DOI:
10.1039/D4RA00677A
(Review Article)
RSC Adv., 2024,
14, 6462-6469
Perspectives on emerging dual carbon fiber batteries: advantages, challenges and prospects
Received
26th January 2024
, Accepted 27th January 2024
First published on 21st February 2024
Abstract
This perspective article describes a new dual carbon fiber battery, where both the cathode and anode are made of carbon fiber. The dual carbon fiber battery combines the advantages of carbon fiber and dual graphite batteries, including a higher working potential compared to lithium-ion batteries, a high areal capacity, and easy access due to the mature manufacturing technology of carbon fibers. In this article, we discuss the mechanism, current status and potential application areas of dual carbon fiber batteries. Additionally, we highlight the challenges and prospects of these batteries.
1. Introduction
With a carbon content of over 90%, carbon fiber possesses a unique combination of advantages, including high strength, high stiffness, and light weight.1 Since the commercialization of high-performance carbon fibers enabled by Union Carbide in the 1960s,2 carbon fiber has become increasingly attractive for high-volume applications, ranging from sporting goods to aircraft structures. Today, this versatile material is being developed for a new application: energy storage.
Regarding the application of carbon fiber in energy storage devices, it has primarily been investigated for its functions as current collectors and anodes. This is due to its high electrical conductivity and three-dimensional (3D) structure.3,4 Carbon fiber current collectors are lighter compared to metal foil current collectors made of aluminum and copper. Additionally, they provide a 3D-interconnected electrically conductive network to the active materials loaded on the carbon fiber, thereby enhancing the electrochemical properties of energy storage devices. For example, Zuo et al.3 developed a three-dimensional multifunctional collector using graphitized carbon fiber to enhance the performance of lithium metal anodes. This collector increased the anode's areal capacity while mitigating the significant volume changes that occur during Li+ insertion/extraction and metallic Li plating/ripping of lithium metal electrodes. As a result, the cycling performance of the battery was improved. Numerous other publications have also demonstrated promising applications of carbon fiber current collectors in energy storage devices.4–8 In addition, carbon fiber, with its high graphite content and the ability to facilitate lithium-ion insertion/extraction into/from graphite, can serve not only as anodes for lithium-ion batteries,9–11 but may provide a high capacity due to its high graphite mass loading.11
Recently, the dual ion battery (DIB) has gained significant attention as a novel battery system due to its advantages of high working voltage, environmental friendliness, and low cost. The concept of the “dual-carbon” or “dual-graphite” battery was introduced by pioneering studies conducted by McCullough et al.12 and Carlin et al.13 Anion intercalation in graphite particles has been demonstrated with ClO4−,14 BF4−,15 PF6−,16 and (CF3SO2)2N− (ref. 17) as well as a number of other ions at high voltages (4.5–5.2 V vs. Li).18 This intercalation process yields a capacity of approximately 90 mA h g−1, which is equivalent to one anion per 24 carbons. When anion-intercalated graphite is combined with a lithiated graphite anode, a complete battery system is formed. During discharge/charge cycles, the cation (such as Li+) in the electrolyte of dual graphite batteries is inserted/extracted at the anode, while the anions are inserted/extracted at the cathode.
With graphite being an important composition of carbon fiber, a carbon fiber electrode can leverage the benefits of both carbon fiber and graphite electrodes. It is not only freestanding but capable of cation/anion intercalation/deintercalation. When utilized as both a cathode and an anode, carbon fiber electrodes form a dual carbon fiber battery. This perspective article aims to showcase the current status of a dual carbon fiber battery and highlight its potentials. The perspective also delves into the mechanism and promising application areas of a dual carbon fiber battery, while also addressing the challenges it faces. Lastly, the prospects for the dual carbon fiber battery are evaluated.
2. Current status of dual carbon fiber batteries
2.1. The discharge/charge mechanisms of dual carbon fiber batteries
The discharge/charge mechanisms of dual carbon fiber batteries will be explained in comparison to those of lithium-ion (Li-ion) batteries and dual graphite batteries (Fig. 1). In Li-ion batteries, the movement of Li+ ions between the cathode and anode enables the charging and discharging processes. In dual graphite batteries, Li+ ions and PF6− anions insert into the graphene layers of a graphite anode and cathode to form the LiCx compound (eqn (1)) and the C24(PF6) complex (eqn (2)) during the discharge process; the reactions are reversed and initiated from the LiCx compound and the C24(PF6) complex at the charge process.
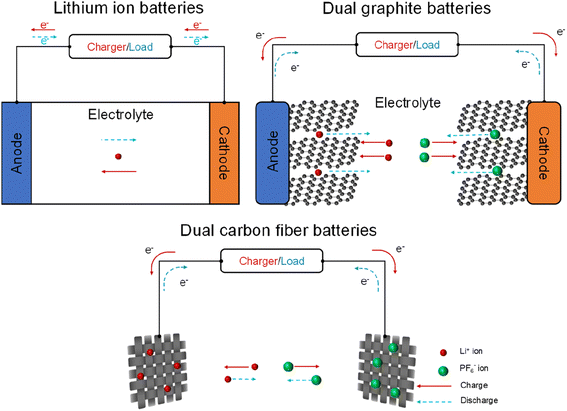 |
| Fig. 1 Schematic of charge/discharge mechanisms of dual carbon fiber batteries in contrast to lithium-ion batteries and dual graphite batteries. | |
When graphite particles are replaced by carbon fiber in dual carbon fiber batteries, PF6− anions and Li+ cations insert into/detach from the carbon fiber cathode and anode, respectively. Although there has been limited investigation into the discharge/charge mechanisms of dual carbon fiber batteries, it can be inferred that Li+ ions and PF6− anions are intercalated into the graphene layers of carbon fiber based on the ex situ XRD spectra of the carbon fiber cathode and anode extracted from a discharged dual carbon fiber battery.19 This is demonstrated by the deviations of the (002) peak (∼26.4°) for the lithiated carbon fiber anode (∼26.2°) and the PF6−-intercalated carbon fiber cathode (∼24.5°). Further research is necessary to gain a deeper understanding of the discharge/charge mechanisms of dual carbon fiber batteries.
2.2. The practicality of dual carbon fiber batteries
Thanks to the mature manufacturing technology of carbon fiber, obtaining the positive and negative electrodes of dual carbon fiber batteries is relatively straightforward. By simply cutting commercialized carbon cloth into the appropriate size, the dimension of carbon fiber (cloth) electrodes can be easily adjusted, which contributes to the feasibility and accessibility of dual carbon fiber batteries. Furthermore, weaving and knitting technology offers a convenient method for fabricating 3-dimensional (3D) carbon fiber cloths, which can enhance the electrochemical properties of carbon fiber electrodes due to their inherently interconnected electrical network.
Interestingly, while most carbon fibers purchased from different companies can serve as the anode of dual carbon fiber batteries, only one type of pitch-based carbon fiber out of five kinds is capable of PF6− intercalation/deintercalation. The dependence of PF6− intercalation/deintercalation on carbon fiber categories presents an intriguing area for investigating the underlying mechanisms. However, due to the limited number of experiments, it is not appropriate to assert the unique applicability of pitch-based carbon fiber for dual carbon fiber batteries. Future experiments are needed to explore the possibility of intercalation/deintercalation of other anions (such as ClO4−, BF4− and (CF3SO2)2N−) into other types of carbon fibers. These efforts will significantly contribute to understanding the discharge/charge mechanisms of dual carbon fiber batteries.
2.3. The performance of dual carbon fiber batterie
Dual carbon fiber batteries offer several advantages. Firstly, they exhibit a significantly higher working voltage (4.4 V) compared to traditional lithium-ion batteries (3.5 V), as demonstrated in a previous publication.19 Secondly, due to the high mass loading of approximately 30 mg cm−2, one 2032-coin cell based on dual carbon fiber batteries achieve a much higher areal capacity (∼2 mA h cm−2) compared to traditional dual graphite batteries.20–23 By combining the voltage and capacity, the energy density of dual carbon fiber batteries can be calculated to be 163 W h kg−1 at 0.1C.
3. Potential applications of dual carbon fiber batteries
The insertion/detachment of anions/cations into/from both the carbon fiber cathode and anode allows for the integration of dual carbon fiber electrodes into the fabrication of energy storage devices. Leveraging the exceptional performance of carbon fiber, including its high mechanical properties and low weight, opens up possibilities for designing batteries with specialized functionalities. Examples of such batteries include fibrous batteries and structural batteries. Exploring these avenues will be an important research direction for dual carbon fiber batteries.
3.1. Fiber-shaped batteries
The rapid growth of wearable electronics has created a strong demand for high-performance flexible energy storage devices to efficiently power these devices and enable wireless communications.24 As a result, significant efforts have been focused on developing fiber-shaped energy storage devices that can be seamlessly integrated into fabrics to create multifunctional wearable energy storage systems.25 Fiber-shaped energy storage devices have garnered increasing research interest worldwide have conducted extensive research on wearable electronics using carbon nanotube (CNT) yarns.26–29 Their approach involves incorporating active materials into fibers and then weaving or knitting these functional fibers into fabrics. For instance, Ren et al.,30 who are part of Peng's group, developed a fiber-shaped lithium-ion battery by encapsulating Li4Ti5O12 and LiMn2O4 nanoparticles as the anode and cathode, respectively, within multi-walled carbon nanotube (MWCNT) yarns.
Despite the as demonstrated advantages of this fiber shaped battery, there are still some drawbacks associated with this method. One major issue arises from the physical interactions between the incorporated active material and CNT, which can lead to a deterioration in electrochemical performance over discharge/charge cycles.
Although the use of carbon fiber31–33 as a replacement for CNT due to its lithiation capability can mitigate the weak interaction issue at the anode side due to its lithiation capability, both CNT and previously reported carbon fiber lack the ability for anion intercalation. Therefore, the incorporation of active cathode materials onto flexible carbon fiber/CNT becomes necessary.
To address this challenge, one solution is to explore the concept of a fiber cathode. This is where dual carbon fiber batteries come into play. With carbon fiber capable of both lithiation and anion intercalation at both the anode and cathode sides, a full battery capable of discharge/charge can be achieved. This approach simplifies the manufacturing processes of parallel, twisting, and coaxial fiber-shaped energy storage devices (Fig. 2).
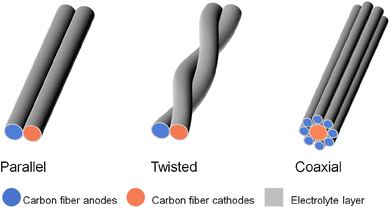 |
| Fig. 2 Configurations of fiber based dual carbon fiber batteries. | |
3.2. Structural batteries
The structured battery is a revolutionary type of rechargeable battery that possesses the unique capability to bear mechanical loads while simultaneously storing electrochemical energy.34–37 This design concept offers the advantages of reducing overall mass and increasing volume efficiency. These characteristics make structural batteries an excellent solution to address the challenges of excessive weight and poor safety typically associated with traditional batteries under mechanical loads. Furthermore, structural batteries hold tremendous potential for applications in electric vehicles, electric airplanes, and other fields.38–40
For the configurations of structural batteries, a comprehensive description can be found in previous reviews.41 In terms of the electrodes, carbon fiber has been extensively employed as the anode material in structural batteries. By combining carbon fiber anodes with 2-dimensional (2D) aluminum current collectors-based cathodes, full structural batteries have been successfully fabricated42 (Fig. 3a). Carbon fiber is commonly used as the current collectors, and there are ongoing explorations to develop modified carbon fiber cathodes with active cathode materials. This research aims to eliminate the need for metal current collectors in structural batteries, thereby achieving further weight reduction, as demonstrated in Fig. 3b.
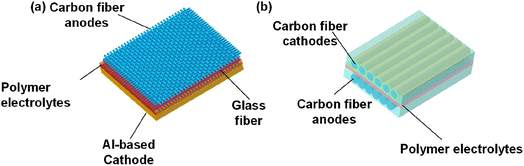 |
| Fig. 3 Configuration of (a) structural batteries with a carbon fiber anode and an Al-based cathode; (b) dual carbon fiber structural batteries. | |
The modified carbon fiber serves multiple functions in dual carbon fiber structural batteries. It not only acts as the cathode and anode but also reinforces the polymer electrolyte matrix. The polymer serves as both the medium for Li+ transportation between the cathode and anode and as the matrix for the composite laminates. While the schematic clearly illustrates the composition of dual carbon fiber structural batteries, one crucial aspect that cannot be explicitly seen is the interface between the polymer electrolyte matrix and the modified carbon fiber. The interfacial Li+ diffusion is greatly influenced by the distribution of active materials on the carbon fiber. The presence of agglomerated LiFePO4 particles may hinder the rapid diffusion of Li+ at the LiFePO4/electrolyte interface. It is possible that the sluggish Li+ diffusion at the LiFePO4/electrolyte interface43 has prevented the demonstration of full batteries based on LiFePO4-modified carbon fiber cathodes and carbon fiber anodes thus far.
Thanks to the anion intercalation capability of carbon fiber at the cathode side, structural batteries based on carbon fiber cathodes and anodes (Fig. 4) can overcome the challenge of active material growth on carbon fiber. This not only simplifies the manufacturing process of structural batteries but also enables the development of all carbon fiber structural batteries.
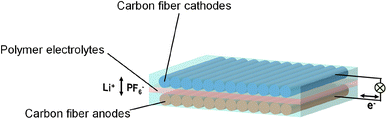 |
| Fig. 4 Schematic of dual carbon fiber structural batteries. | |
4. Challenges
4.1 Low cycling stability
Despite the remarkable advantages of dual carbon fiber batteries, their cycling stability still falls short of practical requirements. As demonstrated in our previous publication,19 the capacity of dual carbon fiber batteries begins to decay from the 20th cycle, experiencing significant capacity loss from cycles 20 to 50, and dropping to 62% of the reversible capacity of the first cycle. By the 80th cycle, the capacity further decreases to 42% of the initial capacity. The observed capacity decay may be attributed to factors such as thickening of the solid-electrolyte interphase (SEI) layer, carbon fiber cracking, low lithiation/delithiation reversibility, among others (Fig. 5). In the future, several strategies will be explored to enhance the stability of dual carbon fiber batteries. These include the development of high voltage electrolyte systems,44 the design of new additives,41 and the exploration of solid-state/quasi-solid-state electrolytes solid state/quasi-solid-state electrolytes.45 These strategies aim to address the challenges associated with cycling stability and improve the overall performance of dual carbon fiber batteries.
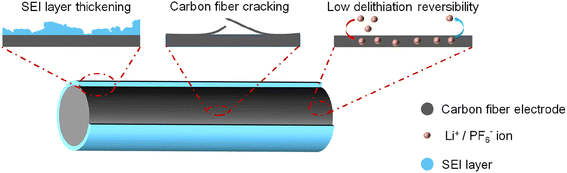 |
| Fig. 5 Low cycling stability of dual carbon fiber batteries. | |
4.2. Unclear charge/discharge mechanisms
In our previous publication,19 we investigated the electrochemical performance of five different types of carbon fibers: mesophase pitch-based carbon fiber (PCF), polyacrylonitrile-based carbon fibers including T300 carbon fiber from Toray Industries Co., Ltd. (TCF), T300 carbon fiber from Jiangsu Hengshen Co., Ltd. (HCF), T300 carbon fiber from TEI composite (SCF), and carbon fiber without a microporous layer and PTFE from CeTech Co., Ltd. (CCF). The test results revealed that all five types of carbon fibers can serve as the anode in dual carbon fiber batteries. However, only the mesophase pitch-based carbon fiber demonstrated the capability to serve as the cathode in dual carbon fiber batteries. The charge/discharge mechanism of carbon fiber electrodes, particularly the carbon fiber cathode, requires further investigation. Given the significant differences in microstructure and crystallization degree between mesophase pitch carbon fiber and the other four types, it is important to elucidate the effects of microstructure and crystallization degree on the lithiation and anion intercalation capabilities (Fig. 6).
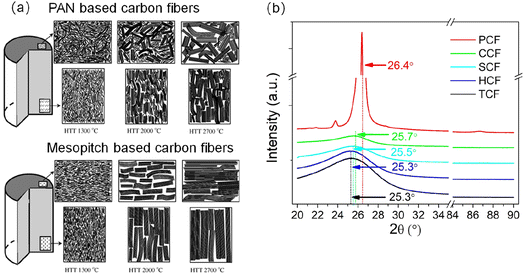 |
| Fig. 6 Underlying mechanisms behind the function of mesopitch carbon fiber as cathode of dual carbon fiber batteries from the microstructural aspect (a) and the graphitization degree aspect (b). | |
4.3 Tailored mechanical properties for fiber-based batteries and structural batteries
While the previous challenges are primarily related to the electrochemical properties of dual carbon fiber batteries, this point pertains more to the mechanical properties of these batteries. When it comes to using fiber-based batteries for wearable electronics, flexibility is a crucial requirement for their components. However, mesophase pitch carbon fiber exhibits significantly lower flexibility compared to polyacrylonitrile-based carbon fiber, which could limit its suitability for fiber-based batteries. Therefore, it is important to optimize the flexibility of mesophase pitch carbon fiber while maintaining satisfactory electrochemical properties, particularly for dual carbon fiber batteries used in wearable electronics. For structural batteries, the low flexibility of carbon fiber is not a major concern. However, the stability of the interface becomes crucial to achieve stable cycling performance. As demonstrated in our previous publication,19 after 50 discharge/charge cycles, the carbon fiber cathode can experience splitting due to significant volume expansion during anion intercalation. When carbon fiber is used in combination with a solid electrolyte for the fabrication of structural batteries, this large volume expansion may lead to the detachment of the solid electrolyte from the carbon fiber electrode. This can negatively impact the interfacial Li+ diffusion and overall electrochemical properties (Fig. 7). Therefore, achieving high interfacial and structural integrity is essential for the stability and performance of structural batteries.
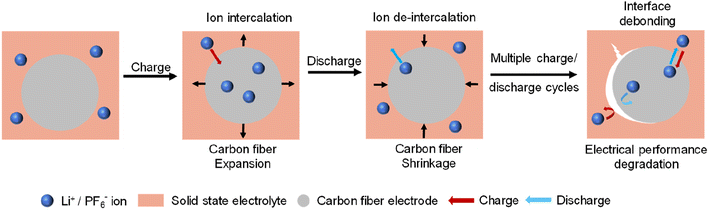 |
| Fig. 7 Possible interface debonding of carbon fiber electrode and solid state electrolyte. | |
5. Outlooks and perspectives
In this perspective, we propose a novel battery that combines the advantages of carbon fiber and dual graphite batteries. We provide some insights into the mechanisms involved in dual carbon fiber batteries, comparing them to lithium-ion batteries and dual graphite batteries (Table 1). Furthermore, we explore the potential applications of these batteries in fibrous batteries and structural batteries. It is anticipated that these advancements will bring significant breakthroughs to the existing batteries in these two domains.
Table 1 Performance comparison of dual carbon fiber batteries and traditional Li-ion batteries
Categories |
Working potential (V) |
Areal capacity (mA h cm−2) |
Energy density (W h kg−1) |
Cost ($ per kW per h) |
The cost is calculated based on the cost of carbon fiber at 11$ per kg. |
Dual carbon fiber batteries |
4.4 |
∼2 |
163 (ref. 19) |
160a |
Traditional Li-ion batteries |
3.5 |
∼1 |
150 (ref. 46) |
153 (ref. 47) |
Despite the inherent advantages of dual carbon fiber batteries, there are still notable challenges that need to be addressed. These challenges include issues such as low cycling stability, an unclear understanding of the discharge/charge mechanism, and a limited range of carbon fiber cathode options, among others. To overcome these challenges, further studies and research are necessary to gain deeper insights into the fundamental aspects of dual carbon fiber batteries. This will help in developing strategies and techniques to enhance their performance and overcome the current limitations.
(1) Even though low cycling stability has been observed in dual carbon fiber batteries, further studies are needed to understand the underlying reasons behind this issue. Potential factors that could contribute to the low cycling stability include a lower utilization rate of active materials due to structural dis-integrity (such as splitting) and slow Li+ diffusion caused by the thick solid-electrolyte interphase (SEI) layer resulting from the decomposition of the carbonate electrolyte, among others. While anion intercalation/deintercalation is a primary mechanism for the discharge/charge process at the cathode side, achieving anion adsorption on carbon fibers may potentially alleviate the low cycling stability. Advanced characterization techniques can be employed in these studies to analyze and investigate these factors in more detail. By gaining a deeper understanding of the root causes, researchers can develop strategies to improve the cycling stability of dual carbon fiber batteries.
(2) Considering that only mesophase pitch carbon fiber allows for anion intercalation/de-intercalation among the five types of carbon fiber, it is evident that certain important parameters influence the electrochemical properties of carbon fiber cathodes. Given the significant microstructural differences between mesophase pitch carbon fiber and other types, it becomes crucial to understand the relationship between microstructure and electrochemical properties of carbon fiber cathodes. This understanding will play a pivotal role in further optimizing the performance of carbon fiber cathodes. By investigating and analyzing the microstructure-electrochemical properties relationship, researchers can identify key parameters and design strategies to enhance the electrochemical performance of carbon fiber cathodes.
(3) With regard to the importance of mechanical properties for both fibers based batteries and structural batteries, it becomes crucial to find a balance between obtaining carbon fiber with satisfactory flexibility or strength while ensuring optimal electrochemical properties. It is important to establish a comprehensive understanding of the microstructure-electrochemical/mechanical properties relationship for both carbon fiber cathodes and anodes. By studying this relationship, researchers can identify the key factors that influence the mechanical properties of carbon fiber without compromising their electrochemical performance. This knowledge will enable the development of carbon fiber materials that possess both desirable mechanical properties and excellent electrochemical performance, thereby advancing the field of fiber-based batteries and structural batteries.
(4) During the charge/discharge processes, both the carbon fiber cathode and anode undergo dynamic volume expansion and contraction. Understanding the performance and microstructure evolution throughout the entire cycle life is crucial for achieving cycling stability in dual carbon fiber batteries. This is particularly important when solid electrolyte is used in the fabrication of these batteries. The evolution of the electrolyte/electrode interface, which is influenced by the volume expansion/contraction of the electrodes, plays a critical role in determining the electrochemical properties of solid-state dual carbon fiber batteries. Therefore, investigating and comprehending these dynamics are essential for optimizing the cycling stability and overall performance of dual carbon fiber batteries, especially those utilizing solid-state electrolytes.
Conflicts of interest
There are no conflicts to declare.
References
- E. Frank, F. Hermanutz and M. R. Buchmeiser, Carbon Fibers: Precursors, Manufacturing, and Properties, Macromol. Mater. Eng., 2012, 297(6), 493–501 CrossRef CAS
. - D. D. Edie, The Effect of Processing on The Structure and Properties of Carbon Fibers, Carbon, 1998, 36(4), 345–362 CrossRef CAS
. - T. T. Zuo, X. W. Wu, C. P. Yang, Y. X. Yin, H. Ye, N. W. Li and Y. G. Guo, Graphitized Carbon Fibers as Multifunctional 3D Current Collectors for High Areal Capacity Li Anodes, Adv. Mater., 2017, 29(29), 6 CrossRef PubMed
. - F. Cheng, X. P. Yang, O. Ka, L. Wen, X. Q. Wang and W. Lu, A 3D Multifunctional Host Anode from Commercial Carbon Cloth for Lithium Metal Batteries, J. Mater. Chem. A, 2023, 11(8), 4205–4219 RSC
. - S. H. Chen, J. F. Wu, R. H. Zhou, Y. Q. Chen, Y. H. Song and L. Wang, Controllable Growth of NiCo2O4 Nanoarrays on Carbon Fiber Cloth and Its Anodic Performance for Lithium-ion Batteries, RSC Adv., 2015, 5(126), 104433–104440 RSC
. - A. B. Ikhe, W. B. Park, S. C. Han, J. Y. Seo, S. Han, K. S. Sohn and M. Pyo, Li+-Intercalated Carbon Cloth for Anode-free Li-ion Batteries with Unprecedented Cyclability, J. Mater. Chem. A, 2022, 10(40), 21456–21464 RSC
. - H. R. Lu, J. Hagberg, G. Lindbergh and A. Cornell, Li4Ti5O12 Flexible, Lightweight Electrodes Based on Cellulose Nanofibrils as Binder and Carbon Fibers as Current Collectors for Li-ion Batteries, Nano Energy, 2017, 39, 140–150 CrossRef CAS
. - T. Q. Liu, W. Q. Wang, M. J. Yi, Q. D. Chen, C. Xu, D. P. Cai and H. B. Zhan, Metal-organic Framework Derived Porous Ternary ZnCo2O4 Nanoplate Arrays Grown on Carbon Cloth as Binder-free Electrodes for Lithium-ion Batteries, Chem. Eng. J., 2018, 354, 454–462 CrossRef CAS
. - J. K. Lee, K. W. An, J. B. Ju, B. W. Cho, W. I. Cho, D. Park and K. S. Yun, Electrochemical Properties of PAN-based Carbon Fibers as Anodes for Rechargeable Lithium-ion Batteries, Carbon, 2001, 39(9), 1299–1305 CrossRef CAS
. - M. H. Kjell, E. Jacques, D. Zenkert, M. Behm and G. Lindbergh, PAN-Based Carbon Fiber Negative Electrodes for Structural Lithium-Ion Batteries, J. Electrochem. Soc., 2011, 158(12), A1455–A1460 CrossRef CAS
. - Y. Fu and Q. Gan, Experimental and Analytical Investigation of the Potential of Carbon Fibres for Use in Multifunctional Batteries, J. Solid State Electrochem., 2023, 27(2), 345–355 CrossRef CAS
. - F. P. McCullough, R. V. Snelgrove and C. A. Levine, Secondary Battery, Dow Chemical Co., USA, 1986, p. 31 Search PubMed
. - R. T. Carlin, H. C. De Long, J. Fuller and P. C. Trulove, Dual Intercalating Molten Electrolyte Batteries, J. Electrochem. Soc., 1994, 141(7), L73 CrossRef CAS
. - V. Suryanarayanan and M. Noel, Effect of Solvents and Solvent Mixtures on Intercalation/De-intercalation Behaviour of Li+ and ClO4- Ions in Polypropylene-graphite Composite Electrodes, J. Power Sources, 2001, 94(1), 137–141 CrossRef CAS
. - Y. J. Wang, J. Y. Li, Y. H. Huang and H. Y. Wang, Anion Storage Behavior of Graphite Electrodes in LiBF4/Sulfone/Ethyl Methyl Carbonate Solutions, Langmuir, 2019, 35(46), 14804–14811 CrossRef CAS PubMed
. - J. A. Seel and J. R. Dahn, Electrochemical Intercalation of PF6- into Graphite, J. Electrochem. Soc., 2000, 147(3), 892–898 CrossRef CAS
. - G. Schmuelling, T. Placke, R. Kloepsch, O. Fromm, H. W. Meyer, S. Passerini and M. Winter, X-ray Diffraction Studies of the Electrochemical Intercalation of Bis(trifluoromethanesulfonyl)imide Anions into Graphite for Dual-ion Cells, J. Power Sources, 2013, 239, 563–571 CrossRef CAS
. - T. E. Sutto, T. T. Duncan and T. C. Wong, X-ray Diffraction Studies of Electrochemical Graphite Intercalation Compounds of Ionic Liquids, Electrochim. Acta, 2009, 54(24), 5648–5655 CrossRef CAS
. - Q. Gan and Y. Fu, Emerging Dual Carbon Fiber Batteries, Electrochim. Acta, 2023, 439, 8 CrossRef
. - J. Inamoto, K. Sekito, N. Kobayashi and Y. Matsuo, Graphene-like Graphite as a Novel Cathode Material with a Large Capacity and Moderate Operating Potential for Dual Carbon Batteries, J. Electrochem. Soc., 2021, 168(1), 010528 CrossRef CAS
. - P. Münster, A. Heckmann, R. Nölle, M. Winter, K. Beltrop and T. Placke, Enabling High Performance Potassium-Based Dual-Graphite Battery Cells by Highly Concentrated Electrolytes, Batter. Supercaps, 2019, 2(12), 992–1006 CrossRef
. - A. Wang, W. Yuan, J. Fan and L. Li, A Dual-graphite Battery with Pure 1-Butyl-1-methylpyrrolidinium bis(trifluoromethylsulfonyl) Imide as the Electrolyte, Energy Technol., 2018, 6(11), 2172–2178 CrossRef CAS
. - L. Fan, Q. Liu, S. Chen, K. Lin, Z. Xu and B. Lu, Potassium-Based Dual Ion Battery with Dual-Graphite Electrode, Small, 2017, 13(30), 1701011 CrossRef PubMed
. - X. Y. Hu, Y. Y. Dou, J. J. Li and Z. F. Liu, Buckled Structures: Fabrication and Applications in Wearable Electronics, Small, 2019, 15(32), 26 Search PubMed
. - X. He, Y. Zi, H. Guo, H. Zheng, Y. Xi, C. Wu, J. Wang, W. Zhang, C. Lu and Z. L. Wang, A Highly Stretchable Fiber-Based Triboelectric Nanogenerator for Self-Powered Wearable Electronics, Adv. Funct. Mater., 2017, 27(4), 1604378 CrossRef
. - G. Huang, Y. Zhang, L. Wang, P. Sheng and H. Peng, Fiber-based MnO2/Carbon Nanotube/Polyimide Asymmetric Supercapacitor, Carbon, 2017, 125, 595–604 CrossRef CAS
. - H. Peng, Aligned Carbon Nanotube/Polymer Composite Films with Robust Flexibility, High Transparency, and Excellent Conductivity, J. Am. Chem. Soc., 2008, 130(1), 42–43 CrossRef CAS PubMed
. - Z. Pan, J. Ren, G. Guan, X. Fang, B. Wang, S.-G. Doo, I. H. Son, X. Huang and H. Peng, Synthesizing Nitrogen-Doped Core–Sheath Carbon Nanotube Films for Flexible Lithium Ion Batteries, Adv. Energy Mater., 2016, 6(11), 1600271 CrossRef
. - P. Chen and H. Peng, High-Performance Graphene Fibers Enabled by Hydration, ACS Cent. Sci., 2020, 6(7), 1040–1042 CrossRef CAS PubMed
. - J. Ren, Y. Zhang, W. Y. Bai, X. L. Chen, Z. T. Zhang, X. Fang, W. Weng, Y. G. Wang and H. S. Peng, Elastic and Wearable Wire-Shaped Lithium-Ion Battery with High Electrochemical Performance, Angew. Chem., Int. Ed., 2014, 53(30), 7864–7869 CrossRef CAS
. - X. Zhu, Q. Li, S. Qiu, X. Liu, L. Xiao, X. Ai, H. Yang and Y. Cao, Hard Carbon Fibers Pyrolyzed from Wool as High-Performance Anode for Sodium-Ion Batteries, J. Mater., 2016, 68(10), 2579–2584 CAS
. - X. Gao, Y. An, W. Zhang, M. Yu, L. Ci and J. Feng, Self-supporting Soft Carbon Fibers as Binder-free and Flexible Anodes for High-performance Sodium-ion Batteries, Mater. Technol., 2018, 33(12), 810–814 CrossRef CAS
. - J. Sun, L. Ma, H. Sun, Y. Xu, J. Li, W. Mai and B. Liu, Design of Free-standing Porous Carbon Fibers Anode with High-efficiency Potassium-ion Storage, Chem. Eng. J., 2023, 455, 140902 CrossRef CAS
. - Y. Yu, B. Zhang, M. Feng, G. Qi, F. Tian, Q. Feng, J. Yang and S. Wang, Multifunctional Structural Lithium-ion Batteries Based on Carbon Fiber Reinforced Plastic Composites, Compos. Sci. Technol., 2017, 147, 62–70 CrossRef CAS
. - N. Ihrner, W. Johannisson, F. Sieland, D. Zenkert and M. Johansson, Structural Lithium-ion Battery Electrolytes via Reaction Induced Phase-separation, J. Mater. Chem. A, 2017, 5(48), 25652–25659 RSC
. - P. Ladpli, R. Nardari, F. Kopsaftopoulos and F.-K. Chang, Multifunctional Energy Storage Composite Structures with Embedded Lithium-ion Batteries, J. Power Sources, 2019, 414, 517–529 CrossRef CAS
. - J. Pyo, H.-W. Park, M.-S. Jang, J.-S. Choi, S. K. Sathish Kumar and C.-G. Kim, Tubular Laminated Composite Structural Battery, Compos. Sci. Technol., 2021, 208, 108646 CrossRef CAS
. - L. E. Asp, M. Johansson, G. Lindbergh, J. Xu and D. Zenkert, Structural Battery Composites: a Review, Funct. Compos. Struct., 2019, 1(4), 042001 CrossRef CAS
. - F. Danzi, R. M. Salgado, J. E. Oliveira, A. Arteiro, P. P. Camanho and M. H. Braga, Structural Batteries, 2021, 26(8), 2203 CAS
. - J. Galos, K. Pattarakunnan, A. S. Best, I. L. Kyratzis, C. H. Wang and A. P. Mouritz, Energy Storage Structural Composites with Integrated Lithium-Ion Batteries: A Review, Adv. Mater. Technol., 2021, 6(8) CAS
. - H. Zhou, H. Li, L. Li, T. Liu, G. Chen, Y. Zhu, L. Zhou and H. Huang, Structural Composite Energy Storage Devices — a Review, Mater. Today Energy, 2022, 24, 100924 CrossRef CAS
. - Y. Zhao, D. Zhao, T. Zhang, H. Li, B. Zhang and Z. Zhenchong, Preparation and Multifunctional Performance of Carbon Fiber-reinforced Plastic Composites for Laminated Structural Batteries, Polym. Compos., 2020, 41(8), 3023–3033 CrossRef CAS
. - J. Hagberg, H. A. Maples, K. S. P. Alvim, J. Xu, W. Johannisson, A. Bismarck, D. Zenkert and G. Lindbergh, Lithium Iron Phosphate Coated Carbon Fiber Electrodes for Structural Lithium-ion Batteries, Compos. Sci. Technol., 2018, 162, 235–243 CrossRef CAS
. - R. Petibon, J. Xia, L. Ma, M. K. G. Bauer, K. J. Nelson and J. R. Dahn, Electrolyte System for High Voltage Li-Ion Cells, J. Electrochem. Soc., 2016, 163(13), A2571 CrossRef CAS
. - M. Y. Tan, D. Safanama, S. S. Goh, J. Y. C. Lim, C.-H. Lee, J. C. C. Yeo, W. Thitsartarn, M. Srinivasan and D. W. H. Fam, Concepts and Emerging Trends for Structural Battery Electrolytes, Chem.–Asian J., 2022, 17(21), e202200784 CrossRef CAS PubMed
. - S. Bruno, J. Hassoun and Y.-K. Sun, Lithium-ion Batteries. A Look into The Future, Energy Environ. Sci., 2011, 4, 3287 RSC
. - S. Orangi, N. Bunyui Manjong, D. Perez Clos, L. Usai, O. Stokke Burheim and A. Hammer Strømman, Trajectories for Lithium-Ion Battery Cost Production: Can Metal Prices Hamper the Deployment of Lithium-Ion Batteries?, Batter. Supercaps, 2023, 6, e202330034 Search PubMed
.
|
This journal is © The Royal Society of Chemistry 2024 |
Click here to see how this site uses Cookies. View our privacy policy here.