DOI:
10.1039/D4RA00990H
(Paper)
RSC Adv., 2024,
14, 8341-8352
Green design and synthesis of some novel thiazolidinone appended benzothiazole–triazole hybrids as antimicrobial agents†
Received
7th February 2024
, Accepted 7th March 2024
First published on 12th March 2024
Abstract
The global increase in bacterial resistance poses a significant threat, jeopardizing the effectiveness of antibiotics. Therefore, the development of new and efficient antimicrobial agents is pre-dominant. Taking this into consideration, in the present study, we designed and reported the facile synthesis of two novel series benzothiazole–triazole and thiazolidinone-appended benzothiazole–triazole hybrids using a molecular hybridization approach in a one-pot process. The synthesized compounds were tested for microbial growth inhibition against bacterial and fungal strains. Among all the synthetics, compounds derived from methoxyphenyl and heteroaromatic ring substitutions exhibited promising inhibitory activity. The current investigation has emphasized that benzothiazole–triazole hybrids incorporating thiazolidinone can be a promising and potent category of molecules with potential biological activities. This sustainable and eco-friendly protocol involves the metal-free, catalyst-free synthesis of bioactive scaffolds, which merges broad tolerance for functional groups with a short reaction time, resulting in good to excellent yields.
Introduction
The substantial discovery of drugs with enhanced applications has been a constant pursuit for a long period of time. A nuanced approach to designing and developing novel chemical entities with improved biological and pharmacological activities in drug discovery involves integrating biologically active fused heterocyclic fragments into a unified framework.1 A simple alternation with the starting material and synthetic route will lead to the sustainable synthesis of stupendous heterocyclic scaffolds. Benzothiazole is a privileged heterocycle found in several drugs and has garnered significant attention due to its versatile applications in diverse fields such as synthetic chemistry, material science, and pharmaceutical chemistry.2–4 Some of the biological properties of benzothiazole-containing heterocyclic scaffolds include antitumor activity,5 immunosuppressive activity,6 and antimicrobial activity.7 They have also been explored as scintillating fluorescent compounds,8 luminescent Ir(III) complexes containing benzothiazole-based ligands with application in organic light-emitting diodes,9 and Pd(II) pincer complexes as anticancer agents.10 Among the class of benzothiazole derivatives, benzo[4,5]thiazolo[2,3-c][1,2,4]triazole scaffolds stand out as the most potent member known to exhibit a broad range of biological activities, as shown in Fig. 1, such as anti-inflammatory activity,11 promising antimicrobial agents,12 commercially available fungicides,13,14 and act as specific kinase inhibitors & receptors,15 and show anticancer activities.16 Moreover, recently it was reported to be a nicotinamide mimicking chemotype that can inhibit different PARP family enzymes.17 In contrast, thiazolidinone has been proven to be an excellent core moiety for the development of various synthetic drugs because of its structural and synthetic diversity and broad spectrum of biological activities.18,19 Various 4-thiazolidinone derivatives have been proposed as anticancer biotargets, such as phosphatase of a regenerating liver (PRL-3),20 tumor necrosis factor TNFα,21 non-nucleoside inhibitors of HIV-RT,22 COX-2 inhibitors,23 SARS-COV-2 protease inhibitors24 and ALR2 inhibitors.25 However, the potent nature of thiazolidinone has been noted for its versatile behavior when combined with other bioactive scaffolds, showcasing a wide range of biological properties.26–28 More specifically, the condensation of the 4-thiazolidinone ring with a benzothiazole nucleus led to compounds that showed promising biological activities.29
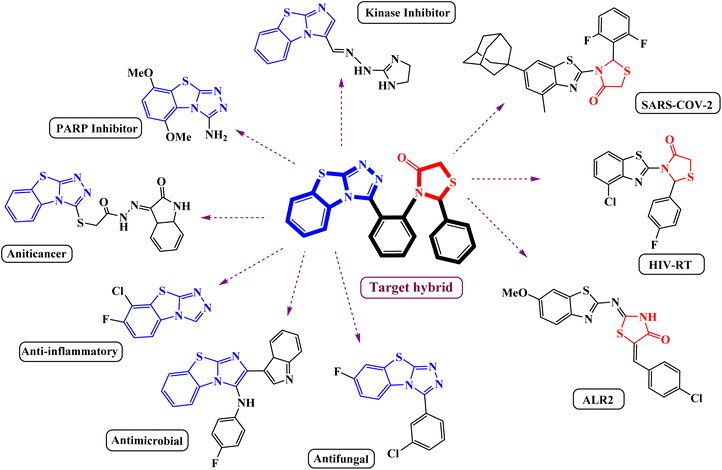 |
| Fig. 1 Synthetic drugs bearing pharmacophore sub-units. | |
Due to their intriguing biological properties and potential as pharmaceutical agents, thiazolidinone-based benzothiazole derivatives have garnered significant interest from the synthetic community. Consequently, various researchers have meticulously documented the progress of this scaffold through chemical modifications.30–32 Recently, Geronikaki et al. reported the potential pharmacological exploitation of thiazole/benzothiazole-based thiazolidinone derivatives as promising drug candidates.24 Khan et al. conducted a stepwise synthesis of thiazolidinone-based benzothiazole derivatives and demonstrated their inhibitory activity.33 The previously studied approaches to access these pharmacophores involve a common strategy employing conventional methods with synthetic precursors and catalysts. Despite the simplicity of this type of sequential synthesis, it remains challenging for chemists to construct molecular hybrids from such a convergent route. As part of our green synthetic strategy toward the synthesis of these potent hybrids, we envisioned that our metal-free approach to designing such a hybrid framework could provide a platform to synthesize a series of compounds with catalyst-free direct functionalizations on readily available starting precursors. Furthermore, such a modular strategy would be amenable to the preparation of analogs of this important scaffold.
Therefore, in light of the above three pharmacophores (benzothiazole, triazole, and thiazolidinones) have been amalgamated within a single novel hybrid using a molecular hybridization approach. With the recent advances in molecular hybridization (MH) strategies, new hybrid structures that exhibit higher biological activity, improved efficiency, and a safer toxicity profile when compared to the parent compounds could be developed by combining the active moieties of various compounds.34,35
The correlation between chemical structure and biological activity is employed to aim that the synergistic effect of these pharmacophores could lead to derive drugs that are both more potent and more specific. In this context, structure–activity relationships (SAR) have been employed to gain a comprehensive understanding of the mechanisms of multi-target mode of action of drugs36 (Fig. 2). Herein, as a part of our endeavour to create a novel bioactive heterocyclic hybrids37–40 we report the synthesis of benzo[4,5]thiazolo[2,3-c][1,2,4]triazole derivatives and thiazolidinone-appended benzo[4,5]thiazolo[2,3-c][1,2,4]triazole derivatives by employing cost-effective starting materials in a one-pot process and further evaluated them for their antimicrobial activities.
 |
| Fig. 2 Design SAR of the target compound. | |
Results and discussion
The general synthetic pathway of benzothiazolo[2,3-c][1,2,4]triazole derivatives 4a–l involves the starting material, 2-hydrazinobenzothiazole which was first prepared by the reported method.41 Firstly, a mixture of 2-mercaptobenzothiazole and hydrazine hydrate was refluxed in ethanol to obtain 2-hydrazinobenzothiazole. The reaction proceeded via a one-pot approach, a mixture of 2-hydrazinobenzothiazole 1, anthranilic acid 2, and various aromatic/heteroaromatic aldehydes 3a–l were treated under reflux conditions to obtain benzothiazole–thiazole derivatives. For another series, the targeted thiazolidinone-appended benzothiazolo[2,3-c][1,2,4]triazole derivatives 6a–h were synthesized via the addition of thioglycolic acid 5 with synthesized benzothiazole–triazoles 4 as depicted in Scheme 1. The reaction underwent screening with various solvents to optimize the reaction conditions, as outlined in Table 1. Ethanol emerged as the optimal solvent for both series, demonstrating superior yields compared to other solvents (Scheme 1 and Table 1). This outcome demonstrates the feasibility of our suggested metal-free reaction involving the synthesis of bioactive benzothiazole–triazole derivatives.
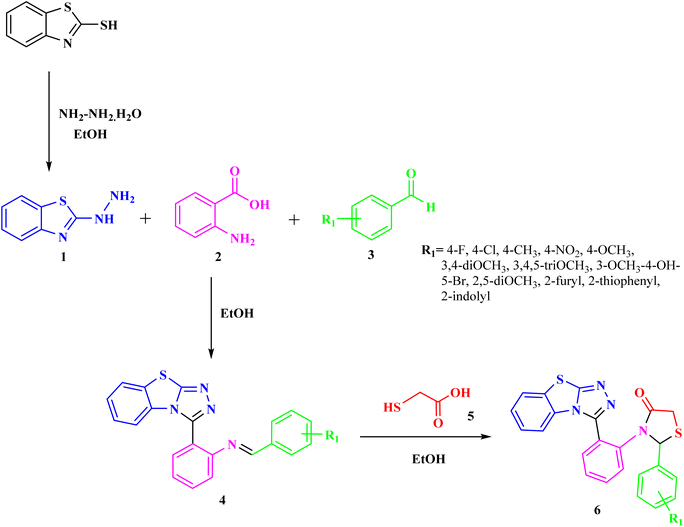 |
| Scheme 1 Synthesis of benzothiazole–triazole 4 and thiazolidinone-appended benzothiazole–triazole hybrids 6. | |
Table 1 Optimization of the reaction conditions of Scheme 1 for the synthesis of compounds 4a and 6a
Entry |
Solvent |
Reaction temp (°C) |
Time (hrs) |
Yielda (%) |
Isolated yields. |
1 |
Dioxane |
60 |
5 |
Traces |
2 |
ACN |
70 |
5 |
46 |
3 |
THF |
70 |
5 |
42 |
4 |
DMF |
70 |
5 |
52 |
5 |
MeOH |
80 |
4 |
65 |
6 |
EtOH |
80 |
2 |
87 |
The mechanistic pathway for the formation of target hybrids 4 and 6 is depicted in Scheme 2. First of all, 2-mercaptobenzothiazole A was reacted with hydrazine hydrate in ethanol to give compound 2-hydrazinobenzothiazole 1. The hydrazine derivative compound was then refluxed with anthranilic acid 2 to obtain intermediate compound E through intramolecular cyclization (C) and dehydrative aromatization (D). In the next step, compound E was treated with aromatic/heteroaromatic aldehydes 3 in ethanol and underwent proton transfer and dehydration to obtain benzothiazolo[2,3-c][1,2,4]triazole derivatives (4a–l) in excellent yields. For the synthesis of our next series, as a result of the condensation reaction of compound 4 and thioglycolic acid 5, the target thiazolidinone appended benzothiazole–triazole hybrids (6a–h) were obtained.
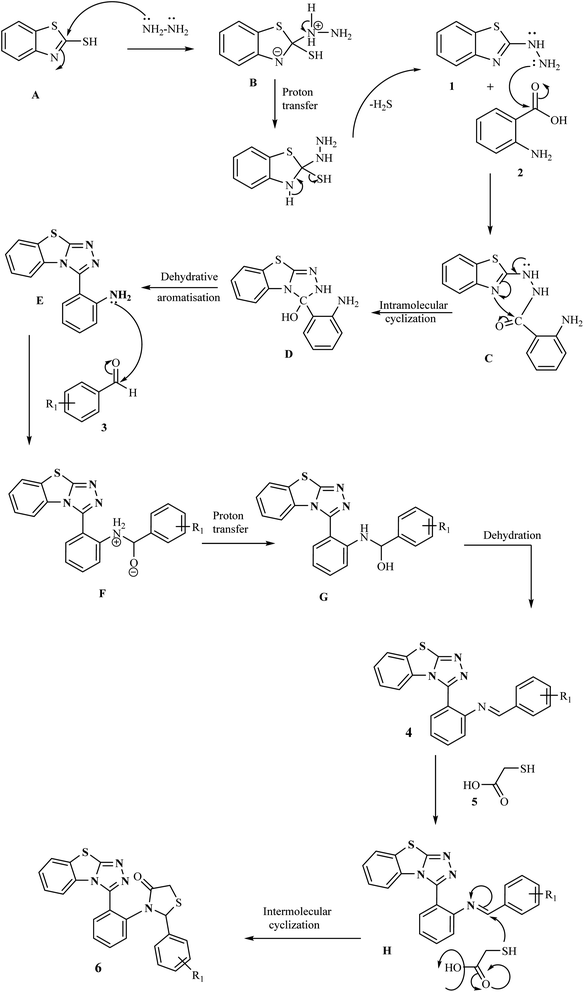 |
| Scheme 2 Plausible mechanistic pathway for the synthesis of target hybrids. | |
The substrate scope and complete reaction conditions involved in the synthesis of benzothiazole–triazole derivatives (4a–l) and thiazolidinone appended benzothiazole–triazole derivatives (6a–h) are illustrated in Schemes 3 and 4 respectively. The structures of all the synthetics 4a–l and 6a–h were in agreement with their spectral and analytical analyses which are summarized in ESI.† From the IR spectrum of compound 4a, the appearance of peaks at 1634 cm−1 indicated the presence of C
N attached to the phenyl ring. The peaks of CH proton in 1H-NMR spectra which are common to all of the compounds, were observed as singlets in the range of 8.27–8.93 ppm. The signals belonging to aromatic protons of benzothiazole ring and phenyl rings were found between 6.58–7.99 ppm. In 13C-NMR spectra, signals belonging to CH proton in all synthesized compounds were detected in the range of 159.0–160.9 ppm. All masses were in accordance with the estimated M+H values.
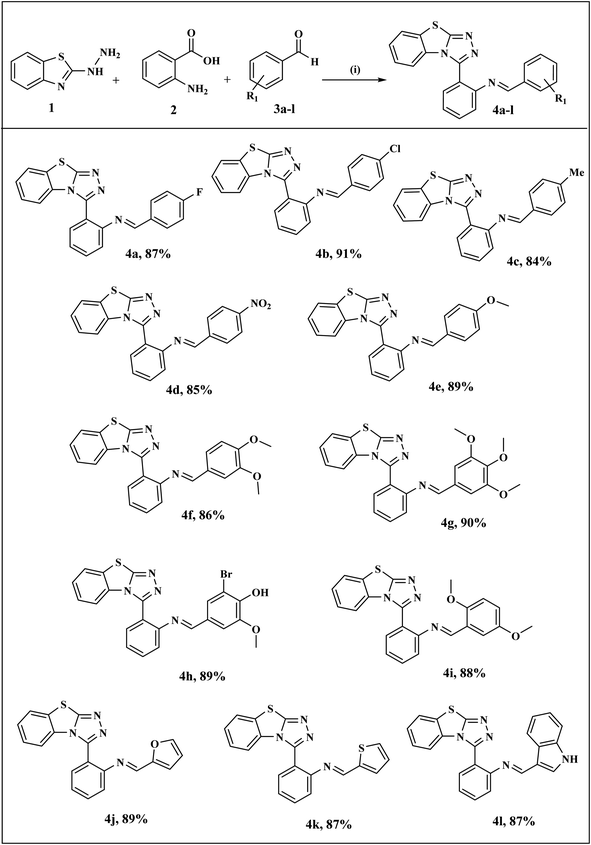 |
| Scheme 3 Synthesis of benzothiazolo[2,3-c][1,2,4]triazole derivatives (4a–l) Reagents and conditions: (i) EtOH, reflux, 2 h. | |
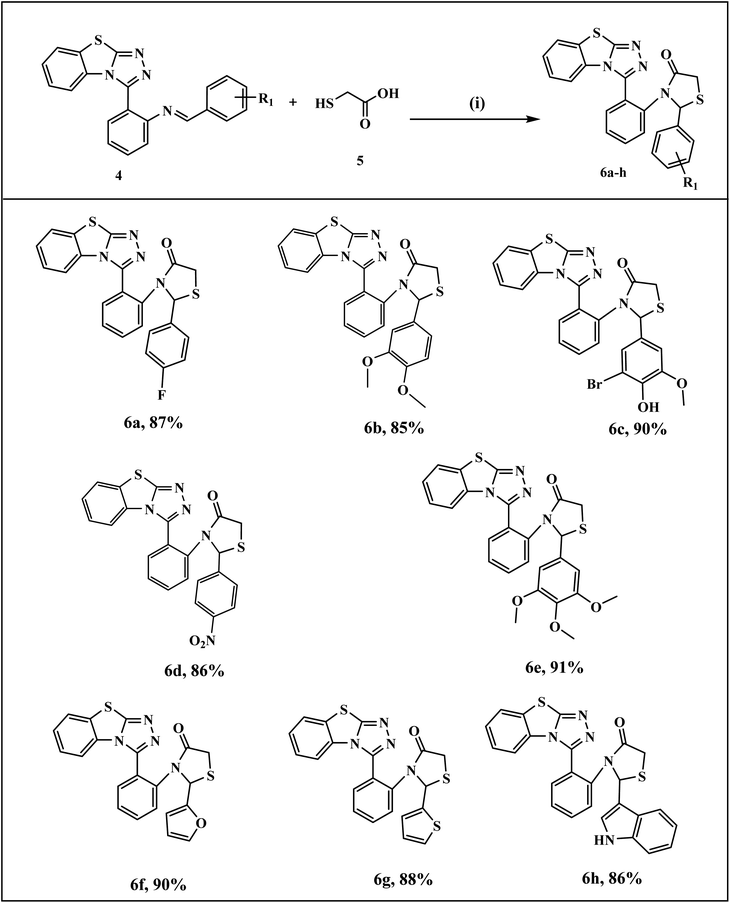 |
| Scheme 4 Synthesis of benzo[4,5]thiazolo[2,3-c][1,2,4]triazol-2-phenylthiazolidin-4-one derivatives (6a–h) Reagents and conditions: (i) EtOH, reflux, 2 h. | |
In IR spectra, characteristic peaks were observed for C
O stretching and C–S stretching of the thiazolidinone derivatives in the range of 1690–1710 cm−1 and 685–692 cm−1 respectively. 1H-NMR spectra of compound 6a indicated the presence of two diastereotopic protons at the C-5 position appearing as doublets at 3.59, and 3.90 ppm and one single proton at C-2 position appearing as singlet at 5.93 ppm. In 13C NMR spectra, chemical shifts of the thiazolidinone hybrids resonate in the region of 33.1–35.1 ppm (methylene carbon, thiazolidinone ring), 168.5–169.9 ppm (carbonyl carbon (>C
O) thiazolidinone ring), 112.0–158.2 ppm (aromatic carbons).
Antimicrobial evaluation
The newly desired synthesized molecular hybrids (6a–h) were screened for their antimicrobial activity against some selected bacterial and fungal strains and examined for the inhibition of the growth of the organism by employing the agar well diffusion method.42 Both Gram-positive (Staphylococcus aureus [MTCC 96], Bacillus subtilis [MTCC 441]) and Gram-negative (Klebsiella planticola [MTCC 530], Pseudomonas aeruginosa [MTCC 2453]) pathogenic bacterial strains were used in the present work with ciprofloxacin as the standard drug. Antifungal activity was conducted against Candida albicans [MTCC 3017], Candida albicans [MTCC 1637], and Aspergillus niger [MTCC 872] using miconazole as the standard drug. The minimum inhibitory concentration (MIC) of the synthesized compounds were tabulated in Tables 2 and 3.
Table 2 Antibacterial activity (in μg mL−1) of the synthesized compounds (6a–h)
Compound |
Gram-positive bacteria (MIC) |
Gram-negative bacteria (MIC) |
S. aureus MTCC 96 |
B. subtilis MTCC 441 |
K. planticola MTCC 530 |
P. aeruginosa MTCC 2453 |
6a |
>125 |
>125 |
>125 |
>125 |
6b |
7.8 |
3.9 |
7.8 |
7.8 |
6c |
>125 |
7.8 |
15.9 |
7.8 |
6d |
7.8 |
3.9 |
7.8 |
>125 |
6e |
1.9 |
3.9 |
3.9 |
3.9 |
6f |
3.9 |
7.8 |
7.8 |
3.9 |
6g |
7.8 |
7.8 |
3.9 |
7.8 |
6h |
1.9 |
1.9 |
3.9 |
1.9 |
Ciprofloxacin |
0.9 |
0.9 |
0.9 |
0.9 |
Table 3 Antifungal activity (in μg mL−1) of the synthesized compounds (6a–h)
Compound |
C. albicans MTCC 3017 (MIC) |
C. albicans MTCC 1637 (MIC) |
A. niger MTCC 872 (MIC) |
6a |
>125 |
>125 |
>125 |
6b |
7.8 |
>125 |
15.9 |
6c |
15.9 |
>125 |
>125 |
6d |
7.8 |
15.9 |
7.8 |
6e |
3.9 |
7.8 |
7.8 |
6f |
7.8 |
3.9 |
15.9 |
6g |
3.9 |
7.8 |
7.8 |
6h |
3.9 |
7.8 |
3.9 |
Miconazole |
1.9 |
1.9 |
1.9 |
In general, most of the tested compounds (6a–h) exhibited a certain degree of inhibitory activity. The SAR studies suggested that compounds 6b, 6d, 6e, 6f, 6g, and 6h exhibited significant inhibitory effects on bacterial and fungal growth (Tables 2 and 3). Among thiazolidinone derivatives, compound 6h bearing indolyl ring was the most active antibacterial agent compared to standard drugs. The hybrid, 6b with a 3,4-diOCH3 phenyl substitution showed a very good activity against different bacterial strains. Compound 6d shows better activity than compound 6a, replacement of 4-fluorophenyl with the 4-nitrophenyl group resulted in a significant increase in the activity. The compound 6c with 3-OCH3 and 4-OH phenyl substitution with bromo at the 5th position exhibited good to moderate antimicrobial activity against the test pathogens except for S. aureus [MTCC 96]. In addition, compound 6e with 3,4,5-triOCH3 phenyl substitution showed excellent activity than 6c and 6d. The compounds 6f and 6g bearing furan and thiophene ring exhibited good to excellent antimicrobial activity against the tested pathogens.
Additionally, our novel synthesized compounds were also screened for antifungal activity (Table 3). The results showed that compound 6h showed potent activity and compound 6e displayed excellent antifungal activity as compared to standard. The superiority of the former could be attributed to the higher basicity given by indole moiety as the pyrrole ring fused with benzene has yielded more fruitful results than phenyl-substituted groups. Interestingly, the replacement of the indole ring with a thiophene ring to afford compound 6g produced stronger antifungal activity but less than compound 6h. In general, the rest of the tested compounds showed significant results. Like in antimicrobial activity, compound 6e also showed excellent to good activity against antifungal strains. Moreover, the methoxy-substituted derivative 6b favored the activity. This demonstrates the substantial impact of the indolyl ring in augmenting the activity of the targeted compounds in both series. The presence of methoxy groups either on o/m/p-positions of the phenyl ring is evident for optimum activity.
We believe that the significant antimicrobial activities of the synthesized compounds could be due to the fusion of the thiazolidinone ring with the benzothiazole–triazole pharmacophore. To date, several papers have shown that 4-thiazolidinone derivatives could potentially be more effective against antibiotic-resistant bacterial strains. Skora et al. reported that synthesized thiazolidinone derivatives showed high antibacterial activity, as they caused a decrease in biofilm biomass at the concentrations.43 As reported by Haroun et al., some new benzothiazole-based thiazolidinones showed potent antimicrobial activity, causing inhibition of bacterial and fungal growth.44 Tratrat et al. highlighted that some synthesized thiazolidinones showed excellent antifungal and antibacterial activities against different pathogenic strains.45 Considering the literature data cited above and our results, it can be concluded that some synthesized thiazolidinone-appended benzothiazole–triazole derivatives can be valuable compounds in the treatment of antibiotic-resistant bacterial strains.
Experimental
Materials and techniques for analysis
All the solvents and reagents (analytical grade) were purchased from commercial suppliers and were used in synthesis without additional purification in the experimental procedures. The melting points of all synthesized compounds were analyzed using a digital melting point apparatus. The purity of the compounds was assessed using thin-layer silica gel-G-coated glass plates with a benzene: ethyl acetate (8
:
2) eluent. Infrared (IR) spectra were recorded using a Shimadzu FT IR-8400S spectrophotometer with KBr pellets. Mass spectrometry measurements were obtained on Waters Xevo G2-S QT LC/MS spectrometer. 1H and 13C NMR spectra were recorded on a Jeol Resonance at 400 and 100 MHz, respectively in dimethyl sulfoxide (DMSO-d6) and CDCl3 as a solvent. Chemical shifts and absorption frequency have been expressed in terms of δ (ppm) and ν (cm−1), respectively. The abbreviations have been used in the spectral data as singlet (s), doublet (d), doublet of doublet (dd), and multiplet (m). ElementarVarioEL III Elemental analyzer is found helpful in elemental analyses.
Antimicrobial activity
Gram-positive (Staphylococcus aureus [MTCC 96], Bacillus subtilis [MTCC 441]) and Gram-negative (Klebsiella planticola [MTCC 530], Pseudomonas aeruginosa [MTCC 2453]) pathogenic bacterial strains and Candida albicans [MTCC 3017], Candida albicans [MTCC 1637] and Aspergillus niger [MTCC 872] fungal strains were used in this experiment. The antimicrobial properties of various synthesized compounds were assessed using the agar well diffusion method. Mueller Hinton agar plates were evenly inoculated with pathogenic strains, each at a concentration equivalent to 0.5 McFarland standards (1.5 × 108 cfu mL−1). In a sterile laminar airflow chamber, 6 mm diameter wells were created on the agar plates. These wells were then filled with test samples dissolved in 2% DMSO, with concentrations ranging from 125 to 0.97 μg mL−1. Ciprofloxacin and miconazole were used as positive controls, while DMSO served as the vehicle control. The plates were incubated at 37 °C for 24 hours for bacteria and 28 °C for 48 hours for fungi. After incubation, the minimum inhibitory concentration (MIC) was determined as the lowest concentration in the series where no inhibition of visual growth was observed in the wells. The entire experiment was conducted in duplicate, and the mean values were reported.
General procedure for the synthesis of benzo[4,5]thiazolo[2,3-c][1,2,4]triazol-3-yl-phenyl-1-phenylmethanimine (4a–l)
The reaction was carried out by using an equimolar mixture of 2-hydrazinobenzothiazole 1 (1 mmol), anthranilic acid 2 (1 mmol), and aromatic/heteroaromatic aldehydes 3a–l (1 mmol), the mixture was refluxed in ethanol (10 mL) as solvent at 80 °C for 2 h which afforded pale yellow colored solid. After the completion of the reaction as indicated by TLC, the reaction mixture was cooled at room temperature, and obtained precipitate was filtered under vacuum, washed with chilled water a few times, dried, and used in the next step without further purification.
General procedure for the synthesis of benzo[4,5]thiazolo[2,3-c][1,2,4]triazol-3-yl-phenyl-2-phenylthiazolidin-4-one (6a–h)
To pure synthesized compounds, benzo[4,5]thiazolo[2,3-c][1,2,4]triazol-3-yl-phenyl-1-phenylmethanimine 4 (1 mmol) with thioglycolic acid 5 (1 mmol) in ethanol (10 mL) were heated at refluxing for 2 h at 80 °C which afforded solid mass. After the completion of the reaction, as indicated by TLC, the reaction mixture was cooled to room temperature, and the resulting precipitate was filtered under vacuum. It was then washed several times with chilled water, and dried, and the desired compounds were obtained with good to excellent yields after further recrystallization. The products were determined via the use of melting point, FT-IR, 1H-NMR, 13C-NMR and mass spectra.
N-(2-(Benzo[4,5]thiazolo[2,3-c][1,2,4]triazol-3-yl)phenyl)-1-(4-fluorophenyl)methanimine (4a). Pale yellow solid, m.p. 284 °C, FT-IR (KBr, cm−1): 1634 (C
N); 1H-NMR (CDCl3, 400 MHz) δ: 8.27 (s, 1H, CH), 7.41–7.45 (m, 4H, Ar–H), 7.33 (d, 2H, Ar–H, J = 8.4 Hz), 6.96 (d, 2H, Ar–H, J = 8.4 Hz), 6.79–6.88 (m, 4H, Ar–H) ppm; 13C-NMR (DMSO-d6, 100 MHz) δ: 161.2, 160.9, 158.4, 153.8, 140.6, 139.7, 133.0, 132.1, 131.5, 130.4, 126.6, 126.2, 124.9, 122.8, 122.6, 122.5, 119.4, 118.9, 116.69, 116.4 ppm; MS (m/z): 373.084 [M+H]+; anal. calcd for C21H13FN4S: C 67.73; H 3.52; N 15.04; S 8.61%; found C 67.85; H 3.49; N 14.99; S 8.55%.
N-(2-(Benzo[4,5]thiazolo[2,3-c][1,2,4]triazol-3-yl)phenyl)-1-(4-chlorophenyl)methanimine (4b). Pale yellow solid, m.p. 282 °C, FT-IR (KBr, cm−1): 1646 (C
N); 1H-NMR (CDCl3, 400 MHz) δ: 8.43 (s, 1H, CH) 7.35–7.44 (m, 4H, Ar–H), 7.12–7.17 (m, 4H, Ar–H), 6.98 (d, 2H, Ar–H, J = 8.8 Hz), 6.79 (d, 2H, Ar–H, J = 8.8 Hz) ppm; 13C-NMR (DMSO-d6, 100 MHz) δ: 160.6, 153.5, 152.2, 148.4, 148.3, 146.1, 144.9, 143.0, 131.2, 131.1, 127.9, 125.4, 124.7, 124.6, 121.8, 118.6, 118.5, 110.7, 110.5, 110.2 ppm; MS(m/z): 389.055 [M+H]+; anal. calcd for C21H13ClN4S: C 64.86; H 3.37; Cl 9.12; N 14.41; S 8.24%; found C 64.98; H 3.34; N 14.36; S 8.18%.
N-(2-(Benzo[4,5]thiazolo[2,3-c][1,2,4]triazol-3-yl)phenyl)-1-(p-tolyl)methanimine (4c). Yellow solid, m.p. 260 °C, FT-IR (KBr, cm−1) δ: 1653 (C
N); 1H-NMR (DMSO-d6, 400 MHz) δ: 8.36 (s, 1H, CH), 7.94–7.99 (m, 4H, Ar–H), 7.66 (dd, 2H, Ar–H, J = 8.0, 6.4 Hz), 7.47 (d, 2H, Ar–H, J = 8 Hz), 7.13 (dd, 4H, Ar–H, J = 8.4, 6.2 Hz), 2.31 (s, 3H, CH3) ppm; 13C-NMR (DMSO-d6, 100 MHz) δ: 160.9, 152.9, 148.1, 148.0, 145.1, 142.1, 138.3, 138.2, 135.5, 135.4, 131.6, 131.5, 129.9, 129.8, 129.6, 128.5, 127.9, 127.8, 126.1, 124.1, 120.9, 21.2 ppm; MS(m/z): 369.110 [M+H]+; anal. calcd for C22H16N4S: C 71.72; H 4.38; N 15.21; S 8.70%; found C 71.84; H 4.35; N 15.16; S 8.64%.
N-(2-(Benzo[4,5]thiazolo[2,3-c][1,2,4]triazol-3-yl)phenyl)-1-(4-nitrophenyl)methanimine (4d). Yellow solid, m.p. 286 °C, FT-IR (KBr, cm−1): 1647 (C
N); 1H-NMR (DMSO-d6, 400 MHz) δ: 8.67 (s, 1H, CH), 7.40–7.44 (m, 3H, Ar–H), 7.20–7.26 (m, 2H, Ar–H), 7.00 (d, 2H, Ar–H, J = 7.2 Hz), 6.88 (d, 2H, Ar–H, J = 7.2 Hz), 6.58–6.68 (m, 3H, Ar–H) ppm; 13C-NMR (DMSO-d6, 100 MHz) δ: 161.6, 160.8, 160.7, 156.2, 155.4, 148.9, 140.1, 139.8, 133.3, 133.1, 131.3, 131.2, 129.8, 126.8, 126.2, 124.8,124.0, 122.2, 119.6, 119.5 ppm; MS(m/z): 400.079 [M+H]+; anal. calcd for C21H13N5O2S: C 63.15; H 3.28; N 17.53; O 8.01; S 8.03%; found C 63.27; H 3.25; N 17.48; O 7.93; S 7.97%.
N-(2-(Benzo[4,5]thiazolo[2,3-c][1,2,4]triazol-3-yl)phenyl)-1-(4-methoxyphenyl)methanimine (4e). White solid, m.p. 245 °C, FT-IR (KBr, cm−1): 1637 (C
N); 1H-NMR (DMSO-d6, 400 MHz) δ: 8.48 (s, 1H, CH), 7.76 (d, 2H, Ar–H, J = 8.4 Hz), 7.63–7.74 (m, 2H, Ar–H), 7.54 (d, 2H, Ar–H, J = 8.4 Hz), 7.41–7.43 (m, 3H, Ar–H), 7.01–7.28 (m, 3H, Ar–H), 3.68 (s, 3H, OCH3) ppm; 13C-NMR (DMSO-d6, 100 MHz) δ: 161.4, 160.4, 154.9, 154.8, 148.9, 145.5, 145.4, 142.7, 139.0, 138.9, 138.8, 136.1, 135.9, 131.5, 129.8, 128.1, 126.9, 125.9, 124.9, 119.6, 55.0 ppm; MS(m/z): 385.104 [M+H]+; anal. calcd for C22H16N4OS: C 68.73; H 4.20; N 14.57; O 4.16; S 8.34%; found C 68.85; H 4.17; N 14.52; O 4.08; S 8.28%.
N-(2-(Benzo[4,5]thiazolo[2,3-c][1,2,4]triazol-3-yl)phenyl)-1-(3,4-dimethoxyphenyl)methanimine (4f). White solid, m.p. 281 °C, FT-IR (KBr, cm−1): 1641 (C
N); 1H-NMR (DMSO-d6, 400 MHz) δ: 8.34 (s, 1H, CH), 7.42–7.59 (m, 4H, Ar–H), 7.31 (s, 1H, Ar–H), 6.95–7.07 (m, 2H, Ar–H), 6.79 (d, 2H, Ar–H, J = 7.6 Hz), 6.64 (d, 2H, Ar–H, J = 7.4 Hz), 3.75 (s, 3H, OCH3), 3.73 (s, 3H, OCH3) ppm; 13C-NMR (DMSO-d6, 100 MHz) δ: 161.9, 161.8, 153.1, 152.9, 146.5, 146.4, 140.5, 140.3, 136.2, 133.8, 133.6, 130.0, 129.8, 124.5, 124.3, 122.7, 122.5, 118.4, 118.1, 53.1, 53.0 ppm; MS(m/z): 415.115 [M+H]+; anal. calcd for C23H18N4O2S: C 66.65; H 4.38; N 13.52; O 7.72; S 7.73%; found C 66.77; H 4.35; N 13.47; O 7.64; S 7.67%.
N-(2-(Benzo[4,5]thiazolo[2,3-c][1,2,4]triazol-3-yl)phenyl)-1-(3,4,5-trimethoxyphenyl)methanimine (4g). White solid, m.p. 220 °C, FT-IR (KBr, cm−1): 1645 (C
N); 1H-NMR (DMSO-d6, 400 MHz) δ: 8.93 (s, 1H, CH), 7.68–7.73 (m, 5H, Ar–H), 7.50–7.54 (m, 3H, Ar–H), 7.38 (s, 1H, Ar–H), 7.36 (s, 1H, Ar–H), 3.61 (s, 3H, di-OCH3), 3.45 (s, 3H, OCH3) ppm; 13C-NMR (DMSO-d6, 100 MHz) δ: 161.3, 160.9, 153.4, 153.1, 148.2, 147.0, 141.9, 141.7, 133.9, 133.2, 132.9, 132.7, 127.1, 126.9, 126.8, 123.2, 123.0, 117.6, 117.5, 117.2, 56.4, 53.1, 53.0 ppm; MS(m/z): 445.126 [M+H]+; anal. calcd for C24H20N4O3S: C 64.85; H 4.54; N 12.60; O 10.80; S 7.21%; found C 64.73; H 4.51; N 12.55; O 10.72; S 7.15%.
4-(((2-(Benzo[4,5]thiazolo[2,3-c][1,2,4]triazol-3-yl)phenyl)imino)methyl)-2-bromo-6-methoxyphenol (4h). Pale yellow solid, m.p. 278 °C, FT-IR (KBr, cm−1): 1639 (C
N); 1H-NMR (DMSO-d6, 400 MHz) δ: 9.49 (s, 1H, OH), 8.64 (s, 1H, CH), 7.46–7.64 (m, 8H, Ar–H), 7.30 (s, 1H, Ar–H), 7.07 (s, 1H, Ar–H), 3.85 (s, 3H, OCH3) ppm; 13C-NMR (DMSO-d6, 100 MHz) δ: 162.2, 159.7, 152.6, 147.9, 147.7, 145.8, 143.8, 143.6, 140.1, 139.8, 137.9, 135.5, 134.3, 130.0, 129.9, 129.8, 128.5, 126.1, 125.3, 120.9, 120.8, 56.4 ppm; MS(m/z): 479.010 [M+H]+; anal. calcd for C22H15BrN4O2S: C 55.12; H 3.15; N 11.69; O 6.68; S 6.69%; found C 55.24; H 3.12; N 11.64; O 6.60; S 6.63%.
N-(2-(Benzo[4,5]thiazolo[2,3-c][1,2,4]triazol-3-yl)phenyl)-1-(2,5-dimethoxyphenyl)methanimine (4i). Pale yellow solid, m.p. 283 °C, FT-IR (KBr, cm−1): 1640 (C
N); 1H-NMR (DMSO-d6, 400 MHz) δ: 8.86 (s, 1H, CH), 7.51 (d, 2H, Ar–H, J = 8 Hz), 7.39 (d, 2H, Ar–H, J = 8 Hz), 7.12–7.28 (m, 2H, Ar–H), 6.88 (d, 2H, Ar–H, J = 8 Hz), 6.75–6.81 (m, 3H, Ar–H), 3.75 (s, 3H, OCH3), 3.61 (s, 3H, OCH3), ppm; 13C-NMR (DMSO-d6, 100 MHz) δ: 161.9, 160.4, 156.5, 155.0, 148.9, 140.0, 135.4, 134.1, 133.8, 129.6, 126.8, 124.9, 124.1, 119.6, 118.5, 118.2, 116.1, 112.7, 111.6, 111.5, 56.0, 55.2 ppm; MS(m/z): 415.115 [M+H]+; anal. calcd for C23H18N4O2S: C 66.65; H 4.38; N 13.52; O 7.72; S 7.73% found C 66.77; H 4.35; N 13.47; O 7.64; S 7.67%.
(E)-N-(2-(Benzo[4,5]thiazolo[2,3-c][1,2,4]triazol-3-yl)phenyl)-1-(furan-2-yl)methanimine (4j). Pale yellow solid, m.p. 276 °C, FT-IR (KBr, cm−1): 1643 (C
N); 1H-NMR (DMSO-d6, 400 MHz) δ: 8.55 (s, 1H, CH), 7.34–7.49 (m, 7H, Ar–H), 7.06–7.14 (m, 2H, Ar–H), 6.74 (dd, 2H, Ar–H, J = 6.8, 7.2 Hz) ppm; 13C-NMR (DMSO-d6, 100 MHz) δ: 161.1, 154.7, 148.9, 138.4, 133.4, 130.8, 129.8, 126.9, 126.4, 124.9, 124.7, 123.2, 123.1, 121.7, 121.6, 119.7, 119.6, 117.9, 112.7 ppm; MS(m/z): 345.073 [M+H]+; anal. calcd for C19H12N4OS: C 66.26; H 3.51; N 16.27; O 4.65; S 9.31% found C 66.38; H 3.48; N 16.22; O 4.57; S 9.25%.
(E)-N-(2-(Benzo[4,5]thiazolo[2,3-c][1,2,4]triazol-3-yl)phenyl)-1-(thiophen-2-yl)methanimine (4k). Pale yellow solid, m.p. 295 °C, FT-IR (KBr, cm−1): 1641 (C
N); 1H-NMR (DMSO-d6, 400 MHz) δ: 8.63 (s, 1H, CH), 7.42–7.48 (m, 4H, Ar–H), 7.26–7.32 (m, 2H, Ar–H), 7.12 (d, 2H, Ar–H, J = 7.4 Hz), 7.03–7.08 (m, 3H, Ar–H) ppm; 13C-NMR (DMSO-d6, 100 MHz) δ: 162.4, 154.9, 154.8, 152.1, 148.9, 142.7, 131.5, 129.4, 128.1, 126.9, 125.9, 124.9, 124.7, 124.6, 122.5, 122.3, 122.1, 119.6 ppm; MS(m/z): 361.050 [M+H]+; anal. calcd for C19H12N4S2: C 63.31; H 3.36; N 15.54; S 17.79% found C 63.43; H 3.33; N 15.49; S 17.73%.
(E)-N-(2-(Benzo[4,5]thiazolo[2,3-c][1,2,4]triazol-3-yl)phenyl)-1-(1H-indol-3-yl)methanimine (4l). Yellow solid, m.p. 289 °C, FT-IR (KBr, cm−1): 1638 (C
N), 3424 (N–H); 1H-NMR (DMSO-d6, 400 MHz) δ: 10.55 (s, 1H, NH), 8.79 (s, 1H, CH), 7.33–7.42 (m, 5H, Ar–H), 7.11–7.18 (m, 3H, Ar–H), 6.96 (s, 1H, Ar–H), 6.71 (dd, 4H, Ar–H, J = 7.2, 6.4 Hz) ppm; 13C-NMR (DMSO-d6, 100 MHz) δ: 162.4, 160.4, 154.9, 148.9, 143.1, 134.4, 134.1, 130.8, 129.7, 128.4, 128.2, 126.9, 124.9, 124.6, 123.8, 123.6, 122.3, 122.2, 121.1, 119.6, 113.5, 112.7, 112.6 ppm; MS(m/z): 394.105 [M+H]+; anal. calcd for C23H15N5S: C 70.21; H 3.84; N 17.80; S 8.15% found C 70.33; H 3.81; N 17.75; S 8.09%.
3-(2-(Benzo[4,5]thiazolo[2,3-c][1,2,4]triazol-3-yl)phenyl)-2-(4-fluorophenyl)thiazolidin-4-one (6a). Pale yellow solid, m.p. 280 °C, FT-IR (KBr, cm−1): 1690 (C
O), 685 (C–S); 1H-NMR (DMSO-d6, 400 MHz) δ: 7.60–7.62 (m, 2H, Ar–H), 7.54–7.58 (m, 2H, Ar–H), 7.42–7.46 (m, 2H, Ar–H), 7.21–7.32 (m, 2H, Ar–H), 6.64–7.05 (m, 4H, Ar–H), 5.93 (s, 1H, CH), 3.90 (d, 1H, CH2, J = 8.8 Hz), 3.59 (d, 1H, CH2, J = 8.8 Hz) ppm; 13C-NMR (DMSO-d6, 100 MHz) δ: 35.5, 77.7, 112.3, 112.7, 119.2, 119.5, 121.9, 123.6, 123.9, 124.8, 126.2, 126.5, 126.8, 129.2, 131.2, 131.6, 133.0, 133.3, 139.8, 140.1, 148.9, 155.4, 169.6 ppm; MS(m/z): 447.067 [M+H]+; anal. calcd for C23H15FN4OS2: C 61.87; H 3.39; F 4.25; N 12.55; O 3.58; S 14.36% found C 61.99; H 3.36; N 12.50; O 3.50; S 14.30%
3-(2-(Benzo[4,5]thiazolo[2,3-c][1,2,4]triazol-3-yl)phenyl)-2-(3,4-dimethoxyphenyl)thiazolidin-4-one (6b). White solid, m.p. 278 °C, FT-IR (KBr, cm−1): 1710 (C
O), 692 (C–S); 1H-NMR (DMSO-d6, 400 MHz) δ: 7.60–7.62 (m, 1H, Ar–H), 7.43–7.45 (m, 3H, Ar–H), 7.18–7.27 (m, 3H, Ar–H), 7.06 (s, 1H, Ar–H), 6.86–6.99 (m, 3H, Ar–H), 6.042 (s, 1H, CH), 4.162 (d, 1H, CH2, J = 9.6 Hz), 3.70 (d, 1H, CH2, J = 9.6 Hz), 3.40 (s, 3H, OCH3), 3.31 (s, 3H, OCH3) ppm; 13C-NMR (DMSO-d6, 100 MHz) δ: 35.3, 53.1, 53.8, 68.3, 112.7, 116.4, 116.7, 119.6, 119.7, 123.2, 123.5, 124.9, 126.4, 126.9, 129.9, 130.8, 133.4, 133.7, 139.4, 139.8, 142.2, 148.9, 154.7, 168.9 ppm; MS(m/z): 489.098 [M+H]+; anal. calcd for C25H20N4O3S2: C 61.46; H 4.13; N 11.47; O 9.82; S 13.12% found C 61.58; H 4.10; N 11.42; O 9.74; S 13.06%
3-(2-(Benzo[4,5]thiazolo[2,3-c][1,2,4]triazol-3-yl)phenyl)-2-(3-bromo-4-hydroxy-5-methoxyphenyl)thiazolidin-4-one (6c). Pale yellow solid, m.p. 272 °C, FT-IR (KBr, cm−1): 1704 (C
O), 689 (C–S); 1H-NMR (DMSO-d6, 400 MHz) δ: 9.53 (s, 1H, OH), 7.72 (s, 1H, Ar–H), 7.52–7.54 (m, 1H, Ar–H), 7.25–7.32 (m, 6H, Ar–H), 6.98–7.11 (m, 1H, Ar–H), 6.77 (s, 1H, Ar–H),6.30 (s, 1H, CH), 4.26 (d, 1H, CH2, J = 5.2 Hz), 3.70 (d, 1H, CH2, J = 5.2 Hz), 3.40 (s, 3H, OCH3) ppm; 13C-NMR (DMSO-d6, 100 MHz) δ: 35.1, 56.0, 72.5, 112.1, 112.7, 119.6, 124.7, 124.9, 125.9, 126.9, 128.1, 129.8, 131.5, 133.7, 133.9, 134.1, 135.5, 139.7, 140.0, 142.7, 148.9, 154.7, 154.9, 169.4 ppm; MS(m/z): 552.993 [M+H]+; anal. calcd for C24H17BrN4O3S2: C 52.09; H 3.10; N 10.12; O 8.67; S 11.59% found C 52.21; H 3.07; N 10.07; O 8.59; S 11.53%.
3-(2-(Benzo[4,5]thiazolo[2,3-c][1,2,4]triazol-3-yl)phenyl)-2-(4-nitrophenyl)thiazolidin-4-one (6d). Yellow solid, m.p. 281 °C, FT-IR (KBr, cm−1): 1708 (C
O), 691 (C–S); 1H-NMR (DMSO-d6, 400 MHz) δ: 7.88–7.92 (m, 1H, Ar–H), 7.58–7.60 (m, 1H, Ar–H), 7.45–7.48 (m, 1H, Ar–H), 7.32–7.37 (m, 5H, Ar–H), 7.19–7.22 (m, 3H, Ar–H), 6.78–6.80 (m, 1H, Ar–H), 6.32 (s, 1H, CH),3.80 (d, 1H, CH2, J = 8.0 Hz), 3.38 (d, 1H, CH2, J = 8.0 Hz), ppm; 13C-NMR (DMSO-d6, 100 MHz) δ: 35.1, 72.6, 111.5, 111.6, 112.7, 116.0, 119.6, 124.1, 124.9, 126.8, 129.6, 136.2, 138.1, 140.0, 144.1, 144.5, 145.1, 146.3, 148.9, 155.0, 156.5, 169.5 ppm; MS(m/z): 474.062 [M+H]+; anal. calcd for C23H15N5O3S2: C 58.34; H 3.19; N 14.79; O 10.14; S 13.54% found C 58.46; H 3.16; N 14.74; O 10.06; S 13.48%.
3-(2-(Benzo[4,5]thiazolo[2,3-c][1,2,4]triazol-3-yl)phenyl)-2-(3,4,5-trimethoxyphenyl)thiazolidin-4-one (6e). White solid, m.p. 216 °C, FT-IR (KBr, cm−1): 1700 (C
O), 687 (C–S); 1H-NMR (DMSO-d6, 400 MHz) δ: 7.72 (s, 1H, Ar–H), 7.53–7.57 (m, 2H, Ar–H), 7.24–7.34 (m, 6H, Ar–H), 6.76 (s, 1H, Ar–H), 6.18 (s, 1H, CH), 4.08 (d, 1H, CH2, J = 7.2 Hz), 3.90 (d, 1H, CH2, J = 7.2 Hz), 3.42 (s, 3H, OCH3), 3.35 (s, 6H, OCH3), ppm; 13C-NMR (DMSO-d6, 100 MHz) δ: 35.1, 56.0, 56.8, 60.3, 72.2, 110.3, 110.7, 112.2, 112.6, 119.5, 122.3, 124.4, 124.8, 125.7, 126.5, 126.8, 128.9, 129.2, 130.2, 130.8, 141.3, 141.6, 148.9, 154.0, 155.3, 169.6 ppm; MS(m/z): 519.108 [M+H]+; anal. calcd for C26H22N4O4S2: C 60.22; H 4.28; N 10.80; O 12.34; S 12.36% found C 60.34; H 4.25; N 10.75; O 12.26; S 12.28%.
3-(2-(Benzo[4,5]thiazolo[2,3-c][1,2,4]triazol-3-yl)phenyl)-2-(furan-2-yl)thiazolidin-4-one (6f). Pale yellow solid, m.p. 271 °C, FT-IR (KBr, cm−1): 1705 (C
O), 688 (C–S); 1H-NMR (DMSO-d6, 400 MHz) δ: 7.72–7.73 (m, 1H, Ar–H), 7.52–7.54 (m, 1H, Ar–H), 7.42–7.45 (m, 1H, Ar–H), 7.24–7.31 (m, 4H, Ar–H), 6.98–7.19 (m, 2H, Ar–H), 6.71–6.75 (m, 1H, Ar–H), 6.31 (s, 1H, CH), 3.61 (d, 1H, CH2, J = 8.8 Hz), 3.41 (d, 1H, CH2, J = 8.8 Hz) ppm; 13C-NMR (DMSO-d6, 100 MHz) δ: 35.2, 67.5, 112.6, 112.7, 113.5, 119.6, 122.0, 122.6, 124.6, 124.9, 126.9, 128.1, 129.7, 130.8, 133.2, 133.4, 134.4, 143.1, 148.9, 154.9, 169.4 ppm; MS(m/z): 419.056 [M+H]+; anal. calcd for C21H14N4O2S2: C 60.27; H 3.37; N 13.39; O 7.65; S 15.32% found C 60.39; H 3.34; N 13.34; O 7.57; S 15.24%.
3-(2-(Benzo[4,5]thiazolo[2,3-c][1,2,4]triazol-3-yl)phenyl)-2-(thiophen-2-yl)thiazolidin-4-one (6g). Pale yellow solid, m.p. 290 °C, FT-IR (KBr, cm−1): 1700 (C
O), 689 (C–S); 1H-NMR (DMSO-d6, 400 MHz) δ: 7.68–7.73 (m, 2H, Ar–H), 7.52–7.54 (m, H, Ar–H), 7.22–7.38 (m, 5H, Ar–H), 6.69–6.71 (m, 3H, Ar–H), 6.11 (s, 1H, CH), 3.61 (d, 1H, CH2, J = 10 Hz), 3.39 (d, 1H, CH2, J = 10 Hz) ppm; 13C-NMR (DMSO-d6, 100 MHz) δ: 35.2, 64.4, 110.4, 110.6, 112.6, 115.4, 115.8, 119.5, 119.8, 121.0, 121.9, 123.7, 124.9, 126.9, 128.9, 129.8, 131.5, 143.8, 149.0, 155.3, 168.8 ppm; MS(m/z): 435.033 [M+H]+; anal. calcd for C21H14N4OS3: C 58.04; H 3.25; N 12.89; O 3.68; S 22.13% found C 58.16; H 3.22; N 12.84; O 3.60; S 22.07%.
3-(2-(Benzo[4,5]thiazolo[2,3-c][1,2,4]triazol-3-yl)phenyl)-2-(1H-indol-3-yl)thiazolidin-4-one (6h). White solid, m.p. 283 °C, FT-IR (KBr, cm−1): 1695 (C
O), 686 (C–S), 3412 (N–H); 1H-NMR (DMSO-d6, 400 MHz) δ: 10.78 (s, 1H, N–H), 7.72–7.78 (m, 3H, Ar–H), 7.52–7.60 (m, 3H, Ar–H), 7.25–7.32 (m, 2H, Ar–H), 7.14–7.17 (m, 3H, Ar–H), 6.88 (s, 1H, Ar–H), 6.74–6.76 (m, 1H, Ar–H), 6.31 (s, 1H, CH), 3.92 (d, 1H, CH2, J = 7.2 Hz), 3.86 (d, 1H, CH2, J = 7.2 Hz) ppm; 13C-NMR (DMSO-d6, 100 MHz) δ: 33.4, 72.2, 112.0, 112.3, 112.8, 114.1, 116.3, 117.5, 119.3, 121.6, 121.9, 123.0, 124.3, 126.5, 127.7, 129.8, 130.0, 132.4, 132.7, 142.1, 142.4, 149.7, 158.2, 169.0 ppm; MS(m/z): 468.087 [M+H]+; anal. calcd for C25H17N5OS2: C 64.22; H 3.66; N 14.98; O 3.42; S 13.71% found C 64.34; H 3.63; N 14.93; O 3.34; S 13.65%.
Conclusions
We have designed and synthesized two novel series of molecular hybrids (4a–l and 6a–h) by amalgamating benzothiazole, triazole, and thiazolidinone pharmacophore sub-units and were screened for antimicrobial properties. Among the tested compounds, 6e and 6h showed promising antibacterial and antifungal activity against the tested pathogenic strains compared to standard. Compounds 6e, 6g, and 6h also exhibited significant activity. The presence of pharmacologically active moieties, combined with a green protocol that operates efficiently in one pot manner under metal- and catalyst-free conditions, makes the synthesized compounds highly scalable. The evaluation results suggest that these compounds are promising candidates for further chemical modification and as biologically active agents.
Abbreviations
ACN | Acetonitrile |
ALR2 | Aldose reductase |
COX | Cyclooxygenase |
DMF | Dimethylformamide |
EtOH | Ethanol |
HIV-RT | Human immunodeficiency virus-reverse transcription |
IR | Infrared |
MeOH | Methanol |
MH | Molecular hybridization |
MIC | Minimum inhibitory concentration |
MTCC | Microbial type culture collection |
NMR | Nuclear magnetic resonance |
PARP | Poly(ADP-ribose) polymerase |
PRL | Phosphatase of a regenerating liver |
SAR | Structure–activity relationships |
SARS-COV-2 | Severe acute respiratory syndrome Coronavirus 2 |
THF | Tetrahydrofuran |
TNF | Tumor necrosis factor |
Author contributions
Deepika Choudhary: conceptualization, methodology, resources, funding, validation, software, supervision; Bhaskar Dwivedi: investigation, methodology, formal analysis, data curation, writing – review & editing; Diksha Bhardwaj: investigation, methodology, formal analysis, visualization, writing – original draft.
Conflicts of interest
There are no conflicts to declare.
Acknowledgements
The authors are grateful for the financial support of the SERB project [grant number SUR/2022/003899]. The authors thank Malaviya National Institute of Technology (MNIT) Jaipur, Banaras Hindu University (BHU), Varanasi, and Central Drug Research Institute (CDRI), Lucknow for the necessary instrument facilities.
References
- H. M. S. Kumar, L. Herrmann and S. B. Tsogoeva, Bioorg. Med. Chem. Lett., 2020, 30, 127514 CrossRef PubMed
. - S. Agarwal, D. Gandhi and P. Kalal, Lett. Org. Chem., 2017, 14, 729 CrossRef CAS
. - T. Selvaraj, R. Rajalingam and V. Balasubramanian, Appl. Surf. Sci., 2018, 434, 781 CrossRef CAS
. - C. S. W. Law and K. Y. Yeong, Expert Opin. Ther. Pat., 2022, 32, 299 CrossRef CAS PubMed
. - G. Trapani, M. Franco, A. Latrofa, A. Reho and G. Liso, Eur. J. Pharm. Sci., 2001, 14, 209 CrossRef CAS PubMed
. - T. Mase, H. Arima, K. Tomioka, T. Yamada and K. Murase, J. Med. Chem., 1986, 29, 386 CrossRef CAS PubMed
. - X. Zhang, J. Jia and C. Ma, Org. Biomol. Chem., 2012, 10, 7944 RSC
. - F. Yan, J. Sun, Y. Zang, Z. Sun, H. Zhang and X. Wang, J. Iran. Chem. Soc., 2020, 17, 3179 CrossRef CAS
. - J. Kuwabara, T. Namekawa, M.-A. Haga and T. Kanbara, Dalton Trans., 2012, 41, 44 RSC
. - V. A. Kozlov, D. V. Aleksanyan, S. G. Churusova, A. A. Spiridonov, E. Y. Rybalkina, E. I. Gutsul, S. A. Aksenova, A. A. Korlyukov, A. S. Peregudov and Z. S. Klemenkova, Int. J. Mol. Sci., 2023, 24, 17331 CrossRef CAS PubMed
. - G. M. Srinivasa, E. Jayachandran, B. Shivakumar and D. S. Rao, Orient. J. Chem., 2004, 20, 103 CAS
. - X. Q. Deng, M. X. Song, C. X. Wei, F. N. Li and Z. S. Quan, Med. Chem., 2010, 6, 313 CrossRef CAS PubMed
. - J. D. Froyd, C. J. Paget, L. R. Guse, B. A. Dreikorn and J. L. Pafford, Phytopathology, 1976, 66, 1135 CrossRef CAS
. - S. Kukreja, A. Sidhu and V. K. Sharma, Res. Chem. Intermed., 2016, 42, 8329 CrossRef CAS
. - D. Geedkar, A. Kumar and P. Sharma, J. Heterocycl. Chem., 2020, 57, 4331 CrossRef CAS
. - A. H. Abdelazeem, A. M. Alqahtani, H. A. Omar and S. N. A. Bukhari, J. Mol. Struct., 2020, 1219, 128567 CrossRef CAS
. - S. Murthy, M. G. Nizi, M. M. Maksimainen, S. Massari, J. Alaviuhkola, B. E. Lippok, C. Vagaggini, S. T. Sowa, A. Galera-Prat, Y. Ashok, H. Venkannagari, R. Prunskaite-Hyyryläinen, E. Dreassi, B. Lüscher, P. Korn, O. Tabarrini and L. Lehtiö, J. Med. Chem., 2023, 66, 1301 CrossRef CAS PubMed
. - P. A. Chawla, S. K. Wahan, M. Negi, A. Faruk and V. Chawla, J. Heterocycl. Chem., 2023, 60, 1248 CrossRef CAS
. - D. Mech, A. Kurowska and N. Trotsko, Int. J. Mol. Sci., 2021, 22, 11533 CrossRef CAS PubMed
. - J. H. Ahn, S. J. Kim, W. S. Park, S. Y. Cho, J. D. Ha, S. S. Kim, S. K. Kang, D. G. Jeong, S. K. Jung, S. H. Lee, H. M. Kim, S. K. Park, K. H. Lee, C. W. Lee, S. E. Ryu and J. K. Choi, Bioorg. Med. Chem. Lett., 2006, 16, 2996 CrossRef CAS PubMed
. - P. H. Carter, P. A. Scherle, J. A. Muckelbauer, M. E. Voss, R. Q. Liu, L. A. Thompson, A. J. Tebben, K. A. Solomon, Y. C. Lo, Z. Li, P. Strzemienski, G. Yang, N. Falahatpisheh, M. Xu, Z. Wu, N. A. Farrow, K. Ramnarayan, J. Wang, D. Rideout, V. Yalamoori, P. Domaille, D. J. Underwood, J. M. Trzaskos, S. M. Friedman, R. C. Newton and C. P. Decicco, Proc. Natl. Acad. Sci. U. S. A., 2001, 98, 11879 CrossRef CAS PubMed
. - A. Petrou, P. Eleftheriou, A. Geronikaki, M. G. Akrivou and I. Vizirianakis, Molecules, 2019, 24, 3821 CrossRef CAS PubMed
. - W. A. A. Fadalya, T. H. Zidana, N. M. Kahka, F. E. A. Mohameda, M. M. Abdelhakeema, R. G. Khalil and M. T. M. Nemr, J. Enzyme Inhib. Med. Chem., 2023, 38, 2281262 CrossRef PubMed
. - A. Petrou, P. Zagaliotis, N. F. Theodoroula, G. A. Mystridis, I. S. Vizirianakis, T. J. Walsh and A. Geronikaki, Molecules, 2022, 27, 2180 CrossRef CAS PubMed
. - A. Kousaxidis, L. Kovacikova, I. Nicolaou, M. Stefek and A. Geronikaki, Med. Chem. Res., 2021, 30, 1837 CrossRef CAS PubMed
. - S. Cascioferro, B. Parrino, D. Carbone, D. Schillaci, E. Giovannetti, G. Cirrincione and P. Diana, J. Med. Chem., 2020, 63, 7923 CrossRef CAS PubMed
. - R. A. Rane, N. U. Sahu and C. P. Shah, Bioorg. Med. Chem. Lett., 2012, 22, 7131 CrossRef CAS PubMed
. - E. Pitta, E. Tsolaki, A. Geronikaki, J. Petrovic, J. Glamočlija, M. Soković, E. Crespan, G. Maga, S. S. Bhunia and A. K. Saxena, MedChemComm, 2015, 6, 319 RSC
. - S. Khan, S. Iqbal, M. Khan, W. Rehman, M. Shah, R. Hussain, L. Rasheed, Y. Khan, A. A. Dera, R. A. Pashameah, E. Alzahrani and A.-E. Farouk, Pharmaceuticals, 2022, 15, 1164 CrossRef CAS PubMed
. - S. J. Gilani, K. Nagarajan, S. P. Dixit, M. Taleuzzaman and S. A. Khan, Arab. J. Chem., 2016, 9, S1523 CrossRef CAS
. - P. P. Prabhu, T. Panneerselvam, C. S. Shastry, A. Sivakumar and S. S. Pande, J. Saudi Chem. Soc., 2015, 19, 181 CrossRef
. - A. Piozzi and J. Lee, Int. J. Mol. Sci., 2024, 25, 325 Search PubMed
. - S. Khan, S. Iqbal, M. Khan, W. Rehman, M. Shah, R. Hussain, L. Rasheed, Y. Khan, A. A. Dera and R. A. Pashameah, Pharmaceuticals, 2022, 15, 1164 CrossRef CAS PubMed
. - D. Kaminskyy, A. Kryshchyshyn and R. Lesyk, Eur. J. Med. Chem., 2017, 10, 542 CrossRef PubMed
. - P. Roszczenko, S. Holota, O. K. Szewczyk, R. Dudchak, K. Bielawski, A. Bielawska and R. Lesyk, Int. J. Mol. Sci., 2022, 23, 13135 CrossRef CAS PubMed
. - R. Perkins, H. Fang, W. Tong and W. J. Welsh, Environ. Toxicol. Chem., 2003, 22, 1666 CrossRef CAS PubMed
. - R. Singh, A. Singh and D. Bhardwaj, ChemistrySelect, 2019, 4, 9600 CrossRef CAS
. - R. Singh, M. R. Saini, D. Bhardwaj and A. Singh, New J. Chem., 2020, 44, 7923 RSC
. - D. Bhardwaj and R. Singh, Tetrahedron Lett., 2021, 85, 153491 CrossRef CAS
. - R. Singh, A. Singh, D. Bhardwaj and S. Sharma, J. Sulfur Chem., 2024, 45, 1 CrossRef CAS
. - S. Tariq, O. Alam and M. Amir, Arch. Pharm. Chem. Life Sci., 2018, 351, e1700304 CrossRef PubMed
. - K. Vaarla, R. K. Kesharwani, K. Santosh, R. R. Vedula, S. Kotamraju and M. K. Toopurani, Bioorg. Med. Chem. Lett., 2015, 25, 5797 CrossRef CAS PubMed
. - B. Skora, A. Lewinska, A. Kryshchyshyn-Dylevych, D. Kaminskyy, R. Lesyk and K. A. Szychowski, Molecules, 2022, 27, 894 CrossRef CAS PubMed
. - M. Haroun, C. Tratrat, K. Kositzi, E. Tsolaki, A. Petrou, B. Aldhubiab, M. Attimarad, S. Harsha, A. Geronikaki, K. N. Venugopala, H. S. Elsewedy, M. Sokovic, J. Glamoclija and A. Ciric, Curr. Top. Med. Chem., 2018, 18, 75 CrossRef CAS PubMed
. - C. Tratrat, A. Petrou, A. Geronikaki, M. Ivanov, M. Kostic, M. Sokovic, I. S. Vizirianakis, N. F. Theodoroula and M. Haroun, Molecules, 2022, 27, 1930 CrossRef CAS PubMed
.
|
This journal is © The Royal Society of Chemistry 2024 |
Click here to see how this site uses Cookies. View our privacy policy here.