DOI:
10.1039/D4RA04965A
(Paper)
RSC Adv., 2024,
14, 37131-37141
Antileishmanial potential of thiourea-based derivatives: design, synthesis and biological activity†
Received
9th July 2024
, Accepted 4th November 2024
First published on
19th November 2024
Abstract
Leishmaniasis is a neglected tropical disease caused by protozoan parasites and transmitted to humans by the sandfly vector. Currently, the disease has limited therapeutic alternatives. Thiourea derivatives were designed, synthesized, and screened for antileishmanial activity. The synthesized compounds 4g, 20a, and 20b demonstrated significant in vitro potency against L. major, L. tropica, and L. donovani promastigotes with IC50 values at low submicromolar concentrations. Compound 4g showed the highest activity against the amastigotes of L. major. In enzyme inhibition assays, compounds 4g, 20a, and 20b demonstrated good inhibitory potential against L. major dihydrofolate reductase (DHFR) and pteridine reductase 1 (PTR1). Reversal of the antileishmanial effect by adding folic acid revealed that the compounds 4g, 20a, and 20b act through an antifolate mechanism. Cytotoxicity data on normal human embryonic kidney cells (HEK-293) showed that the synthesized compounds displayed better safety profiles. Docking experiments on the enzymes L. major DHFR and PTR1 demonstrated the significant interactions with the active pocket residues of the target enzymes.
Introduction
The parasitic disease leishmaniasis is a neglected tropical disease (NTD) mainly caused by twenty species of Leishmania. All Leishmania infections commence with the sandfly bite, which introduces the protozoan parasite into the host's blood through the skin during its blood feeding. Every year about one million people get affected by leishmaniasis, which is becoming a social, health, and economic burden on many countries in subtropical regions. In the Middle East, Northwestern China, and Northern Africa, the main cause of cutaneous leishmaniasis is Leishmania major.1–5
The symptoms of leishmaniasis depend upon the clinical form, i.e., cutaneous and visceral, and the type of the species.6 The three well-known clinical forms are cutaneous leishmaniasis abbreviated as CL, mucocutaneous leishmaniasis abbreviated as MCL and visceral leishmaniasis, also called kala-azar, abbreviated as VL.7 Post-kala-azar dermal leishmaniasis abbreviated as PKDL is the least common clinical form of the disease.8 The general symptoms are skin rashes, erythema, and papules that later become scars as seen in CL and fever, black spots on the skin, and enlargement of the spleen as seen in visceral leishmaniasis.8,9 Besides the skin, other parts of the body, such as the ears, lips, mucosa of the nose, and mouth, are also affected by leishmaniasis.10 The disease is widely distributed in the tropics as well as subtropics.11 In the US, leishmania cases are diagnosed among travellers to endemic regions, armed forces from the military, and immigrants.12 In the life cycle of Leishmania, the parasite protozoan experiences a digenetic style, i.e., promastigotes and amastigotes.13 The promastigote forms of the parasite multiply extracellularly in the vector's digestive tract or gut and are transmitted to the mammalian cells during the vector's blood-feeding meal, where they convert into amastigote forms, which are responsible for the clinical manifestation of leishmaniasis.14
The proliferation and growth of the Leishmania parasite are affected by different metabolic pathways. The metabolic pathway related to folate plays a crucial role in the biochemical processes; it depends on reduced pterin and tetrahydrofolate as essential cofactors. Hence, the most suitable targets associated with the folate metabolic pathway include pteridine reductase-1 and dihydrofolate reductase. An NADPH-dependent key enzyme called DHFR in the folate metabolism is responsible for the formation of deoxythymidine monophosphate (dTMP) from deoxy-uridine monophosphate (dUMP) and the conversion of dihydrofolate/folate to tetrahydrofolate. Thus, biosynthesis of thymidine and subsequent DNA biosynthesis can be prevented by the inhibition of DHFR, and as a result, this will disturb the parasite's folate metabolic pathway in Leishmania, which leads to the death of the parasite.5,15–18
Currently, the basis of antileishmanial treatment is chemotherapy. Different drug options are available and the choice of medication differs according to the disease state, parasite form, and geographic location.19 Drugs already available for treatment purposes are pentavalent antimonials (I.V), miltefosine (oral), pentamidine (intramuscular), liposomal amphotericin B (I.M and I.V), and paromomycin (topical). These drug candidates are in general associated with undesirable complications such as organ toxicity, teratogenicity, prolonged hospital stay, and reaction at the injection site. In addition, high costs and resistance remain the most important issues.10 Therefore, there is a need to seek alternative drug candidates and explore new therapeutic interventions to improve the existing therapy.
Several heterocyclic scaffolds such as triazole, imidazole, pyrazole, quinoline, pyrimidine, quinazoline, benzimidazole, pyrrole, and indole are being evaluated for the treatment of leishmaniasis.20,21 Moreover, N-acyl/dimeric N-arylpiperazine and halogen-rich salicylanilides have also been reported as potential antileishmanial agents.22,23 Thiourea, an organosulfur compound, is known for its therapeutic uses, including treating co-infections, acting as an antioxidant, exhibiting antibacterial activity, and showing anti-cancer properties.24 Thiourea derivatives were screened against parasitic protozoa for exploring their anti-amoebic and anti-leishmanial properties.25 Thiourea derivatives have been shown to inhibit both the wild-type and the resistant mutant forms of Plasmodium falciparum PfDHFR.26 Moreover, compounds belonging to the thiourea group (I–IV) have also been reported in the literature as antileishmanial therapeutics (Fig. 1). The cyclic propane derivatives of thiourea were screened against the promastigote form of L. major and showed significant antileishmanial potential.27 Other compounds of the thiourea class include II and III, which have also demonstrated antileishmanial potential against L. major.28 Compound IV, which is the basis of the rationale for the current study, was screened against both promastigote and amastigote forms of L. major and demonstrated efficient EC50 values with a good safety profile on mammalian cell lines.29 The compound (IV) possesses the thiourea functional group as its important constituent where only one hydrogen atom of the NH2 group of the thiourea moiety is substituted by an aromatic system.
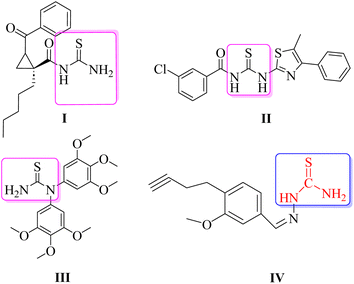 |
| Fig. 1 Thiourea derivatives reported against leishmaniasis. Structure IV is chosen for the rationale of the current study. | |
Materials and methods
General information
All the chemicals and reagents were obtained from commercial sources (Sigma-Aldrich and Merck) and used without any further purification. Melting points of the compounds were determined on the Stuart SMP10 apparatus. Reactions were monitored by thin-layer chromatography (TLC) using silica gel aluminium sheets. To determine the molecular mass, high-resolution mass spectrometry was carried out using Bruker Daltonics TSQ Quantum Classic LC-MS equipment at the Chemistry Department, University of Minnesota, USA. Only a positive method was used for ionization with the polymer PEG 300 or PPG 420. Proton (1H) and carbon (13C) NMR were taken using 400 MHz and 100 MHz, spectrometer, respectively. The chemical shift values and coupling constants were presented as delta (δ) in ppm and J in Hz, respectively. The multiplicities were represented by ‘s’ for singlet, ‘d’ for doublet, ‘t’ for triplet, and ‘q’ for quintet. Analytical grade acetone, methanol, ethanol, chloroform, dimethyl sulfoxide (DMSO), and n-hexane were used to synthesize the designed thiourea derivatives. Molecular sieves of 3 Å size were heat-activated and used for the drying of acetone.
Reaction procedure for the synthesis of thiourea derivatives (4a–4h and 5a–5d)
The thiourea derivatives were synthesized according to the previous method with slight modification.30 To the aromatic carboxylic acid (3 mmol) was added thionyl chloride (324 µL, 4.5 mmol). The mixture was heated to reflux along with stirring for 1 to 2 h. The resulting acid chloride was cooled to room temperature and dissolved in dry acetone. Then 3 mmol (292 mg) potassium thiocyanate was added to the solution and stirred for 2 h. Equimolar aromatic secondary amines and substituted anilines were added to the organic isothiocyanate mixture and refluxed for 3 to 4 hours to get the final product thiourea. The mixture was cooled, filtered, and washed with cold water. Finally, the product was dried and recrystallized with ethanol or methanol.
(N-((4-Methoxyphenyl)carbamothioyl)furan-2-carboxamide) (4a).
Off-white solid, yield: 73%, molecular weight 276.31, m.p. 99–101 °C, 1H NMR (400 MHz, CDCl3): δ 3.79 (s, 3H, methoxy-H), 6.53 (d, J = 2.8 Hz, 1H, furan-C2–H), 6.88 (d, J = 8.5 Hz, 2H, benzene-C9,11–H), 7.20 (d, J = 3.5 Hz, 1H, furan-C3–H), 7.48 (s, 1H, furan-C1–H), 7.54 (d, J = 8.5 Hz, 2H, benzene-C8,12–H), 7.98 (s, 1H, thiourea-NH–H), 13C NMR (100 MHz, DMSO-d6) δ ppm: 55.4 (methoxy-C), 77.2, 112.5, 114.2, 114.9, 121.6, 130.3, 144.0, 147.9 (Ar–C), 155.9 (C
O), 176.5 (thiourea C
S), anal. calcd for C13H12N2O3S, C, 56.51; H, 4.38; N, 10.14; O, 17.37; S, 11.60, found C, 56.49; H, 4.37; N, 10.11; O, 17.31; S, 11.57, HRMS: m/z: 299.0178 [M + Na]+.
(N-((2-Hydroxyphenyl)carbamothioyl)furan-2-carboxamide) (4b).
Light brown solid, yield 72%, molecular weight 262.28, m.p. 191–193 °C, 1H NMR (400 MHz, DMSO-d6) δ 6.58 (dd, J = 5.1, 1.5 Hz, 1H, furan-C2–H), 6.88 (dd, J = 8.4, 1.6 Hz, 1H, benzene-C11–H), 7.12–6.97 (m, 2H, benzene-C9,8–H), 7.38 (dd, J = 5.2, 1.6 Hz, 1H, furan-C3–H), 7.67 (dd, J = 8.0, 1.6 Hz, 1H, benzene-C8–H), 7.71 (t, J = 1.7 Hz, 1H, furan-C1–H), 8.35 (s, 1H, C12-hydroxyl-H), 10.55 (s, 1H, thiourea-NH–H), 11.26 (s, 1H, thiourea-NH–H), 13C NMR (100 MHz, DMSO-d6) δ ppm: 112.7, 115.4, 116.6, 121.6, 123.8, 125.5, 128.3, 145.6, 150.3, 150.8 (Ar–C), 160.3 (C
O), 179.2 (C
S), anal. calcd for C12H10N2O3S; C, 54.95; H, 3.84; N, 10.68; O, 18.30; S, 12.22, found C, 54.90; H, 3.80; N, 10.67; O, 18.27; S, 12.19, HRMS: m/z: 285.1198 [M + Na]+.
(N-((3-Hydroxyphenyl)carbamothioyl)furan-2-carboxamide) (4c).
Dark yellow solid, yield 87%, molecular weight 262.28, m.p. 175–176 °C, NMR (400 MHz, CDCl3) δ 5.19 (s, 1H, C11 phenyl hydroxy-H), 6.63 (dd, J = 3.7, 1.7 Hz, 1H, furan-C2–H), 6.75 (dd, J = 8.0, 2.4 Hz, 1H, phenol-C8–H), 7.12 (dd, J = 8.4, 1.7 Hz, 1H, phenol-C12-H), 7.24 (d, J = 16.1 Hz, 1H, phenol-C9–H), 7.38 (d, J = 3.7 Hz, 1H, furan-C3–H), 7.45 (t, J = 2.2 Hz, 1H, furan-C1–H), 7.64–7.59 (m, 1H, phenol-C10–H), 9.17 (s, 1H, thiourea-NH–H), 12.30 (s, 1H, thiourea-NH–H); 13C NMR (100 MHz, CDCl3) δ ppm, 108.5, 110.2, 112.7, 115.7, 116.6, 130.3, 140.0, 145.6, 150.8 (Ar–C), 157.9 (C–OH), 160.3 (C
O), 178.4 (thiourea C
S); anal. calcd for C12H10N2O3S; C, 54.95; H, 3.84; N, 10.68; O, 18.30; S, 12.22, found, C, 54.90; H, 3.80, N, 10.67; O, 18.27; S, 12.20, HRMS: m/z: 285.0317 [M + Na]+.
4-(3-(Furan-2-carbonyl)thioureido)benzoic acid (4d).
Yellow color solid, yield 77%, molecular weight 290.29, m.p. 275–277 °C, 1H NMR (400 MHz, DMSO-d6); δ 6.73 (dd, J = 3.7, 1.7 Hz, 1H, furan-C2–H), 7.86–7.79 (m, 3H, C8,12,3–H), 7.97–7.90 (m, 2H, benzene-C9,11–H), 8.04 (dd, J = 1.7, 0.8 Hz, 1H, furan-C1–H), 11.35 (s, 1H, thiourea-NH–H), 12.49 (s, 1H, thiourea-NH–H), 13C NMR (100 MHz, DMSO-d6) δ ppm, 112.6, 116.6, 121.1 (Ar–C), 126.7 (C–COOH), 130.5, 141.4, 145.5, 150.7(Ar–C), 160.2 (C
O), 168.4 (C
O–OH), 178.5 (thiourea C
S), anal. calcd for, C13H10N2O4S; C, 53.79; H, 3.47; N, 9.65; O, 22.05; S, 11.04, found C, 53.69; H, 3.41; N, 9.59; O, 22.09; S, 11.09. HRMS: m/z: 313.0252 [M + Na]+.
(N-((4-Methoxyphenyl)carbamothioyl)thiophene-2-carboxamide) (4e).
Light yellow solid, yield 71%, molecular weight 292.37, m.p. 175–176 °C, 1H NMR (400 MHz, DMSO-d6) δ 3.78 (s, 3H, methoxy-H), 6.96–6.88 (m, 2H, phenol-C8–12–H), 7.21 (dd, J = 6.5, 5.4 Hz, 1H, thiophene-C2–H), 7.62–7.54 (m, 2H, benzene-C9,11–H), 7.97 (dd, J = 5.4, 1.7 Hz, 1H, thiophene-C1–H), 8.21 (dd, J = 6.5, 1.6 Hz, 1H, thiophene-C3–H), 11.40 (s, 1H, thiourea-NH–H), 11.48 (s, 1H, thiourea-NH–H), 13C NMR (100 MHz, DMSO-d6) δ ppm; 55.33 (methoxy-C), 114.4, 126.5, 128.0, 129.6, 130.9, 132.2, 138.5 (Ar–C), 156.3 (C–O), 162.5(C
O), 178.3 (thiourea C
S); anal. calcd for C13H12N2O2S2, C, 53.41; H, 4.14; N, 9.58; O, 10.94; S, 21.93, found, C, 53.39; H, 4.11; N, 9.54; O, 10.92; S, 21.90, HRMS: m/z: 315.0300 [M + Na]+.
(N-((2-Hydroxyphenyl)carbamothioyl)thiophene-2-carboxamide) (4f).
Dark yellow solid, yield 70%, molecular weight 278.34, m.p. 228–229 °C, 1H NMR (400 MHz, DMSO-d6) δ ppm; 6.79 (td, J = 7.8, 1.4 Hz, 1H, phenol-C11–H), 6.89 (dd, J = 8.1, 1.4 Hz, 1H, thiophene-C2–H), 7.03 (td, J = 7.7, 1.6 Hz, 1H, phenol-C10–H), 7.22–7.16 (m, 1H, phenol-C8–H), 7.94 (d, J = 4.9 Hz, 1H, phenol-C9–H), 8.23 (d, J = 3.9 Hz, 1H, thiophene-C1–H), 8.40 (dd, J = 8.1, 1.6 Hz, 1H, thiophene-C3–H), 10.44 (s, 1H, C12–hydroxyl group–H), 11.36 (s, 1H, thiourea-NH–H), 12.70 (s, 1H, thiourea-NH–H), 13C NMR (100 MHz, DMSO-d6) δ ppm; 115.5, 118.8, 123.7, 126.1, 127.1, 129.2, 133.0, 135.7, 136.7 (Ar–C), 149.3 (C–OH), 162.5 (C
O), 177.6 (thiourea C
S), analysis calcd for C12H10N2O2S2; C, 51.78; H, 3.62; N, 10.06; O, 11.50; S, 23.04, found, C, 51.71; H, 3.58; N, 10.09; O, 11.48; S, 23.09, HRMS: m/z: 301.1078 [M + Na]+.
(N-((3-Hydroxyphenyl)carbamothioyl)thiophene-2-carboxamide) (4g).
Light yellow solid, yield 77%, molecular weight 278.34, m.p. 190–191 °C, 1H NMR (400 MHz, DMSO-d6) δ 6.63 (dd, J = 8.1, 2.4 Hz, 1H, phenol-C10-H), 6.98 (dd, J = 7.9, 2.0 Hz, 1H, phenol-C8–H), 7.16 (t, J = 8.0 Hz, 1H, thiophene-C2–H), 7.24–7.16 (m, 2H, phenol-C9,12–H), 7.99 (dd, J = 4.9 Hz, 1H, thiophene-C1–H), 8.31 (d, J = 3.9 Hz, 1H, thiophene-C3–H), 9.68 (s, 1H, C11–hydroxyl-OH–H), 11.48 (s, 1H, thiourea-NH–H), 12.36 (s, 1H, thiourea-NH–H), 13C NMR (100 MHz, DMSO-d6) δ ppm; 115.5, 118.8, 123.7, 126.1, 127.1, 129.2, 133.0, 135.7, 136.7 (Ar–C), 149.3 (C–OH), 162.5 (C
O), 177.6 (thiourea C
S), anal. calcd for C12H10N2O2S2, C, 51.78; H, 3.62; N, 10.06; O, 11.50; S, 23.04, found, C, 51.73; H, 3.59; N, 10.01; O, 11.45; S, 23.07, HRMS: m/z: 301.1078 [M + Na]+.
4-(3-(Thiophene-2-carbonyl)thioureido)benzoic acid (4h).
White solid, yield 78%, molecular weight 306.35, m.p. 268–269 °C, 1H NMR (400 MHz, DMSO-d6) δ 7.21 (dd, J = 5.0, 3.7 Hz, 1H, thiophene-C2–H), 7.91–7.80 (m, 3H, thiophene-C1, benzoic acid –C9,11-H), 7.98–7.87 (m, 2H, benzoic acid-C8,12-H), 8.03 (dd, J = 3.8, 1.2 Hz, 1H, thiophene-C3–H), 10.44 (s, 1H, thiourea-NH–H), 12.73 (s, 1H, thiourea-NH–H), 13C NMR (100 MHz, DMSO-d6) δ 180.3 (thiourea C
S), (160.6 carbonyl–C
O), 143.3, 140.0, 135.9, 133.2, 132.8, 130.7, 130.3, 130.1, 128.5, 123.9, 119.8 (Ar–C) anal. calcd for C13H10N2O3S2, C, 50.97; H, 3.29; N, 9.14; O, 15.67; S, 20.93, found, C, 50.94; H, 3.26; N, 9.10; O, 15.64; S, 20.91, HRMS: m/z: 329.0124 [M + Na]+.
N-(Pyridin-2-ylcarbamothioyl)furan-2-carboxamide (5a).
Light orange solid, yield 75%, molecular weight 247.27, m.p. 225–226 °C; 1H NMR (400 MHz, DMSO-d6) δ 6.74 (dd, J = 3.6, 1.7 Hz, 1H, furan-C2–H), 7.23 (t, J = 6.3 Hz, 1H, pyridine-C10-H), 7.56 (t, 1H, pyridine-C9–H), 7.87 (td, J = 7.8, 1.9 Hz, 1H, furan-C3–H), 8.05 (d, J = 1.7 Hz, 1H, furan-C1–H), 8.42 (dd, J = 5.0, 1.8 Hz, 1H, pyridine-C11-H), 8.68 (t, 1H, pyridine-C8–H), 11.40 (s, 1H, thiourea-NH–H), 12.97 (s, 1H, thiourea-NH–H). 13C NMR (100 MHz, DMSO-d6) δ, 177.85 (thiourea-C
S), (156.01, carbonyl C
O), 147.6, 139.9, 135.0, 119.0, 117.5, 117.4, 113.2, 112.9, 31.1, Ar–C), anal. calcd for C11H9N3O2S C, 53.43; H, 3.67; N, 16.99; O, 12.94; S, 12.97, found, C, 53.39; H, 3.63; N, 16.96; O, 12.90; S, 12.94, HRMS: m/z: 270.0301 [M + Na]+.
(N-(Thiazol-2-ylcarbamothioyl)furan-2-carboxamide) (5b).
Light yellow solid, yield 79%, molecular weight 253.29, m.p. 196–197 °C, 1H NMR (400 MHz, DMSO-d6): δ 6.58 (dd, J = 5.1, 1.5 Hz, 1H, furan-C2–H), 6.78 (d, J = 4.4 Hz, 1H, thiazole-C9–H), 7.12 (d, J = 4.6 Hz, 1H, thiazole-C8–H), 7.32 (dd, J = 5.2, 1.6 Hz, 1H, furan-C3–H), 7.81 (t, J = 1.7 Hz, 1H, furan-C1–H), 11.44 (s, 1H, thiourea-NH–H), 11.82 (s, 1H, thiourea-NH–H), 13C NMR (100 MHz, DMSO-d6) δ ppm: 111.6, 112.7, 116.6, 135.3, 145.6, 150.8, 160.4 (Ar–C), 161.6 (C
O), 176.4 (thiourea C
S), anal. calcd for C9H7N3O2S2; C, 42.68; H, 2.79; N, 16.59; O, 12.63; S, 25.31, found, C, 42.63; H, 2.75; N, 16.57; O, 12.60; S, 25.29, HRMS: m/z: 276.0191 [M + Na]+.
N-(Pyridin-2-ylcarbamothioyl)thiophene-2-carboxamide (5c).
Orange solid, yield 73%, molecular weight 297.78, m.p. 195–196 °C, 1H NMR (400 MHz, DMSO-d6) δ 7.24 (ddt, J = 5.0, 3.7, 2.3 Hz, 1H, thiophene-C2–H), 7.48 (ddd, J = 8.0, 4.7, 1.1 Hz, 1H, benzene-C8–H), 8.05 (dd, J = 5.0, 1.2 Hz, 1H, thiophene-C3–H), 8.31 (dt, J = 4.8, 1.5 Hz, 1H, benzene-C9–H), 8.42–8.34 (m, 2H, benzene-C10, thiophene-C1–H (m), 11.94 (s, 1H, thiourea-NH–H), 12.51 (s, 1H, thiourea-NH–H), 13C NMR (100 MHz, DMSO-d6) δ 180.7, thiourea C
S), 162.7 (carbonyl C
O), 147.8, 146.2, 137.4, 136.7, 136.2, 133.6, 133.2, 129.2, 123.6 (Ar–C), anal. calcd for, C11H9N3OS2, C, 44.37; H, 2.71; Cl, 11.90; N, 14.11; O, 5.37; S, 21.53, found, C, 44.31; H, 2.66; Cl, 11.88; N, 14.08; O, 5.35; S, 21.49, HRMS: m/z: 285.9771 [M + Na]+.
(N-(Thiazol-2-ylcarbamothioyl)thiophene-2-carboxamide) (5d).
Light yellow color solid, yield 75%, molecular weight 269.36, m.p. 188–189, 1H NMR (400 MHz, DMSO-d6) δ 7.24 (dd, J = 5.0, 3.9 Hz, 1H, thiophene-C2–H), 7.31 (d, J = 3.5 Hz, 1H, thiazol-C10–H), 7.62 (dd, J = 3.6 Hz, 1H, thiophene-C1–H), 8.09–8.02 (m, 1H, thiazol-C9–H), 8.37 (dd, J = 3.9 Hz, 1H, thiophene-C3–H), 12.09 (s, 1H, thiourea-NH–H), 13.99 (s, 1H, thiourea-NH–H), 13C NMR (100 MHz, DMSO-d6) δ ppm 111.9, 128.0, 129.6, 132.2, 135.5, 138.7, 161.6 (Ar–C), 162.6 (C
O), 176.6 (thiourea C
S), anal. calcd for C9H7N3OS3, C, 40.13; H, 2.62; N, 15.60; O, 5.94; S, 35.71, found, C, 40.11; H, 2.59; N, 15.57; O, 5.89; S, 35.73, HRMS: m/z: 292.0660 [M + Na]+.
General procedure for the synthesis of 1-(3-oxo-butanoyl)piperidine-4-carboxylic acid (8)
Synthesis of compound 8 was carried out by using our previously reported procedure.4 A mixture of piperidine-4-carboxylic acid (7) and ethyl acetoacetate was heated under reflux in absolute ethanol as the solvent. After completion of the reaction, the reaction mixture was cooled. Ethanol was evaporated and the crude material was purified by using silica gel chromatography (n-hexane/ethyl acetate, 20
:
1).
Transparent liquid, yield 80%, 1H NMR (400 MHz, CDCl3) δ 12.07 (s, 1H, –OH), 3.89 (s, 2H, –CH2), 3.27–3.19 (m, 4H, 2× pip-CH2), 3.09–3.04 (m, 1H, pip-CH), 2.69 (s, 3H, CH3), 2.24–2.17 (m, 2H, pip-CH2), 1.76–1.62 (m, 2H, pip-CH2).
General procedure for the synthesis of 1-(2-amino-4-methyl-6-phenylpyrimidine-5-carbonyl)piperidine-4-carboxylic acid (15)
Our previously reported multistep protocol was followed for the synthesis of 2-aminopyrimidine 15.31,32 The traditional Biginelli reaction was used to synthesize compound 12. Pyridinium chlorochromate (PCC) oxidised compound 12 into compound 13. Dihydropyrimidine (12, 5 mmol) was stirred with 30 mmol of PCC in DCM. Following the completion of the reaction (TLC, 15 hours), the solvent was removed, and silica gel chromatography (CH2Cl2/CH3OH, 9
:
1) was used to purify the crude product. Compound 13 (10 mmol) was then refluxed for 30 to 45 minutes in POCl3. After that, the reaction mixture was distilled to get rid of POCl3. The obtained crude product was purified using silica gel chromatography (DCM/CH3OH, 10
:
1). Compound 14 (10 mmol) was suspended in acetone. After adding 20 mmol of ammonium acetate, the mixture was stirred for three to four hours at RT. Compound 15 was obtained by filtering out the resultant precipitates and washing and drying them with diethyl ether.
White solid, yield 63%, m.p. 145–147 °C, 1H NMR (400 MHz, CDCl3) δ 12.07 (s, 1H, –OH), 7.37–7.28 (m, 5H, ArH), 6.69 (s, 2H, NH2), 3.78–3.70 (m, 2H, pip-CH2), 3.64–3.57 (m, 2H, pip-CH2), 3.06–3.03 (m, 1H, pip-CH), 2.56 (s, 3H, CH3), 2.39–2.30 (m, 2H, pip-CH2), 1.92–1.84 (m, 2H, pip-CH2). 13C NMR (100 MHz, CDCl3) δ 180.81, 167.52, 163.90, 163.11, 158.06, 136.09, 129.75, 129.28 (2C), 128.96 (2C), 113.20, 44.26 (2C), 40.70, 27.44 (2C), 23.46.
General procedure for the synthesis of thiourea derivatives (20a–b)
20 mmol of o-(p-tolyl)-chlorothionoformate 16 was added to a stirred solution of 2-aminopyrimidine 15 (20 mmol) in 30 mL acetone. The mixture was stirred continuously at RT for two hours. The completion of the reaction was verified using the TLC method. The precipitates obtained were filtered to obtain intermediate 17, which was used for further steps without any purification. The next step involved dissolving 10 mmol of compounds 18 and 19, 16 mmol of triethylamine, and crude intermediate 17 in 1,4-dioxane. The reaction mixture was then heated for three hours at 60 °C. After washing the resultant precipitates using diethyl ether and drying them, compounds 20a–b were obtained.33
1-(2-(3-(Furan-2-ylmethyl)thioureido)-4-methyl-6-phenyl pyrimidine-5-carbonyl)piperidine-4-carboxylic acid (20a).
Off-white solid, yield 70%, m.p. 195–197 °C, 1H NMR (400 MHz, CDCl3) δ 12.07 (s, 1H, –OH), 10.75 (s, 1H, NH), 8.73 (t, J = 3.4 Hz, 1H, NH–CH2), 7.48 (d, J = 6.0 Hz, 2H, ArH) 7.36–7.29 (m, 5H, ArH), 7.20 (d, 1H, J = 3.6 Hz, 2H, ArH) 6.57 (dd, J = 1.6 Hz, 3.6 Hz, 2H, ArH), 4.68 (d, J = 3.4 Hz, 2H, CH2–NH) 3.77–3.72 (m, 2H, pip-CH2), 3.63–3.57 (m, 2H, pip-CH2), 3.04 (p, 1H, J = 5.8 Hz, pip-CH), 2.56 (s, 3H, CH3), 2.37–2.33 (m, 2H, pip-CH2), 1.92–1.85 (m, 2H, pip-CH2). 13C NMR (100 MHz, CDCl3) δ 180.8, 177.5, 167.4, 162.1, 158.9, 157.8, 154.6, 142.5, 137.0, 129.8 (2C), 129.5, 128.9 (2C), 117.3, 110.5, 107.5, 44.2 (2C), 40.7, 38.7, 27.4 (2C), 23.2. LCMS: m/z = 480.1 [M + H]+; anal. calcd for C24H25N5O4S, C, 60.11; H, 5.25; N, 14.60; found, C, 60.20; H, 5.27; N, 14.61.
1-(4-Methyl-6-phenyl-2-(3-(thiophen-2-ylmethyl)thioureido) pyrimidine-5-carbonyl)piperidine-4-carboxylic acid (20b).
Yellow solid, yield 61%, m.p. 205–207 °C, 1H NMR (400 MHz, DMSO-d6) δ 12.1 (s, 1H, –OH), 10.7 (s, 1H, NH), 8.73 (t, J = 3.3 Hz, 1H, NH–CH2), 7.86 (d, J = 4.9 Hz, 1H, ArH), 7.35 (d, J = 4.2 Hz, 1H, ArH), 7.34–7.28 (m, 5H, ArH), 6.92 (d, J = 4.8 Hz, 1H, ArH), 4.68 (d, J = 3.3 Hz, 2H, CH2–NH), 3.67–3.60 (m, 2H, pip-CH2), 3.62–3.58 (m, 2H, pip-CH2), 3.04 (p, 1H, J = 5.8 Hz, pip-CH), 2.57 (s, 3H, CH3), 2.35–2.29 (m, 2H, pip-CH2), 1.92–1.88 (m, 2H, pip-CH2). 13C NMR (100 MHz, DMSO-d6) δ 181.8, 178.5, 168.4, 163.1, 159.9, 158.8, 155.6 (2C), 141.5, 138.2, 130.5, 128.9 (2C), 127.9 (2C), 119.3, 111.5, 109.5, 43.2 (2C), 41.6, 38.7, 26.4 (2C), 22.9. LC/MS: m/z = 496.5 [M + H]+; anal. calcd for C24H25N5O3S2, C, 58.16; H, 5.08; N, 14.13; found, C, 58.07; H, 5.05; N, 14.14.
Biological screening
In vitro antileishmanial activity.
For the in vitro antileishmanial assay, the promastigotes of L. major, L. tropica, and L. donovani were used, while for the amastigote assay, only L. donovani was used. The screening was done according to the reported procedure with slight modifications.34 The amastigote forms of the parasite were produced by the reported method.35 In brief, the parasites of each strain were cultured in tissue flasks containing the Gibco RPMI-1640 growth medium (Invitrogen) that was supplemented with 10% HIFcs (heat-inactivated fetal calf serum). The antibiotics penicillin (100 IU), streptomycin (100 µg mL−1), and L-glutamine 1% (Sigma) were also added to the medium. The compounds to be tested were first uniformly dissolved in DMSO to a concentration of 1 mg mL−1. Serial dilutions (three-fold) of the reference miltefosine, test sample, and standard solutions were also prepared with an appropriate concentration in fresh complete media. 100 µL culture media of 3 × 106 promastigotes of each strain, L. major, L. tropica, and L. donovani, were seeded in 96-well flat-bottom plates. The dilutions of compounds were introduced to their respective wells containing promastigotes. The wells containing promastigotes only were the positive control, while the wells containing 1% DMSO and the growth media alone were the negative control. After 24 h, a fluorescent dye alamarBlue (10 µL) was introduced to the respective wells and the fluorescence was measured at 630 and 540 nm after 48 h.
In the amastigote assay, serial dilutions of the synthesized compounds were made to a final concentration of 10–0.04 µg mL−1 using 50 µL culture media in 96-well flat-bottom plates. Then, to each well 50 µL suspension containing 2 × 103 cells per mL amastigotes was added. The plates were then incubated under 5% CO2 at 31 °C for 72 h. After 68 hours of incubation period, 10 µL of fluorochrome solution was added to each well and absorbance was recorded at the wavelengths of 530 nm and 590 nm for excitation and emission, respectively. The experiments were carried out in triplicate. Finally, the IC50 values were determined from the dose–response curve using GraphPad Prism 3.0. The results were expressed as mean ± SEM of the triplicate.
Reversal of antileishmanial activity.
Folate reversal was carried out as described by the previous method with slight modification.36 In this method, trimethoprim (TOP) was utilized as a positive control. The synthesized compounds 4c and 4g were used in concentrations of 0.50 µM and 0.26 µM, respectively, while the folic acid was used in 100 µL and 20 µL. The assay was carried out in two stages. First, the test compounds (4c and 4g) were incubated with 106 Leishmania cells suspended in a growth medium without the competitor folic acid. After 1 h, the parasite cells were washed with phosphate buffer saline (PBS), and centrifuged, media were discarded and the cells were re-suspended in a 24-well plate for incubation, counting, and calculation of percent survival. In the second stage, the same procedure was repeated with the addition of the competitor folic acid in the concentration mentioned above. Once again, the parasite cells were washed with PBS and centrifuged, media were discarded, and the cells were re-suspended in growth media for measurement of percent survival.
In vitro cytotoxicity testing.
This assay was carried out using normal human embryonic kidney cells (HEK-293) according to the reported method with slight modification.37 Here, in a 96-well plate, the test compounds in the concentration range of 0.1–1 mM were added to 1 × 105 cells per well under a 5% CO2 incubator at 36 °C for about 72 h. The culture medium (DMEM; Gibco), added with 5% heat-inactivated fetal bovine serum (HIFBS), was used as a growth medium for HEK-293 cells. Using the crystal violet method of staining, the cytotoxicity potential was determined in the form of CC50 value, which is the concentration of the test compound at 50% inhibition. For each compound, the experiment was performed in triplicate and GraphPad software was used for CC50 value calculation.
L. major DHFR (LmDHFR) enzyme inhibition assay.
By using our previously reported method, enzyme inhibition assay was performed on cloned and over-expressed L. major DHFR (LmDHFR).4,38
Docking studies
The ligand–receptor docking of the newly synthesized compounds (4g, 20a, and 20b) on LmDHFR was carried out by using our previously constructed homology model,4 while Leishmania major pteridine reductase 1 was retrieved with PDB ID: 2BFM. All the docking studies were performed on the Molecular Operating Environment, and the best-docked pose was visualized on Discovery Studio.
A database of the selected synthesized compounds was prepared, and energy was minimized to obtain stabilized bond angles and geometries. The database file was saved in mdb format. The site finder option of the selected active pocket of the targeted protein was done by keeping all amino acid residues as reported in the literature.39 Furthermore, the docking procedure was validated by docking the standard drugs trimethoprim and methotrexate. The synthesized compounds were docked by retaining 20 poses for each docked ligand. The 2D interactions for the most suitable docked poses were presented, and binding energy (S) for each docked pose was noted.
Results and discussion
Design rationale
Thiourea derivatives in the current study have been designed in comparison to N,N-disubstituted thiourea derivative II (Fig. 1). The compounds 4a–h were designed by using aniline derivatives on one side of the thiourea functionality and either a furan or thiophene ring on another side, while compounds 5a–d were designed to accommodate 2-pyridine and 2-thiazole as R2 substituents. Inspired by our previous study, the dihydropyrimidine-based framework has been employed to design and synthesize a series of derivatives. Biginelli type of dihydropyrimidine-2-ones (V) emerged as potent inhibitors of L. major DHFR. In the current research, we selected 2-aminopyrimidine synthesized from DHPM-2-one. The important aspect was that we modified the C-5 ester to piperidine-4-carboxylic acid to obtain 20a–b. Piperidine-4-carboxylic acid has been reported as an important scaffold in most antileishmanial derivatives (Fig. 2).
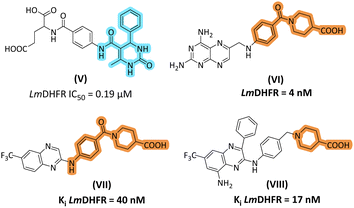 |
| Fig. 2 Design rationale of current study based on our previously reported DHPM-2-one based inhibitor of LmDHFR (V); piperidine-4-carboxylic acid-based inhibitors of LmDHFR (VI–VIII). | |
Chemistry
All the furan/thiophene carboxylic acid derivatives (4a–h and 5a–d) were synthesized as per the general scheme shown in Scheme 1. The starting materials for Scheme 1 were furan carboxylic acid, thiophene carboxylic acid, and thionyl-chloride, which were refluxed for 2–3 h, leading to the formation of the respective intermediate acid chlorides. The synthesized acid chloride intermediates were further reacted with KSCN at room temperature for 2–3 h to give isothiocyanates. The final products thiourea derivatives (4a–4h and 5a–5d) were obtained by refluxing the respective isothiocyanates with substituted anilines and primary amines.
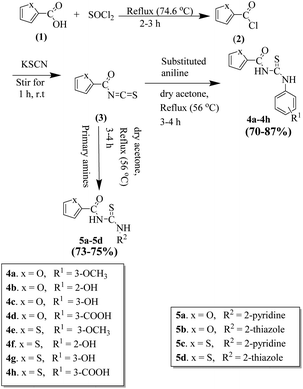 |
| Scheme 1 Schematic for the synthesis of thiourea derivatives (4a–4h and 5a–5d). | |
In the next step, we synthesized pyrimidine derivatives 20a–b. For this purpose, Biginelli dihydropyrimidine derivative (12) was synthesized by using benzaldehyde (10), urea (11), and 1-(3-oxobutanoyl)piperidine-4-carboxylic acid (8, synthesized via Scheme 2). Pyridinium chlorochromate (PCC) was used to oxidize 12 to derivative 13, which was further reacted with POCl3 to yield 2-chloro derivative 14.36 2-Chloro derivative 14 was finally reacted with ammonium acetate to give the target 2-aminopyridine derivative 15 (Scheme 3).
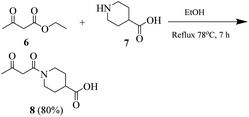 |
| Scheme 2 Schematic for the synthesis of 1-(3-oxobutanoyl)piperidine-4-carboxylic acid (8). | |
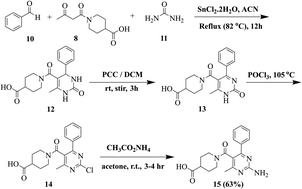 |
| Scheme 3 Schematic for the synthesis of 2-aminopyridine derivative 15. | |
Finally, 2-aminopyridine-based thiourea derivatives (20a–b) were synthesized by the reaction of (15) with o-(p-tolyl)chlorothionoformate (16) in the first step and then with furan-2-ylmethanamine (18) and thiophen-2-ylmethanamine (19) using dioxane solvent to obtain the target thiourea derivatives 20a–b (Scheme 4).
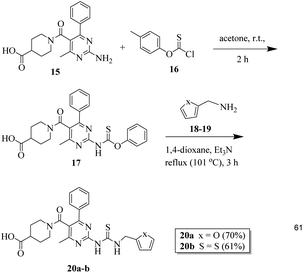 |
| Scheme 4 Schematic for the synthesis of 2-aminopyridine-based thiourea derivatives (20a–b). | |
Antileishmanial assay
In vitro antileishmanial activity against promastigotes and amastigotes.
A total of 14 compounds were used in the assay. The Leishmania parasite chosen for the study includes L. major, L. tropica, and L. donovani. The promastigote assay was performed against all these three forms of the parasite, while the amastigote assay was performed against L. major only. The minimum concentration of the drug needed to inhibit 50% promastigotes or amastigotes in the exponential stage of growth is termed IC50. In this assay, the activity was presented in terms of IC50. Most compounds displayed considerable antileishmanial activity ranging from micromolar to sub-micromolar. IC50 values were recorded in the range of 0.14–15.06 µM against promastigote forms and 0.31–8.50 µM against amastigote forms (Table 1). The results were interesting as most of the compounds showed inhibitory potential. 4c, 5a, 4f, 4g, 5c, 4h, 20a, and 20b stand out to be the significantly active compounds in promastigote and amastigote assays. Also, these were selected to be tested for in vivo antileishmanial activity.
Table 1 Representation of in vitro antileishmanial activity and IC50 values of the synthesized compounds against the promastigotes of L. major, L. tropica, and L. donovani and the amastigotes of L. majora
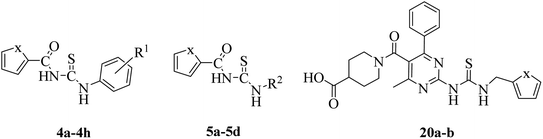
|
Comp. no. |
R1 |
R2 |
X |
Antileishmanial activity IC50 (µM) ± SEM* |
Promastigotes |
Amastigotes |
L. major
|
L. tropica
|
L. donovani
|
L. major
|
n = 3, SEM* = standard error of mean, n.d* = not determined.
|
4a
|
3-OCH3 |
— |
O |
5.34 ± 0.21 |
7.22 ± 0.61 |
10.61 ± 1.12 |
n.d* |
4b
|
2-OH |
— |
O |
6.08 ± 0.14 |
4.19 ± 0.10 |
2.46 ± 0.04 |
n.d |
4c
|
3-OH |
— |
O |
0.55 ± 0.01 |
0.68 ± 0.05 |
0.48 ± 0.04 |
0.67 ± 0.01 |
4d
|
3-COOH |
— |
O |
0.83 ± 0.02 |
1.07 ± 0.08 |
1.88 ± 0.11 |
3.24 ± 0.01 |
4e
|
3-OCH3 |
— |
S |
1.14 ± 0.05 |
1.23 ± 0.04 |
1.14 ± 0.02 |
n.d |
4f
|
2-OH |
— |
S |
0.38 ± 0.02 |
0.29 ± 0.01 |
0.41 ± 0.02 |
0.58 ± 0.01 |
4g
|
3-OH |
— |
S |
0.29 ± 0.01 |
0.48 ± 0.02 |
0.24 ± 0.01 |
0.31 ± 0.01 |
4h
|
3-COOH |
— |
S |
0.24 ± 0.03 |
0.33 ± 0.04 |
0.82 ± 0.04 |
3.04 ± 0.01 |
5a
|
— |
2-Pyridine |
O |
0.97 ± 0.10 |
0.94 ± 0.06 |
2.30 ± 0.01 |
8.50 ± 0.01 |
5b
|
— |
2-Thiazole |
O |
12.91 ± 0.42 |
12.38 ± 0.28 |
15.06 ± 0.01 |
n.d |
5c
|
— |
2-Pyridine |
S |
0.73 ± 0.09 |
0.79 ± 0.08 |
1.26 ± 0.01 |
n.d |
5d
|
— |
2-Thiazole |
S |
1.57 ± 0.04 |
1.53 ± 0.03 |
6.42 ± 0.21 |
n.d |
20a
|
— |
— |
O |
0.18 ± 0.01 |
n.d |
n.d |
0.47 ± 0.01 |
20b
|
— |
— |
S |
0.14 ± 0.01 |
n.d |
n.d |
0.39 ± 0.01 |
Miltefosine |
|
|
|
3.80 ± 0.16 |
2.12 ± 0.04 |
1.97 ± 0.07 |
2.89 ± 0.06 |
Structure–activity relationship (SAR) of thiourea derivatives concerning in vitro antileishmanial activity.
In both promastigote and amastigote assays, the activity significantly increased when the OH was positioned at the meta-position. The IC50 values of compound 4c were 0.55 µM, 0.68 µM, and 0.48 µM for the promastigotes of L. major, L. tropica, and L. donovani, respectively, and 0.67 µM for the amastigotes of L. major. In a similar structure, the activity decreased when the phenol group's OH was positioned at the ortho-position, as shown for compound 4b (IC50 of 6.08 µM, 4.19 µM and 2.46 µM for the promastigotes of L. major, L. tropica and L. donovani, respectively). It is intriguing that both activities were significantly further increased when the thiophene ring replaced the furan ring with OH positioned at the meta-position of the benzene ring, as shown by the compound 4f (IC50 of 0.38 µM, 0.29 µM, and 0.41 µM for the promastigotes of the above mentioned three species and 0.58 µM for the amastigotes of L. major). Compound 4g with OH positioned at the ortho position of the benzene also demonstrated high activity against all the tested targets (Table 1). These findings suggested that the thiophene ring linked to the phenol group through thiourea can further enhance the activity. We also anticipated that the unique groups in the thiourea compounds could serve as active pharmacophores for target recognition besides their role as physicochemical parameter modulators (molecular docking study). The compound 5c with the pyridine ring and thiophene ring showed decreased activity against the promastigote forms of all three species. Compound 5a also possesses a pyridine ring but the ring in this case is furan, which has also demonstrated good activity against both L. major promastigotes (IC50 = 0.97 µM) and amastigotes (IC50 = 8.50 µM). This reflects the role of pyridine in antileishmanial activity. The thiazole ring of compounds 5b and 5d displayed a decreased activity compared to the standard miltefosine.
Synthesis of a small set of 2-aminopyrimidine and furan-2-yl methanamine/thiophen-2-ylmethanamine-based thiourea analogues 20a–b (Scheme 4) was also explored to evaluate their potential as antileishmanial agents. The synthesized analogues showed higher potency compared with the 4a–h and 5a–d series of compounds against L. major promastigotes. Analogues 20a and 20b also possessed good potential against the amastigotes of L. major with IC50 values of 0.47 and 0.39 µM, respectively.
In conclusion, among the 4a–h and 5a–d series of compounds, it has been observed that the OH group, its position, and the type of ring, whether furan or thiophene, largely affect the antileishmanial activity of these compounds. Therefore, we can conclude that the activity is determined not only by the position of the –OH group but also by the type of ring, whether thiophene or furan, which also plays a role in determining antileishmanial potential. However, 20a and 20b thioureas show excellent results due to the presence of an aminopyridine scaffold. Compounds 4g, 20a, and 20b were selected for further studies due to their significant antileishmanial results.
In vitro inhibition of L. major dihydrofolate reductase (LmDHFR) and L. major PTR1.
From the in vitro antileishmanial assays, we selected 4g, 20a, and 20b to assess their inhibitory activities against LmDHFR and LmPTR1. 50% enzyme inhibition (IC50) values were used to determine the effectiveness of the synthesized compounds. IC50 values of the synthesized compounds are given in Table 2.
Table 2
In vitro inhibition results of L. major dihydrofolate reductase (LmDHFR)a
IC50 (µM) ± SEM* |
Compound no. |
L. major DHFR |
L. major PTR1 |
SEM* = standard error of the mean (n = 3).
|
4g
|
8.09 ± 0.01 |
0.58 ± 0.05 |
20a
|
2.63 ± 0.01 |
15.86 ± 0.01 |
20b
|
1.14 ± 0.01 |
19.89 ± 0.01 |
MTX |
0.004 ± 0.000 |
0.173 ± 0.008 |
Compounds 4g, 20a, and 20b showed good inhibitory activities with IC50 values of 8.09, 2.63, and 1.14 µM, respectively, for LmDHFR and IC50 values of 0.58, 15.86, and 19.89 µM, respectively, for LmPTR1.
In vivo antileishmanial activity.
The selected compounds 4g, 20a, and 20b were further explored for their in vivo antileishmanial activities. In this assay, 1.4 × f 106 promastigotes of L. tropica KWH23 were used, and the results were observed after 30–120 days (Table 3). In five tested groups, there was a significant reduction in the lesion size of the mice from 0.93 ± 0.22 mm to 0.38 ± 0.18 mm after being treated with the tested sample 20a (98.02% cure). On the other hand, the negative group displayed a mean lesion size up to 1.51 ± 0.51 mm (p > 0.05), whereas in the reference miltefosine group, the mean lesion size (end of 8th week) reduced from 0.94 ± 0.30 mm to 0.34 ± 0.40 mm. The tested samples 4g and 20b showed mean lesion sizes of 0.52 ± 0.80 mm to 0.32 ± 0.70 mm and 0.80 ± 0.12 mm to 0.60 ± 0.50 mm, respectively, with a corresponding percent cure rate of 91.51% and 86.28% after 8 weeks of treatment. It was proved from the results that the tested synthesized compounds exhibited potent in vivo antileishmanial activities against L. tropica. Compounds 20a and 4g showed significant micro-molar anti-leishmanial activity with a maximum cure rate, which suggested that proceeding with further studies on these derivatives would help to gain a better insight and a profound understanding of the biological activities along with the evaluation of the safety potential of the selected candidates.
Table 3
In vivo antileishmanial activities of the potent synthesized compoundsa
Compound no. |
Dose (mg kg−1 for five days) |
(Mean, lesion, mm ± SD*) pre-treatment |
Mean (lesion, mm ± SD*) post-treatment (after 8 weeks) |
Percent-cure rate |
Mice cured/mice infected |
Survival time (mean, months) |
n = 3. Data are mean lesion size (mm) ± SD and SD* = standard deviation.
|
4g
|
30 |
0.52 ± 0.80 |
0.32 ± 0.70 |
91.51% |
6/6 |
≥2 months |
20a
|
30 |
0.93 ± 0.22 |
0.38 ± 0.18 |
98.02% |
6/6 |
≥2 months |
20b
|
30 |
0.80 ± 0.26 |
0.60 ± 0.54 |
86.28% |
5/6 |
≥2 months |
Miltefosine |
15 |
0.94 ± 0.30 |
0.34 ± 0.40 |
95.10% |
6/6 |
≥2 months |
NC |
30 |
0.71 ± 0.51 |
1.51 ± 0.51 |
0.000% |
0/6 |
≥2 months |
Folic acid causes a reversal of the antileishmanial activity of the active compounds.
Reports revealed the potential of thio-based compounds to inhibit the leishmania enzymes dihydrofolate reductase (DHFR) and pteridine reductase (PTR1).40 These enzymes are responsible for Leishmania's resistance to available drugs. Hence, we expect that the target thiourea compounds would possibly exhibit the antileishmanial effect through folic acid pathway inhibition. This pathway in Leishmania involving one carbon transfer is based on an important cofactor tetrahydrofolate and another moiety pterin. The DHFR enzyme of Leishmania is different from human DHFR and it is responsible for the conversion of dihydrofolate to tetrahydrofolate. The parasite cell then uses this tetrahydrofolate for its growth and multiplication. It is a well-known fact that Leishmania species are resistant to DHFR inhibitors and they are no longer effective against Leishmania because of resistance.41,42 This resistance is induced by the presence of another enzyme called pteridine reductase PTR1, which provides an alternative salvage pathway that delivers meagre folate when the DHFR-TS is blocked. Intriguingly, this salvage enzyme PTR1 is not found in human hosts and thus serves as an important target for antileishmanial drug development.43 The antifolate mechanism of the compounds 4g, 20a, and 20b was confirmed by the previous method as described with slight modification.29 In this method, folic acid was added to the parasite first and then it was exposed to the tested compounds in the concentration above the IC50 values. The assay used trimethoprim as a positive control. The exposure led to almost 99% survival of the parasite, which means that our compounds act through an antifolate mechanism. It should be noted that folic acid is a substrate, and it competes with inhibitors for the above-mentioned enzymes DHFR and PTR1. As shown in Table 4 the antileishmanial effect of the compounds 4g, 20a, and 20b was reversed upon the addition of the substrate folic acid. From this data, the compounds 4g, 20a and 20b could be linked to the folate metabolism pathway targeting most probably the folate metabolism enzymes DHFR and PTR1. This was also confirmed by in vitro inhibition assay results (Table 2).
Table 4 Inhibition of the folic acid pathway expressed as percentage survival
Compounds |
No competitor |
Folic acid |
20 µM |
100 µM |
Percentage survival is obtained by the formula = 100 − %AP, where %AP is the percent of growth inhibited by either the compound or positive control. Folate activity (through the action on DHFR and PTR1) was obtained with the compounds 20a, 20b, and 4g, along with a positive control trimethoprim.
|
4g
|
a41% |
a81% |
a96% |
20a
|
a45% |
a88% |
a98% |
20b
|
a36% |
a77% |
a91% |
Trimethoprim |
a6% |
— |
a99% |
In vitro cytotoxicity testing.
Cytotoxicity assay of the three most potent compounds was performed to ascertain the safety of the synthesized derivatives. HEK-293 cells obtained from African green monkey kidney cells were used in the assay as reported by the previous method with slight modification.30 The cells were incubated for a period of 72 h with different dilutions of the compounds 4g, 20a, and 20b. The concentration at which 50% of HEK-293 cells are killed is termed CC50 value, which was found to be 539–786 µM (Fig. 3).
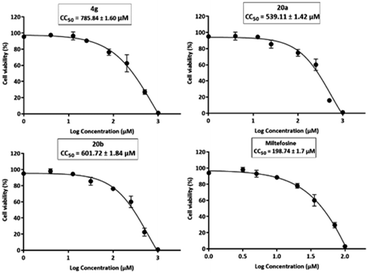 |
| Fig. 3 Graphs of cytotoxicity concentration 50 (CC50) of the final compounds (4g, 20a, and 20b and standard drug miltefosine) on HEK-293 cells. | |
Evaluation of in silico studies
Docking analysis.
To get an insight into the antileishmanial mechanism at the molecular level, docking studies were performed on both in vitro tested enzymes (LmDHFR and PTR1) to explore the binding interactions. Docking studies on LmDHFR were carried out on our previously reported homology-modelled enzyme, while the crystal structure of L. major PTR1 was obtained by using PDB ID: 2BFM. It is a tetrameric protein, where each single chain contains 288 amino acid residues and a co-crystallized ligand trimethoprim. The synthesized compounds were docked in the active pocket of target proteins with amino acid residues and the important interactions are displayed by the compounds 4g, 20a, and 20b.
Docking studies on LmDHFR.
In the binding site of homology-modelled LmDHFR, compound 4g established two additional hydrogen bonds with amino acid residues Val156 and Asp52. In addition, one π–π stacking interaction and one π–sulphur type of hydrophobic interaction were also observed (Fig. 4a). Compound 20a displayed hydrophobic π–π stacked interaction with Phe56, π–sulphur with Met53, and H-bond hydrophilic interactions with Ala159 and Gly42 (Fig. 4b). Compound 20b also displayed the best binding affinities among the docked derivatives by forming five H-bond hydrophilic binding affinities with Gly43, Gly158, Asp41, Ile39, and Ala159. It also exhibited π–sulphur hydrophobic interaction with Met53 and Phe91, and π–π–T shaped interaction with Phe56 (Fig. 4c). The standard drug trimethoprim interacts with Ala32, Ile39, and Gly42 via hydrogen bond interactions, while Ile45 interacts with trimethoprim, forming a π–σ bond (Fig. 4d).
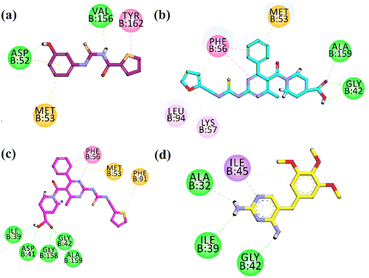 |
| Fig. 4 Two-dimensional (2D) interaction plots of the potent compounds (a) 4g, (b) 20a, (c) 20b and (d) standard drug trimethoprim in the binding region of homology modelled L. major DHFR. | |
Docking studies on PTR-1.
Docking studies were also performed on pterin reductase (PTR1) to get a deeper insight and to explore the binding interactions. The crystal structure of Leishmania major pteridine reductase 1 (PDB ID: 2BFM) is a tetrameric protein, where each single chain contains 288 amino acid residues and a co-crystallized ligand trimethoprim.
Compound 4g was a phenol derivative that established two additional hydrogen bonds with the amino acid residues of Val180 and Tyr194. In addition, two more hydrogen bonds were found between Ser111 and thiocarbamoyl functional groups as well as between Arg17 and the oxygen of the carbamoyl group. This resulted in a significantly high binding energy of −6.8573 kcal mole−1. This compound also exhibited π–sulphur and π–cation interaction with Phe113. There was also a π–alkyl interaction with Pro224, as presented in Fig. 5.
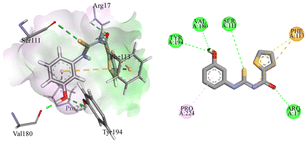 |
| Fig. 5 Putative binding mode of compound 4g in Leishmania major pteridine reductase 1 protein (PDB ID: 2BFM). | |
Conclusions
In this study, thiourea derivatives were designed, synthesized, and identified as antileishmanial agents in in vitro and in vivo tests. Most of the thiourea derivatives displayed moderate to high antileishmanial activity against the promastigotes of three species L. major, L. tropica, and L. donovani, and the amastigotes of L. major as compared to the reference drug miltefosine. 2-Aminopyrimidine-piperidine-4-carboxylic acid-based thiourea hybrids demonstrated excellent in vitro inhibition potential against the promastigotes and amastigotes of L. major. The selected compounds 4g, 20a, and 20b also exhibited good in vitro results against LmDHFR and LmPTR1 enzymes. The reversal of antileishmanial activity by adding folic acid suggested that the compounds exert their action through the anti-folate pathway, which involves the inhibition of Leishmania DHFR and PTR1 enzymes. The compounds appeared safe on normal human embryonic kidney cells (HEK-293). Molecular docking studies of compound 4g on L. major PTR1 and compounds 4g and 20a–b on L. major DHFR presented significant interactions with specific amino acids of the surrounding pockets. The evaluation of the results showed that compounds 4g and 20a–b could be promising leads/hits and enrich the arsenal of antileishmanial drug development.
Ethical statement
We followed the Ethical Guidelines for Animal Testing. The Ethical Committee of the Department of Pharmacy at Bacha Khan University, Pakistan via departmental ethical committee number (DECN/-2023–05) approved all the protocols. All the in vivo experiments were conducted by the Animals Bye-Laws of 2008 (Scientific Procedure, Issue-I).
Data availability
The data supporting this article have been included as part of the ESI.†
Author contributions
YMSA and UR conceived, designed, and supervised this study. Both were involved in all the phases (from synthesis to pharmacological evaluation and manuscript writing/editing) that led to the completion of this manuscript. AH synthesized compounds with the help of MY. In vitro experiments were performed by AH and FH. Docking studies were performed FH and AM. Docking results were analysed and written by UR. MSJ performed in vivo studies. Compound characterization and cytotoxicity studies were performed at the Department of Medicinal Chemistry, University of Minnesota, Minneapolis, USA under the supervision of TS. AH, MY, and FH drafted the manuscript. YMSA and UR reviewed and edited the drafts. All the authors have read the manuscript and approved it for publication. The authors declare that there is no conflict of interest.
Conflicts of interest
The authors declare no conflict of interest.
Acknowledgements
Abdul Hadi thanks the Higher Education Commission (HEC) Pakistan for supporting the research conducted under the Faculty Development Program (FDP/FMD3-001). Abdul Hadi also thanked the Medicinal Chemistry Department at the University of Minnesota, USA for conducting a portion of his PhD research.
References
- O. Gupta, T. Pradhan, R. Bhatia and V. Monga, Eur. J. Med. Chem., 2021, 223, 113606 CrossRef CAS PubMed.
- K. Sweiss, A. Y. Naser, M. Samannodi and H. Alwafi, BMC Infect. Dis., 2022, 22, 398 CrossRef PubMed.
- M. Zucca and D. Savoia, Open J. Med. Chem., 2011, 5, 4 CrossRef CAS PubMed.
- M. Bibi, N. A. Qureshi, A. Sadiq, U. Farooq, A. Hassan, N. Shaheen, I. Asghar, D. Umer, A. Ullah and F. A. Khan, Eur. J. Med. Chem., 2021, 210, 112986 CrossRef CAS PubMed.
- A. Sabt, W. M. Eldehna, T. M. Ibrahim, A. A. Bekhit and R. Z. Batran, Eur. J. Med. Chem., 2023, 246, 114959 CrossRef CAS PubMed.
- S. Sasidharan and P. Saudagar, Parasitol. Res., 2021, 120, 1541–1554 CrossRef PubMed.
- M. Akhoundi, K. Kuhls, A. Cannet, J. Votýpka, P. Marty, P. Delaunay and D. Sereno, PLoS Neglected Trop. Dis., 2016, 10, e0004349 CrossRef PubMed.
- H. J. de Vries and H. D. Schallig, Am. J. Clin. Dermatol., 2022, 23, 823–840 CrossRef PubMed.
- I. Abadías-Granado, A. Diago, P. Cerro, A. Palma-Ruiz and Y. Gilaberte, Actas Dermo-Sifiliogr., 2021, 601–618 CrossRef PubMed.
- S. Pradhan, R. Schwartz, A. Patil, S. Grabbe and M. Goldust, Clin. Exp. Dermatol., 2022, 47, 516–521 CrossRef CAS PubMed.
- D. Steverding, Parasites Vectors, 2017, 10, 1–10 CrossRef PubMed.
- I. Kevric, M. A. Cappel and J. H. Keeling, Dermatol. Clin., 2015, 33, 579–593 CrossRef CAS PubMed.
- G. Volpedo, R. H. Huston, E. A. Holcomb, T. Pacheco-Fernandez, S. Gannavaram, P. Bhattacharya, H. L. Nakhasi and A. R. Satoskar, Expert Rev. Vaccines, 2021, 20, 1431–1446 CrossRef CAS PubMed.
- L. Monzote, Open Antimicrob. Agents J., 2009, 1, 9–19 CAS.
- A. H. Hassan, K. Mahmoud, T.-N. Phan, M. A. Shaldam, C. H. Lee, Y. J. Kim, S. B. Cho, W. A. Bayoumi, S. M. El-Sayed and Y. Choi, Eur. J. Med. Chem., 2023, 250, 115211 CrossRef CAS PubMed.
- H. Istanbullu, G. Bayraktar, G. Karakaya, H. Akbaba, N. E. Perk, I. Cavus, C. Podlipnik, K. Yereli, A. Ozbilgin and B. D. Butuner, Eur. J. Med. Chem., 2023, 247, 115049 CrossRef CAS PubMed.
- J. A. Shiran, B. Kaboudin, N. Panahi and N. Razzaghi-Asl, Eur. J. Med. Chem., 2024, 116396 CrossRef PubMed.
- S. Kapil, P. Singh, A. Kashyap and O. Silakari, SAR QSAR Environ. Res., 2019, 30, 919–933 CrossRef CAS PubMed.
- D. O. Santos, C. E. Coutinho, M. F. Madeira, C. G. Bottino, R. T. Vieira, S. B. Nascimento, A. Bernardino, S. C. Bourguignon, S. Corte-Real and R. T. Pinho, Parasitol. Res., 2008, 103, 1–10 CrossRef PubMed.
- N. Razzaghi-Asl, S. Sepehri, A. Ebadi, P. Karami, N. Nejatkhah and M. Johari-Ahar, Mol. Diversity, 2020, 24, 525–569 CrossRef CAS PubMed.
- R. Pal, G. Teli, M. J. Akhtar and G. S. P. Matada, Eur. J. Med. Chem., 2023, 115927 Search PubMed.
- S. B. Ansari, S. Kamboj, K. Ramalingam, R. Meena, J. Lal, R. Kant, S. K. Shukla, N. Goyal and D. N. Reddy, Bioorg. Chem., 2023, 137, 106593 CrossRef CAS PubMed.
- J. Lal, K. Ramalingam, R. Meena, S. B. Ansari, D. Saxena, S. Chopra, N. Goyal and D. N. Reddy, Eur. J. Med. Chem., 2023, 246, 114996 CrossRef CAS PubMed.
- A. Shakeel, A. A. Altaf, A. M. Qureshi and A. Badshah, J. Drug Des. Med. Chem., 2016, 2, 10 Search PubMed.
- P. W. Meireles, D. P. de Souza, M. G. Rezende, M. P. G. Borsodi, D. E. De Oliveira, L. C. R. P. da Silva, A. M. T. de Souza, G. M. Viana, C. R. Rodrigues and F. A. do Carmo, Curr. Drug Delivery, 2020, 17, 694–702 CrossRef CAS PubMed.
- M. Sharma and P. M. Chauhan, Future Med. Chem., 2012, 4, 1335–1365 CrossRef CAS PubMed.
- B. Mohammadi-Ghalehbin, J. A. Shiran, N. Gholizadeh and N. Razzaghi-Asl, Mol. Diversity, 2023, 27, 1531–1545 CrossRef CAS PubMed.
- G. M. Viana, D. C. Soares, M. V. Santana, L. H. do Amaral, P. W. Meireles, R. P. Nunes, L. C. R. P. da Silva, L. C. de Sequeira Aguiar, C. R. Rodrigues and V. P. de Sousa, Chem. Pharm. Bull., 2017, 65, 911–919 CrossRef CAS PubMed.
- M. G. Temraz, P. A. Elzahhar, A. E.-D. A. Bekhit, A. A. Bekhit, H. F. Labib and A. S. Belal, Eur. J. Med. Chem., 2018, 151, 585–600 CrossRef CAS PubMed.
- A. Mahmood, S. J. A. Shah and J. Iqbal, Eur. J. Med. Chem., 2022, 231, 114162 CrossRef CAS PubMed.
- M. A. Javed, N. Ashraf, M. Saeed Jan, M. H. Mahnashi, Y. S. Alqahtani, B. A. Alyami, A. O. Alqarni, Y. I. Asiri, M. Ikram and A. Sadiq, ACS Chem. Neurosci., 2021, 12, 4123–4143 CrossRef CAS PubMed.
- M. A. Javed, M. S. Jan, A. M. Shbeer, M. Al-Ghorbani, A. Rauf, P. Wilairatana, A. Mannan, A. Sadiq, U. Farooq and U. Rashid, Biomed. Pharmacother., 2023, 159, 114239 CrossRef CAS PubMed.
- A. Tahir, B. Mobeen, F. Hussain, A. Sadiq and U. Rashid, RSC Adv., 2024, 14, 14742–14757 RSC.
- S. N. Khattab, N. S. Haiba, A. M. Asal, A. A. Bekhit, A. A. Guemei, A. Amer and A. El-Faham, Bioorg. Med. Chem. Lett., 2017, 27, 918–921 CrossRef CAS PubMed.
- M. Teixeira, R. de Jesus Santos, R. Sampaio, L. Pontes-de-Carvalho and W. L. dos-Santos, Parasitol. Res., 2002, 88, 963–968 CrossRef PubMed.
- C. Mendoza-Martínez, N. Galindo-Sevilla, J. Correa-Basurto, V. M. Ugalde-Saldivar, R. G. Rodríguez-Delgado, J. Hernández-Pineda, C. Padierna-Mota, M. Flores-Alamo and F. Hernández-Luis, Eur. J. Med. Chem., 2015, 92, 314–331 CrossRef PubMed.
- M. Tonelli, E. Gabriele, F. Piazza, N. Basilico, S. Parapini, B. Tasso, R. Loddo, F. Sparatore and A. Sparatore, J. Enzyme Inhib. Med. Chem., 2018, 33, 210–226 CrossRef CAS PubMed.
- U. Rashid, R. Sultana, N. Shaheen, S. F. Hassan, F. Yaqoob, M. J. Ahmad, F. Iftikhar, N. Sultana, S. Asghar and M. Yasinzai, Eur. J. Med. Chem., 2016, 115, 230–244 CrossRef CAS PubMed.
- F. Hussain, A. Tahir, M. S. Jan, N. Fatima, A. Sadiq and U. Rashid, RSC Adv., 2024, 14, 10304–10321 RSC.
- E. Lebrun, Y. Tu, R. van Rapenbusch, A. R. Banijamali and W. O. Foye, Biochim. Biophys. Acta, 1990, 1034, 81–85 CrossRef CAS PubMed.
- T. J. Vickers and S. M. Beverley, Essays Biochem., 2011, 51, 63–80 CrossRef CAS PubMed.
- B. Nare, L. W. Hardy and S. M. Beverley, J. Biol. Chem., 1997, 272, 13883–13891 CrossRef CAS PubMed.
- F. H. A. Leite, T. Q. Froes, S. G. da Silva, E. I. M. de Souza, D. G. Vital-Fujii, G. H. G. Trossini, S. S. da Rocha Pita and M. S. Castilho, Eur. J. Med. Chem., 2017, 132, 322–332 CrossRef CAS PubMed.
|
This journal is © The Royal Society of Chemistry 2024 |
Click here to see how this site uses Cookies. View our privacy policy here.