DOI:
10.1039/D4QO01692K
(Research Article)
Org. Chem. Front., 2024,
11, 6768-6777
A domino reaction strategy for facile and modular construction of synthetically challenging functionalized ortho-fluoroanilines†‡
Received
11th September 2024
, Accepted 28th September 2024
First published on 30th September 2024
Abstract
The selective formation of ortho-fluoroanilines, representing versatile intermediates for the pharmaceutical and fine chemical industries, relies to date on, e.g., transition-metal-catalyzed fluorination of azobenzenes, which must be preformed from aniline derivatives. While few efficient methods for aniline synthesis were reported, sustainable, straightforward, and selective synthesis of fluoroanilines, and in particular ortho-fluoroanilines, remains challenging. Herein, we describe a domino approach that involves the simultaneous construction of a benzene ring and the installation of both amine and fluorine groups in a single operation under metal-free conditions, starting from readily available acyclic compounds. The developed atom- and cost-efficient, highly convenient, selective, and environmentally friendly four-step domino process allows the formation of a variety of functionalized ortho-fluoroanilines with yields of up to 80% and bypasses the selectivity issues of transition-metal-catalyzed aniline fluorination reactions. Furthermore, we show that the new domino products can efficiently be utilized to synthesize fluorinated azo dye and (tetrahydro)quinazoline derivatives in a bioactive form, i.e., possessing a first-time proven micromolar antiviral activity and high selectivity (EC50 (HCMV) down to 1.9 ± 0.7 μM, CC50 up to >100 μM), under conventional and/or visible-light mediated conditions.
Introduction
Aniline is a prominent aromatic amine compound broadly used as a versatile chemical precursor for the synthesis of bioactive compounds,1–4 polymers,5,6 and azo dyes.7–9 There is also a growing number of fluorinated aniline-based drugs ranging from anti-inflammatory, antidepressant, antibiotic, antiviral, and anticancer derivatives (selected examples are shown in Fig. 1a).10–14 Fluorinated drugs can exhibit enhanced effectivity and selectivity, potentially leading to increased biological activity. This is often attributed to the possibility of improving many chemical and biological properties (e.g., solubility, lipophilicity, membrane permeability, metabolic stability, and receptor binding) when exchanging hydrogen with fluorine in the parental compound.11,15,16 Fluoroanilines also find application for the synthesis of polyfluoroanilines17 and fluorinated azo dyes18,19 (Fig. 1b and c), as fluorine in comparison to hydrogen can improve material properties.20 These examples from life and material sciences stress the importance of ortho-fluoroanilines as versatile compounds. While there are already several procedures towards aniline derivatives, like reduction of the corresponding nitrobenzene as first described by Zinin,21 palladium-catalyzed Buchwald–Hartwig C–N cross-coupling reaction,22 Ullmann-type amination,23 and more recently, iridium-/cobalt-photocatalyzed dehydrogenative coupling between amine and cyclohexanone by Leonori,24 and transition-metal-free C–N cross-coupling,25 only rare examples for synthesis of fluoroanilines were reported. Whereas fluorination of aliphatic systems can be carried out under relatively mild conditions,26 the fluorination of aromatic systems, especially for the synthesis of fluorine-containing aniline structures, remains challenging (selected examples are depicted in Fig. 1d–f).27–29 Recently, a para-selective nucleophilic fluorination method of anilides, using an electrochemically generated hypervalent iodine mediator (ArIF2), was reported, with Et3N·5HF serving as fluoride source (Fig. 1d).30 The utilization of anilide as opposed to aniline was imperative due to the ring's lowered electron density. Also, an electrophilic aromatic fluorination approach was explored with aniline (Fig. 1e).31 By employing an excess of elemental fluorine, a mixture of para-, meta-, and ortho-monosubstituted fluoroanilines was obtained.31 Moreover, elemental fluorine is challenging to handle, and this method's site-selective preparation of fluoroanilines is subpar. Although ortho-fluoroanilines find broad application in the synthesis of heterocycles,32–35 efficient synthetic strategies for highly substituted ortho-fluoroanilines are barely known. Current methodologies rely mainly on commercially available unsubstituted ortho-fluoroaniline, subject to altering via postmodifications.
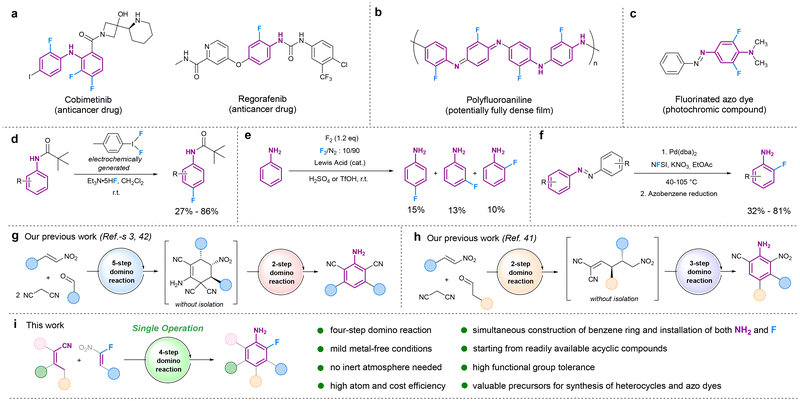 |
| Fig. 1 (a) Examples of fluoroaniline-containing drug compounds; (b) structure of polyfluoroaniline; (c) fluorinated azo dye; (d–f) literature known fluorinations of anilines; (g and h) previously reported domino approaches toward functionalized anilines by our research group; (i) this work: new straightforward four-step domino reaction toward functionalized fluoroaniline compounds. | |
Recently, an alternative route was reported, demonstrating the nitrate-promoted C–H fluorination of azobenzenes to synthesize substituted ortho-fluoroanilines. This approach utilizes the reduction of fluorinated azobenzenes to ortho-fluoroanilines after fluorination reaction (Fig. 1f).36 While this method was proven useful in terms of selectivity, as only ortho-fluoroanilines were synthesized, atom-efficiency still poses a challenge, as the reaction can only proceed via the detour of the azobenzene, which must also be pre-formed from aniline derivatives. Therefore, the formation of ortho-fluorinated anilines by this route is complex, and a convenient, straightforward, and environmentally friendly synthetic method for ortho-fluoroaniline compounds remains to be developed. In addition, arylated anilines are widely used in the materials and life sciences, and the standard method for C–C bond formation between aryl rings is still palladium-catalyzed cross-couplings in most cases. Due to the ever-increasing price for palladium and other late transition metals,37 a more sustainable route toward arylated anilines and, in particular, arylated fluoroanilines is desirable.
To address the mentioned gaps and synthetic challenges disparity, we applied the concept of domino reactions, which represent a powerful toolbox in organic synthesis to economically and sustainably install molecular complexity, starting from simple compounds.38 Domino processes avoid intermediate isolation and purification steps and are time-saving, waste-reducing, atom-efficient and can be considered as a green method superior to stepwise synthetic approaches.38–40 Although recently, we reported access to new aniline derivatives using different domino reactions and one-pot processes (Fig. 1g and h),3,41–43 to the best of our knowledge, a domino approach has never been used before to form an aromatic ring, and to install both amine and fluorine groups in a single operation while starting from readily available acyclic compounds. Herein, we report for the first time the straightforward synthesis of synthetically challenging functionalized ortho-fluoroanilines via a metal-free four-step domino reaction starting from readily available acyclic fluoro-nitrostyrenes and α,α-dicyanoolefine precursors (Fig. 1i). The developed atom- and cost-efficient, highly selective, and environmentally friendly domino process enables the formation of a variety of functionalized ortho-fluoroanilines with yields of up to 80%. The new products could be efficiently used to synthesize a fluorinated azo dye and (tetrahydro)quinazoline derivatives with antiviral properties.
Results and discussion
Synthesis of fluoroanilines
To overcome the drawbacks of existing fluorination methods, we envisioned a new metal-free domino reaction toward ortho-fluoroanilines using fluoro-nitrostyrenes and α,α-dicyanoolefines as starting compounds (Fig. 1i and 2a, b). At the beginning of our investigation, we focused on the optimization of the proposed two-component four-step linear domino reaction, which involves vinylogous Michael reaction/cyclization/imine–enamine tautomerization/aromatization steps (Fig. 2c). We intended to exploit the higher C–F bond strength in comparison to that of C–N (C–NO2) in selected substrate compounds, fluoro-nitrostyrenes, to form functionalized ortho-fluoroanilines (instead of ortho-nitroanilines41,43) in a single operation through a linear domino process. We applied preformed α,α-dicyanoolefins (Knoevenagel product 1a–e and 4a–i) since a Knoevenagel condensation step was not feasible in the initially investigated three-component reaction using ketone, malononitrile, and fluoro-nitrostyrene as starting compounds. In such a three-component reaction, we observed that the Knoevenagel condensation with ketone is reversible. As a result, a side product (Michael's reaction product out of fluoro-nitrostyrene and malononitrile) was formed instead of the desired domino product. We, therefore, preformed the α,α-dicyanoolefins and applied them for the new two-component domino reaction.
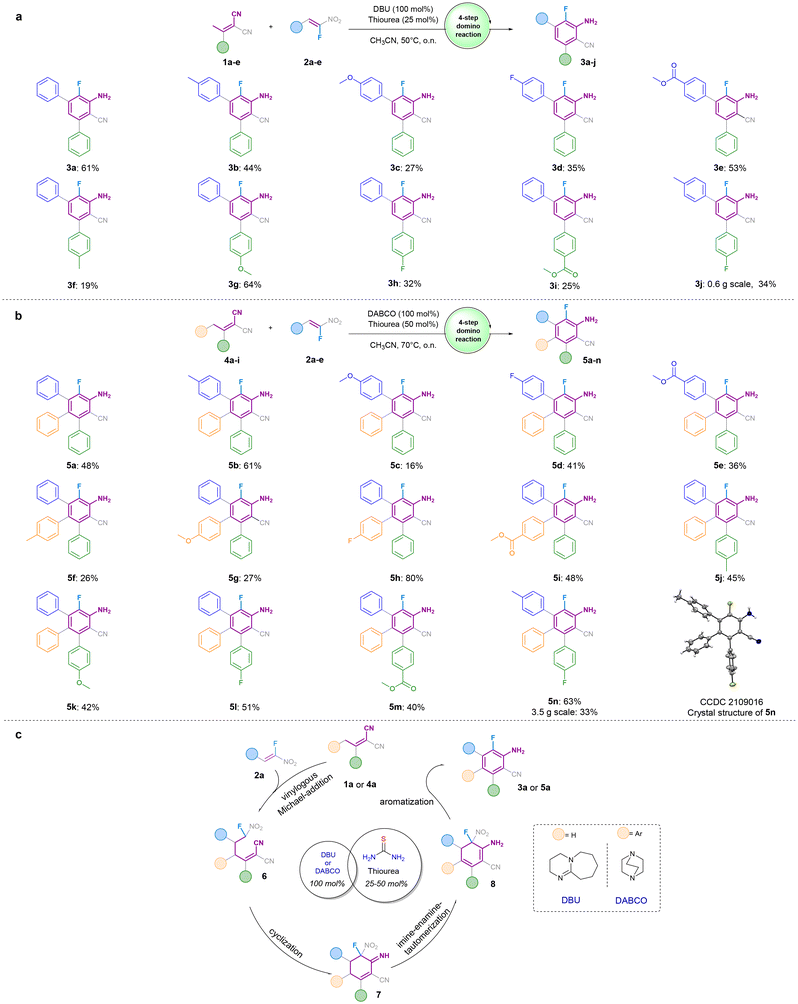 |
| Fig. 2 (a) Scope of meta-terphenyls. Conditions: α,α-dicyanoolefin (200 μmol), fluoro-nitrostyrene (200 μmol), DBU (100 mol%), thiourea (25 mol%), acetonitrile (2 mL), 50 °C. (b) Scope of triphenylanilines. Conditions: α,α-dicyanoolefin (200 μmol), fluoro-nitrostyrene (200 μmol), DABCO (100 mol%), thiourea (50 mol%), acetonitrile (2 mL), 70 °C. (c) Postulated mechanism of the new four-step domino process. | |
Utilizing the combination of DBU and thiourea (optimized conditions, see Table S1 of ESI‡) for the selected model reaction of α,α-dicyanoolefin 1a and fluoro-nitrostyrene 2a, we were able to isolate the desired ortho-fluoroaniline 3a in 61% yield over four steps (Fig. 2). Subsequently, the influence of substituents at the phenyl group of fluoro-nitrostyrene 2 on the reaction outcome was examined. The presence of methyl substituent (with +I effect) in the para-position of the phenyl group exhibits a slightly lower product yield (cf. 44% (3b) vs. 61% (3a), Fig. 1a). Fluoro-nitrostyrenes bearing electron-withdrawing groups (e.g., F, ester moiety) in para-positions of the phenyl group resulted in the desired ortho-fluoroanilines 3d and 3e in good yields of 35%/53% compared to ortho-fluoroaniline 3c (27% yield) with electron-donating methoxy substituent. Notably, even though the para-methoxy substituent enriches the electron density of the fluoro-nitrostyrene and decreases its electrophilicity and, therefore, the Michael acceptor's reactivity, the corresponding product 3c was obtained in satisfactory yield. Next, the influence of different substituents at the phenyl group of α,α-dicyanoolefins 1 on the reaction outcome was evaluated (Fig. 2b). The application of 1 with methyl group (+I effect) and/or electron-withdrawing substituents (e.g., F, ester moiety) resulted in the corresponding diaryl-substituted ortho-fluoroanilines 3f, 3h, 3i, and 3j with moderate yields of 19%–34%. In contrast, the electron-donating methoxy group was well tolerated in the developed reaction and gave the corresponding product 3g with a yield of 64%. This result underscores that the higher electron density is beneficial for the nucleophilicity of the vinylogous donors 1.
Regarding the triaryl-substituted ortho-fluoroanilines 5a–5n, similar observations were made concerning the influence of substituents at the phenyl group of fluoro-nitrostyrene 2 on the observed yields (products 5a–5e). Variation of substituents on the phenyl ring, standing in para-position to the amine group (see products 5f–5i), has the most perceptible impact on the yield: fluorine substitution increased the yield significantly (to 80%) compared to electron-donating groups, which have higher electron density, lowering the C–H acidity of the CH2 group, which needs to be deprotonated to enable the nucleophilic addition. While both the ester group and the fluoro substitution (5i and 5h, respectively), increase the acidity of the CH2 group, the difference in yield can be explained by the fact that the ester group mesomerically stabilizes the negative charge after deprotonation.
The delocalisation of charge results in a decreased reactivity for the nucleophilic attack compared to fluorine substitution. The good yield of 80% for fluoro-derivative 5h corresponds to an excellent average yield of 95% per step, underlining the practical applicability of the developed domino approach. Notably, the derivatisation of the lower aryl ring has less impact on the yield (see 5j–5n) compared to their meta-terphenyl analogues.
Synthesis of tetrahydroquinazoline and quinazoline scaffolds
To use the new fluoroanilines as precursors for synthesis of e.g., heterocyclic compounds, we performed large-scale synthesis of 3j and 5n as depicted in Fig. 2a and b. Given the fluorescence exhibited by both derivatives in solution, we investigated their absorption and emission in acetonitrile (see ESI, Fig. S1 and S2‡).
First, we attempted to utilize amine and nitrile moieties on the obtained fluoroanilines for functionalization. From literature,3 we know unsubstituted quinazolines 9 and 10 are directly accessible from o-amino nitrile by condensation with formamide. In order to get access to a broader array of quinazolines, we reduced the nitrile group of our triaryl-substituted ortho-fluoroaniline 5n to yield the corresponding diamine 11 using borane dimethylsulfide as a reducing agent.
Azo dyes are among the most significant classes of chromophores with diverse applications in the scientific, industrial, and pharmaceutical sectors.44 Taking into consideration the attractiveness of fluorinated azo dyes as photochromic compounds18,19 we additionally synthesized vividly red fluorinated azo dye 12 utilizing tert-butylnitrite to form the diazonium cation, and N,N-dimethylaniline as the required nucleophile.
Utilizing 11 as starting material, we obtained tetrahydroquinazolines 13–15 and quinazolines 16–18, with potential to display antiviral and other biological activity. All tetrahydroquinazolines 13–15 were synthesized by condensation reactions with the corresponding aldehyde or ketone utilizing silica as Lewis acid catalyst. By employing a variety of aldehydes, either aromatic or propargylic aldehydes, three quinazolines were obtained utilizing a literature known procedure under involvement of singlet oxygen, in situ generated by visible light using Rose Bengal as photosensitizer.45 Through this route quinazoline derivatives 16, 17, and 18 were obtained, although the aldehyde containing the artemisinin scaffold needed to be precondensed using the same method towards tetrahydroquinazolines as above, followed by aromatization via involvement of singlet oxygen.
In the past, our and other groups showed the high potential of hybrid drugs exhibiting strongly improved activities against viruses, malaria and other pathogens.46–50 This was the motivation to prepare and to investigate the artemisinin-based hybrid compounds 15 and 16 (Fig. 3).
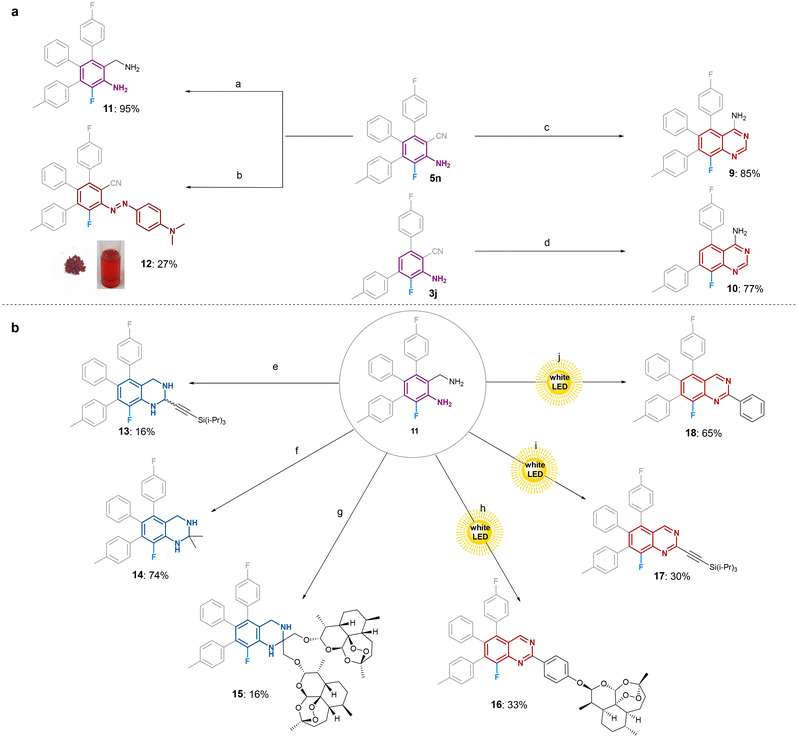 |
| Fig. 3 Post-modifications of synthesized fluoroanilines: (a) 1. SMe2BH3·Et2O, THF, 2. H2O, HCl, reflux. (b) 1. tert-Butylnitrite, acetonitrile, 0 °C, 2. N,N-Dimethylaniline, 0 °C to r.t. (c and d) Formamide, 210 °C. (e) Corresponding aldehyde, SiO2, CHCl3, r.t. (f) SiO2, acetone, r.t. (g) Corresponding ketone, SiO2, CHCl3, r.t., (h), 1. SiO2, corresponding aldehyde, CH2Cl2, 40 °C 2. Rose Bengal, DMF, O2, LED (white light), r.t. (i) and (j), Corresponding aldehyde, Rose Bengal, DMF, O2, LED (white light), r.t. | |
Cyclic voltammetric (CV) investigations of the novel azo dye 12
As studies by means of cyclic voltammetry revealed, the novel azo dye 12 exhibits both oxidative and reductive stability at a glassy carbon electrode in aprotic media (Fig. 4a). An irreversible oxidation event is observed at Ep = 1.16 V vs. SCE, which can be assigned to the oxidation of the amine moiety.51 Upon increasing scan rates, this amine oxidation becomes partially reversible (Fig. 4b). After rapid chemical follow-up reactions, the resulting species are reduced at Ep = −0.39 V vs. SCE. Interestingly, this reduction is more facile on a platinum electrode surface as indicated by a more pronounced reductive event at Ep = −0.32 V (Fig. 4c). In accordance with previous reports,52,53 a quasi-reversible one-electron reduction is observed at Ep = −1.37 V vs. SCE, forming a radical anion. Again, a subsequent chemical follow-up reaction generates a new species which gets oxidized at Ep = −0.45 V vs. SCE. The relative intensity of the oxidation event at Ep = −0.45 V vs. SCE, compared to the reversible response of the reduction event at Ep = −1.37, decreases with higher scan rates (Fig. 4d). This observation could be explained by a rapid intramolecular reaction of the formed radical with the nitrile group.54
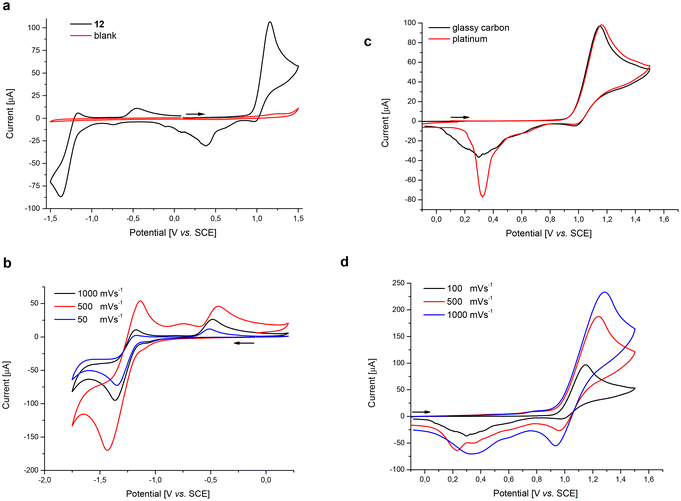 |
| Fig. 4 Cyclic voltammetry of 12 (5 mM). (a) at 100 mV s−1 in DCM with n-Bu4NPF6 (0.1 M); (b) at different scan rates in DCM with n-Bu4NPF6 (0.1 M) in oxidative potential regimes; (c). at 100 mV s−1 in DCM with n-Bu4NPF6 (0.1 M) at a glassy carbon (black) or a platinum (red) working electrode; (d) at different scan rates in DCM with n-Bu4NPF6 (0.1 M) in reductive potential regimes. | |
Antiviral activity
Previously, quinazoline compounds have been frequently used by numerous independent researchers, including our group,55–58 for the development of antiviral candidates and clinically approved drugs.59 As our recently reported autofluorescent quinazoline compounds have shown an initial, very promising evidence of intracellular bioactivity, including antiviral potential in cultured-cell models,3 we decided to specifically evaluate the novel heterocyclic compounds for their activity against the human cytomegalovirus (HCMV). We also tested a selected fluoroaniline derivative, as arylated anilines have the potential to exhibit interesting bioactivities e.g., antitumor properties.60 To this end, anti-HCMV activity was determined by an established quantitative system based on the recombinant HCMV AD169-GFP that expresses the green fluorescent protein (GFP) allowing for direct quantitation of intracellular viral replication (Fig. 5, blue). The compounds were incubated on virus-infected primary human foreskin fibroblasts (HFFs) at serial dilutions under identical conditions. Antiviral activity was defined as treatment-induced reduction of the GFP signal compared to DMSO-treated cells. In parallel, putative compound cytotoxicity was determined by Neutral Red uptake assay (NRA) using uninfected HFFs (Fig. 5, orange). On this basis, the effective concentrations, as required for half-maximal reduction of viral replication (EC50) or half-maximal cytotoxic activity (CC50), then referring to the selectivity index CC50/EC50 (SI), were determined in detail. The approved antiviral drug ganciclovir (GCV)61 was used as a reference compound. Of note, all new compounds analyzed displayed anti-HCMV activity within a range of micromolar concentrations, and their antiviral efficacies varied in a structure–activity-related manner, which may be subject to further characterization. In specific terms, compounds 5n, 14, 16, and 18 displayed a relatively weak but clearly measurable antiviral activity, with EC50 values above >10 μM, whereby only one compound, 14, was additionally limited by cytotoxicity. It is worth noting that 5n caused visible needle-like precipitations on the cells at concentrations of approximately 3 μM or higher. Excellent EC50 values of 1.9 ± 0.7 μM for artemisinin-containing tetrahydroquinazoline 15, and 2.4 ± 1.6 μM for quinazoline derivative 9 indicate a comparable antiviral efficacy to that of the reference drug GCV (EC50 = 2.60 ± 0.50 μM). The CC50 values for 9, and 15 were >100 μM and 31.5 ± 6.3 μM, respectively. In addition, compound 9 induced alterations in cell morphology, when applied at concentrations between 25–100 μM. These microscopically visible effects, however, could be distinguished from drug-induced cytotoxicity, since the respective measurements performed by NRA showed that neither of the compounds had a cytotoxic, negative impact on cell viability at concentrations up to 100 μM. Taken together, among this series of bioactive tetrahydroquinazolines, two of the analyzed compounds showed a very pronounced anti-HCMV activity. This characteristic was reflected by SI values of >41.2 and 17.0 for 9 and 15, respectively, underlining their strong antiviral activity.
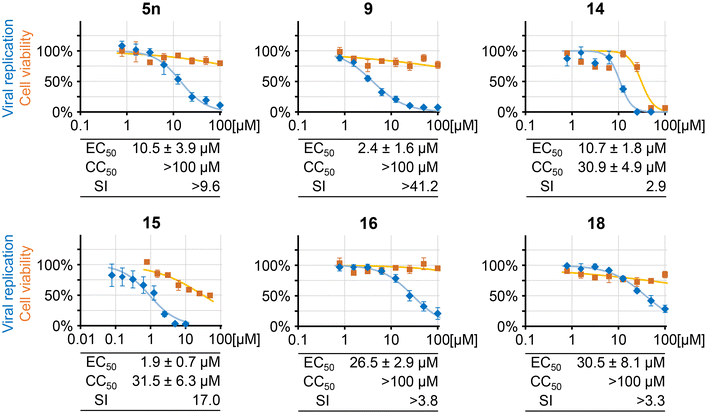 |
| Fig. 5 Anti-HCMV activity of selected ortho-fluoroaniline 5n and fluorine-containing quinazolines 9, 14, 15, 16, 18. Viral replication (blue) was assessed in a GFP-based replication assay using HCMV AD169-GFP for the infection of HFFs. Antiviral compounds were added immediately post-infection. Cells were fixed 7 d post-infection to perform quantitative GFP fluorometry. Cell viability (orange) was determined by performing NRA in parallel uninfected cells. The percentage of cell viability and viral replication was calculated and compared to DMSO-treated cells. Values represent mean ± SD of triplicate (NRA) or quadruplicate (GFP) determinations. | |
Conclusion
In summary, we developed a facile domino strategy to access synthetically challenging high-value functionalized ortho-fluoroanilines using readily available fluoro-nitrostyrenes and α,α-dicyanoolefines as starting compounds. The tangible advantages, i.e., absence of transition-metal catalysts, utilization of commercially available and/or easily accessible substrates, mild reaction conditions, simplicity, and single work-up procedure, make this metal-free four-step domino process highly appealing. The obtained products were applied for the efficient construction of a novel azo dye and various (tetrahydro)quinazolines through conventional and/or visible-light mediated reactions. A new azo dye was investigated regarding its oxidative and reductive stability using cyclovoltammetry. Importantly, in vitro bioactivity evaluation of the newly prepared tetrahydroquinazoline and quinazoline compounds demonstrated a strong antiviral efficacy against HCMV (EC50 values down to 1.9 ± 0.7 μM) and high selectivity (CC50 up to >100 μM).
Author contributions
B. W. G., S. K., and Y. G. carried out all synthetic work and conducted the domino reactions. F. H., J. L., and C. W. performed the in vitro studies under the supervision of M. M., M. S. carried out the CV measurements under the supervision of L. A., B. W. G., S. K., and S. B. T. wrote the manuscript with input from all authors. S. B. T. conceived and directed the research.
Data availability
All data are available from the authors upon reasonable request.
Conflicts of interest
There are no conflicts to declare.
Acknowledgements
We gratefully acknowledge financial support from the Deutsche Forschungsgemeinschaft (DFG, grants TS 87/28-1 for S. B. T. and AC 118/16-1 for L. A.) and Volkswagen-Stiftung (grant AZ-9B783-M. M./S. B. T.). We thank Dr Frank Hampel (FAU) for X-ray structure analysis of compound 5n.
References
- E. Vitaku, D. T. Smith and J. T. Njardarson, Analysis of the structural diversity, substitution patterns, and frequency of nitrogen heterocycles among U.S. FDA approved pharmaceuticals, J. Med. Chem., 2014, 57, 10257–10274 CrossRef CAS PubMed.
- L. Q. Sun, L. Zhu, K. Qian, B. Qin, L. Huang, C. H. Chen, K. H. Lee and L. Xie, Design, synthesis, and preclinical evaluations of novel 4-substituted 1,5-diarylanilines as potent HIV-1 non-nucleoside reverse transcriptase inhibitor (NNRTI) drug candidates, J. Med. Chem., 2012, 55, 7219–7229 CrossRef CAS PubMed.
- F. E. Held, A. A. Guryev, T. Frohlich, F. Hampel, A. Kahnt, C. Hutterer, M. Steingruber, H. Bahsi, C. von Bojnicic-Kninski, D. S. Mattes, T. C. Foertsch, A. Nesterov-Mueller, M. Marschall and S. B. Tsogoeva, Facile access to potent antiviral quinazoline heterocycles with fluorescence properties via merging metal-free domino reactions, Nat. Commun., 2017, 8, 15071 CrossRef PubMed.
- B. W. Ramsey, J. Davies, N. G. McElvaney, E. Tullis, S. C. Bell, P. Dřevínek, M. Griese, E. F. McKone, C. E. Wainwright, M. W. Konstan, R. Moss, F. Ratjen, I. Sermet-Gaudelus, S. M. Rowe, Q. Dong, S. Rodriguez, K. Yen, C. Ordoñez and J. S. Elborn, A CFTR Potentiator in Patients with Cystic Fibrosis and the G551D Mutation, N. Engl. J. Med., 2011, 365, 1663–1672 CrossRef CAS PubMed.
- Z. A. Boeva and V. G. Sergeyev, Polyaniline: Synthesis, properties, and application, Polym. Sci. Ser. C, 2014, 56, 144–153 CrossRef CAS.
- V. Babel and B. L. Hiran, A review on polyaniline composites: Synthesis, characterization, and applications, Polym. Compos., 2021, 42, 3142–3157 CrossRef CAS.
- F. A. Jerca, V. V. Jerca and R. Hoogenboom, Advances and opportunities in the exciting world of azobenzenes, Nat. Rev. Chem., 2022, 6, 51–69 CrossRef PubMed.
- D. Li, Z. Wang, L. Wang, X. Xu and H. Zhang, Ultrasonic Extraction Coupled with Capillary Electrophoresis for the Determination of Azo Dyes in Lipsticks Using Ionic Liquid as Dynamic Coating and Background Electrolyte, Chin. J. Chem., 2011, 29, 147–152 CrossRef CAS.
- R. I. Alsantali, Q. A. Raja, A. Y. A. Alzahrani, A. Sadiq, N. Naeem, E. U. Mughal, M. M. Al-Rooqi, N. El Guesmi, Z. Moussa and S. A. Ahmed, Miscellaneous azo dyes: a comprehensive review on recent advancements in biological and industrial applications, Dyes Pigm., 2022, 199, 110050 CrossRef CAS.
- S. Purser, P. R. Moore, S. Swallow and V. Gouverneur, Fluorine in medicinal chemistry, Chem. Soc. Rev., 2008, 37, 320–330 RSC.
- J. Wang, M. Sanchez-Rosello, J. L. Acena, C. del Pozo, A. E. Sorochinsky, S. Fustero, V. A. Soloshonok and H. Liu, Fluorine in pharmaceutical industry: fluorine-containing drugs introduced to the market in the last decade (2001-2011), Chem. Rev., 2014, 114, 2432–2506 CrossRef CAS PubMed.
- Y. Zhou, J. Wang, Z. Gu, S. Wang, W. Zhu, J. L. Acena, V. A. Soloshonok, K. Izawa and H. Liu, Next Generation of Fluorine-Containing Pharmaceuticals, Compounds Currently in Phase II-III Clinical Trials of Major Pharmaceutical Companies: New Structural Trends and Therapeutic Areas, Chem. Rev., 2016, 116, 422–518 CrossRef CAS PubMed.
- A. S. Aldoshin, A. A. Tabolin, S. L. Ioffe and V. G. Nenajdenko, Green, Catalyst-Free Reaction of Indoles with β-Fluoro-β-nitrostyrenes in Water, Eur. J. Org. Chem., 2018, 3816–3825 CrossRef CAS.
- Y. Ogawa, E. Tokunaga, O. Kobayashi, K. Hirai and N. Shibata, Current Contributions of Organofluorine Compounds to the Agrochemical Industry, iScience, 2020, 23, 101467 CrossRef CAS PubMed.
- J. Moschner, V. Stulberg, R. Fernandes, S. Huhmann, J. Leppkes and B. Koksch, Approaches to Obtaining Fluorinated alpha-Amino Acids, Chem. Rev., 2019, 119, 10718–10801 CrossRef CAS PubMed.
- C. Upadhyay, M. Chaudhary, R. N. De Oliveira, A. Borbas, P. Kempaiah, P. Singh and B. Rathi, Fluorinated scaffolds for antimalarial drug discovery, Expert Opin. Drug Discovery, 2020, 15, 705–718 CrossRef CAS PubMed.
- A. H. Kwon, J. A. Conklin, M. Makhinson and R. B. Kaner, Chemical synthesis and characterization of fluoro-substituted polyanilines, Synth. Met., 1997, 84, 95–96 CrossRef CAS.
- N. Ishikawa, M. J. Namkung and T. L. Fletcher, Fluorinated Azo Dyes.1a I. Synthesis and Spectral Properties of 3,5-Difluoro-4-N-methylaminoazobenzene, 2,6-Difluoroacetanilide, and Related Compounds, J. Org. Chem., 1965, 30, 3878–3882 CrossRef CAS.
- M. J. Namkung, N. K. Naimy, C. A. Cole, N. Ishikawa and T. L. Fletcher, Fluorinated azo dyes. II. Synthesis and spectral properties of 2,6-difluoro-4 and 2,3,5,6-tetrafluoro-4-aminoazobenzene and their N-methylated and 4′-ethyl derivatives, J. Org. Chem., 1970, 35, 728–733 CrossRef CAS.
- R. Berger, G. Resnati, P. Metrangolo, E. Weber and J. Hulliger, Organic fluorine compounds: a great opportunity for enhanced materials properties, Chem. Soc. Rev., 2011, 40, 3496–3508 RSC.
- N. Zinin, Beschreibung einiger neuer organischer Basen, dargestellt durch die Einwirkung des Schwefelwasserstoffes auf Verbindungen der Kohlenwasserstoffe mit Untersalpetersäure, J. Prakt. Chem., 1842, 27, 140–153 CrossRef.
- P. Ruiz-Castillo and S. L. Buchwald, Applications of Palladium-Catalyzed C-N Cross-Coupling Reactions, Chem. Rev., 2016, 116, 12564–12649 CrossRef CAS PubMed.
- C. W. Cheung and X. Hu, Amine synthesis via iron-catalysed reductive coupling of nitroarenes with alkyl halides, Nat. Commun., 2016, 7, 12494 CrossRef PubMed.
- S. U. Dighe, F. Juliá, A. Luridiana, J. J. Douglas and D. Leonori, A photochemical dehydrogenative strategy for aniline synthesis, Nature, 2020, 584, 75–81 CrossRef PubMed.
- P. S. Fier and S. Kim, Transition-Metal-Free C–N Cross-Coupling Enabled by a Multifunctional Reagent, J. Am. Chem. Soc., 2024, 146, 6476–6480 CrossRef CAS PubMed.
- P. T. Nyffeler, S. G. Duron, M. D. Burkart, S. P. Vincent and C. H. Wong, Selectfluor: mechanistic insight and applications, Angew. Chem., Int. Ed., 2004, 44, 192–212 CrossRef PubMed.
- D. J. Adams and J. H. Clark, Nucleophilic routes to selectively fluorinated aromatics, Chem. Soc. Rev., 1999, 28, 225–231 RSC.
- A. J. Cresswell, S. G. Davies, P. M. Roberts and J. E. Thomson, Beyond the Balz-Schiemann reaction: the utility of tetrafluoroborates and boron trifluoride as nucleophilic fluoride sources, Chem. Rev., 2015, 115, 566–611 CrossRef CAS PubMed.
- D. Q. Liang, Y. N. Li, S. L. Gao, R. L. Li, X. G. Li, B. L. Wang and H. Yang, Amide-assisted radical strategy: metal-free direct fluorination of arenes in aqueous media, Green Chem., 2017, 19, 3344–3349 RSC.
- M. Berger, M. S. Lenhard and S. R. Waldvogel, Para-Fluorination of Anilides Using Electrochemically Generated Hypervalent Iodoarenes, Chemistry, 2022, 28, e202201029 CrossRef CAS PubMed.
- J. P. Alric, B. Marquet, T. Billard and B. R. Langlois, Electrophilic aromatic fluorination with fluorine: meta-Directed fluorination of anilines, J. Fluorine Chem., 2005, 126, 659–665 CrossRef.
-
B. C. Soderberg, in Comprehensive Organometallic Chemistry II, ed. E. W. Abel, F. G. A. Stone and G. Wilkinson, Elsevier, Oxford, 1995, pp. 241–297 Search PubMed.
-
M. M. Heravi and L. Mohammadkhani, in Adv. Heterocycl. Chem, ed. E. F. V. Scriven and C. A. Ramsden, Academic Press, 2020, vol. 131, pp. 351–403 Search PubMed.
-
K. Pedretty, K. Tillett, W. Tsuei and J. M. Lopchuk, in Prog. Heterocycl. Chem, ed. G. W. Gribble and J. A. Joule, Elsevier, 2021, vol. 32, pp. 193–240 Search PubMed.
-
A. D. Sonawane and M. Koketsu, in Comprehensive Heterocyclic Chemistry IV, ed. D. S. Black, J. Cossy and C. V. Stevens, Elsevier, Oxford, 2022, pp. 685–712 Search PubMed.
- Y. J. Mao, G. Luo, H. Y. Hao, Z. Y. Xu, S. J. Lou and D. Q. Xu, Anion ligand promoted selective C-F bond reductive elimination enables C(sp(2))-H fluorination, Chem. Commun., 2019, 55, 14458–14461 RSC.
- D. Lichte, N. Pirkl, G. Heinrich, S. Dutta, J. F. Goebel, D. Koley and L. J. Goossen, Palladium-Catalyzed para-C-H Arylation of Anilines with Aromatic Halides, Angew. Chem., Int. Ed., 2022, 61, e202210009 CrossRef CAS PubMed.
-
L. F. Tietze, Domino Reactions, VCH, Weinheim, 2014 Search PubMed.
- K. Zhao, Y. Zhi, T. Shu, A. Valkonen, K. Rissanen and D. Enders, Organocatalytic Domino Oxa-Michael/1,6-Addition Reactions: Asymmetric Synthesis of Chromans Bearing Oxindole Scaffolds, Angew. Chem., Int. Ed., 2016, 55, 12104–12108 CrossRef CAS PubMed.
- S. Sopeña, M. Cozzolino, C. Maquilón, E. C. Escudero-Adán, M. Martínez Belmonte and A. W. Kleij, Organocatalyzed Domino [3+2] Cycloaddition/Payne-Type Rearrangement using Carbon Dioxide and Epoxy Alcohols, Angew. Chem., Int. Ed., 2018, 57, 11203–11207 CrossRef PubMed.
- B. W. Grau, S. Bonisch, A. Neuhauser, F. Hampel, A. Gorling and S. B. Tsogoeva, Facile Access to Challenging ortho-Terphenyls via Merging Two Multi-Step Domino Reactions in One-Pot: A Joint Experimental/Theoretical Study, ChemCatChem, 2019, 11, 3982–3992 CrossRef CAS.
- F. Plass, S. Bonisch, F. Held, T. Ullrich, F. E. J. Fischer, A. Guryev, A. Gorling, A. Kahnt and S. B. Tsogoeva, Controlling and Fine-Tuning Charge-Transfer Emission in 2,6-Dicyanoaniline Multichromophores Prepared through Domino Reactions: Entry to a Potentially New Class of OLEDs, J. Org. Chem., 2021, 86, 6111–6125 CrossRef CAS PubMed.
- B. W. Grau, M. Dill, F. Hampel, A. Kahnt, N. Jux and S. B. Tsogoeva, Four-Step Domino Reaction Enables Fully Controlled Non-Statistical Synthesis of Hexaarylbenzene with Six Different Aryl Groups, Angew. Chem., Int. Ed., 2021, 60, 22307–22314 CrossRef CAS PubMed.
- K. Mezgebe and E. Mulugeta, Synthesis and pharmacological activities of azo dye derivatives incorporating heterocyclic scaffolds: a review, RSC Adv., 2022, 12, 25932–25946 RSC.
- T. Yamaguchi, Y. Sugiura, E. Yamaguchi, N. Tada and A. Itoh, Synthetic Method for the Preparation of Quinazolines by the Oxidation of Amines Using Singlet Oxygen, Asian J. Org. Chem., 2017, 6, 432–435 CrossRef CAS.
- A. Çapcı, L. Herrmann, H. M. Sampath Kumar, T. Fröhlich and S. B. Tsogoeva, Artemisinin-derived dimers from a chemical perspective, Med. Res. Rev., 2021, 41, 2927–2970 CrossRef PubMed.
- H. M. S. Kumar, L. Herrmann and S. B. Tsogoeva, Structural hybridization as a facile approach to new drug candidates, Bioorg. Med. Chem. Lett., 2020, 30, 127514 CrossRef PubMed.
- B. Sharma, P. Singh, A. K. Singh and S. K. Awasthi, Advancement of chimeric hybrid drugs to cure malaria infection: An overview with special emphasis on endoperoxide pharmacophores, Eur. J. Med. Chem., 2021, 219, 113408 CrossRef CAS PubMed.
- S. B. Tsogoeva, Recent Progress in the Development of Synthetic Hybrids of Natural or Unnatural Bioactive Compounds for Medicinal Chemistry, Mini-Rev. Med. Chem., 2010, 10, 773–793 CrossRef CAS PubMed.
- L. F. Tietze, H. P. Bell and S. Chandrasekhar, Natural Product Hybrids as New Leads for Drug Discovery, Angew. Chem., Int. Ed., 2003, 42, 3996–4028 CrossRef CAS PubMed.
- H. J. Yang, D. O. Wipf and A. J. Bard, Application of Rapid Scan Cyclic Voltammetry to a Study of the Oxidation and Dimerization of N,N-Dimethylaniline in Acetonitrile, J. Electroanal. Chem., 1992, 331, 913–924 CrossRef CAS.
- J. L. Sadler and A. J. Bard, Electrochemical reduction of aromatic azo compounds, J. Am. Chem. Soc., 2002, 90, 1979–1989 CrossRef.
- Y. Q. Huang and J. Lessard, Electrochemical Behaviour of Nitrobenzene, Nitrosobenzene, Azobenzene, and Azoxybenzene on Hg, Pt, Cu, and Ni Electrodes in Aprotic Medium, Electroanalysis, 2016, 28, 2716–2727 CrossRef CAS.
- K. Sun, Q. Y. Lv, Y. W. Lin, B. Yu and W. M. He, Nitriles as radical acceptors in radical cascade reactions, Org. Chem. Front., 2021, 8, 445–465 RSC.
- C. Hutterer, S. Hamilton, M. Steingruber, I. Zeitträger, H. Bahsi, N. Thuma, Z. Naing, Z. Örfi, L. Örfi, E. Socher, H. Sticht, W. Rawlinson, S. Chou, V. J. Haupt and M. Marschall, The chemical class of quinazoline compounds provides a core structure for the design of anticytomegaloviral kinase inhibitors, Antiviral Res., 2016, 134, 130–143 CrossRef CAS PubMed.
- M. Marschall, T. Stamminger, A. Urban, S. Wildum, H. Ruebsamen-Schaeff, H. Zimmermann and P. Lischka, In Vitro Evaluation of the Activities of the Novel Anticytomegalovirus Compound AIC246 (Letermovir) against Herpesviruses and Other Human Pathogenic Viruses, Antimicrob. Agents Chemother., 2012, 56, 1135–1137 CrossRef CAS PubMed.
- M. Schleiss, J. Eickhoff, S. Auerochs, M. Leis, S. Abele, S. Rechter, Y. Choi, J. Anderson, G. Scott, W. Rawlinson, D. Michel, S. Ensminger, B. Klebl, T. Stamminger and M. Marschall, Protein kinase inhibitors of the quinazoline class exert anti-cytomegaloviral activity in vitro and in vivo, Antiviral Res., 2008, 79, 49–61 CrossRef CAS PubMed.
- T. Herget, M. Freitag, M. Morbitzer, R. Kupfer, T. Stamminger and M. Marschall, Novel Chemical Class of pUL97 Protein Kinase-Specific Inhibitors with Strong Anticytomegaloviral Activity, Antimicrob. Agents Chemother., 2004, 48, 4154–4162 CrossRef CAS PubMed.
- R. K. Avery, S. Alain, B. D. Alexander, E. A. Blumberg, R. F. Chemaly, C. Cordonnier, R. F. Duarte, D. F. Florescu, N. Kamar, D. Kumar, J. Maertens, F. M. Marty, G. A. Papanicolaou, F. P. Silveira, O. Witzke, J. Wu, A. K. Sundberg, M. Fournier and S. T. Investigators, Maribavir for Refractory Cytomegalovirus Infections With or Without Resistance Post-Transplant: Results From a Phase 3 Randomized Clinical Trial, Clin. Infect. Dis., 2021, 75, 690–701 CrossRef PubMed.
- C.-F. Lin, Y.-H. Lo, M.-C. Hsieh, Y.-H. Chen, J.-J. Wang and M.-J. Wu, Cytotoxicities, cell cycle and caspase evaluations of 1,6-diaryl-3(Z)-hexen-1,5-diynes, 2-(6-aryl-3(Z)-hexen-1,5-diynyl)anilines and their derivatives, Bioorg. Med. Chem., 2005, 13, 3565–3575 CrossRef CAS PubMed.
- F. Hahn, A. Niesar, C. Wangen, M. Wild, B. Grau, L. Herrmann, A. Capci, A. Adrait, Y. Couté, S. B. Tsogoeva and M. Marschall, Target verification of artesunate-related antiviral drugs: Assessing the role of mitochondrial and regulatory proteins by click chemistry and fluorescence labeling, Antiviral Res., 2020, 180, 104861 CrossRef CAS PubMed.
Footnotes |
† In honor of Professor H. Ila's 80th birthday. |
‡ Electronic supplementary information (ESI) available: Synthetic procedures, fluorescence and NMR spectra of the hybrid compounds, additional biological data and explanations. CCDC 2109016. For ESI and crystallographic data in CIF or other electronic format see DOI: https://doi.org/10.1039/d4qo01692k |
|
This journal is © the Partner Organisations 2024 |
Click here to see how this site uses Cookies. View our privacy policy here.