cookies. View our privacy policy here.