DOI:
10.1039/D3RA08653D
(Paper)
RSC Adv., 2024,
14, 7572-7581
Design and preparation of novel antioxidant and antibacterial films containing procyanidins and phycocyanin for food packaging†
Received
19th December 2023
, Accepted 26th February 2024
First published on 4th March 2024
Abstract
The purpose of this study was to design a novel antioxidant and antibacterial film for food packaging using food-grade raw materials. The films were designed and fabricated based on carboxymethyl chitosan and pectin incorporated with procyanidins (PCs) and phycocyanin (Phy) by the tape casting method. The effects of different proportions of PCs and Phy on the properties and functions of the prepared films were studied. The results showed that the thickness of films could range from 55 to 70 μm, with dense network structure and uniform distribution of elements. Compared with C-Film group, the film loaded with PCs and Phy had lower water solubility and swelling rate, and higher tensile strength and elongation at break. FITR and XRD spectra revealed the molecular interaction mechanism among carboxymethyl chitosan, pectin, PCs and Phy, which could effectively endow the films with ultraviolet barrier properties. Moreover, the addition of PCs and Phy could effectively improve the antioxidant capacity and antibacterial effect of films, for example, the free radical scavenging abilities of most films were above 80% when the concentration of PCs was 40 μg mL−1. In view of these functional properties, the prepared film containing PCs and Phy have been successfully used in food packaging, which was proved by the preservation experiment of grapes. This study can provide theoretical and technical guidance for the preparation of biodegradable antibacterial films, and their application in the food packaging field.
Introduction
Packaging films are of great significance for food processing, transportation, storage and marketing. Traditional food packaging materials are mainly composed of petroleum-based non-biodegradable polymers, and they have negative impacts on the environment.1 Considering that packaging films need to be in contact with the surface of food, it may be more acceptable if these films are prepared using natural, safe, renewable and biodegradable food-grade biopolymers.2 For example, polysaccharide, protein and starch are all effective candidates to replace petroleum-based polymers. However, compared with plastic packaging, these biopolymers used alone have some shortcomings such as poor mechanical resistance and water vapor barrier performance, which limit their application in packaged food.3,4 How to design and fabricate green edible films using these biopolymers, in addition to improving their physicochemical properties, and endowing them with high antioxidant and long-term antibacterial functions, is the focus of attention in this field.
Carboxymethyl chitosan (CMCS), as an important amphiphilic derivative of chitosan, can be used in the food field after being approved by FDA. CMCS possesses many advantages, such as nontoxic, biodegradable, biocompatible and antibacterial ability in a wide pH range (3–11), making it an ideal material for the preparation of film.5,6 For instance, Zimet et al. reported that the introduction of CMCS in chitosan films could improve the physicochemical properties and enhance the antibacterial activity of films.7 However, pure CMCS film is fragile and hydrophilic, and has poor antioxidant ability, which seriously limits its large-scale application in the field of food packaging.8 Fortunately, the strengthened biopolymer-based films can be obtained by the combination of different biological macromolecules in a convenient and green way, so that the films have better mechanical properties and biological activity. Pectin is one of the most abundant edible polysaccharides extracted from cell walls of most plants and fruits.9 Because of the biocompatibility, biodegradability, gelatinization and film-forming abilities, pectin is an ideal biopolymer suitable for developing bio-based food packaging films.10,11 As an anionic polysaccharide, a large number of negatively charged carboxyl groups in the chemical structure of pectin, which can be combined with CMCS by electrostatic interaction to form new films. However, it is necessary to introduce additional antioxidant and antibacterial agents to enhance the functional performance of films.
The so-called active intelligent packaging is designed by deliberately adding some functional ingredients, such as organic acids and polyphenols, into the packaging system. Procyanidins (PCs), a kind of phenolic compounds, account for more than 90% of the total commercial tannin production. The existence of hydroxyl groups in PCs determines their strong antioxidant activity.12,13 They also have many physiological activities such as bacteriostasis, anti-inflammation, weight loss and anti-hypertension activities, so they can be used as food packaging materials. For example, PCs could destroy the integrity and permeability of cell wall and membrane of Staphylococcus aureus, pathogenic Escherichia coli and other bacteria, and then affect protein synthesis or combine with DNA to exert their antibacterial function.14,15 Phycocyanin (Phy), as another food active ingredient with antioxidant and antimicrobial activity, mainly isolated from cyanobacteria, rhodophyta or glaucophyta in the ocean.16,17 This protein is one of the rare blue pigment proteins in nature, which is rich in nutrition, safe and non-carcinogenic, so it can be widely used as colorant or additive in food, cosmetics and medicines.18 Chentir et al. used Phy mix with gelatin to obtain film with effective antibacterial activity against Gram-positive and Gram-negative bacteria.19 However, the synergistic antioxidant and antimicrobial effect between PCs and Phy has not been studied.
Therefore, the aim of this paper was to prepare a novel biodegradable film, using CMCS and pectin as film matrix, PCs and Phy as colorants, antioxidant and antimicrobial agents to prolong the preservation time of food. The effects of different mass ratio of PCs and Phy on the chemical structure, surface morphology, water resistance, mechanical properties, optical property and thermal stability of the composite films were characterized. Furthermore, the scavenging free radicals and inhibiting ability to Gram-positive and Gram-negative harmful bacteria were studied, and the synergistic effect between PCs and Phy was analyzed. Finally, the application of this film on food preservation was discussed using grape and fresh-cut apple as model. This research is expected to provide strategic and technical guidance for the preparation and application of food-grade antibacterial films.
Experimental
Materials
Procyanidins (PCs, purity ≥ 95%) were bought from Tianjin Jianfeng Co., Ltd (Tianjin, China). Phycocyanin (Phy) was bought from Zhejiang Binmei Biotechnology Co., Ltd (Taizhou, China). Pectin, glycerol, and carboxymethyl chitosan (CMCS) were obtained from Shanghai Aladdin Biochemical Technology Co., Ltd (Shanghai, China). Escherichia coli (E. coli, CVCC 1527) and Staphylococcus aureus (S. aureus, CGMCC 12155) were all from the strains collected in laboratory.
Preparation of PCs and Phy-based films
The preparation process of films (Fig. 1) was as follow: CMCS solution (1 wt%) was obtained by dispersing 1 g CMCS sample in distilled water, and then adding different weights of PCs (0, 15, 30, 45 and 60 mg) in 15 mL CMCS solution to obtain PC–CMCS solution. Subsequently, pectin and glycerol were uniformly dispersed in 100 mL water to obtain 2 wt% pectin solution, followed by adding different mass of Phy (0, 15, 30, 45 and 60 mg) in 15 mL pectin solution to obtain Phy–pectin solution. The two solutions were cross-mixed and stirred for 2 min to obtain a uniform film-forming solution, in which the mixing principle of two solutions was that the total mass of PCs and Phy was 60 mg. The mixed solution of CMCS and pectin without PCs and Phy was used as control. The fluorescence and ultraviolet visible (UV-vis) spectra of different film-forming solutions were measured by Cary Eclipse fluorescence spectrometer (Agilent, America) and UV-vis absorption spectrophotometer UV2600 (Shimadzu, Japan), respectively.
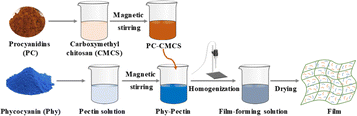 |
| Fig. 1 Schematic illustration of the fabrication of film-forming solution and PCs-Phy films. | |
The above-mentioned film-forming solutions were slowly added into glass dishes (6 cm × 6 cm), and dried in an oven at 30 °C for 18 h. Subsequently, the dried films were manually stripped from casting surface, and stored at room temperature and 50% relative humidity for analysis. The film without PCs and Phy was named C-Film, and the films with PCs of 60, 45, 30, 15 and 0 mg and the corresponding Phy of 0, 15, 30, 45 and 60 mg were named PPF-1, PPF-2, PPF-3, PPF-4 and PPF-5.
Morphology characterization of films
The surface morphology of C-Film, PPF-1, PPF-2, PPF-3, PPF-4 and PPF-5 was analyzed by TM3030Plus scanning electron microscope (SEM, Hitachi, Japan). Films were observed up to 200× and 2000× magnification at an accelerating voltage of 5 kV. The elements on the surface of different films were mapped by using an X-MaXN Energy-Dispersive Xray spectrometer (EDS, Oxford, England).
Dry weight, water solubility and swelling ratio
An approximately 2 cm × 2 cm film sample was dried at 105 °C, until a constant weight value was achieved. Dry weight was the constant weight divided by the initial weight, and expressed in %.
The experimental conditions of water solubility of film were referred to the method reported by Zhang et al. with slightly modification.20 The above-mentioned film with constant weight was soaked at 25 °C for 12 h, and other conditions remained unchanged.
To measure the swelling rate of film, film with constant weight was put in distilled water for 1 h. Then, the film was taken out of water, and the excess water on the surface of film was absorbed and weighed. Swelling ratio was analyzed using the eqn (1):
|
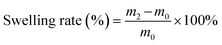 | (1) |
where
m0 standards for the initial constant weight, and
m2 standards for the weight after immersion in water for 1 h.
Structure and thermal stability of films
The possible molecular interactions in film including PCs, Phy, CMCS and pectin were recorded by a VERTEX70 Fourier transform infrared spectroscopy (FTIR, Bruker, Germany). The film was cut into small pieces, mixed with dried KBr, ground and pressed into a pellet. X-ray diffraction (XRD) curves of PCs, Phy, CMCS, pectin and films in the ranges of 5 to 80° (2θ) were characterized by XRD-6100 instrument (Shimadzu, Japan) at a scanning speed of 8° min−1. The thermal stability of films was analyzed via differential scanning calorimetry (DSC)-1 instrument (Mettler-Toledo, Germany).
Mechanical properties
The tensile strength (TS) and elongation at break (EAB) of the prepared films were measured by tensile analyzer to reflect the mechanical properties of the films. The films were cut into 1 cm × 4 cm rectangular slices, and tested at a speed of 50 mm min−1 until the breakage occurred. The TS and EAB of films were calculated according to the following equations: |
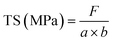 | (2) |
where F represents the maximum stress (N) that the film can withstand when breaking, a is the thickness of film (mm), and b is the width of film (mm). |
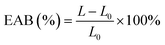 | (3) |
where L is the length of films reached at the time of fracture (mm), and L0 is the initial length (mm).
Color, opacity and UV-barrier properties determination
The color of films as a function of PCs and Phy ratio were recorded by using a Color 15 colorimeter (X-Rite, America) equipped with standard color plate for calibration. The experimental parameters are as follows: D65 standard light source, 10° plane measurement, and 10 mm measuring aperture. The obtained color parameters (L*, a* and b*) could be used to calculate the total differences of film colors (ΔE), as follows (4): |
 | (4) |
The measuring method of opacity of films was referred to that of Bai et al., that was, inserting rectangular film samples into a cuvette, and the ultraviolet absorption value was measured by of UV-vis absorption spectrophotometer.21 The formula for calculating the opacity of different films was as follows (5):
where
A600 is the absorption value of films at 600 nm, and
D standards for the thickness (mm) of films.
The transmittance and absorbance spectra of different films was recorded by UV-vis absorption spectrophotometer.
Antioxidant capacity
Antioxidant performance of the prepared films was reflected by the radical scavenging assay of DPPH and ABTS. Firstly, 10 mg film was dissolved in water, and incubated overnight away from light to obtain colloidal solution. Subsequently, the solution was diluted to different concentrations, and the antioxidant capacity was measured according to the DPPH and ABTS scavenging free radical experiments previously reported by Tie et al.22
Antibacterial test
E. coli and S. aureus were taken as the representative food susceptible strains to assess the antibacterial performance of films. The growth changes of food harmful bacteria incubated in the culture medium were studied by Qin et al.23 Two kinds of bacteria with certain inoculation amount were transferred to the corresponding medium containing the colloidal solution of film, and then incubated at 37 °C for 12–16 h with mild shaking. The inhibition effect was evaluated by recording the turbidity of culture medium periodically at 600 nm by UV-vis spectrophotometer.
Application of film in food packaging
Fresh grapes and apples were bought from the local Dazhang supermarket (Luoyang, China). Grapes were packed with C-Film, PPF-1, PPF-2, PPF-3, PPF-4 and PPF-5 films, respectively, and the unpacked were selected as blank control group. Grapes of different treatment groups were exposed to the conditions of 25 °C for 7 d. During this period, grapes in each group were exposed to UV and water every day to simulate harsh conditions, that was 2 h of UV rays and 2 min of small water rinsing, and compared the decay of grapes.
Fresh apples were sliced and packaged with the prepared films. The samples were exposed to UV for 2 h every day at room temperature, and the changes of appearance, color and weight of apples were observed after exposure for 3 d.
Evaluation of the biodegradability of films
Soil and river water from riverside were used as simulated soil and water to test the biodegradability of films. The films were cut into 2 cm × 2 cm square slices, weighed, placed in soil to analyse the weight change of residual film samples at room temperature, and placed in river water to observe the dispersion of films.
Statistical analysis
The measurements of physicochemical indexes, antioxidant and antibacterial capacities were measured at least three times, and experimental data were expressed by mean ± standard deviation. The significant difference of values (p < 0.05) was analyzed by one-way analysis of variance (ANOVA) in SPSS 16.0 software.
Results and discussion
Appearance and characterization of film-forming solution
PCs and Phy were red-brown and blue active ingredients, respectively, when they were added into CMCS and pectin solutions, the prepared film-forming solutions could show different colors. As shown in Fig. 2A, C-Film samples without PCs and Phy are beige yellow, PPF-1 and PPF-5 with PCs and Phy are reddish brown and blue, respectively, while PPF-2, PPF-3 and PPF-4 mixed with PCs and Phy are brown in different degrees. Subsequently, fluorescence and UV-vis spectra were used to analyze whether the optical properties of PCs and Phy in the films changed. The fluorescent spectrum of Phy showed an emission peak at 652 nm, and PPF-5 had a similar peak at about 650 nm, but the peak intensity was low at the same concentration (Fig. 2B). Moreover, PPF-2, PPF-3 and PPF-4 films containing both PCs and Phy did not show similar emission peaks, indicated that the presence of PCs could quench the fluorescence of Phy. In UV-vis absorption spectra, there were two absorption peaks of Phy were observed belonging to tryptophan (277 nm) and tyrosine residues of tetrapyrrole (616 nm), and one absorption peak (280 nm) of PCs was attributed to the conjugate structure of A-ring and B-ring (Fig. 2C).24 All the films had the absorption peak of PCs and Phy at 280 nm (Fig. 2D). The only difference was that PPF-5 film containing Phy could shift to 614 nm at tyrosine residue, compared with the absorption of Phy. These results indicated that the co-existence of PCs and Phy could affect the fluorescence and UV-vis spectra properties.
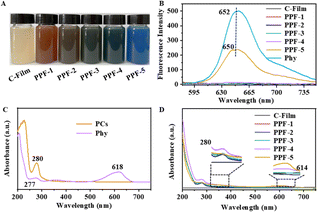 |
| Fig. 2 (A) The appearance of film-forming solution. (B) Fluorescence spectra of C-Film, PPF-1, PPF-2, PPF-3, PPF-4, PPF-5 and Phy. Ultraviolet visible (UV-vis) spectra of (C) PCs, Phy, and (D) C-Film, PPF-1, PPF-2, PPF-3, PPF-4, PPF-5. | |
Optical pictures and surface microstructure of films
The film-forming solutions were added into molds and dried to obtain films, and the color of films were shown in Fig. 3A, which were consist with the film-forming solutions. Subsequently, in order to better understand the effect of the addition of active ingredients on the morphology of films, the morphology was recorded by SEM. The surface of C-Film was rough, with uneven size and relatively inconspicuous spherical structure (Fig. 3B). When PCs were added into the film-forming solution, the surface of PPF-1 film was uniform spherical structure, and there were gaps between different spheres. The surface of PPF-5 films with only Phy was relatively homogeneous and dense, with only a small amount of spherical structure and no cracks, and this might be related to the molecular interaction between protein and polysaccharide. This phenomenon was basically consistent with the surface morphology changes described by Tavakoli et al., the films prepared by mixing anthocyanins and other active components with gelatin and soybean polysaccharide.25 PPF-2, PPF-3 and PPF-4 films contained PCs and Phy at the same time, and a wrinkle similar to network structure was attached with their surfaces, which could effectively ensure the compactness of the films. This indicated that the cross-linking action between PCs and proteins might improve the properties of films.
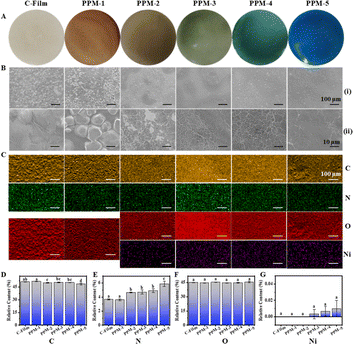 |
| Fig. 3 (A) Optical images, (B) surface microstructure and (C) element mapping images of C-Film, PPF-1, PPF-2, PPF-3, PPF-4 and PPF-5, and Bii are the magnified images of Bi. The relative element content analysis of (D) C, (E) N, (F) O and (G) Ni in different films. | |
Additionally, the element spectra and mapping images in Fig. S1† and 3C show that C-Film and PPF-1 films are composed of C, N and O elements, and the distribution of C and O elements has obvious convex structure. PPF-2, PPF-3, PPF-4 and PPF-5 films also contained Ni in addition to the above-mentioned elements, and the distribution of these elements were relatively uniform. Fig. 3D–G show the contents of C, N, O and Ni, and the relative contents of N and Ni in PPF-5 film were relative higher. The results proved that the films containing PCs and Phy were successfully prepared.
Physical properties of the prepared films
Thickness is an important parameter that it can directly affect the coating function of films. Compared with C-Film (69.81 μm), the addition of active ingredients significantly reduced the thickness of films (Fig. 4A). When the ratio of PCs to Phy was 1
:
1, the thickness of PPF-3 film was the lowest, 55.65 μm, which might be due to the gaps on the surface of CMCS-pectin films filled with PCs and Phy.26
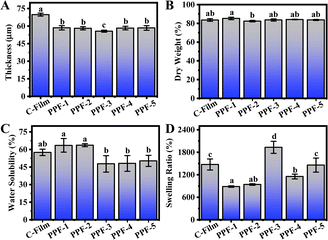 |
| Fig. 4 (A) Thickness, (B) dry weight, (C) water solubility and (D) swelling ratio of C-Film, PPF-1, PPF-2, PPF-3, PPF-4 and PPF-5 films. | |
Packaging films should maintain a certain moisture level in the packaging products, so it is of great significance to know the moisture barrier performance of film for its application. Water resistance properties, including dry weight, solubility and swelling behavior of the edible bioactive film were characterized, and results were presented in Fig. 4B–D. Dry weight of C-Film in the control group was 83.62%, and the dry weight of PPF-1 to PPF-5 films prepared by the incorporation of PCs and Phy changed slightly compared with that of C-Film, but remained in the range of 82.41 to 85.30% (p < 0.05). Water solubility, as one of the important indexes to judge the usability of packaging materials, increased significantly in PPF-1 film containing only PCs compared with that of C-Film, which might be related to a large number of free hydroxyl groups in PPF-1 film and the high hydrophilicity of PCs. However, the water solubility of the prepared films of PPF-2 to PPF-5 decreased with the decrease of PCs content, and the lowest water solubility of PPF-3 and PPF-4 were 47.72 and 48.09%. This indicated that the hydrophobicity of the composited films was increased by a small number of aromatic rings in the structure of PCs and the interaction between PCs and Phy. Generally, the lower water solubility indicated that the prepared film has higher water resistance performance.27,28 In order to further measure the water resistance of these films, swelling ratio of films in water was measured. As shown in Fig. S2,† when the prepared films were soaked in distilled water for 1 h, C-Film was partially dissolved, PPF-1 and PPF-2 films containing more PCs were only partially sampled due to the solubility of PCs in water, while PPF-3, PPF-4 and PPF-5 could maintain their integrity well, which was consistent with the above-mentioned water solubility results. Compared with PPF-3 and PPF-5 films, the swelling ratio of PPF-4 films prepared was relatively low (Fig. 4D). The analysis results of water resistance showed that the composite films prepared with the mass ratio of 1
:
1 or 1
:
3 of PCs and Phy had relatively low water solubility and swelling ratio, and could maintain the integrity of the films well.
Structure and thermal stability analysis of films
FTIR spectroscopy was used to analyze the interaction of natural CMCS, pectin, PCs and Phy, and the functional groups and structural properties of the prepared films. FTIR spectra of biopolymers and films containing different masses of PCs and Phy at the selected wavenumber range of 400–4000 cm−1 were presented in Fig. 5A–C. The band of CMCS at 3412 cm−1 belonged to the stretching vibration of O–H and NH2, and the typical peak at 2910 cm−1 were assigned to the stretching vibration of –CH. The asymmetric and symmetric peaks of carboxyl group in CMCS appeared at 1608 and 1434 cm−1, and the stretching vibration of C–O was attributed to the peak at 1068 cm−1. For pectin, the bands at 3450 and 2936 cm−1 were responsible for O–H vibration of carboxyl group and C–H vibration of methyl group. The bands at 1756, 1644 and 1448 cm−1 belonged to C
O components of ester bonds, asymmetric and symmetric stretching vibrations of carboxyl groups in pectin structure, respectively (Fig. 5A). However, compared with CMCS and pectin, the absorption peaks of C-Film prepared by these two macromolecules were red-shifted at 3390, 1612 and 1413 cm−1, which was related to the changes of O–H, COOH and NH2 groups caused by the intermolecular hydrogen bonds and amide bonds.29 The characteristic peaks of PCs at 3374 and 2925 cm−1 were belonged to O–H and C–H vibration, and the peaks at 1608, 1519 and 1442 cm−1 were responsible for C
C skeleton of benzene rings (Fig. 5B). FTIR spectra of Phy showed that the spectral bands of pure Phy at 3313 cm−1 for N–H and O–H vibration, 1658 cm−1 for C
O vibration, 1543 cm−1 for C
C and C–N vibration, 1400 cm−1 for carboxyl vibration, and 1248 cm−1 for C–N in-plane bending and N–H vibration.30 Compared with C-Film, the characteristic peak of PPF-1 film prepared with only PCs appeared at 1527 cm−1 was the C
C skeleton of benzene rings of PCs (Fig. 5C). Subsequently, with the addition of different masses of Phy, PPF-2, PPF-3, PPF-4 and PPF-5 films also showed similar characteristic peaks with slight shift, and no other peaks of PCs and Phy in these films, indicating that they were well distributed in these films.
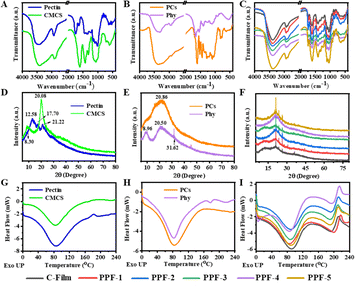 |
| Fig. 5 (A–C) FTIR spectra, (D–F) XRD spectra and (G–I) DSC curves of CMCS, pectin, PCs, Phy, C-Film, PPF-1, PPF-2, PPF-3, PPF-4 and PPF-5 films. | |
The crystal difference between the raw materials and the prepared films were analyzed by XRD, the crystalline material could possess several sharp peaks, and amorphous material could show a wide background pattern. As shown in Fig. 5D, pure CMCS shows two broad peaks at 8.30 and 20.08°, and pectin displays three diffraction peaks at 12.58, 17.70 and 21.22°, respectively, which were similar to Sari's previous work.31 The C-Film had a broad peak around 21.00°, and three sharp peaks at 21.02, 23.40 and 26.16°, indicated that C-film possessed the crystal and amorphous crystal structure of the biological macromolecules used. Moreover, there was a broad peak at 2θ value of 20.86° for PCs, and several diffraction peaks at 8.96, 20.50 and 31.62° for Phy (Fig. 5E). The films prepared by PCs and Phy showed similar diffraction peaks with C-Film, and different proportions of PCs and Phy did not cause obvious changes of XRD peaks (Fig. 5F).
It is important to evaluate the thermal stability of film from the perspective of food packaging, which is helpful to analyze the heat resistance of film during sterilization or microwave heating.10 As shown in Fig. 5G–I, the first endothermic peaks of CMCS, pectin, PCs, Phy and the prepared films were all around 85 °C, and the peak values of films were higher than that of raw materials. This change might be due to the evaporation of water in materials or the denaturation of Phy. There was a single endothermic peak at 177 °C in the DSC curve of Phy, and it might be corresponded to the melting point of the crystal region of Phy. However, this endothermic peak was not found in the films of PPF-2–PPF-5 with Phy, indicated that Phy was successfully loaded into the CMCS-pectin film, resulting in the decrease or even disappearance of crystallinity. The exothermic peaks appeared at 212, 217, 215, 210, 214 and 211 °C for C-Film, PPF-1, PPF-2, PPF-3, PPF-4 and PPF-5, respectively, and the peak values of films were close. This might be related to the depolymerization and thermal decomposition of polysaccharide or protein skeleton, and no obvious difference was observed in different films.
Mechanical properties of films
The mechanical properties of films mainly depend on the composition of films, the intermolecular and intramolecular interactions. The type and concentration of components in the film-forming matrix will affect the interaction between molecules. The influence of different mass ratios of PCs and Phy on the mechanical properties of the prepared films is shown in Fig. 6. With the increase of Phy, the film had higher tensile strength, which was related to the interaction among protein, polysaccharide and polyphenols. When the mass ratio of PCs to Phy was 1
:
3, the PPF-4 film had high tensile strength (34.12 MPa) and elongation at break (93.09%), so might possess great potential application in food packaging.
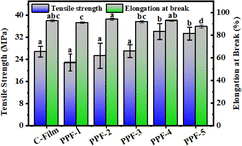 |
| Fig. 6 The tensile strength and elongation at break of C-Film, PPF-1, PPF-2, PPF-3, PPF-4 and PPF-5 films. | |
Color, opacity and UV-barrier properties of films
Color and opacity are important indexes to assess the physicochemical characteristics of bioactive films. The color properties of bioactive films were recognized as important indexes of packaging materials included appearance, utilization and consumer acceptance, and the influence of different PCs and Phy incorporation ratios on color parameter of film was shown in Fig. 7A–C and S3.†32 The results showed that, compared with C-Film, the brightness (L*) of PPF-1∼5 films decreased with the decrease of PCs and the increase of Phy, the redness/greenness (a*) and yellowness/blueness (b*) values also showed a similar trend. After adding PCs, the color of PPF-1 film changed from light color (C-Film) to reddish brown, the composite films of PPF-2, PPF-3 and PPF-4 gradually tended to yellow-green color, and PPF-5 film containing only Phy was blue (p < 0.05). This trend was consolidated by the color difference ΔE values, and a great significant difference existed in the prepared films with the change of the ratio of PCs to Phy. Moreover, opacity of films was assessed according to their absorbance at 600 nm. As shown in Fig. 7D, the opacity values of C-Film and PPF-1 films are lower, 0.53 and 1.86, respectively, and the opacity of the prepared films increases significantly with the incorporation of Phy. Chentir et al. also obtained a similar experimental trend when studying the influence of different concentrations of Phy on the transparency of gelatin films.19 The results showed that the opacity value of PPF-3 film prepared with the mass ratio of PCs to Phy of 1
:
1, was relatively low at 3.06.
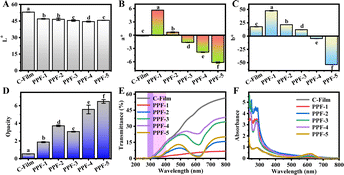 |
| Fig. 7 Color parameters included (A) L*, (B) a* and (C) b*, (D) opacity, (E) light transmittance, (F) absorbance of C-Film, PPF-1, PPF-2, PPF-3, PPF-4 and PPF-5 films. | |
Furthermore, if the packaging film possesses UV barrier ability, it could effectively avoid food oxidation, discoloration and nutrient loss, and the barrier ability of films was evaluated by measuring light transmittance and absorption values. As shown in Fig. 7E, C-Film shows relatively high transmittance in the UV (200–400 nm) and visible (400–800 nm) regions. With the addition of PCs, the barrier property of PPF-1 film was higher than that of C-Film, which could be attributed to the strong absorption of benzene ring and phenolic hydroxyl groups in the structure of PCs. However, PPF-2, PPF-3 and PPF-4 films prepared with different proportions of PCs and Phy had lower UV barrier properties at 200–400 nm than that of PPF-1, suggesting that the interaction between PCs and Phy could lead to the decrease of UV absorption ability. PPF-5 film containing only Phy had relatively good UV barrier in the range of 200–400 nm, and lower transmittance in visible region about 620 nm. Moreover, the ultraviolet absorption spectra shown in Fig. 7F displayed that PPF-1–5 films prepared by PCs and Phy had higher absorbance in the region of 200–400 nm, and further proved that the prepared films had higher ultraviolet barrier performance.
Antioxidant capacity analysis
The oxidation resistance of C-Film, PPF-1, PPF-2, PPF-3, PPF-4 and PPF-5 were evaluated by DPPH and ABTS antioxidant assays. As shown in Fig. 8A, C-Film without PCs exhibit poor antioxidant capacity, and no concentration-dependent antioxidant behavior is found. The antioxidant capacity of PPF-5 was higher than that of C-Film, the sample containing different concentrations of Phy were still purple and its scavenging ability was lower than 20% (Fig. S4A†). The DPPH scavenging ability of PPF-1, PPF-2, PPF-3 and PPF-4 was positively correlated with the concentration of PCs, and displayed a dose dependence behavior in the range of 0–20 μg mL−1. For the concentration of PCs at 40 μg mL−1, the scavenging rate of films was over 80% except PPF-4. However, at the same concentration of PCs, the radical reducing ability of PPF-2, PPF-3 and PPF-4 films prepared by the combination of PCs and Phy was lower than PPF-1 film containing only PCs due to the interaction between polyphenols and protein. In previous studies, they also reported that the phenolic hydroxyl groups of PCs could interact with protein, thus affecting the antioxidant performance of active ingredients.33 The ability of scavenging ABTS free radicals was evaluated by recording the absorbance value at 734 nm. The green color of PPF-1, PPF-2, PPF-3 and PPF-4 films gradually decreased with the increase of PCs concentration, suggesting that the antioxidant capacity increased (Fig. S4B† and 8B). Moreover, the scavenging property of PPF-3 was slightly higher than that of PPF-4 film (p < 0.05). The results of antioxidant activity experiments proved that the addition of active ingredients in CMCS-pectin film could significantly improve the antioxidant performance of films.
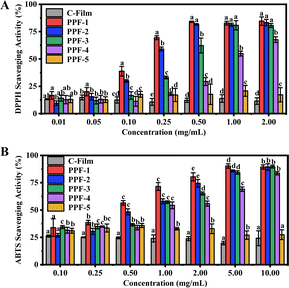 |
| Fig. 8 (A) 1,1-Diphenyl-2-picrylhydrazyl (DPPH) and (B) 2,2-azino-bis(3-ethylbenzothiazoline-6-sulfonic acid) (ABTS) scavenging ability of C-Film, PPF-1, PPF-2, PPF-3, PPF-4 and PPF-5. | |
Antibacterial activity analysis
Bacterial proliferation is one of the important factors causing food spoilage. Therefore, it is necessary to know the antibacterial capacity of films applied to food packaging. In this experiment, E. coli and S. aureus were used as representative bacteria to assess the antibacterial capacity of films with different ratios of PCs and Phy, and the results were presented in Fig. 9. Pure CMCS-pectin film exhibited a certain inhibitory effect on E. coli and S. aureus due to the good antibacterial ability of CMCS compared with the control group. With the addition of PCs and Phy, the antibacterial capacity of the prepared films increased, and PPF-3 film prepared by 1
:
1 ratio of PCs to Phy had higher antibacterial activity than PPF-1 and PPF-5 films prepared by PCs or Phy alone. It had been reported that PCs would not inhibit or even promote the growth of bacteria at low concentrations, but they would play an inhibitory role at relatively high concentrations. However, when mixed with water-soluble polysaccharide, the mixture showed an obviously synergistic inhibitory effect against E. coli, and improved the adverse effects of PCs at low concentration to E. coli.34 Therefore, the films obtained by mixing PCs and Phy might exert its antibacterial effect better. The chemical bonds between PCs and proteins were non-covalent interaction, which belonged to reversible reaction and would not destroy the structural integrity of PCs and Phy.35
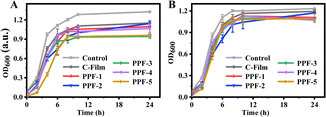 |
| Fig. 9 The growth curves of (A) E. coli (CVCC 1527) and (B) S. aureus (CGMCC 12155). | |
Application analysis of films in food packaging
The above studies showed that the prepared films had UV blocking, antioxidant and antibacterial effects, and the potential application of bioactive film as packaging material was further verified by taking grapes as samples. The optical pictures of grapes and packaging films before and after storage for 7 d was presented in Fig. 10, and observation results showed that compared with air and paper, the prepared films could effectively slow down the water loss of grapes. However, compared with C-Film, PPF-1 and PPF-2 films were partially dissolved after washing with water, which would cause water loss and wrinkles on the grape surface. Other films such as PPF-3, PPF-4 and PPF-5 did not show this phenomenon, suggesting that they had good water barrier performance. After ultraviolet irradiation, all groups of grapes except those packed with PPF-4 film appeared different degrees of discoloration and corruption, indicated that PPF-4 film could effectively block ultraviolet rays. Moreover, grapes wrapped with PE film could maintain the moisture of grapes, but the surface color changed after 7 d of ultraviolet irradiation. Grapes wrapped with tinfoil almost did not change, which was basically consistent with that of PPF-4 packaging. The prepared bioactive films could prolong the shelf life of grapes significantly, thus had a wide potential application prospect in food storage.
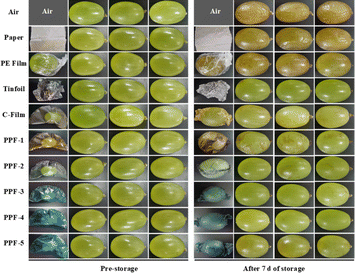 |
| Fig. 10 Visual changes of grapes packed with different packaging materials after 7 d of storage. | |
Moreover, the application of the prepared films in fresh-cut fruits was also studied. For fresh-cut fruits, color was a key sensory parameter, so the easily browned apples were selected as a model to analyse the performance of films. As shown in Fig. S5A,† the surface of untreated fresh-cut apples appears browning, dehydration and wrinkling after being stored for 3 d. The browning degree of apples coated with the prepared films was lower, but there was also a phenomenon of water loss, which was higher than that of apples wrapped with PE film and tinfoil. The specific degree of color change was shown in Fig. S5B–E,† after 3 days of storage, the color parameters of uncoated apples changed significantly, that was, the brightness decreased, and the red and yellow values increased. The color degree of apples coated with PPF-1 film containing only PCs was relatively deepened, which was related to the release of PCs caused by water permeation into the film. However, the color change of apples coated with other films was relatively low. In addition, the weight change of apples further proved that the films had a low blocking effect on water loss in apples (Fig. S5F†). This result indicated that the application effect of the films in fresh-cut fruit was relatively poor, which was lower than that in fresh and intact fruit storage.
Biodegradability analysis of films
To prove that the obtained films was green and environmentally-friendly, the biodegradability of films was analysed by simulating soil and river water experiments. As shown in Fig. 11A, the longer the film was incubated in soil, the lighter the weight of the remaining film was. When the incubation time reached 120 h, PPF-4 and PPF-5 films were completely degraded. There was a similar phenomenon appeared when incubating with river water (Fig. 11B). PCs and Phy were released from films, and the films began to break and decompose with the incubation time prolonging. All these phenomena proved that the prepared films had good biodegradability and were friendly to the environment.
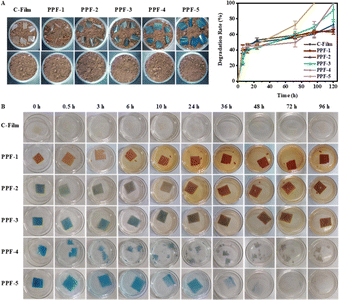 |
| Fig. 11 (A) Degradation rate and (B) visual changes of the prepared films in simulated soil and river water. | |
Overall, compared with PPF-1 and PPF-5 films prepared by single PCs and Phy, PPF-4 film containing PCs and Phy with a ratio of 1
:
3 had better ultraviolet barrier, fruit preservation and biodegradation performance. The film obtained by adding these two active ingredients in CMCS and pectin solutions had better functional properties. Jamróz et al. also obtained a similar result, that was, the incorporation of curcumin extract and lemongrass essential oil in film at the same time could effectively improve the anti-ultraviolet, antioxidant and antibacterial activities, and reducing the weight loss of tomatoes during storage.36 Zhang et al. added red cabbage pigment and enterocin CHQS into chitosan and gelatin solutions, and the obtained film had UV-blocking, antioxidant and bacteriostatic abilities. Compared with the film containing only red cabbage pigment, the addition of enterocin CHQS significantly expanded the range of the bacterial inhibition of films.37 All these proved that the films prepared with double active ingredients had better functional properties.
Conclusion
In summary, the functional films containing PCs and Phy based on food-grade CMCS and pectin were successfully prepared by the tape casting method. The films incorporated with PCs and Phy possessed dense network structures, low water solubility and swelling rate, and higher tensile strength and elongation at break. The molecular interaction among CMCS, pectin, PCs and Phy could effectively improve the ultraviolet barrier properties of the prepared films. Moreover, the addition of PCs and Phy endowed the biodegradable films strong antioxidant and antibacterial effects, thus prolonging the shelf life of grapes, but not fresh-cut fruit. This study can provide technical support for the application of antioxidant and antibacterial film in food packaging field.
Author contributions
Shanshan Tie: conceptualization, data curation, formal analysis, investigation, methodology, software, validation, writing – original draft, writing – review & editing. Qing Zhang: conceptualization, data curation, formal analysis, investigation. Yixuan Zhao: validation, formal analysis, methodology, investigation. Ying Wu: writing – review & editing. Dasu Liu: validation, formal analysis, investigation. Lina Zhao: validation, formal analysis, investigation. Shaobin Gu: project administration, resources, supervision, visualization, writing – review & editing.
Conflicts of interest
The authors declare no competing financial interest.
Acknowledgements
This work was supported by the National Science Foundation of China (32302069), the Youth Talent Support Program of Henan Province (2023HYTP009) and the General Program of the Science Foundation of Henan Province (222300420429).
Notes and references
- Z. Zou, B. B. Ismail, X. Zhang, Z. Yang, D. Liu and M. Guo, Improving barrier and antibacterial properties of chitosan composite films by incorporating lignin nanoparticles and acylated soy protein isolate nanogel, Food Hydrocolloids, 2023, 134, 108091 CrossRef CAS.
- F. Liu, Y. Liu, Z. Sun, D. Wang, H. Wu, L. Du and D. Wang, Preparation and antibacterial properties of ε-polylysine-containing gelatin/chitosan nanofiber films, Int. J. Biol. Macromol., 2020, 164, 3376–3387 CrossRef CAS.
- W. Janik, M. Nowotarski, D. Y. Shyntum, A. Banaś, K. Krukiewicz, S. Kudła and G. Dudek, Antibacterial and biodegradable polysaccharide-based films for food packaging applications: Comparative study, Materials, 2022, 15, 3236 CrossRef CAS.
- H. Dai, Y. Chen, H. Chen, Y. Fu, L. Ma, H. Wang, Y. Yu, H. Zhu and Y. Zhang, Gelatin films functionalized by lignocellulose nanocrystals-tannic acid stabilized Pickering emulsions: Influence of cinnamon essential oil, Food Chem., 2023, 401, 134154 CrossRef CAS PubMed.
- Q. Xie, D. Luo, K. Mu and W. Xue, Preparation and characterization of carboxymethyl chitosan/nano-MgO/red cabbage anthocyanins multifunctional films via colloid formation and its application on shrimp preservation, Food Packag. Shelf Life, 2023, 37, 101074 CrossRef CAS.
- J. Chen, L. Luo, C. Cen, Y. Liu, H. Li and Y. Wang, The nano antibacterial composite film carboxymethyl chitosan/gelatin/nano ZnO improves the mechanical strength of food packaging, Int. J. Biol. Macromol., 2022, 220, 462–471 CrossRef CAS PubMed.
- P. Zimet, Á. W. Mombrú, D. Mombrú, A. Castro, J. P. Villanueva, H. Pardo and C. Rufo, Physico-chemical and antilisterial properties of nisin-incorporated chitosan/carboxymethyl chitosan films, Carbohydr. Polym., 2019, 219, 334–343 CrossRef CAS PubMed.
- L. Wang, L. Lei, K. Wan, Y. Fu and H. Hu, Physicochemical properties and biological activity of active films based on corn peptide incorporated carboxymethyl chitosan, Coatings, 2021, 11, 604 CrossRef CAS.
- S. Šešlija, A. Nešić, J. Ružić, M. K. Krušić, S. Veličković, R. Avolio, G. Santagata and M. Malinconico, Edible blend films of pectin and poly(ethylene glycol): Preparation and physico-chemical evaluation, Food Hydrocolloids, 2018, 77, 494–501 CrossRef.
- R. Priyadarshi, S.-M. Kim and J.-W. Rhim, Pectin/pullulan blend films for food packaging: Effect of blending ratio, Food Chem., 2021, 347, 129022 CrossRef CAS PubMed.
- H.-X. Gao, Z. He, Q. Sun, Q. He and W.-C. Zeng, A functional polysaccharide film forming by pectin, chitosan, and tea polyphenols, Carbohydr. Polym., 2019, 215, 1–7 CrossRef CAS.
- H. Pan, X. Shen, W. Tao, S. Chen and X. Ye, Assembly of oil-based microcapsules coated with proanthocyanidins as a novel carrier for hydrophobic active compounds, J. Agric. Food Chem., 2020, 68, 5715–5722 CrossRef CAS PubMed.
- S. Ramziia, H. Ma, Y. Yao, K. Wei and Y. Huang, Enhanced antioxidant activity of fish gelatin–chitosan edible films incorporated with procyanidin, J. Appl. Polym. Sci., 2018, 135, 45781 CrossRef.
- C. Tang, B. Xie and Z. Sun, Antibacterial activity and mechanism of B-type oligomeric procyanidins from lotus seedpod on enterotoxigenic Escherichia coli, J. Funct. Foods, 2017, 38, 454–463 CrossRef CAS.
- X. Li, C. He, L. Song, T. Li, S. Cui, L. Zhang and Y. Jia, Antimicrobial activity and mechanism of Larch bark procyanidins against Staphylococcus aureus, Acta Biochim. Biophys. Sin., 2017, 49, 1058–1066 CrossRef CAS.
- D. V. Sarada, C. Sreenath Kumar and R. Rengasamy, Purified C-phycocyanin from Spirulina platensis (Nordstedt) Geitler: A novel and potent agent against drug resistant bacteria, World J. Microbiol. Biotechnol., 2011, 27, 779–783 CrossRef CAS.
- P. Yu, Y. Wu, G. Wang, T. Jia and Y. Zhang, Purification and bioactivities of phycocyanin, Crit. Rev. Food Sci. Nutr., 2017, 57, 3840–3849 CrossRef CAS PubMed.
- E. Mohammadi-Gouraji, S. Soleimanian-Zad and M. Ghiaci, Phycocyanin-enriched yogurt and its antibacterial and physicochemical properties during 21 days of storage, LWT--Food Sci. Technol., 2019, 102, 230–236 CrossRef CAS.
- I. Chentir, H. Kchaou, M. Hamdi, M. Jridi, S. Li, A. Doumandji and M. Nasri, Biofunctional gelatin-based films incorporated with food grade phycocyanin extracted from the Saharian cyanobacterium Arthrospira sp, Food Hydrocolloids, 2019, 89, 715–725 CrossRef CAS.
- X. Zhang, W. Cai, L. Hao and W. Zhang, In situ preparation and properties of poly(vinyl alcohol)/carboxymethyl chitosan/cyanidin hydrogel films, J. Nanomater., 2019, 2019, 1–8 CAS.
- M.-Y. Bai, Q. Zhou, J. Zhang, T. Li, J. Cheng, Q. Liu, W.-R. Xu and Y.-C. Zhang, Antioxidant and antibacterial properties of essential oils-loaded β-cyclodextrin-epichlorohydrin oligomer and chitosan composite films, Colloids Surf., B, 2022, 215, 112504 CrossRef CAS PubMed.
- S. Tie, W. Su, X. Zhang, Y. Chen, X. Zhao and M. Tan, pH-responsive core-shell microparticles prepared by a microfluidic chip for the encapsulation and controlled release of procyanidins, J. Agric. Food Chem., 2021, 69, 1466–1477 CrossRef CAS PubMed.
- Y. Qin, S. Zhang, J. Yu, J. Yang, L. Xiong and Q. Sun, Effects of chitin nano-whiskers on the antibacterial and physicochemical properties of maize starch films, Carbohydr. Polym., 2016, 147, 372–378 CrossRef CAS PubMed.
- S. Tan, X. Tan, Z. Chi, D. Zhang and W. Li, In vitro assessment of the toxicity of lead (Pb2+) to phycocyanin, Chemosphere, 2018, 192, 171–177 CrossRef CAS PubMed.
- S. Tavakoli, E. Mubango, L. Tian, Y. B. ŃDri, Y. Tan, H. Hong and Y. Luo, Novel intelligent films containing anthocyanin and phycocyanin for nondestructively tracing fish spoilage, Food Chem., 2023, 402, 134203 CrossRef CAS.
- J. Hu, D. Li, Q. Huai, M. Geng, Z. Sun, M. Wang, S. Wang, Y. Li and H. Zheng, Development and evaluation of soybean protein isolate-based antibacterial nanocomposite films containing nano-TiO2, Ind. Crops Prod., 2023, 197, 116620 CrossRef CAS.
- R. Zhao, J. Chen, S. Yu, R. Niu, Z. Yang, H. Wang, H. Cheng, X. Ye, D. Liu and W. Wang, Active chitosan/gum Arabic-based emulsion films reinforced with thyme oil encapsulating blood orange anthocyanins: Improving multi-functionality, Food Hydrocolloids, 2023, 134, 108094 CrossRef CAS.
- X. Nie, Y. Gong, N. Wang and X. Meng, Preparation and characterization of edible myofibrillar protein-based film incorporated with grape seed procyanidins and green tea polyphenol, LWT--Food Sci. Technol., 2015, 64, 1042–1046 CrossRef CAS.
- J. Sun, Z. Wei and C. Xue, Preparation and characterization of multifunctional films based on pectin and carboxymethyl chitosan: Forming microchambers for high-moisture fruit preservation, Food Packag. Shelf Life, 2023, 37, 101073 CrossRef CAS.
- G. Zhang, H. Zhao, T. Guan and Z. Ma, Complexation of phycocyanin with hydroxypropyl-β-cyclodextrin and its application in blue beer containing quinoa saponins as foaming agents, Front. Nutr., 2023, 10, 1209193 CrossRef PubMed.
- M. Sari, J. Kaban and Z. Alfian, A novel composite
membrane pectin from Cyclea Barbata Miers blend with chitosan for accelerated wound healing, Polym. Test., 2021, 99, 107207 CrossRef CAS.
- X. Zong, X. Zhang, K. Bi, Y. Zhou, M. Zhang, J. Qi, X. Xu, L. Mei, G. Xiong and M. Fu, Novel emulsion film based on gelatin/polydextrose/camellia oil incorporated with Lactobacillus pentosus: Physical, structural, and antibacterial properties, Food Hydrocolloids, 2021, 121, 107063 CrossRef CAS.
- S. Tie, S. Xiang, Y. Chen, F. Qiao, W. Cui, W. Su and M. Tan, Facile synthesis of food-grade and size-controlled nanocarriers based on self-assembly of procyanidins and phycocyanin, Food Funct., 2022, 13, 4023–4031 RSC.
- J. Wang, W. Zhang, C. Tang, J. Xiao, B. Xie and Z. Sun, Synergistic effect of B-type oligomeric procyanidins from lotus seedpod in combination with water-soluble Poria cocos polysaccharides against E. coli and mechanism, J. Funct. Foods, 2018, 48, 134–143 CrossRef CAS.
- S. Tie, X. Zhang, H. Wang, Y. Song and M. Tan, Procyanidins-loaded complex coacervates for improved stability by self-crosslinking and calcium ions chelation, J. Agric. Food Chem., 2020, 68, 3163–3170 CrossRef CAS.
- E. Jamróz, A. Cabaj, J. Tkaczewska, A. Kawecka, P. Krzyściak, M. Szuwarzyński, T. Mazur and L. Juszczak, Incorporation of curcumin extract with lemongrass essential oil into the middle layer of triple-layered films based on furcellaran/chitosan/gelatin hydrolysates – In vitro and in vivo studies on active and intelligent properties, Food Chem., 2023, 402, 134476 CrossRef.
- A. Zhang, Y. Han and Z. Zhou, Characterization of citric acid crosslinked chitosan/gelatin composite film with enterocin CHQS and red cabbage pigment, Food Hydrocolloids, 2023, 135, 108144 CrossRef CAS.
Footnote |
† Electronic supplementary information (ESI) available: EDS element, water solubility, color parameter and optical images of radical scavenging ability. See DOI: https://doi.org/10.1039/d3ra08653d |
|
This journal is © The Royal Society of Chemistry 2024 |