DOI:
10.1039/D3RA07078F
(Paper)
RSC Adv., 2024,
14, 995-1008
Design, synthesis, pharmacological evaluation, and in silico studies of the activity of novel spiro pyrrolo[3,4-d]pyrimidine derivatives†
Received
17th October 2023
, Accepted 9th December 2023
First published on 2nd January 2024
Abstract
In the present study, spiro compounds are shown to have distinctive characteristics because of their interesting conformations and their structural impacts on biological systems. A new family of functionalized spiro pyrrolo[3,4-d]pyrimidines is prepared via the one-pot condensation reaction of amino cyclohexane derivatives with benzaldehyde to prepare fused azaspiroundecanedione and azaspirodecenone/thione derivatives. A series of synthesized spiro compounds were scanned against DPPH and evaluated for their ability to inhibit COX-1 and COX-2. All compounds exhibit significant antiinflammatory activity, and they inhibited both COX-1 and COX-2 enzymes with a selectivity index higher than celecoxib as a reference drug. The most powerful and selective COX-2 inhibitor compounds were 11 and 6, with selectivity indices of 175 and 129.21 in comparison to 31.52 of the standard celecoxib. However, candidate 14 showed a very promising antiinflammatory activity with an IC50 of 6.00, while celecoxib had an IC50 of 14.50. Our findings are promising in the area of medicinal chemistry for further optimization of the newly designed and synthesized compounds regarding the discussed structure–activity relationship study (SAR), in order to obtain a superior antioxidant lead compound in the near future. All chemical structures of the novel synthesized candidates were unequivocally elucidated and confirmed utilizing spectroscopic and elemental investigations.
Introduction
The pyrimidine ring is an azaaromatic scaffold that can be found in many molecules of biological or pharmaceutical interest. Multicomponent reactions (MCRs) are interesting types of reaction due to the mixing of three or more reactants in one-pot and the generation of one product, and they are economically useful and environmentally secure compared to multi-step methods. Spiro heterocycles have significant uses in the pharmaceutical industry.1 The spiro heterocyclic structure is a distinguishing property of various natural and synthetic compounds with extraordinary biological activity.2–4 The potential application of spiro heterocycles in pharmaceutical chemistry has been widely reported due to their good pharmacological characteristics5–11 and geochemistry.12–14 In addition, they have different uses as photocurrent resources15 and asymmetric catalysts.16 Recently, piperidines have been among the most crucial synthetic building blocks for drug development and are essential to the pharmaceutical sector.17 Together with alkaloids, their derivatives are found in more than twenty different families of drugs. Numerous reviews on distinct piperidine synthesis techniques,18–21 their functionalization,22 and their pharmacological applications23–25 have been published recently. Moreover, the bile pigments bilirubin and biliverdin, as well as vitamin B12, include analogs of one of the heterocycles, pyrrole, which is not naturally generated.26–28 Pharmaceutical effects of pyrrole-ring-containing medications include antipsychotic,29 antiinflammatory,30,31 analgesic, antidepressant,32 antimicrobial,33 anticonvulsant,34 antineoplastic,35,36etc. More active compounds have been produced by combining different pharmacological agents with a pyrrole and pyrrolidine ring structure.37–39 There has been growing interest in the biological effects of pyrrole and its derivatives.40,41 Antioxidant activity has been demonstrated for pyrroles and their derivatives, which include an active hydrogen atom (N–H).42–44 Pyrimidines and pyridine-related compounds, on the other hand, are an important family of heterocycles due to their numerous chemical and biological uses. They are frequently used in the fields of medicine and material science.45–47 Their pharmacological effects include those that are antiinflammatory,48 antipyretic,49 antihypotensive,50 anticonvulsant,51 antiviral,52 antibacterial,53 and antidiabetic.54 We previously developed and produced some novel antiinflammatory and antioxidant molecules.55 One of the best-known computational tools applied in the field of medicinal chemistry is molecular docking, which is one of the most effective techniques to exhibit the architectures expected to have a high impact on specific proteins.56 Consequently, it will be possible to recognize which specific structural modifications are required to achieve outstanding efficiency in a simple and low-cost way.57–60 Fortunately, our candidates exhibited significant antiinflammatory inhibitory activity. These candidates also exhibited considerable antioxidant activity compared to ascorbic acid. The main objective of our study was to continue our efforts in this approach by synthesizing and characterizing a new set of spiro heterocyclic compounds and estimating their energies, which is crucial for both theoretical investigations and chemical reactivity.
Results and discussion
Chemistry
Our strategy to design and synthesize novel heterocyclic moieties involves the nucleophilic addition of the amino group of cyclohexyl amine to the heteroallene carbon of benzoyl isothiocyanate through aza Michael reaction producing acyl thiourea 3 that undergoes cyclization through the evolution of H2S and basic hydrolysis forming spiro pyrazole 4. The IR spectrum of 4 shows C
S absorption at 1248 cm−1. The downfield singlet signals located at 8.1 and 8.08 ppm were attributed to NH and NH2, respectively. Base-mediated cyclo-condensation of compound 1 and diethyl succinate resulted in pyridine cyclization affording spiro derivative 6. The NH downfield exchangeable singlet signal for NH was observed at 8.15 ppm, and elemental analysis data have been discussed for a representative (Scheme 1).
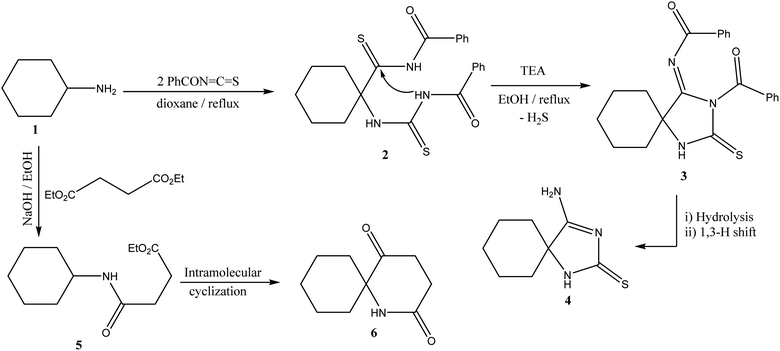 |
| Scheme 1 Synthetic route of spiro azines 4 and 6. | |
Compound 1 was cyclized with ethyl cyanoacetate at room temperature to give 4-amino-1-azaspiro[4.5]dec-3-en-2-one 8.1 The latter compound underwent cyclization with cyclohexanone and benzaldehyde through 1,4 additions to give a polycyclic derivative 11. The carbonyl absorption band of 11 was exhibited at 1636 cm−1. The exchangeable NH signal was detected at 9.16 ppm, and the carbonyl carbon signal was shown at 198.26 ppm (Scheme 2).
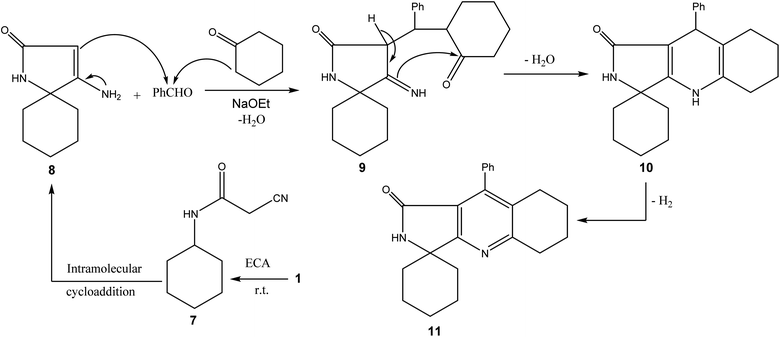 |
| Scheme 2 Synthesis of polycyclic azine 11. | |
Reacting spiro compound 8 with equivalent amounts of benzaldehyde and thiourea provided pyrimidine derivative 14. NH, C
O, and C
S absorption bands of 14 were shown at 3349, 1670, and 1251 cm−1, respectively. The 1H NMR spectrum of 14 showed two NH signals at 8.25 and 8.23 ppm.
Cyclic enamine 8 was allowed to react with a mixture of ethyl acetoacetate and benzaldehyde in a basic medium providing non-isolable Michael adduct 15 that goes through cyclodehydration and hydrolysis, followed by evolution of CO2 and subsequent aromatization. The NH and C
O peaks were shown at 3348 and 1668 cm−1, respectively. The NH singlet signal was located at 8.25 ppm and the carbonyl carbon signal was detected at 160.32 ppm (Scheme 3).
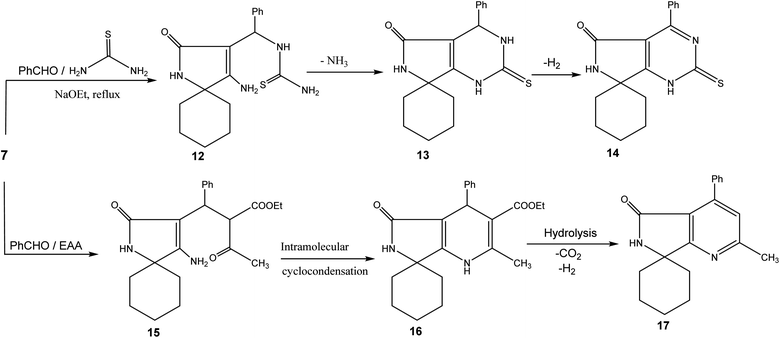 |
| Scheme 3 Synthesis of pyrimidine and pyridine derivatives 14 and 17. | |
In silico studies
Molecular docking studies.
Docking studies are used to predict the effectiveness of newly synthesized compounds. These theoretical studies (molecular docking) emphasize the bioactivities of our candidates. Molecular Operating Environment (MOE) 2015 software was used to assess our candidates. Due to the structural similarity of our candidates with indomethacin and celecoxib, we performed docking studies for the active sites of proteins COX-1 (PDB: 3KK6) and COX-2 (PDB: 4COX).61,62 Therefore, the crystal structure of cyclooxygenase-2 enzyme in a complex with indomethacin (PDB: 4COX) and the crystal structure of cyclooxygenase-1 in a complex with Celecoxib (PDB: 3KK6) were downloaded from the protein data bank. All proteins were prepared by removing water molecules and 3D protonation, then fixing the potential energies. Table 1 reveals the docking studies results of compounds 4, 6, 11, 14, and 16 with the receptors of the largest pocket of chain A of cyclooxygenase-2 enzyme 4COX. Indomethacin as a reference compound was re-docked in the prepared largest pocket to bind with Arg120, Tyr355, and Ser530 via three hydrogen bonds at 2.84, 2.85, and 2.92 Å with binding score −8.8 kcal mol−1. Our candidates exhibit significant binding scores by comparing with indomethacin. Compounds 14 and 17 show superb antiinflammatory activities due to their high stabilization inside the active site of the COX-2 enzyme with considerable binding scores (−6.5 and −5.5 kcal mol−1, respectively). These candidates were bound with crucial amino acids Arg120 and Tyr355 via hydrogen bonding, as shown in Table 2. Compounds 4, 6, and 11 exhibit moderate inhibition values for the COX-2 enzyme with notable binding scores of ₋5.01, −5.5, and −5.03 kcal mol−1, respectively, as shown in Tables 1 and 2.
Table 1 The binding scores, receptor interactions, distances, and energies of our promising candidates 4, 6, 11, 14, and 17 compared to indomethacin as a reference standard inside the binding pocket of cyclooxygenase-2
Ligand |
Docking score |
RMSD |
Interaction |
Distance (Å) |
E (kcal mol−1) |
Ligand |
Receptor |
Type of interaction |
4
|
−5.01 |
1.2 |
S12 |
Val523 |
H-acceptor |
3.79 |
−1.1 |
S12 |
Gly526 |
H-acceptor |
3.66 |
−0.9 |
6
|
−5.5 |
1.4 |
O13 |
Arg120 |
H-acceptor |
3.19 |
−3.7 |
11
|
−5.03 |
1.3 |
6-Ring |
Arg120 |
Pi-cation |
4.39 |
−1.2 |
14
|
−6.5 |
0.78 |
S3 |
Arg120 |
H-acceptor |
3.81 |
−0.8 |
17
|
−5.5 |
1.3 |
O16 |
Arg120 |
H-acceptor |
3.17 |
−0.8 |
O16 |
Tyr355 |
H-acceptor |
2.60 |
−0.9 |
Indomethacin (docked) |
−8.8 |
1.2 |
O7 |
Arg120 |
H-acceptor |
2.84 |
−3.9 |
O7 |
Tyr355 |
H-acceptor |
2.85 |
−3.5 |
O25 |
Ser530 |
H-acceptor |
2.92 |
−1.2 |
O6 |
Arg120 |
Ionic |
2.86 |
−5.5 |
O7 |
Arg120 |
Ionic |
2.84 |
−5.6 |
Table 2 Predicted two and three-dimensional binding interactions of 4, 6, 11, 14, and 17 visualized in the cyclooxygenase-2 active site (PDB: 4COX) compared to the predicted binding orientations of indomethacin; ligands are shown in green
Ligand |
2D receptor interaction |
3D receptor interaction |
Indomethacin |
|
|
Compound 4 |
|
|
Compound 6 |
|
|
Compound 11 |
|
|
Compound 14 |
|
|
Compound 17 |
|
|
In the current work, our candidates exhibit very promising activities against the COX-1 enzyme compared with celecoxib as an isolated reference ligand from the original protein pocket, as shown in Table 3. Celecoxib was stabilized inside cyclooxygenase-1 with binding energy −7.7 kcal mol−1 through the formation of three H-bonds with the amino acid residues Leu352, His90, and Ser353. Compounds 14 and 17 show very promising inhibition potential of the COX-1 enzyme with high binding scores (−6.78 and −6.9 kcal mol−1, respectively). Candidate 11 reveals moderate inhibition of COX-1 through interaction with amino acid residue Ile 523 inside the prepared protein pocket with binding energy −6.26 kcal mol−1, while candidates 4 and 6 show modest inhibition activities, as shown in Tables 3 and 4.
Table 3 The binding scores, receptor interactions, distances, and energies of our promising candidates 4, 6, 11, 14, and 17 compared to celecoxib as a reference standard inside the binding pocket of cyclooxygenase-1
Ligand |
Docking score |
RMSD |
Interaction |
Distance (Å) |
E (kcal mol−1) |
Ligand |
Receptor |
Type of interaction |
4
|
−5.07 |
0.74 |
N11 |
Met 522 |
H-donor |
3.56 |
−0.8 |
S12 |
Ser 353 |
H-acceptor |
3.49 |
−1.7 |
6
|
−4.6 |
1.55 |
O12 |
Arg120 |
H-acceptor |
2.94 |
−1.2 |
11
|
−6.26 |
0.77 |
O19 |
Ile 523 |
H-acceptor |
3.16 |
−0.7 |
14
|
−6.78 |
1.02 |
N9 |
Ser 530 |
H-donor |
2.81 |
−2.0 |
S3 |
Ser 353 |
H-acceptor |
3.63 |
−1.2 |
17
|
−6.9 |
1.9 |
6-Ring |
Ser 353 |
Pi–H |
4.43 |
−1.2 |
Celecoxib (docked) |
−7.7 |
0.8 |
N3 |
Leu 352 |
H-donor |
2.81 |
−7.0 |
O2 |
His 90 |
H-acceptor |
2.88 |
−2.8 |
5-Ring |
Ser 353 |
Pi–H |
4.35 |
−0.9 |
Table 4 Predicted two and three-dimensional binding interactions of 4, 6, 11, 14, and 17 visualized in the cyclooxygenase-1 active site (PDB: 3KK6) compared to the predicted binding orientation of celecoxib; ligands are shown in green
Ligand |
2D receptor interaction |
3D receptor interaction |
Celecoxib |
|
|
Compound 4 |
|
|
Compound 6 |
|
|
Compound 11 |
|
|
Compound 14 |
|
|
Compound 17 |
|
|
In vitro antiinflammatory activity
The human red blood cell (RBC) membrane stabilization assay is a method used to evaluate the ability of a substance to prevent damage to the RBC membrane. The assay is based on the principle that certain substances can stabilize the RBC membrane and prevent hemolysis, or the breakdown of RBCs subjected to stressors. The extent of hemolysis is measured by spectrophotometry or by other methods such as the release of hemoglobin. The degree of RBC membrane stabilization can be quantified by calculating the percentage of hemolysis inhibition.
The human RBC membrane stabilization assay is commonly used to evaluate the antiinflammatory and antioxidant activity of natural products, such as plant extracts, and synthetic compounds. The assay can also be used to assess the potential toxicity of drugs and chemicals to RBC membranes. All the tested compounds showed in vitro antiinflammatory activity upon using human red blood cell hemolysis and membrane stabilization assay. As shown in Table 5, of the newly synthesized azaspiro compounds, compound 4 exhibited potent hemolytic inhibition of 89.9% in comparison to 90% of that of the standard indomethacin, when all of the tested compounds were used at a concentration of 200 μg mL−1. Other cyclohexane pyrrolo compounds showed hemolysis inhibitions varying from 61.3% to 79.2% when used at the same concentration of 200 μg mL−1. On the other hand, previous studies have reported that some azaspiro compounds had higher efficacy and selectivity than polyunsaturated fatty acids in alleviating inflammatory symptoms in prototypical autoimmune diseases.63
Table 5 Hemolysis inhibition (%) for the synthesized compounds at different concentrations
Sample concentration (μg mL−1) |
100 |
200 |
400 |
600 |
800 |
1000 |
Comp. 4 |
88.4 |
89.9 |
92.8 |
94.6 |
96.0 |
97.8 |
Comp. 6 |
58.2 |
61.3 |
69.0 |
73.2 |
78.2 |
84.7 |
Comp. 11 |
76.1 |
79.2 |
107.8 |
86.4 |
91.1 |
96.0 |
Comp. 14 |
67.6 |
74.4 |
78.8 |
82.6 |
85.1 |
94.0 |
Comp. 17 |
69.2 |
74.6 |
79.4 |
82.7 |
85.4 |
90.1 |
Standard (indomethacin) |
88.0 |
90.1 |
93.5 |
95.3 |
97.3 |
98.9 |
GPCRs are very important to prevent colorectal cancer progression, as it is essential to maintain the integrity of the mucosal barriers.64 Azaspiro compounds were evaluated biologically, and tested as potential antitumor agents.65 They affect different cell cycle stages, and granular actin diffusion through the cytoplasm was observed using confocal microscopy. Other compounds containing azaspiro rings exhibited a potent kinase inhibitor activity.66
These compounds can be used to stop metastasis and the progression of tumors. Tyrosine kinase inhibitors were found to reduce proinflammatory cytokines such as TNF-alpha and IL-1 in addition to reducing the expression of cyclooxygenase 2 and inducible nitric oxide synthase (INOS).67 It was also found that compounds containing the cyclohexane group attenuated the cardiac injury induced by CCl4 in Wistar rats. They inhibited lipooxygenase and decreased inflammatory markers like TNF-α and IL-6.68 Additionally, new cyclohexane fused spiroselenuranes showed potent antioxidant activity.69 It has been reported that some azaspiro compounds had antioxidant and antielastase activity,70 as it was found that human neutrophil elastase modulates cytokine and growth factor expression. The antiinflammatory activity of the newly synthesized compounds was also evaluated for COX-1/COX-2 inhibitory activities using an ovine COX-1/human recombinant COX-2 assay kit, and celecoxib was used as a reference standard drug as shown in Table 6. The results revealed that all the tested compounds showed a potent antiinflammatory activity and they inhibited both COX-2 and COX-1 enzymes, and with a selectivity index higher than that of celecoxib as a reference drug. The most powerful and selective COX-2 inhibitor compounds were 11 and 6 with selectivity indices of 175 and 129.21 in comparison to 31.52 of the standard celecoxib.
Table 6
In vitro antiinflammatory activity for COX-1 and COX-2
Sample number |
IC50a (μM) COX-1 |
IC50a (μM) COX-2 |
IC50b (μM) COX-1/COX-2 |
Values are means of three determinations acquired using an ovine COX-1/COX-2 assay kit (catalog no. 560131; Cayman Chemicals Inc. Ann Arbour, MI, USA). Celecoxib was used as a reference drug.
In vitro COX-2 selectivity index (IC50 of COX-1/COX-2).
|
4
|
7.50 |
0.135 |
55.56 |
6
|
11.50 |
0.089 |
129.21 |
11
|
10.50 |
0.060 |
175.00 |
14
|
6.00 |
0.080 |
75.00 |
17
|
8.00 |
0.099 |
80.81 |
Celecoxib |
14.50 |
0.0460 |
31.52 |
In vitro antioxidant activity
Free radicals and reactive oxygen species are associated with aging, inflammation, cancer, atherosclerosis, and neurodegenerative disorders. Antioxidants play an important role to prevent and or stop the progression of such conditions.71 The antioxidant activity of the newly synthesized compounds was evaluated by DPPH (2,2-diphenyl-1-picrylhydrazyl) assay. This assay principle is based on electron transfer, where the DPPH radical, a stable free radical, is reduced by an antioxidant to form a non-radical form. The degree of discoloration of the DPPH radical solution is measured spectrophotometrically at 517 nm, and the decrease in absorbance indicates the scavenging activity of the antioxidant.
The antioxidant activity of a compound is expressed as the percentage of DPPH radical scavenging, and the IC50 value, which is the concentration of the antioxidant required for scavenging of 50% of the DPPH radicals as a measure of the antioxidant capacity. It was found that all the tested compounds had different antioxidant activities (at a concentration of 1000 μg mL−1) to that of ascorbic acid as a standard drug (Table 7). As per Jun et al. and referenced textbooks,72,73 antioxidant effectiveness is categorized based on IC50 values. An IC50 below 50 μg mL−1 denotes highly potent antioxidant activity, while activity is labeled as strong within the range of 50–100 μg mL−1, moderate at 101–250 μg mL−1, and weak at 250–500 μg mL−1, and the compound is deemed inactive at an IC50 of greater than 500 μg mL−1. The obtained results revealed that compound 11 exhibited a strong antioxidant activity while compound 6 had a moderate activity with IC50 values of 33.0 and 94.04 μg mL−1 in comparison to 4.08 μg mL−1 of the standard ascorbic acid, and DPPH scavenging percentages of 86.1%, 74.4% and 97%, respectively. Compounds 14 and 4 demonstrated the lowest antioxidant activity, with IC50 values of 222.9 and 263.3 μg mL−1, respectively, along with DPPH scavenging percentages of 66.5% and 61.6%, respectively.
Table 7 Antioxidant assay for the tested compounds
Compound |
IC50 |
DPPH scavenging% |
Comp. 4 |
263.3 |
61.1 |
Comp. 6 |
94.04 |
74.4 |
Comp. 11 |
33.0 |
86.1 |
Comp. 14 |
222.9 |
66.5 |
Standard (ascorbic acid) |
4.08 |
97 |
Materials and methods
Synthesis
N-((1-(Benzoylcarbamothioyl)cyclohexyl)carbamothioyl)benzamide (2).
A mixture of cyclohexyl amine (0.025 mol) and benzoyl isothiocyanate (0.025 mol) in dioxane (15 mL) was heated under reflux for 30 min., then stirred for 12 hours and the formed precipitate was filtered off, dried, and recrystallized from dilute ethanol to give compound 3 as a yellowish white powder.
M.P. 130 °C, yield: 96%. IR (KBr) νmax: 3220, 3299 (NH), 2935 (CH aliphatic), 1675 (C
O), 1233 (C
S) cm−1. 1H NMR (400 MHz, DMSO-d6) δ (ppm): 11.23 (s, 1H, NH), 9.85 (s, 1H, NH), 9.56 (s, 1H, NH), 7.48–7.93 (m, 10H, Ph-H), 2.2 (m, 10H, cyclohexane-H). Anal. Calcd for C22H23N3O2S2 (425.57): C, 62.09; H, 5.45; N, 9.87; S, 15.07; found: C, 62.14; H, 5.42; N, 9.85; S, 15.04.
4-Amino-1,3-diazaspiro[4.5]dec-3-ene-2-thione (4).
A mixture of compound 2 (0.025 mol) and TEA (4 drops) in 15 mL of ethanol was heated under reflux for 2 hours. The precipitate formed after concentrating the reaction mixture and acidification with acetic acid was filtered off, dried, and recrystallized from dilute ethanol to give compound 6 as a white powder.
M.P.: 110 °C, yield: 93%. IR (KBr) νmax: 3279 (NH), 2933 (CH aliphatic), 1248 (C
S) cm−1. 1H NMR (400 MHz, DMSO-d6) δ (ppm): 8.10 (s, 1H, NH), 8.08 (s, 2H, NH2), 2.2 (m, 10H, Ph-H), 1.27–1.75 (m, 10H, cyclohexane-H). Anal. Calcd for C8H13N3S (183.27): C, 52.43; H, 7.15; N, 22.93; S, 17.50; found: C, 52.38; H, 7.18; N, 22.90; S, 17.55.
1-Azaspiro[5.5]undecane-2,5-dione (6).
A mixture of cyclohexylamine (0.02 mol), diethyl succinate (0.02 mol), and NaOH (0.02 mol) in ethanol (15 mL) was heated under reflux for 5 hours. The precipitate formed after concentrating the reaction mixture and acidification with HCl was filtered off, dried, and recrystallized from ethanol to give compound 16 as white crystals.
M.P.: 190 °C, yield: 65%. IR (KBr) νmax: 2937 (CH aliphatic), 1665 (C
O) cm−1. 1H NMR (400 MHz, DMSO-d6) δ (ppm): 8.15 (s, 1H, exchangeable with D2O, NH), 2.41–2.50 (m, 4H, 2CH2, pyridine-H) 1.18–1.92 (m, 10H, cyclohexane-H). Anal. Calcd for C10H15NO2 (181.23): C, 66.27; H, 8.34; N, 7.73; found: C, 66.32; H, 8.29; N, 7.77.
9′-Phenyl-5′,6′,7′,8′-tetrahydrospiro[cyclohexane-1,3′-pyrrolo[3,4-b]quinolin]-1′(2′H)-one (11).
A mixture of enaminone 8 (0.02 mol), cyclohexanone (0.02 mol), benzaldehyde (0.02 mol), and sod. ethoxide (0.02) in ethanol (15 mL) was stirred for 2 hours and then heated under reflux for 2 hours. The precipitate formed after concentrating the reaction mixture and acidification with HCl was filtered off, dried, and recrystallized from EtOH/AcOH to give compound 21 as a yellowish-white powder.
M.P.: 90 °C, yield: 75%. IR (KBr) νmax: 2927 (CH aliphatic), 1636 (C
O) cm−1. 1H NMR (400 MHz, DMSO-d6) δ (ppm): 9.16 (s, 1H, exchangeable with D2O, NH), 7.07–7.39 (m, 5H, Ph-H) 1.02–3.28 (m, 18H, cyclohexane-H). 13C NMR (400 MHz, DMSO-d6) δ (ppm): 26.97, 118.11, 118.29, 123.57, 124.28, 127.83, 128.37, 129.33, 129.49, 130.53, 130.83, 131.52, 135.05, 144.31, 152.51, 198.26. Anal. Calcd for C22H24N2O (332.44): C, 79.48; H, 7.28; N, 8.43; found: C, 79.55; H, 7.21; N, 8.47.
4′-Phenyl-2′-thioxo-1′,2′-dihydrospiro[cyclohexane-1,7′-pyrrolo[3,4-d]pyrimidin]-5′(6′H)-one (14).
A mixture of enaminone 8 (0.01 mol), benzaldehyde (0.01 mol), thiourea (0.01 mol), and sod. ethoxide (0.01) in ethanol (15 mL) was heated under reflux for 4 hours. The precipitate formed after concentrating the reaction mixture and acidification with HCl was filtered off, dried, and recrystallized from dilute ethanol to give compound 14 as a white powder.
M.P.: 130 °C, yield: 90%. 3349 (NH), 2920 (CH aliphatic), 1670 (C
O), 1251 (C
S) cm−1. 1H NMR (400 MHz, DMSO-d6) δ (ppm): 8.25 (s, 1H, NH), 8.23 (s, 1H, NH), 7.56–8.10 (m, 5H, Ph-H) 1.0–1.80 (m, 10H, cyclohexane-H). 13C NMR (400 MHz, DMSO-d6) δ (ppm): 24.77, 25.14, 32.00, 49.02, 107.13, 116.42, 129.21, 129.88, 132.03, 132.16, 149.91, 160.35. Anal. Calcd for C17H17N3OS (311.40): C, 65.57; H, 5.50; N, 13.49; S, 10.30; found: C, 65.61; H, 5.46; N, 13.47; S, 10.26.
2′-Methyl-4′-phenylspiro[cyclohexane-1,7′-pyrrolo[3,4-b]pyridin]-5′(6′H)-one (17).
A mixture of enaminone 8 (0.01 mol), ethyl acetoacetate (0.01), benzaldehyde (0.01 mol), and TEA (4 drops) in dioxane (20 mL) was heated under reflux for 3 hours. The precipitate formed after concentrating the reaction mixture and acidification with acetic acid was filtered off, dried, and recrystallized from ethanol to give compound 17 as a white powder.
M.P.: 125 °C, yield: 95%. 3348 (NH), 2919 (CH aliphatic), 1668 (C
O), 1603 (C
N) cm−1. 1H NMR (400 MHz, DMSO-d6) δ (ppm): 8.25 (s, 1H, NH), 7.27–8.10 (m, 6H, Ph-H + pyridine-H), 3.66 (s, 3H, CH3), 1.13–1.81 (m, 10H, cyclohexane-H). 13C NMR (400 MHz, DMSO-d6) δ (ppm): 24.76, 25.13, 31.98, 49.00, 107.12, 116.40, 129.18, 129.86, 132.02, 132.13, 149.89, 160.32. Anal. Calcd for C19H20N2O (292.37): C, 78.05; H, 6.89; N, 9.58; found: C, 78.12; H, 6.84; N, 9.63.
In vitro antiinflammatory activity
Using human RBC hemolysis and membrane stabilization assay.
Preparation of erythrocyte suspension.
Fresh whole blood (3 mL) collected from healthy volunteers into heparinized tubes was centrifuged at 3000 rpm for 10 min. A volume of normal saline equivalent to that of the supernatant was used to dissolve the red blood pellets. The volume of the dissolved red blood pellets obtained was measured and reconstituted as a 40% v/v suspension with isotonic buffer solution (10 mM sodium phosphate buffer, pH 7.4). The buffer solution contained 0.2 g of NaH2PO4, 1.15 g of NaH2PO4, and 9 g of NaCl in 1 liter of distilled water. The reconstituted red blood cells (resuspended supernatant) were used.72
Hypotonicity-induced hemolysis.
Samples used in this test were dissolved in distilled water (hypotonic solution). The hypotonic solution (5 mL) containing graded doses of the sample (100, 200, 400, 600, 800, and 1000 μg mL) was put into duplicate pairs (per dose) of centrifuge tubes. Isotonic solution (5 mL) containing graded doses of the sample (100–1000 μg mL−1) was also put into duplicate pairs (per dose) of centrifuge tubes. Control tubes contained 5 mL of the vehicle (distilled water) and 5 mL of 200 μg mL−1 of indomethacin. Erythrocyte suspension (0.1 mL) was added to each of the tubes and mixed gently. The mixtures were incubated for 1 hour at room temperature (37 °C), and afterwards, centrifuged for 3 min at 1300 g. Absorbance (OD) of the hemoglobin content of the supernatant was estimated at 540 nm using a Spectronic (Milton Roy) spectrophotometer. The percentage of hemolysis was calculated by assuming the hemolysis produced in the presence of distilled water to be 100%. The percent inhibition of hemolysis by the extract was calculated thus:
% Inhibition of hemolysis = 1 − ((OD2 − OD1)/(OD3 − OD1)) × 100 |
where OD1 = absorbance of test in an isotonic solution, OD2 = absorbance of test in a hypotonic solution, OD3 = absorbance of control in a hypotonic solution.
In vitro inhibitory effect of COX-1 and COX-2
All the synthesized compounds are evaluated for their ability to inhibit COX-1 and COX-2 using an ovine COX-1/COX-2 inhibitor screening assay kit (catalog no. 560131; Cayman Chemicals Inc. Ann Arbour, MI, USA) that utilizes the peroxidase component of COX. The peroxidase activity is assayed colorimetrically by monitoring the appearance of oxidized N,N,N′,N′-tetramethyl-1,4-phenylenediamine (TMPD), which is produced during the reduction of PGG2 to PGH2, at 590 nm.38 The results are listed in Table 6 as the compound's concentration causing 50% enzyme inhibition (IC50) and they are the means of three determinations. In addition, the selectivity index (SI values), which was defined as IC50 (COX-1)/IC50 (COX-2), was calculated for the tested compounds. Additionally, the IC50 values of celecoxib (the reference standard) on COX-1 and COX-2 were determined.73
In vitro antioxidant activity by DPPH scavenging%
Free radical scavenging activities of different newly synthesized compounds were measured using 1,1-diphenyl-2-picrylhydrazyl (DPPH).74 In brief, a 0.1 mM solution of DPPH in ethanol was prepared. This solution (1 mL) was added to 3 mL of different samples in ethanol at a concentration of 1000 μg mL−1. The mixture was shaken vigorously and allowed to stand at room temperature for 30 min, then the absorbance was measured at 517 nm by using a spectrophotometer (UV-VIS Milton Roy). The reference standard compound being used was ascorbic acid and the experiment was done in triplicate. The IC50 value of the sample, which is the concentration of sample required to inhibit 50% of the DPPH free radical, was calculated using the log dose inhibition curve. A lower absorbance of the reaction mixture indicated higher free radical activity. The percent DPPH scavenging effect was calculated by using the following equation:
DPPH scavenging effect (%) or percent inhibition = A0 − A1/A0 × 100. |
where A0 was the absorbance of the control reaction and A1 was the absorbance in the presence of a test or standard sample.
Molecular docking studies
The molecular docking program, Molecular Operating Environment (MOE) version 2015 software, can directly simulate the interaction of ligands with enzymes cyclooxygenase-1 and cyclooxygenase-2. The X-ray crystallographic structures of the cyclooxygenase-2 enzyme (PDB ID: 4COX) and cyclooxygenase-1 enzyme (PDB: 3KK6) were downloaded from the protein data bank.75,76 Celecoxib and indomethacin, as reference anti-inflammatory agents, and all candidates were drawn using ChemDraw, copied into the MOE program window, modified to take on 3D shapes, and corrected for partial charges, and their energies were minimized to the lowest possible level. Proteins (PDB code: 4COX and 3KK6) were prepared by removing water molecules and adding 3D hydrogen atoms. Finally, before proceeding with the docking process, we chose the most suitable pocket protein by subjecting it to the site finder option on the MOE 2015 program.
Conclusion
The authors have developed a novel, rapid and efficient protocol for the synthesis of novel spiro derivatives. Out of our belief in the biological efficiency of spiro hetero moieties, we aimed to synthesize fused azaspiroundecanedione and azaspirodecenone/thione derivatives. Fortunately, the most powerful and selective COX-2 inhibitor compounds were 11 and 6, with selectivity indices of 175 and 129.21, in comparison to 31.52 of the standard celecoxib. However, candidate 14 showed a very promising antiinflammatory activity with an IC50 of 6.00, while celecoxib had an IC50 of 14.50. Candidate 11 exhibited a potent antioxidant activity of 86%, while ascorbic acid as a standard reference inhibits oxidation by 97%. Interestingly, most of our newly synthesized candidates give significant yields (over 90%), such as candidates 2, 3, 14, and 17. Compounds 14 and 17 exhibit significant inhibition activities against enzymes COX-1 and COX-2. In silico studies showed that candidates 14 and 17 inhibit COX-1 with significant docking scores of −6.78 and −6.9 kcal mol−1, respectively, compared to −7.7 kcal mol−1 for celecoxib. These are promising lead compounds, which via structural modification lead to the design and synthesis of novel powerful antioxidants. Furthermore, the mechanism of action for the new compounds was proposed to involve cytochrome c peroxidase inhibitors via molecular docking, and compared to ascorbic acid as a reference standard.
Consent for publication
All authors consent to the publication.
Ethics approval
This article does not include any studies with human participants or animals performed by any of the authors.
Data availability
All data generated or analyzed during this work are available from the corresponding author on request.
Conflicts of interest
The authors declare that they have no conflicts of interest.
Acknowledgements
The authors extend their appreciation to the Deanship of Scientific Research at King Khalid University for funding this work through the large group Research Project under grant number (RGP2/413/44).
References
- A. Mermer, T. Keles and Y. Sirin, Recent studies of nitrogen containing heterocyclic compounds as novel antiviral agents: a review, Bioorg. Chem., 2021, 114, 105076, DOI:10.1016/j.bioorg.2021.105076.
- I. Natsutani, R. Iwata, Y. Yamai, K. Ishida, Y. Nagaoka and T. Sumiyoshi, Design, synthesis, and evaluations of spiro-fused benzoxaborin derivatives as novel boron-containing compounds, Chem. Biol. Drug Des., 2019, 93, 657–665, DOI:10.1111/cbdd.13496.
- M. Moghaddam-Manesh, D. Ghazanfari, E. Sheikhhosseini and M. Akhgar, MgO-Nanoparticle-Catalyzed Synthesis and Evaluation of Antimicrobial and Antioxidant Activity of New Multi-Ring Compounds Containing Spiro[indoline-3,4′-[1,3] dithiine], ChemistrySelect, 2019, 4(31), 9247–9251, DOI:10.1002/slct.201900935.
- M. M. K. Amer, M. Abdelaziz, W. S. Shehab, M. H. Abdellattif and S. M. Mouneir, Recent advances in chemistry and pharmacological aspects of 2-pyridone scaffolds, J. Saudi Chem. Soc., 2021, 25(6), 101259, DOI:10.1016/j.jscs.2021.101259.
- S. Balasubramanian, C. Ramalingan, G. Aridoss and S. Kabilan, Synthesis, and study of antibacterial and antifungal activities of novel 8-methyl-7,9-diaryl1,2,4,8-tetraazaspiro [4.5] decan-3-thiones, Eur. J. Med. Chem., 2005, 40, 694–700, DOI:10.1016/j.ejmech.2005.02.001.
- J.-L. Reymond and M. Awale, Exploring chemical space for drug discovery using the chemical universe database, ACS Chem. Neurosci., 2012, 3(9), 649–657, DOI:10.1021/cn3000422.
- M. J. James, P. O'Brien, R. J. K. Taylor and W. P. Unsworth, Synthesis of Spirocyclic Indolenines, Chem.–Eur. J., 2016, 22(9), 2856–2881, DOI:10.1002/chem.201503835.
- P. N. Kalaria, S. C. Karad and D. K. Raval, A review on diverse heterocyclic compounds as the privileged scaffolds in antimalarial drug discovery, Eur. J. Med. Chem., 2018, 158, 917–936, DOI:10.1016/j.ejmech.2018.08.040.
- W. Li, S. J. Zhao, F. Gao, Z. S. Lv, J. Y. Tu and Z. Xu, Synthesis and in vitro anti-tumor, anti-mycobacterial and anti-HIV activities of diethylene-glycol-tethered bis-isatin derivatives, ChemistrySelect, 2018, 3, 10250–10254, DOI:10.1002/slct.201802185.
- X. Zhao, S. T. Chaudhry and J. Mei, Heterocyclic building blocks for organic semiconductors. Heterocyclic chemistry in the 21st Century a Tribute to Alan Katritzky, Adv. Heterocycl. Chem., 2017, 121, 133–171, DOI:10.1016/bs.aihch.2016.04.009.
- T. A. Khattab and M. A. Rehan, A Review on Synthesis of Nitrogen-Containing Heterocyclic Dyes for Textile Fibers - Part 2: Fused Heterocycles, Egypt, J. Chem., 2018, 61, 989–1018, DOI:10.21608/ejchem.2018.4131.1363.
-
C. Lamberth and J. Dinges, Bioactive Heterocyclic Compound Classes: Agrochemicals, Wiley, KGaA, 2012 Search PubMed.
- R. Ramachandran, M. Rani, S. Senthan, Y. T. Jeong and S. Kabilan, Synthesis, spectral, crystal structure and in vitro antimicrobial evaluation of imidazole/benzotriazole substituted piperidin-4-one derivatives, Eur. J. Med. Chem., 2011, 46, 1926–1934, DOI:10.1016/j.ejmech.2011.02.036.
- A. Sethukumar, P. S. Anand, C. U. Kumar and B. A. Prakasam, Synthesis, stereochemical and biological studies of some N-cyclohexylcarbamoyl-2,6-diarylpiperidin4-ones, J. Mol. Struct., 2017, 1130, 352–362, DOI:10.1016/j.molstruc.2016.10.035.
- R. Pradhan, M. Patra, A. K. Behera, B. K. Mishra and R. K. Behera, A synthon approach to spiro compounds, Tetrahedron, 2006, 62, 779–828, DOI:10.1016/j.tet.2005.09.039.
- K. Ding, Z. Han and Z. Wang, Spiro Skeletons: A Class of Privileged Structure for Chiral Ligand Design, Chem.–Asian J., 2009, 4, 32–41, DOI:10.1002/asia.200800192.
-
T. Sandmeier and E. M. Carreira, Synthetic Approaches to Nonaromatic Nitrogen Heterocycles, Chapter 10 Modern Catalytic Enantioselective Approaches to Piperidines, John Wiley & Sons Ltd., Hoboken, NJ, USA, 2020, pp. 249–271, DOI:10.1002/9781119708841.ch10.
- R. W. Bates, W. Ko and V. Barát, The endo-aza-Michael addition in the synthesis of piperidines and pyrrolidines, Org. Biomol. Chem., 2020, 18, 810–829, 10.1039/C9OB02388G.
- N. Kaur, N. Ahlawat, Y. Verma, P. Grewal, P. Bhardwaj and N. K. Jangid, Cu-assisted C–N bond formations in six-membered N-heterocycle synthesis, Synth. Commun., 2020, 50, 1075–1132, DOI:10.1080/00397911.2019.1695278.
- A. Mostafa MK, M. H. Abdellattif, S. M. Mouneir, W. A. Zordok and W. S. Shehab, Synthesis, DFT calculation, pharmacological evaluation, and catalytic application in the synthesis of diverse pyrano [2, 3-c] pyrazole derivatives, Bioorg. Chem., 2021, 114, 105136 CrossRef PubMed.
- M. Slivka and N. Korol, Synthesis of mononuclear heterocycles via electrophilic cyclization, Monatsh. Chem., 2022, 153, 1–8, DOI:10.1007/s00706-021-02869-6.
-
G.-Q. Liu and T. Opatz, Chapter Two-Recent Advances in the Synthesis of Piperidines: Functionalization of Preexisting Ring Systems, in Advances in Heterocyclic Chemistry. Volume 125, ed. E. F. V. Scriven and C. A. Ramsden, Academic Press, Cambridge, MA, USA, 2018, pp. 107–234, DOI:10.1016/bs.aihch.2017.10.001.
- E. Mezeiova, K. Spilovska, E. Nepovimova, L. Gorecki, O. Soukup, R. Dolezal, D. Malinak, J. Janockova, D. Jun and K. Kuca,
et al., Profiling donepezil template into multipotent hybrids with antioxidant properties, J. Enzym. Inhib. Med. Chem., 2018, 33, 583–606, DOI:10.1080/14756366.2018.1443326.
- A. Rathore, V. Asati, K. S. Kashaw, S. Agarwal, D. Parwani, S. Bhattacharya and C. Mallick, The Recent Development of Piperazine and Piperidine Derivatives as Antipsychotic Agents, Mini-Rev. Med. Chem., 2021, 21, 362–379, DOI:10.2174/1389557520666200910092327.
- W. S. Shehab, A. F. EL-Farargy, A. O. Abdelhamid and M. Abdelaziz, Synthesis and biological application of pyranopyrimidine derivatives catalyzed by efficient nanoparticles and their nucleoside analogues, Synth. Commun., 2019, 49(24), 3560–3572, DOI:10.1080/00397911.2019.1679538.
- D. Osman, A. Cooke, T. R. Young, E. Deery and N. J. Robinson,
et al., The requirement for cobalt in vitamin B12: a paradigm for protein metalation, Biochim. Biophys. Acta Mol. Cell Res., 2021, 1868, 118896, DOI:10.1016/j.bbamcr.2020.118896.
- M. L. Santos, M. D'Ambrosio, A. P. Rodrigo, A. J. Parola and P. M. Costa, A transcriptomic approach to the metabolism of tetrapyrrolic photosensitizers in a marine annelid, Molecules, 2021, 26, 3924, DOI:10.3390/molecules26133924.
- D. D. Haines and A. Tosaki, Heme degradation in pathophysiology of and countermeasures to inflammation-associated disease, Int. J. Mol. Sci., 2020, 21, 9698, DOI:10.3390/ijms21249698.
- A. Thurkauf, J. Yuan, N. Chen, J. W. F. Wasley, R. Meade, K. H. Woodruff, K. Huston and P. C. Ross, 1-Phenyl-3-(aminomethyl)pyrroles as Potential Antipsychotic Agents. Synthesis and Dopamine Receptor Binding, J. Med. Chem., 1995, 38(25), 4950–4952, DOI:10.1021/jm00025a013.
- W. W. Wilkerson, R. A. Copeland, M. Covington and J. M. Trzaskos, Antiinflammatory 4,5-Diarylpyrroles. 2. Activity as a Function of Cyclooxygenase-2 Inhibition, J. Med. Chem., 1995, 38(20), 3895–3901, DOI:10.1021/jm00020a002.
- A. Kajal, S. Bala, N. Sharma, S. Kamboj and V. Saini, Therapeutic Potential of Hydrazones as Antiinflammatory Agents, Int. J. Med. Chem., 2014, 2014, 761030, DOI:10.1155/2014/761030.
- K. N. de Oliveira, P. Costa, J. R. Santin, L. Mazzambani, C. Bürger, C. Mora and R. J. Nunes,
et al., Synthesis and antidepressant-like activity evaluation of sulphonamides and sulphonyl-hydrazones, Bioorg. Med. Chem., 2011, 19(14), 4295–4306, DOI:10.1016/j.bmc.2011.05.056.
- L. Yurttaþ, Y. Özkay, Z. A. Kaplancýklý, Y. Tunalı and H. Karaca, Synthesis and antimicrobial activity of some new hydrazone-bridged thiazole-pyrrole derivatives, J. Enzyme Inhib. Med. Chem., 2013, 28(4), 830–835, DOI:10.3109/14756366.2012.688043.
- J. V. Ragavendran, D. Sriram, S. K. Patel, I. V. Reddy, N. Bharathwajan, J. Stables and P. Yogeeswari, Design and synthesis of anticonvulsants from a combined phthalimide–GABA–anilide and hydrazone pharmacophore, Eur. J. Med. Chem., 2007, 42(2), 146–151, DOI:10.1016/j.ejmech.2006.08.010.
- S. I. Alqasoumi, M. M. Ghorab, Z. H. Ismail, S. M. Abdel-Gawad, M. S. A. El-Gaby and H. M. Aly, Novel Antitumor Acetamide, Pyrrole, Pyrrolopyrimidine, Thiocyanate, Hydrazone, Pyrazole, Isothiocyanate and Thiophene Derivatives Containing a Biologically Active Pyrazole Moiety, Arzneimittelforschung, 2009, 59(12), 666–671, DOI:10.1055/s-0031-1296457.
- A. A. El-Tombary and S. A. M. El-Hawash, Synthesis, Antioxidant, Anticancer and Antiviral Activities of Novel Quinoxaline Hydrazone Derivatives and their Acyclic C-Nucleosides, Med. Chem., 2014, 10(5), 521–532, DOI:10.2174/15734064113096660069.
- B. M. Cawrse, N. M. Robinson, N. C. Lee, G. M. Wilson and K. L. Seley-Radtke, Structural and biological investigations for a series of n-5 substituted pyrrolo [3,2-d] pyrimidines as potential anticancer therapeutics, Molecules, 2019, 24, 2656, DOI:10.3390/molecules24142656.
- U. Leone Roberti Maggiore, M. Valenzano Menada, P. L. Venturini and S. Ferrero, The potential of sunitinib as a therapy in ovarian cancer, Expert Opin. Invest. Drugs, 2013, 22(12), 1671–1686, DOI:10.1517/13543784.2013.841138.
- D. K. Lang, R. Kaur, R. Arora, B. Saini and S. Arora, Nitrogen-containing heterocycles as anticancer agents: an overview, Anticancer Agents Med. Chem., 2020, 20, 2150–2168, DOI:10.2174/1871520620666200705214917.
- B.-C. Ivan, S.-F. Barbuceanu, C. M. Hotnog, A. I. Anghel, R. V. Ancuceanu, M. A. Mihaila, L. I. Brasoveanu, S. Shova, C. Draghici, O. T. Olaru, G. M. Nitulescu, M. Dinu and F. Dumitrascu, New Pyrrole Derivatives as Promising Biological Agents: Design, Synthesis, Characterization, In Silico, and Cytotoxicity Evaluation, Int. J. Mol. Sci., 2022, 23, 8854, DOI:10.3390/ijms23168854.
- M. S. Kamel, A. Belal, M. O. Aboelez, E. K. Shokr, H. Abdel-Ghany, H. S. Mansour, A. M. Shawky and M. A. E. A. A. A. El-Remaily, Microwave-Assisted Synthesis, Biological Activity Evaluation, Molecular Docking, and ADMET Studies of Some Novel Pyrrolo [2,3-b] Pyrrole Derivatives, Molecules, 2022, 27, 2061, DOI:10.3390/molecules27072061.
- E. O. Hamed, D. A. Elsayed, M. G. Assy and W. S. Shehab, Design, Synthesis, Docking, 2D-QSAR Modelling, Anticancer and Antioxidant Evaluation of Some New Azo-Compounds Derivatives and Investigation of Their Fluorescence Properties, ChemistrySelect, 2022, 7(41), e202202534 CrossRef CAS.
- P. D. MacLean, E. E. Chapman, S. L. Dobrowolski, A. Thompson and L. R. C. Barclay, Pyrroles As Antioxidants: Solvent Effects and the Nature of the Attacking Radical on Antioxidant Activities and Mechanisms of Pyrroles, Dipyrrinones, and Bile Pigments, J. Org. Chem., 2008, 73(17), 6623–6635, DOI:10.1021/jo8005073.
- J. D. Bhosale, A. R. Shirolkar and U. D. Pete,
et al., Synthesis, characterization and biological activities of novel substituted formazans of 3,4-dimethyl-1H-pyrrole-2-carbohydrazide derivatives, J. Pharm. Res., 2013, 7(7), 582–587, DOI:10.1016/j.jopr.2013.07.022.
- R. Mohareb and S. Fahmy, Reaction of malononitrile and ethyl cyanoacetate: a novel synthesis of polyfunctional pyridine derivatives, Z. Naturforsch. B Chem. Sci., 1985, 40, 1537–1540, DOI:10.1515/znb-1985-1120.
- H. Khalaf, H. Tolan, M. El-Bayaa, M. Radwan, M. El-Manawaty and W. El-Sayed, Synthesis and anticancer activity of new pyridine-thiophene and pyridine-furan
hybrid compounds, their sugar hydrazone, and glycosyl derivatives, Russ. J. Gen. Chem., 2020, 90, 1706–1715, DOI:10.1134/S1070363220090182.
- V. Sharma, N. Chitranshi and A. K. Agarwal, Significance and biological importance of pyrimidine in the microbial world, Int. J. Med. Chem., 2014, 2014, 202784, DOI:10.1155/2014/202784.
- S. Maddila, S. Gorle, N. Seshadri, P. Lavanya and S. B. Jonnalagadda, Synthesis, antibacterial and antifungal activity of novel benzothiazole pyrimidine derivatives, Arabian J. Chem., 2016, 9, 681–687, DOI:10.1016/j.arabjc.2013.04.003.
- K. A. El-Sharkawy, M. M. AlBratty and H. A. Alhazmi, Synthesis of some novel pyrimidine, thiophene, coumarin, pyridine and pyrrole derivatives and their biological evaluation as analgesic, antipyretic and antiinflammatory agents, Braz. J. Pharm. Sci., 2018, 54, e00153, DOI:10.1590/s2175-97902018000400153.
- M. De Luca, G. Ioele and G. Ragno, 1, 4-Dihydropyridine antihypertensive drugs: recent advances in photostabilization strategies, Pharmaceutics, 2019, 11, 85, DOI:10.3390/pharmaceutics11020085.
- K. Kaminski, J. Obniska, A. Zagorska and D. Maciag, Synthesis, physicochemical and anticonvulsant properties of new N-(pyridine-2-yl) derivatives of 2-azaspiro[4.4]nonane and [4.5]decane-1,3-dione. Part II, Arch. Pharm. Chem. Life Sci., 2006, 339, 255–326, DOI:10.1002/ardp.200500219.
- N. Wang, Q. Yang, Z. Deng, X. Mao and Y. Peng, Rhodium-catalyzed merging of 2-arylquinazolinone and 2,2-difluorovinyltosylate: diverse synthesis of monofluoroolefin quinazolinone derivatives, ACS Omega, 2020, 5, 14635–14644, DOI:10.1021/acsomega.0c01344.
- S. Kumar and B. Narasimhan, Therapeutic potential of heterocyclic pyrimidine scaffolds, Chem. Cent. J., 2018, 12, 1–29, DOI:10.1186/s13065-018-0406-5.
- H. W. Lee, B. Y. Kim, J. B. Ahn, S. K. Kang, J. H. Lee, J. S. Shin, S. K. Ahn, S. J. Lee and S. S. Yoon, Molecular design, synthesis, and hypoglycemic and hypolipidemic activities of novel pyrimidine derivatives having thiazolidinedione, Eur. J. Med. Chem., 2005, 40, 862–874, DOI:10.1016/j.ejmech.2005.03.019.
- A. M. Abdel Hamid, A. H. Amer, M. G. Assy, W. A. Zordok, S. M. Mouneir, S. El-Kalyoubi and W. S. Shehab, Synthesis, pharmacological evaluation, DFT calculation, and theoretical, Bioorg. Chem., 2023, 131, 106280, DOI:10.1016/j.bioorg.2022.106280.
-
G. M. Morris and M. Lim-Wilby, Molecular Docking, in Molecular Modeling of Proteins. Methods Molecular Biology™, ed. A. Kukol, Humana Press, 2008, vol 443, DOI:10.1007/978-1-59745-177-2_19.
- M. Abdelaziz, W. S. Shehab, A. A. Al-Karmalawy, A. F. EL-Farargy and M. H. Abdellattif, Design, Synthesis, Biological Evaluation, 2D-QSAR Modeling, and Molecular Docking Studies of Novel 1H-3-Indolyl Derivatives as Significant Antioxidants, Int. J. Mol. Sci., 2021, 22, 10396, DOI:10.3390/ijms221910396.
- W. S. Shehab, M. Abdelaziz, N. K. R. Elhoseni, M. G. Assy, M. H. Abdellattif and E. O. Hamed, Design, Synthesis, Molecular Docking, and Evaluation Antioxidant and Antimicrobial Activities for Novel 3-Phenylimidazolidin-4-One and 2-Aminothiazol-4-One Derivatives, Molecules, 2022, 27, 767, DOI:10.3390/molecules27030767.
- L. M. Abbass, S. A. Sadeek, M. Abdelaziz, W. A. A. Zordok and M. S. El-Attar, Synthesis of some new nanoparticles mixed metal complexes of febuxostat in presence of 2, 2′-bipyridine: Characterization, DFT, antioxidant and molecular docking activities, J. Mol. Liq., 2023, 122460, DOI:10.1016/j.molliq.2023.122460.
- M. H. M. AbdEl-Azim, M. Abdelaziz, S. M. Mouneir, A. F. EL-Farargy and W. S. Shehab, Ecofriendly synthesis of pyrano[2,3-d]pyrimidine derivatives and related heterocycles with anti-inflammatory activities, Arch. Pharm., 2020, 353, e2000084, DOI:10.1002/ardp.202000084.
- A. E. Cleves and A. N. Jain, Chemical and protein structural basis for biological crosstalk between PPARα and COX enzymes, J. Comput. Aided Mol. Des., 2015, 29, 101–112, DOI:10.1007/s10822-014-9815-2.
- S. D. Oniga, L. Pacureanu, C. I. Stoica, M. D. Palage, A. Crăciun, L. R. Rusu, E.-L. Crisan and C. Araniciu, COX Inhibition Profile and Molecular Docking Studies of Some 2 (Trimethoxyphenyl)-Thiazoles, Molecules, 2017, 22, 1507, DOI:10.3390/molecules22091507.
- M. Wannick, S. Bezdek, N. Guillen, M. Thieme, F. Meshrkey, S. Mousavi, M. Seeling, F. Nimmerjahn, A. Mócsai, D. Zillikens, T. Sezin and C. D. Sadik, Oral administration of the selective GPR120/FFA4 agonist compound A is not effective in alleviating tissue inflammation in mouse models of prototypical autoimmune diseases, Pharmacol. Res. Perspect., 2018, 8, e00438, DOI:10.1002/prp2.438.
- F. Rubbino, V. Garlatti, V. Garzarelli, L. Massimino, S. Spanò, P. Iadarola, M. Cagnone, M. Giera, M. Heijink, S. Guglielmetti, V. Arena, A. Malesci, L. Laghi, S. Danese and S. Vetrano, GPR120 prevents colorectal adenocarcinoma progression by sustaining the mucosal barrier integrity, Sci. Rep., 2022, 10, 381, DOI:10.1038/s41598-021-03787-7.
- S. V. Shmakov, D. K. Latypova, T. V. Shmakova, A. A. Rubinshtein, M. V. Chukin, S. G. Zhuravskii, N. A. Knyazev, A. V. Stepakov, M. M. Galagudza and V. M. Boitsov, Biological evaluation of 3-azaspiro[bicyclo[3.1.0]hexane-2,5'-pyrimidines] as potential antitumor agents, Int. J. Mol. Sci., 2022, 23, 10759, DOI:10.3390/ijms231810759.
- F. Başoğlu, N. Ulusoy-Güzeldemirci, G. Akalın-Çiftçi, S. Çetinkaya and A. Ece, Novel imidazo[2,1-b]thiazole-based anticancer agents as potential focal adhesion kinase inhibitors: Synthesis, in silico and in vitro evaluation, Chem. Biol. Drug Des., 2021, 98, 270–282, DOI:10.1111/cbdd.13896.
- S. Cuzzocrea, M. C. McDonald, E. Mazzon, D. Siriwardena, G. Calabrò, D. Britti, G. Mazzullo, A. De Sarro, A. P. Caputi and C. Thiemermann, The tyrosine kinase inhibitor tyrphostin AG126 reduces the development of acute and chronic inflammation, Am. J. Pathol., 2000, 157, 145–158, DOI:10.1016/S0002-9440(10)64526-9.
- R. M. AlSaffar, S. Rashid, S. B. Ahmad, M. U. Rehman, I. Hussain, S. Parvaiz Ahmad and M. A. Ganaie, D-limonene (5 (one-methyl-four-[1-methylethenyl]) cyclohexane) diminishes CCl4 induced cardiac toxicity by alleviating oxidative stress, inflammatory and cardiac markers, Redox Rep., 2022, 27, 92–99, DOI:10.1080/13510002.2022.2062947.
- P. R. Prasad, H. B. Singh and R. J. Butcher, Synthesis, structure and antioxidant activity of cyclohexene-fused selenuranes and related derivatives, Molecules, 2015, 20, 12670–12685, DOI:10.3390/molecules200712670.
- N. Onul, O. Ertik, N. Mermer and R. Yanardag, Synthesis and antioxidant, antixanthine oxidase, and antielastase activities of novel N,S-substituted polyhalogenated nitrobutadiene derivatives, J. Biochem. Mol. Toxicol., 2018, 32, e22021, DOI:10.1002/jbt.22021.
- J. A. Berliner and J. W. Heinecke, The role of oxidized lipoproteins in atherogenesis, Free Radic. Biol. Med., 1996, 20, 707–727, DOI:10.1016/0891-5849(95)02173-6.
- A. S. Abdelbaky, T. A. Abd El-Mageed, A. O. Babalghith, S. Selim and A. M. H. A. Mohamed, Green synthesis and characterization of ZnO nanoparticles using pelargonium odoratissimum (L.) aqueous leaf extract and their antioxidant, antibacterial and antiinflammatory activities, Antioxidants, 2022, 11, 1444, DOI:10.3390/antiox11081444.
- R. J. Kulmacz and W. E. Lands, Requirements for hydroperoxide by the cyclooxygenase and peroxidase activities of prostaglandin H synthase, Prostaglandins, 1983, 25, 531–540, DOI:10.1016/0090-6980(83)90025-4.
- S. Y. Jadhav, R. B. Bhosale, S. P. Shirame, S. B. Patil and S. D. Kulkarni, PEG mediated
synthesis and biological evaluation of asymmetrical pyrazole curcumin analogues as potential analgesic, antiinflammatory and antioxidant agents, Chem. Biol. Drug Des., 2015, 85, 377–384, DOI:10.1111/cbdd.12416.
-
https://www.rcsb.org/structure/4COX
.
-
https://www.rcsb.org/structure/3KK6
.
|
This journal is © The Royal Society of Chemistry 2024 |
Click here to see how this site uses Cookies. View our privacy policy here.