DOI:
10.1039/D4RA01534G
(Paper)
RSC Adv., 2024,
14, 16432-16444
An environmentally sustainable approach for the utilization of Erigeron bonariensis as a green inhibitor against weathering steel corrosion in acidic media
Received
28th February 2024
, Accepted 3rd May 2024
First published on 21st May 2024
Abstract
In the realm of corrosion mitigation, the search for sustainable and ecologically accountable inhibitors attracts significant interest from the environmental point of view. This study investigates the intriguing possibilities presented by Erigeron bonariensis (EB) as a green and innovative corrosion inhibitor for weathering steel in 1 M H2SO4. EB, a naturally abundant plant species, holds promise as a green and sustainable inhibitor due to its inherent chemical composition in the environment. The intricate interplay between the phytochemical constituents of the extract and the corrosive environment is meticulously deciphered. Furthermore, the environmentally benign nature of the inhibitor adds an extra layer of significance to its application, aligning with contemporary green chemistry principles. The inhibition effect of Erigeron bonariensis (EB) extract on the corrosion of mild steel in acidic media (H2SO4) was studied using weight loss, absorption studies, phytochemical analysis, electrochemical methods, and scanning electron microscopy. The experimental findings revealed that an increase in inhibitor concentration is correlated with higher inhibition efficiency. The adsorption of inhibitor molecules on the mild steel surface was found to agree with the UV-Vis adsorption spectrum. Additionally, a surface study conducted using scanning electron microscopy indicated notable differences in the presence and absence of inhibitors for weathering steel. At 2000 mg L−1, EB extract has the best inhibitory efficiency for weathering steel in 1 M H2SO4 of 99.50% by the leaf part, followed by 94.35% by the flower part, and 85.22% by the stem part. Overall, this study suggests that EB extract serves as a promising alternative for corrosion prevention, demonstrating significant inhibition efficiency.
Introduction
Weathering steel is widely used in various hardware and structural projects owing to its favourable mechanical characteristics and reduced need for manual labour in environmental remediation. However, the industrial process of acid pickling weathering steel involves potent acids to eliminate impurities and unwanted surface deposits, which can lead to substantial economic drawbacks.1,2 In order to mitigate the aggressive nature of acidic solutions, inhibitors are commonly employed to delay the corrosive effects on metal objects that directly affect circular economy. While numerous industrial inhibitors have been developed and effectively deployed to counteract steel corrosion in acidic environments,2 a significant drawback of many of these inhibitors lies in their lack of environmental friendliness, often stemming from their toxicity and high cost.3
To counteract the aggressive nature of acidic solutions used in pickling, inhibitors are commonly added. These inhibitors help delay the corrosive effects on metal objects, thus contributing to the circular economy. While many industrial inhibitors have been developed and deployed effectively to combat steel corrosion in acidic environments, they often lack environmental friendliness.4 This deficiency stems from their toxicity and high cost. Addressing this challenge has led to a critical need for new corrosion inhibitors that are either non-toxic or have low toxicity levels. The focus has been on identifying economically viable and highly effective compounds that have minimal adverse impacts on the environment.5 However, the use of synthetic compounds in corrosion inhibition may pose potential environmental and public health risks. Therefore, there is a growing emphasis on the search for economical, environmentally benign, and biodegradable corrosion inhibitors, which has garnered increased attention in recent years.4
Addressing this challenge has made it crucial to seek out new corrosion inhibitors that are either non-toxic or have low toxicity levels. The focus on developing environment-friendly corrosion inhibitors has been on finding economically viable and highly effective compounds that have little to no adverse impact on the environment. However, the utilization of synthetic compounds may carry potential environmental and public health risks. Hence, there is a growing emphasis on the quest for economical, ecologically benign, and biodegradable corrosion inhibitors,6 which has gained heightened attention in recent times. Quercetin, rutin, naringenin, luteolin, caffeic acid, apigenin, stigmasterol, and baicalein are the prime constituents present in various parts of EB. The structural representation of all major phytochemicals is mentioned in Fig. 1.
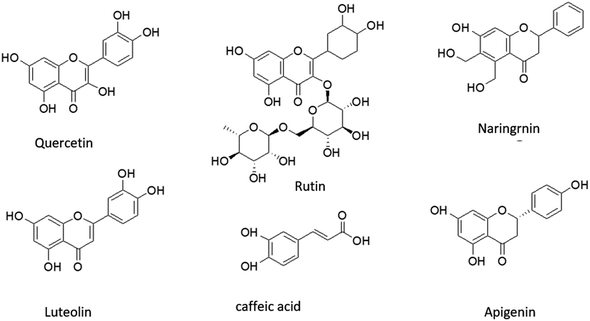 |
| Fig. 1 Major phytochemicals present in Erigeron bonariensis. | |
Weathering steel is a popular choice for hardware and structural projects due to its favorable mechanical properties and its ability to undergo environmental remediation with minimal manual labor. However, the industrial process of acid pickling of weathering steel involves the use of strong acids to remove impurities and unwanted surface deposits. Unfortunately, this process can result in significant economic drawbacks.
Since the 1940s, inhibitors have been instrumental in reducing corrosion rates without substantially altering the concentration of any corrosive agent. Organic compounds comprising nitrogen atoms, including imidazolines, amides, amidoamines, amines, and their salts have proven to be effective as corrosion inhibitors in pipelines transporting hydrocarbons with CO2 in the oil and gas industry. The efficacy of an inhibitor in corrosion control hinges on various factors, including its formulation, chemical structure, affinity for binding to particular metal surfaces, and the environmental conditions in which it is applied.7
Several inhibitors commercially available for corrosion protection include zinc phosphate coatings, which are effective for steel surfaces and provide excellent corrosion resistance by forming dense phosphate layers, according to Vizureanu et al. (2020).8 Sodium molybdate, a molybdate inhibitor, commonly used for cooling water systems and boilers, forms protective layers inhibiting corrosion, as reported by Ali et al. (2009).9 These inhibitors are commercially available and play essential roles in corrosion prevention across various industrial applications.
Given the importance of acid pickling of weathering steel and the need for environment-friendly corrosion inhibitors, further exploration and development in this area are warranted. Expanding on the discussion of acid pickling and the challenges associated with it will provide readers with a comprehensive understanding of the context and significance of the research presented in the manuscript.
However, a prominent drawback of most of these inhibitors lies in their lack of ecological compatibility, coupled with their toxic nature and high costs. To tackle this challenge, the exploration of novel non-toxic or low-toxic corrosion inhibitors has become an imperative pursuit. The quest for environment-friendly corrosion inhibitors has primarily focused on identifying cost-effective, efficient compounds that exert minimal or negligible impact on the environment.10 However, the use of synthetic substances may potentially exert profound adverse effects on the ecosystem and pose risks to public health. This has fuelled a recent surge in the emphasis on seeking corrosion inhibitors that are not only economically viable but also environmentally benign and biodegradable.6
The core objective of this current study revolves around examining the adsorption characteristics and conducting corrosion inhibition assessments of EB extract.1,2 Extensive research is being conducted on this plant, which originates from Asia and goes by multiple names such as flax-leaf fleabane, wavy-leaf fleabane, Argentine fleabane, hairy horseweed, and asthma weed. The elements of EB were isolated one by one and then improved to assess their capability to inhibit corrosion in weathering steel within an acidic environment.6 Till now, EB plant has not been explored as an anti-corrosive material and we obtained excellent results on weathering steel in acidic media. Additionally, we studied different parts of EB plant to acknowledge their efficiency variation. Detailed discussions encompass the mechanism and efficacy of the inhibition process. Furthermore, the surface characteristics of both the weathering steel and the extract were closely examined using scanning electron microscopy (SEM).11
Experimental
Preparation and extraction process of the plant
The EB plant was collected from a dense farm situated in the area. Fresh and green EB was washed in tap water to remove any flammable mud residue, dried for almost one week in a dry shelter; oven drying was avoided because of plant degradation. Afterwards, the stem, leaves and flower were separated individually and then crushed into powder using a grinder.12 The end of the Soxhlet apparatus was plugged with cotton and an even cotton bed was prepared afterwards. The Soxhlet apparatus was filled with separately ground dried plant parts and again a cotton bed was made on top to avoid the drainage of the ground plant.13 The extraction solvent, i.e., ethanol, was added into a round bottom flask, ensuring the level in the RB, and boiling chips were added to avoid bumping. Then, the RB was connected to the Soxhlet apparatus and put on a heating plate. The condenser was assembled and attached to the already connected system.14 This system was allowed to run properly for two days until the colourless liquid was drained out. Then, the solution (extract + solvent) in the RB was collected by reassembling the system. In the next step, the solvent was required to extract from the solution in order to get the crude plant extract.15 450 mL of ethanol and 100 g of powdered material were refluxed continuously for 3 hours at 75 °C. The pH level of the liquid solution following the reflux process was found to be 9 after filtration.16 The filtrate was condensed to 100 cc of a dark greenish-black residue, which was then subjected to two days of drying at 60 °C in a vacuum drying oven.13 The dark greenish-black raw residue, weighing approximately 4.5 grams, was subsequently obtained, and ensured to be completely dry before being stored in a desiccator; the detailed methodology is given in Fig. 2. Test solutions with predetermined concentrations (25, 50, 75, and 100 mg L−1) were prepared by diluting the extract with acidic media. Among these concentrations, the 100 mg L−1 solution of EB extract exhibited the greatest level of solubility in acidic media.11
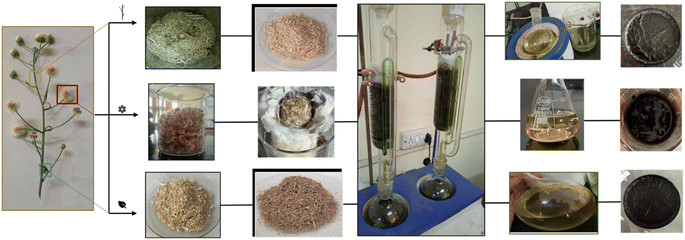 |
| Fig. 2 Extraction methodology used from the plant to the crude form. | |
Weight loss analysis
In this research, the composition of weathering steel is described as follows: it contains 97.50% iron (Fe), 0.083% carbon (C), 0.12% phosphorus (P), 0.45% chromium (Cr), 0.27% nickel (Ni), and 0.43% copper (Cu). To conduct the weight loss experiment, the specimens were cut into dimensions measuring 1 square centimetre on each edge.2 In corrosion studies, weight loss is often used as a common and straightforward method to assess the corrosion rate of a material.17
Before being inserted into the freshly prepared test solution, these chopped weathering steel pieces13 were refined with emery paper of different grades, i.e., 100, 200, 500, 1000, and 1500, the samples were cleaned with acetone to remove any grease and then rinsed with distilled water. Sulfuric acid was diluted to create a corrosive solution with a certain concentration of the acidic medium. The basic representation of weight loss at different ppm values is given in Fig. 3.
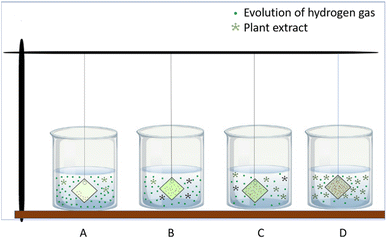 |
| Fig. 3 Representing weight loss analysis with increasing concentrations. (A)–(D) Represents the plant extract at 500, 1000, 1500, 2000 ppm, respectively. | |
Adsorption studies
In the field of plant corrosion, adsorption studies show a vital character in understanding and mitigating the detrimental effects of corrosion on industrial equipment and infrastructure.18 This phenomenon has significant implications for corrosion because it can either enhance or inhibit the corrosion process.3 By analysing the adsorption characteristics, scientists can gain insights into the protective properties of different materials and develop effective corrosion prevention strategies.19 These studies involve subjecting metallic samples to controlled environments that simulate real-world corrosion conditions, such as exposure to aggressive chemicals, elevated temperatures, and humidity.20
Through adsorption studies, researchers can identify the types of corrosive species that are most likely to adhere to the metal surface, understand the kinetics and thermodynamics of the adsorption process, and determine the factors influencing adsorption, such as pH, temperature, and surface roughness.1,21 Overall, adsorption studies in plant corrosion provide valuable insights into the fundamental mechanisms of corrosion and aid in the development of effective strategies to mitigate corrosion-related damage in industrial settings.22 By understanding the adsorption behaviour of corrosive substances, researchers can work towards improving the durability, reliability, and safety of plant infrastructure.23
Phytochemical analysis
Photochemical analysis is a branch of analytical chemistry that focuses on studying the chemical changes that occur in substances when they are exposed to light.2 It entails the application of a range of spectroscopic methods to detect and measure the outcomes and quantities of photochemical reactions.24 Erigeron bonariensis, commonly known as the Fleabane daisy or Buenos Aires fleabane, is a flowering plant native to South America.25 As for the phytochemical analysis of EB, specific composition of photochemical compounds in EB may vary depending on factors such as geographical location, environmental conditions, and plant growth stage.26
UV spectroscopy
UV spectroscopy is widely used for the quantitative analysis of compounds, such as determining the concentration of an analyte. Another useful application of UV spectroscopy is that it can be employed to study reaction kinetics by monitoring changes in absorbance over time. It allows researchers to investigate reaction rates, mechanisms, and the effect of different factors on the reaction progress. UV spectroscopy is utilized to determine the concentration and structural characteristics of proteins and nucleic acids. It helps in assessing the purity, secondary structure, and stability of biomolecules. UV spectroscopy is an adaptable and extensively used technique due to its simplicity, rapidity, and sensitivity. It enables researchers to gain insights into the electronic properties and behaviour of various substances, contributing to a wide range of scientific investigations and applications.
Electrochemical characterization
Electrochemical technique was employed to investigate the redox behaviour of chemical species in solution. When a working electrode is immersed in an electrolyte solution and a voltage is applied, redox reactions occur at the electrode's surface, leading to the flow of electrons and the generation of a current.27 This current is then measured as a function of the applied potential. The working electrode was made using the weathering steel rod, and the outer covering was provided with the help of Araldite standard epoxy adhesive, as shown in Fig. 4.
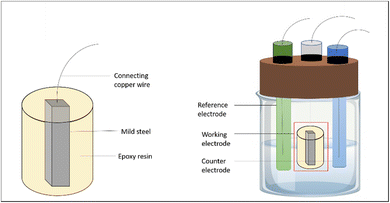 |
| Fig. 4 Graphical representation of electrodes used in electrochemical analysis. | |
The electrochemical analysis of the weathering steel sample in 1 M acidic media, both with and without the EB inhibitor, was carried out. The potential was swept from a negative to a positive value and back in a triangular waveform.
Anodic Peak: In the absence of an inhibitor, an anodic peak corresponding to the oxidation of iron occurred. The current associated with this peak provides information about the corrosion rate of weathering steel in the acidic environment.
Inhibitor Effect: In the presence of the EB inhibitor, changes in the anodic peak were observed. The inhibitor molecules adsorb on the weathering steel surface, reducing the corrosion rate by forming a protective layer.
Peak Shift and Current Reduction: The inhibitor may cause a shift in the anodic peak potential to more positive values and a reduction in the peak current, indicating its effectiveness in inhibiting the corrosion process.
The data obtained from the EIS experiments can be used to calculate important corrosion parameters, such as corrosion current density and corrosion rate, both with and without the inhibitor. The inhibition efficiency can be determined by comparing these values. Electrochemical analysis (PDP and EIS) of the steel coupons was performed with a Metrohm Autolab electrochemical analyser. There were three electrodes connected in the corrosion cell: a working electrode (steel), a reference electrode (saturated calomel), and a counter electrode (platinum). The steel was coated with Araldite resin, leaving a 1 cm2 active region exposed for reaction. Current–potential curves were obtained by varying the electrode potential between −250 mV and +250 mV with the open circuit potential.
Scanning electron microscopic study
SEM analysis can reveal details about the surface features, such as the shape, size, and texture of the sample. For EB, SEM analysis could potentially show the morphology of its leaves, flowers, or other plant parts, including the arrangement of trichomes (hairs) or other surface structures. Additionally, SEM analysis can be combined with EDS to determine the elemental composition of the sample. EDS allows for the identification and mapping of elements present in the sample by measuring the characteristic X-rays emitted when the sample is bombarded with the electron beam. This information can provide insights into the chemical composition and elemental distribution within EB. SEM analysis is a technique used to obtain the high-resolution images of the surface of a sample. It allows for the observation of the sample's topography and morphology from the micro-to nanoscale level.
SEM analysis can be useful for studying the flower structure, including the petals, reproductive organs (such as the pistil and stamens), and pollen grains. This information can aid in the identification and classification of the species and provide insights into the plant's reproductive biology.
Surface interactions: SEM analysis can be used to investigate the interaction of EB with other plant extracts. This examination of surface structures provides insights into potential symbiotic or pathogenic relationships.
Results and discussion
Weight loss analysis
Firstly, the prepared specimens of the weathering steel material were studied typically in the form of flat coupons or cylinders. The specimens should have a known initial weight. Now, the specimens were exposed to the corrosive environment of interest.6 This can be done by immersing them in a corrosive solution, exposing them to gases, or subjecting them to other relevant environmental conditions.6 The specimens (chopped steel chips) were kept in a corrosive environment for a specific period, here, 24 and 48 hours. The duration can range from hours to months, depending on the research goals.28 After the exposure period, the specimens were removed from the corrosive environment and cleaned thoroughly to remove any corrosion products or contaminants. Then, they were dried, and their final weight was measured using a weight balance with sufficient precision.29 Weight loss is assessed by computing the variance between the initial weight (W0) and the concluding weight (W1).24,30 The corrosion rate is subsequently computed by dividing the weight loss by both the duration of exposure and the specimen's surface area.24 Typically, the corrosion rate is expressed in terms of the amount of mass lost per unit area over a specific period, such as millimeters per year (mm per year) or grams per square meter per day (g per m2 per day).
By monitoring the weight loss and calculating the corrosion rate, researchers can assess the effectiveness of different materials, coatings, or corrosion inhibitors in preventing or reducing corrosion.31 Weight loss measurements are just one of the several methods used in corrosion research. Other techniques such as electrochemical measurements, surface analysis, and microscopy are often employed to provide a more comprehensive understanding of corrosion processes.32,33
|
 | (1) |
|
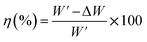 | (2) |
W′, Δ
W,
θ and
η represents the weight of chopped weathering steel plate immersed in acidic media, difference between the weight of chopped before and after immersion in prepared extract acidic media surface coverage and efficiency of inhibition for the weight loss analysis. The data recording during the weight loss of various parts like leaves, stem and flower is given in
Tables 1–3 with every fine detail like surface coverage (
θ) and efficiency (
η). The number in the subscript explains the duration of exposure to the media, for example, 1 represents the duration of 24 hours and 2 represents the date according to 48 hours. 0 as subscript represents the initial conditions of chopped weathering steel chips weight.
Table 1 Compiled weight loss data on the leaves extract of EB plant
ppm |
W1 (W0 − W1) |
W2 (W0 − W2) |
θ1 |
θ2 |
η1 |
η2 |
0 |
3.419 |
3.719 |
00 |
00 |
00 |
00 |
500 |
0.676 |
2.069 |
0.8022 |
0.3948 |
80.22 |
39.48 |
1000 |
0.138 |
0.632 |
0.9596 |
0.8151 |
95.96 |
81.51 |
1500 |
0.114 |
0.522 |
0.9665 |
0.8473 |
96.65 |
84.73 |
2000 |
0.017 |
0.225 |
0.9950 |
0.9341 |
99.50 |
93.41 |
Table 2 Compiled weight loss data on the stem extract of EB plant
ppm |
W1 (W0 − W1) |
W2 (W0 − W2) |
θ1 |
θ2 |
η1 |
η2 |
0 |
3.419 |
3.719 |
00 |
00 |
00 |
00 |
500 |
0.832 |
1.567 |
0.7566 |
0.5416 |
75.66 |
54.16 |
1000 |
0.684 |
1.426 |
0.7999 |
0.5829 |
79.99 |
58.29 |
1500 |
0.526 |
1.502 |
0.8461 |
0.5610 |
84.61 |
56.10 |
2000 |
0.505 |
0.345 |
0.8522 |
0.8996 |
85.22 |
89.96 |
Table 3 Compiled weight loss data on the flower extract of EB plant
ppm |
W1 (W0 − W1) |
W2 (W0 − W2) |
θ1 |
θ2 |
η1 |
η2 |
0 |
3.419 |
3.791 |
00 |
00 |
00 |
00 |
500 |
0.742 |
1.428 |
0.7829 |
0.5823 |
78.29 |
58.23 |
1000 |
0.409 |
0.919 |
0.8803 |
0.7312 |
88.03 |
73.12 |
1500 |
0.279 |
0.671 |
0.9183 |
0.8037 |
91.83 |
80.37 |
2000 |
0.193 |
0.530 |
0.9435 |
0.8449 |
94.35 |
84.49 |
θ1 and η1 are the surface coverage and efficiency after 24 hours at different ppm values. Similarly, θ2 and η2 are the surface coverage and efficiency after 48 hours at different ppm values. The effectiveness of corrosion inhibition, as well as the corrosion rate and surface coverage, were examined through the weight loss technique for extracts from different parts of EB with different concentrations (ranging from 0 to 2000 ppm), and the findings are detailed in Table 1. It is evident from Table 1 that an increase in inhibitor concentration leads to a clear improvement in the inhibition efficiency. This improvement occurs because when the inhibitor attaches itself to the metal surface, it creates a protective layer that diminishes the corrosion process. The comparison efficiency chart mentions different parts of EB plant in Fig. 5.
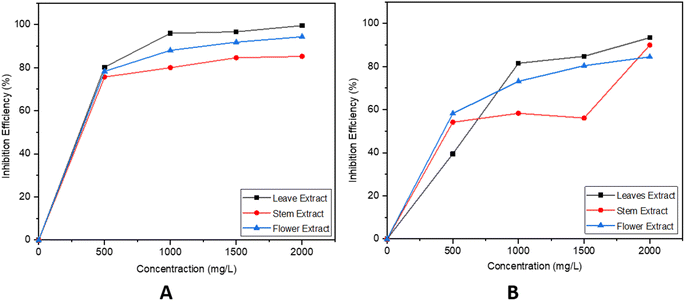 |
| Fig. 5 (A and B) Represent the variation in the inhibition efficiency of leaves, stem and flower at different concentrations for 24 and 48 hours, respectively. | |
Phytochemical analysis
Various tests were formed for the detection of different types of natural products such as alkaloid, flavonoid, tannin, saponin, phenol, terpenoids, amino acids, carbohydrates and glycosides in different solvents. Mayer's, Wagner's and Hager's test were used for the detection of alkaloids, and Salkowaski's test was performed for the detection of terpenoids and steroidal. Froth's test and lead acetate test were performed for saponins and tannins, repetitively. The presence and absence of natural products was tested on the basis of the above-mentioned tests, and the details are mentioned in Tables 4–6 for their parts like stem, leaves and flowers, respectively.
Table 4 Phytochemical scrutiny of the stem of EB plant
Test |
Aqueous |
Acetone |
Methanol |
Alkaloid |
Negative |
Negative |
Negative |
Tannin |
Positive |
Positive |
Positive |
Saponin |
Negative |
Negative |
Negative |
Flavonoid |
Positive |
Positive |
Positive |
Phenol |
Positive |
Positive |
Positive |
Terpenoids |
Negative |
Negative |
Negative |
Amino acid |
Negative |
Negative |
Negative |
Carbohydrates |
Negative |
Negative |
Negative |
Glycoside |
Positive |
Positive |
Positive |
Table 5 Phytochemical scrutiny of the leaves of EB plant
Test |
Aqueous |
Acetone |
Methanol |
Alkaloid |
Negative |
Negative |
Negative |
Tannin |
Positive |
Positive |
Positive |
Saponin |
Negative |
Negative |
Negative |
Flavonoid |
Positive |
Negative |
Negative |
Phenol |
Positive |
Positive |
Positive |
Terpenoids |
Positive |
Negative |
Negative |
Amino acid |
Negative |
Negative |
Negative |
Carbohydrates |
Negative |
Negative |
Negative |
Glycoside |
Negative |
Negative |
Positive |
Table 6 Phytochemical scrutiny of the flower of EB plant
Test |
Aqueous |
Acetone |
Methanol |
Alkaloid |
Negative |
Negative |
Negative |
Tannin |
Positive |
Positive |
Positive |
Saponin |
Negative |
Negative |
Negative |
Flavonoid |
Positive |
Negative |
Negative |
Phenol |
Positive |
Positive |
Positive |
Terpenoids |
Negative |
Negative |
Negative |
Amino acid |
Negative |
Negative |
Negative |
Carbohydrates |
Negative |
Negative |
Negative |
Glycoside |
Negative |
Negative |
Positive |
UV spectroscopy
The UV spectra of EB extract (leaves) were analysed both before and after the corrosion test, and their representations are displayed in Fig. 6. Upon examining the UV spectra, it becomes evident that the absorbance of the corrosive medium, in which the low carbon steel sample was not immersed, is higher compared to the scenario where the steel sample was soaked for 24 hours. Clearly, it is evident that when the low carbon steel sample was immersed in the acidic EB extract solution, certain molecules from the solution adhered to the metal surface. This is indicated by alterations in the absorption maximum value (λmax) or changes in absorbance, suggesting the creation of a complex between the steel surface and inhibitor molecules.27
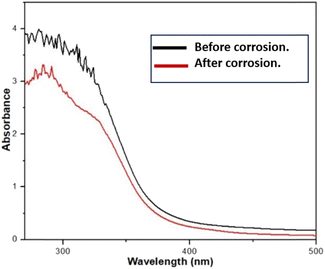 |
| Fig. 6 UV spectra of the EB extract before and after the corrosion test. | |
Electrochemical characterization
In the potentiodynamic polarization (PDP) study using the corrosive solvent with various extract concentrations, Fig. 7 clearly demonstrates the anodic and cathodic polarisation interaction of steel coupons, and Table 1 enlists the related corrosion measurements and inhibition efficiencies. The specification of the instrument used is Company Metrohm, Model-PGSTAT101, software-NOVA 2. The scan rate of PDP according to the Tafel curves is 0.001 V s−1. The anodic and cathodic section current density decreases in the presence of the EB extract. Open current potential was performed for 45 minutes before polarization. This behaviour demonstrated that the inhibitor may stop the cathodic hydrogen evolution as well as anodic metal oxidation. The anodic as well as cathodic Tafel graphs (βa and βc) with the EB extract were changed with certain concentrations, which show that the inhibitor affects the cathode hydrogen gas evolution and interferes with the anode iron dissolving process.34 Thus, in a solution of acidic media, the EB extract regulates both cathodic and anodic corrosion reactions. This should indicate that adding more EB extract prevents the steel corrosion process. According to Table 1, the corrosion current density reduces as the EB extract concentration increases. At a concentration of 2000 mg L−1, the lowest corrosion current density was 2.7 × 10−4 A cm−2 and the best inhibition efficiency of 90% was observed. In addition, Table 7 demonstrates a decline in the corrosion rate in the presence of the extract; all this is due to the adsorption capacity of the extract on the surface of the metal, which provides the resistance against metal dissolution.
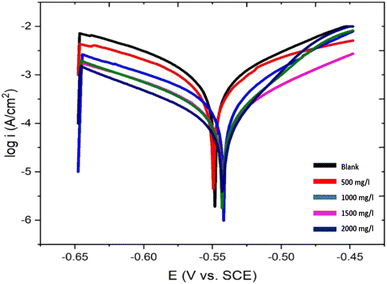 |
| Fig. 7 Tafel polarization curves for weathering steel in acidic media without and with different concentrations of EB leaves extract. | |
Table 7 Polarization parameters both with and without the EB extract at various concentrations
Extract concentration (mg L−1) |
Ecorr (V vs. SCE) |
Icorr (A cm−2) |
βa (V dec−1) |
βc (V dec−1) |
Corrosion rate |
Efficiency (η%) |
0 |
−0.807 |
2.9 × 10−3 |
0.08537 |
0.06263 |
34.03 |
0 |
500 |
−0.796 |
7.36 × 10−4 |
0.1684 |
0.0826 |
8.26 |
74.62 |
1000 |
−0.549 |
4.60 × 10−4 |
0.0997 |
0.08348 |
5.20 |
84.13 |
1500 |
−0.429 |
4.0 × 10−4 |
0.1463 |
0.1088 |
4.24 |
86.20 |
2000 |
−0.528 |
2.7 × 10−4 |
0.1300 |
0.0974 |
1.24 |
90.68 |
The following relationship is used to calculate efficiency
|
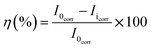 | (3) |
where
Iicorr and
I0corr are the corrosion current density values with and without plant extract, respectively.
33
The parameters, including those in Table 7, such as corrosion potential (Ecorr), corrosion current density (Icorr), anodic and cathodic Tafel slope (βa and βc) and inhibition efficiency (η%), were calculated by eqn (3).
The corrosion inhibition efficiency of the steel plate under the inhibitor may vary with surface roughness or porosity. Higher porosity can provide more sites for inhibitor adsorption, potentially enhancing the inhibition efficiency. The surface porosity percentage fraction was determined using PDP (Tafel polarization) data. The equation used to calculate the porosity percentage (PR%) in this scenario is as follows.
where
PR represents the total porosity, while
R0P and
RP denote the polarization resistance of the uninhibited and inhibited substrates, respectively.
Fig. 8 displays the change in porosity percentage with increasing EB extract concentration for the investigated steel at 25 °C. The data illustrates a significant decrease in porosity percentage as the concentration of inhibitor increases. As a result, the corrosion resistance is enhanced in the inhibited substrates compared to the uninhibited ones. This observed trend is further supported by the data obtained from Tafel plot measurements.
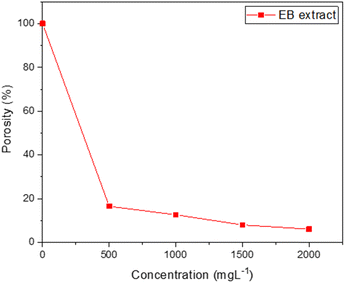 |
| Fig. 8 Porosity percentage as a function of EB extract. | |
The electrochemical characterization of EB as an eco-friendly inhibitor against weathering steel corrosion in acidic solution provides valuable insights into its inhibitive properties.35 The results suggest that EB extract has the potential to effectively reduce the corrosion rate of weathering steel, making it a promising candidate for eco-friendly corrosion inhibition in acidic environments. Further studies, including long-term immersion tests and real-world applications, are warranted to validate its practical utility. The Tafel polarization curves for weathering steel in acidic media without and with different concentrations of EB leaves extract is explained in Fig. 7 analyses of the electrochemical studies.
Electrochemical impedance spectroscopy
To investigate the impedance parameters of mild steel samples in 1 M H2SO4 with varying concentrations of EB inhibitor, the electrochemical work station PGSTAT204 with FRA32M Module was used to analyze the electrochemical aspects of weathering steel corrosion with the help of a three-electrode cell assembly with weathering steel coupons (1 cm × 1 cm × 4 cm) as the working electrode, saturated calomel electrode (SCE) as the reference electrode, and a large area platinum mesh as the counter electrode. Electrochemical impedance spectroscopy (EIS) measurements were conducted at 27 ± 1 °C. Electrochemical impedance spectroscopy was carried out at open circuit potential (OCP) (constant OCP to achieve accuracy) in the frequency range from 0.1 Hz to 100 kHz at 5 mV amplitude. The EIS curves, including the Nyquist and Bode plots, for mild steel with and without EB extract are shown in Fig. 10(A) and (B) and 11. A stable potential (vs. SCE) corresponding to the free corrosion potential (Ecorr vs. SCE) of mild steel was promptly achieved. In the Nyquist plot, the semicircle in each curve, attributed to charge transfer resistance, represents a time constant. Increasing the concentration of EB extract enlarges the diameter of the capacitive loop, indicating an enhancement of the inhibition effect.36 In the EIS curves of the blank solution, inductive loops exist at low frequency, resulting from the relaxation of adsorbed intermediate products from the steel surface, and the relevant circuit model is given in Fig. 9. For the other concentrations, the disappearance of the inductive loop is considered a “degradation” phenomenon for EIS. In the Bode plot, the variation trend of impedance value with EB extract concentration is consistent with the semicircle diameter in the Nyquist plot (Fig. 11). All phase angle–frequency curves show a single wave, confirming the one-time constant obtained by the Nyquist plot.
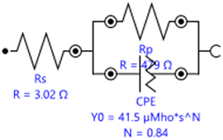 |
| Fig. 9 Equivalent circuit model adapted to fit the impedance data. | |
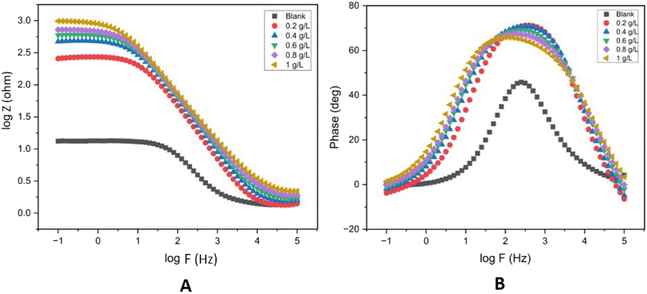 |
| Fig. 10 Bode plots (A and B) for mild steel in 1 M H2SO4 with 0–1 g L−1 concentration of EB extract at OCP at room temperature (27 ± 1 °C). | |
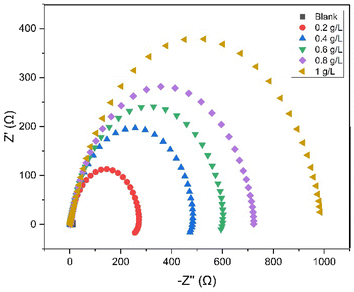 |
| Fig. 11 Nyquist plots for mild steel in 1 M H2SO4 with 0–1 g L−1 concentrations of EB extract at OCP at room temperature (27 ± 1 °C). | |
EB extract, known for its high levels of phenolic compounds, can adsorb onto the steel surface, forming a protective coat that inhibits corrosion by obstructing the transfer of charges and ions. The results clearly demonstrate that EB inhibitor effectively inhibits the corrosion of mild steel at any concentration used, and increasing the inhibitor concentration enhances the inhibition effectiveness.
Scanning electron microscopy
Fig. 12 represents the effect of corrosion with or without inhibitor, and in Fig. 13, the SEM images illustrating non-corroded, corroded, and inhibited low carbon steel coupons are presented. As described in the experimental section, we conducted a comparative analysis of these SEM images. The surface of the non-corroded weathering steel appears to be in pristine condition, whereas we observed significant damage on the surface of the corroded weathering steel. This damage occurred due to the immersion of low carbon steel in the corrosive medium, leading to surface corrosion.37 In contrast, for the inhibited steel coupon, the surface displays notable improvement owing to the adsorption of the inhibitor.
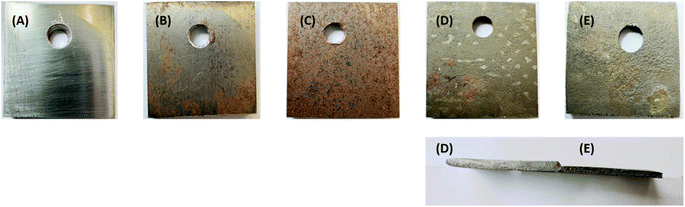 |
| Fig. 12 Representation of steel coupons before and after corrosion. (A) Represents steel coupon before corrosion, (B and C) represents steel coupons with or without plant extract and placed open in a moist environment for 2 months, respectively. (D and E) Show the thickness of coupons in acid with or without plant extract for the corrosion test, respectively. | |
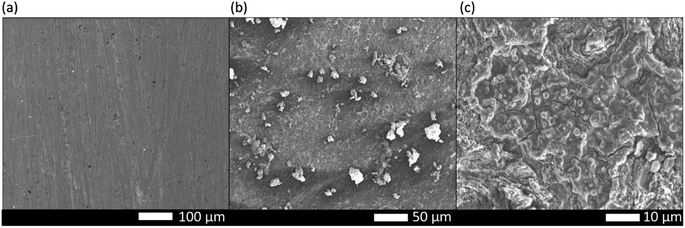 |
| Fig. 13 SEM images of polished weathering steel under different conditions: (a) weathering steel immersed in acidic media without plant extract for 24 hours; (b) weathering steel immersed in acidic media for 24 hours; and (c) weathering steel immersed in acidic media in the presence of EB extract for 48 hours. | |
Fig. 13 depicts the surface morphology of weathering steel in an acidic solution both without and with an EB inhibitor. Fig. 13 shows a SEM picture of polished weathering steel, Fig. 13(a) shows a weathering steel sample that has been submerged in acidic media for 24 hours, and Fig. 13(b) shows a weathering steel specimen that has been submerged in acidic media while being exposed to EB extract for 24 hours. Fig. 13(c) shows a weathering steel specimen that has been submerged in acidic media while being exposed to EB extract for 48 hours. The surface of weathering steel is damaged by corrosion because of its extremely rough morphology when submerged in acidic solution in the absence of an inhibitor, but the presence of EB extract causes less surface damage than the absence of the extract.38
Proposed mechanism
On the foundation of molecular adsorption, the corrosion prevention of weathering steel by the extract from EB in acidic solution may be explained. The investigated inhibitor could have attached itself to the weathering steel surface through one or more of the subsequent mechanisms.
(a) Formation of donor–acceptor interactions between the d-orbitals of iron atoms on the surface and the electrons present in aromatic rings of the inhibitor.
(b) Establishment of interactions between the vacant d-orbital of surface iron atoms and the heteroatoms of the inhibitor possessing unshared electron pairs.
Detailed mechanistic illustration is given in Fig. 14. In a broader context, there are two plausible ways in which the inhibitor could adsorb onto the steel surface.39 One approach involves the inhibitor displacing water molecules from the steel surface and engaging in electron sharing between the inhibitor's heteroatoms and the iron atoms within the steel. The expulsion of water molecules from the steel surface and the subsequent sharing of electrons between the inhibitor's heteroatoms and the iron atoms in the steel are two distinct processes that contribute to the inhibitor's adsorption onto the steel surface.
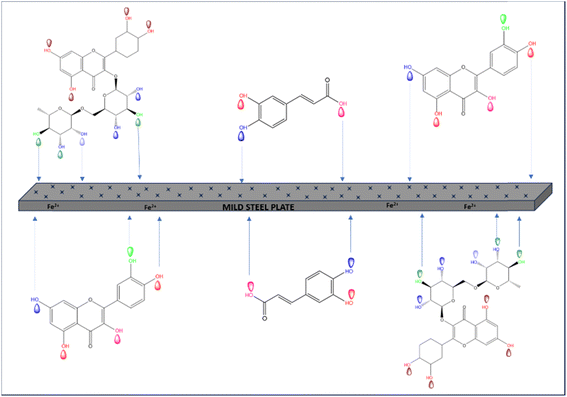 |
| Fig. 14 Mechanism of corrosion inhibition. | |
Conclusion and future prospectives
The extract derived from different parts of the EB plant demonstrates effective corrosion inhibition properties for weathering steel in an acidic solution, even at very low concentrations. Analysis using PDP indicates that the inhibitor likely functions in a combined inhibitory manner. According to electrochemical measurements, the inhibitor displays a remarkable inhibition efficiency of 99% at an inhibitor concentration of 2000 mg L−1. Furthermore, increasing the inhibitor concentration is correlated with an enhanced inhibition efficiency. SEM and weight loss analyses collectively suggest that the corrosion inhibition mechanism primarily involves adsorption. The findings strongly suggest that the EB extract functions as a mixed-type inhibitor in the environment.
Certainly, there are some potential future directions and avenues of exploration based on the general concept and methodology of utilizing EB as a corrosion inhibitor in an acidic environment.
Investigating the impact of different extraction methods, solvent types, and concentrations on the inhibitory efficiency of EB could involve the following. (1) Exploring various solvents and extraction parameters to enhance the concentration of active corrosion-inhibiting compounds. (2) Exploring the possibility of combining the EB extract with other natural inhibitors or traditional inhibitors to enhance the overall corrosion protection. (3) Investigating if these combinations yield synergistic effects that result in greater inhibition efficiencies. (4) Extending the exposure time of corrosion tests to evaluate the long-term effectiveness of the EB extract could provide insights into the durability and stability of the inhibition over extended periods. (5) Transitioning from controlled laboratory environments to real-world scenarios, such as industrial settings. (6) Testing the inhibitor on actual metal structures exposed to corrosive conditions to assess its practical effectiveness. (7) Investigating the compatibility of the EB extract with other materials that might be present in the industrial processes where corrosion inhibition is needed. (8) Ensuring that the inhibitor does not interfere with the desired properties of other materials. (9) Diving deeper into understanding the specific mechanisms by which the EB extract interacts with the metal surface and inhibits corrosion, which could involve advanced spectroscopic techniques and computational modelling. (10) Conducting a comprehensive assessment of the environmental impact of using EB extract as a corrosion inhibitor. (11) Considering factors such as the scalability of extraction, biodegradability, and ecological implications. (12) Performing field trials where the inhibitor is applied to actual structures exposed to corrosive environments. (13) Regularly monitoring and evaluating the performance of the inhibitor under real-world conditions. By pursuing these future directions, researchers can deepen their understanding of EB as a corrosion inhibitor and work towards its practical application in diverse industrial settings.
Conflicts of interest
The authors declare no conflict of interest.
Acknowledgements
The authors would like to thank the Researchers Supporting Project Number (RSPD2024R729), King Saud University, Riyadh, Saudi Arabia. The authors are also grateful to Chandigarh University, Gharuan for providing other financial assistance for research.
References
- H. M. Abd El-Lateef, M. A. Abo-Riya and A. H. Tantawy, Empirical and Quantum Chemical Studies on the Corrosion Inhibition Performance of Some Novel Synthesized Cationic Gemini Surfactants on Carbon Steel Pipelines in Acid Pickling Processes, Corros. Sci., 2016, 108, 94–110, DOI:10.1016/j.corsci.2016.03.004.
- M. A. Amin, S. S. Abd El-Rehim, E. E. F. El-Sherbini and R. S. Bayoumi, The Inhibition of Low Carbon Steel Corrosion in Hydrochloric Acid Solutions by Succinic Acid. Part I. Weight Loss, Polarization, EIS, PZC, EDX and SEM Studies, Electrochim. Acta, 2007, 52(11), 3588–3600, DOI:10.1016/j.electacta.2006.10.019.
- A. Saxena, D. Prasad, R. Haldhar, G. Singh and A. Kumar, Use of Saraca Ashoka Extract as Green Corrosion Inhibitor for Mild Steel in 0.5 M H2SO4, J. Mol. Liq., 2018, 258(17), 89–97, DOI:10.1016/j.molliq.2018.02.104.
- M. Goyal, S. Kumar, I. Bahadur, C. Verma and E. E. Ebenso, Organic Corrosion Inhibitors for Industrial Cleaning of Ferrous and Non-Ferrous Metals in Acidic Solutions: A Review, J. Mol. Liq., 2018, 256, 565–573, DOI:10.1016/j.molliq.2018.02.045.
- C. Verma, E. E. Ebenso, M. A. Quraishi and C. M. Hussain, Recent Developments in Sustainable Corrosion Inhibitors: Design, Performance and Industrial Scale Applications, Mater. Adv., 2021, 2(12), 3806–3850, 10.1039/d0ma00681e.
- S. Deng and X. Li, Inhibition by Ginkgo Leaves Extract of the Corrosion of Steel in HCl and H 2SO 4 Solutions, Corros. Sci., 2012, 55, 407–415, DOI:10.1016/j.corsci.2011.11.005.
- L. Ghalib, Chapter 1 - Impact of Presence of CO2 in Natural Gas Streams, in In Micro and Nano Technologies, Nanomaterials for Carbon Dioxide Capture and Conversion Technologies, ed. S. Ali Mazari and M. T. Nabisab Mujawar Mubarak, Elsevier, 2023, pp. 1–24, DOI:10.1016/B978-0-323-89851-5.00002-0.
- D. P. Burduhos-Nergis, P. Vizureanu, A. V. Sandu and C. Bejinariu, Evaluation of the Corrosion Resistance of Phosphate Coatings Deposited on the Surface of the Carbon Steel Used for Carabiners Manufacturing, Appl. Sci., 2020, 10(8), 2753, DOI:10.3390/APP10082753.
- M. R. Ali, C. M. Mustafa and M. Habib, Effect of Molybdate, Nitrite and Zinc Ions on the Corrosion Inhibition of Mild Steel in Aqueous Chloride Media Containing Cupric Ions, J. Sci. Res., 2009, 1(1), 82–91, DOI:10.3329/jsr.v1i1.1053.
- A. Farhadian, A. Rahimi, N. Safaei, A. Shaabani, M. Abdouss and A. Alavi, A Theoretical and Experimental Study of Castor Oil-Based Inhibitor for Corrosion Inhibition of Mild Steel in Acidic Medium at Elevated Temperatures, Corros. Sci., 2020, 175, 108871, DOI:10.1016/j.corsci.2020.108871.
- N. Gunavathy and S. C. Murugavel, Corrosion Inhibition Study of Bract Extract of Musa Acuminata Inflorescence on Mild Steel in Hydrochloric Acid Medium, IOSR J. Appl. Chem., 2013, 5(2), 29–35 CrossRef.
- S. Hejazi, S. Mohajernia, M. H. Moayed, A. Davoodi, M. Rahimizadeh, M. Momeni, A. Eslami, A. Shiri and A. Kosari, Electrochemical and Quantum Chemical Study of Thiazolo-Pyrimidine Derivatives as Corrosion Inhibitors on Mild Steel in 1M H2SO4, J. Ind. Eng. Chem., 2015, 25, 112–121, DOI:10.1016/j.jiec.2014.10.020.
- A. S. Ishak and N. Mohd, Thermodynamic Study of Corrosion Inhibition of Mild Steel in Corrosive Medium by Piper Nigrum Extract, Indian J. Sci. Technol., 2015, 8(17), 1–7 Search PubMed.
- G. Ji, S. Anjum, S. Sundaram and R. Prakash, Musa Paradisica Peel Extract as Green Corrosion Inhibitor for Mild Steel in HCl Solution, Corros. Sci., 2015, 90, 107–117, DOI:10.1016/j.corsci.2014.10.002.
- X. Zheng, S. Zhang, W. Li, L. Yin, J. He and J. Wu, Investigation of 1-Butyl-3-Methyl-1H-Benzimidazolium Iodide as Inhibitor for Mild Steel in Sulfuric Acid Solution, Corros. Sci., 2014, 80, 383–392, DOI:10.1016/j.corsci.2013.11.053.
- J. Omprakash, N. V. Prasad, G. Prasad, V. G. Krishnan and G. S. Kumar, Electrical Measurements on Musa Acuminata Colla, Univers. J. Electr. Electron. Eng., 2014, 2(8), 320–327, DOI:10.13189/ujeee.2014.020803.
- N. Soltani, N. Tavakkoli, M. Khayatkashani, M. R. Jalali and A. Mosavizade, Green Approach to Corrosion Inhibition of 304 Stainless Steel in Hydrochloric Acid Solution by the Extract of Salvia Officinalis Leaves, Corros. Sci., 2012, 62, 122–135, DOI:10.1016/j.corsci.2012.05.003.
- M. A. Quraishi, A. Singh, V. K. Singh, D. K. Yadav and A. K. Singh, Green Approach to Corrosion Inhibition of Mild Steel in Hydrochloric Acid and Sulphuric Acid Solutions by the Extract of Murraya Koenigii Leaves, Mater. Chem. Phys., 2010, 122(1), 114–122, DOI:10.1016/j.matchemphys.2010.02.066.
- K. Rose, B. S. Kim, K. Rajagopal, S. Arumugam and K. Devarayan, Surface Protection of Steel in Acid Medium by Tabernaemontana Divaricata Extract: Physicochemical Evidence for Adsorption of Inhibitor, J. Mol. Liq., 2016, 214, 111–116, DOI:10.1016/j.molliq.2015.12.008.
- M. Mahdavian and M. M. Attar, Electrochemical Behaviour of Some Transition Metal Acetylacetonate Complexes as Corrosion Inhibitors for Mild Steel, Corros. Sci., 2009, 51(2), 409–414, DOI:10.1016/j.corsci.2008.11.010.
- P. C. Okafor, M. E. Ikpi, I. E. Uwah, E. E. Ebenso, U. J. Ekpe and S. A. Umoren, Inhibitory Action of Phyllanthus Amarus Extracts on the Corrosion of Mild Steel in Acidic Media, Corros. Sci., 2008, 50(8), 2310–2317, DOI:10.1016/j.corsci.2008.05.009.
- G. Sığırcık, D. Yildirim and T. Tüken, Synthesis and Inhibitory Effect of N,N′-Bis(1-Phenylethanol)Ethylenediamine against Steel Corrosion in HCl Media, Corros. Sci., 2017, 120, 184–193, DOI:10.1016/j.corsci.2017.03.003.
- T. Ibrahim, H. Alayan and Y. Al. Mowaqet, The Effect of Thyme Leaves Extract on Corrosion of Mild Steel in HCl, Prog. Org. Coat., 2012, 75(4), 456–462, DOI:10.1016/j.porgcoat.2012.06.009.
- R. A. Ukpe, S. A. Odoemelam, A. O. Odiongenyi and N. O. Eddy, Inhibition of the Corrosion of Aluminium in 0.1 M HCl by Ethanol Extract of Mango Peel Waste (EMPW), J. Bioprocess. Chem. Eng., 2014, 2(2), 1–9 Search PubMed.
- M. Shabani-Nooshabadi, S. M. Ghoreishi, Y. Jafari and N. Kashanizadeh, Electrodeposition of Polyaniline-Montmorrilonite Nanocomposite Coatings on 316L Stainless Steel for Corrosion Prevention, J. Polym. Res., 2014, 21(4), 1–10, DOI:10.1007/s10965-014-0416-5.
- S. Sasmal, S. Majumdar, M. Gupta, A. Mukherjee and P. K. Mukherjee, Pharmacognostical, Phytochemical and Pharmacological Evaluation for the Antipyretic Effect of the Seeds of Saraca Asoca Roxb, Asian Pac. J. Trop. Biomed., 2012, 2(10), 782–786, DOI:10.1016/S2221-1691(12)60229-9.
- K. A. Alamry, A. Khan, J. Aslam, M. A. Hussein and R. Aslam, Corrosion Inhibition of Mild Steel in Hydrochloric Acid Solution by the Expired Ampicillin Drug, Sci. Rep., 2023, 13(1), 1–15, DOI:10.1038/s41598-023-33519-y.
- A. A. Rahim, E. Rocca, J. Steinmetz, M. J. Kassim, R. Adnan and M. Sani Ibrahim, Mangrove Tannins and Their Flavanoid Monomers as Alternative Steel Corrosion Inhibitors in Acidic Medium, Corros. Sci., 2007, 49(2), 402–417, DOI:10.1016/j.corsci.2006.04.013.
- M. Shabani-Nooshabadi, S. M. Ghoreishi and M. Behpour, Electropolymerized Polyaniline Coatings on Aluminum Alloy 3004 and Their Corrosion Protection Performance, Electrochim. Acta, 2009, 54(27), 6989–6995, DOI:10.1016/j.electacta.2009.07.017.
- M. Shabani-Nooshabadi and M. S. Ghandchi, Santolina Chamaecyparissus Extract as a Natural Source Inhibitor for 304 Stainless Steel Corrosion in 3.5% NaCl, J. Ind. Eng. Chem., 2015, 31(15), 231–237, DOI:10.1016/j.jiec.2015.06.028.
- L. Li, X. Zhang, J. Lei, J. He, S. Zhang and F. Pan, Adsorption and Corrosion Inhibition of Osmanthus Fragran Leaves Extract on Carbon Steel, Corros. Sci., 2012, 63, 82–90, DOI:10.1016/j.corsci.2012.05.026.
- V. V. Torres, R. S. Amado, C. F. de Sá, T. L. Fernandez, C. A. da S. Riehl, A. G. Torres and E. D'Elia, Inhibitory Action of Aqueous Coffee Ground Extracts on the Corrosion of Carbon Steel in HCl Solution, Corros. Sci., 2011, 53(7), 2385–2392, DOI:10.1016/j.corsci.2011.03.021.
- S. Y. Al-Nami, A. M. Alturki and A. M. Wahba, Eco-Friendly Methanolic Myrrh Extract Corrosion Inhibitor for Aluminum in 1 M HCl, ACS Omega, 2023, 8, 30917–30928, DOI:10.1021/acsomega.3c02009.
- H. Kumar, V. Yadav, Kr. Anu, S. Saha and N. Kang, Adsorption and Inhibition Mechanism of Efficient and Environment Friendly Corrosion Inhibitor for Mild Steel: Experimental and Theoretical Study, J. Mol. Liq., 2021, 338, 116634, DOI:10.1016/j.molliq.2021.116634.
- D. Wang, Y. Li, B. Chen and L. Zhang, Novel Surfactants as Green Corrosion Inhibitors for Mild Steel in 15% HCl: Experimental and Theoretical Studies, Chem. Eng. J., 2020, 402, 126219, DOI:10.1016/j.cej.2020.126219.
- A. Kadhim, N. Betti, H. A. Al-Bahrani, M. K. S. Al-Ghezi, T. Gaaz, A. H. Kadhu and A. Alamiery, A Mini Review on Corrosion, Inhibitors and Mechanism Types of Mild Steel Inhibition in an Acidic Environment, Int. J. Corros. Scale Inhib., 2021, 10(3), 861–884, DOI:10.17675/2305-6894-2021-10-3-2.
- T. D. Manh, T. L. Huynh, B. V. Thi, S. Lee, J. Yi and N. Nguyen Dang, Corrosion Inhibition of Mild Steel in Hydrochloric Acid Environments Containing Sonneratia Caseolaris Leaf Extract, ACS Omega, 2022, 7(10), 8874–8886, DOI:10.1021/acsomega.1c07237.
- A. Dehghani, G. Bahlakeh, B. Ramezanzadeh and M. Ramezanzadeh, Potential Role of a Novel Green Eco-Friendly Inhibitor in Corrosion Inhibition of Mild Steel in HCl Solution: Detailed Macro/Micro-Scale Experimental and Computational Explorations, Constr. Build. Mater., 2020, 245, 118464, DOI:10.1016/j.conbuildmat.2020.118464.
- Y. Boughoues, M. Benamira, L. Messaadia and N. Ribouh, Adsorption and Corrosion Inhibition Performance of Some Environmental Friendly Organic Inhibitors for Mild Steel in HCl Solution via Experimental and Theoretical Study, Colloids Surf., A, 2020, 593, 124610, DOI:10.1016/j.colsurfa.2020.124610.
|
This journal is © The Royal Society of Chemistry 2024 |
Click here to see how this site uses Cookies. View our privacy policy here.