DOI:
10.1039/D4RA05383D
(Paper)
RSC Adv., 2024,
14, 29891-29895
Iodophor-catalyzed sulfenylation of indoles with sulfonyl hydrazides for the synthesis of 3-sulfenylindoles†
Received
25th July 2024
, Accepted 12th September 2024
First published on 19th September 2024
Abstract
An iodophor-catalyzed sulfenylation of indoles using sulfonyl hydrazides as sulfur source to synthesize 3-sulfenylindoles in aqueous phase has been achieved. Notably, iodophor as catalyst and solvent is inexpensive, commercially available and no innocuous to the environment. The method is also easy to operate. Moreover, the synthetic strategy features a wide range of substrates with excellent tolerance to diverse functional groups. A plausible mechanism for the iodophor-mediated 3-sulfenylation of indoles with sulfonyl hydrazides has been proposed. In addition, 3-(phenylthio)-1H-indole was obtained on a multi-gram scale.
Introduction
3-Sulfenylindoles are widely found in natural products and synthetic pharmaceuticals.1 In particular, 3-sulfenylindoles attract considerable attention for their medicinal value in the treatment of HIV,2 cancer,3 obesity,4 heart disease5 and allergies.6 They are also used as potent inhibitors of tubulin polymerization.7 In addition, 3-sulfenylindoles are often used as intermediates in organic synthesis.8 Generally there are two synthetic routes to synthesize 3-sulfenylindoles. One route is the 3-sulfenylation of indole with sulfenylating agents, such as sulfenyl halides,9 N-thioimides,10 sulfonium salts,11 thiols,12 disulphides,13 or other sources of sulfur.14,15 However, many of these sulfenylating agents are moisture and air sensitive, highly volatile and have unpleasant odours. In addition, many 3-sulfenylations require harsh reaction conditions, an inert atmosphere, and suffer from low functional group tolerance, or produce by-products that are environmentally unfriendly. Alternatively, 3-sulfenylindoles are synthesized through the annulation/cyclization of 2-(1-alkynyl)-benzenamines with sulfenylating agents such as disulfides and arylsulfenyl chlorides in the presence of transition metal catalysts16 or a stoichiometric amount of n-Bu4NI.17 However, these strategies are limited to the use of toxic and unstable sulfone halides as reaction partners. To overcome these problems, there is an urgent need to develop direct 3-sulfenylation of indoles under metal-free and mild conditions using stable and readily available materials.
Sulfonyl hydrazides are stable, commercially available and odourless, and have been extensively used in the synthesis of hydrazones and heterocycles.18 In addition, by cleaving their sulfur–nitrogen bonds, sulfonyl hydrazides can be used as environmentally friendly sources of sulfur.19 In 2013, the iodine-catalyzed regioselective sulfenylation of indoles with sulfonyl hydrazides was originally presented by Tian et al.20 The sulfenylation proceeded smoothly in the presence of 10 mol% I2, providing a range scope of 3-sulfenylindoles in moderate to excellent yields with extremely high regioselectivity. Subsequently, Wang and co-workers reported the sulfenylation of indoles with sulfonyl hydrazides in water without any catalyst or additive.21 The method has the low tolerance to steric hindrance of hydrazones and suffers from long time and high temperature. In the same year, Barman et al. reported a microwave-assisted synthetic approach for the sulfenylation of indoles with sulfonyl hydrazides, using a DBU-based ionic liquid.22 Various aryl and alkyl sulfonyl hydrazides smoothly reacted with indoles to afford a series of 3-sulfenylindoles in moderate to excellent yields in only 10 min. Other 3-sulfenylation of indoles with sulfonyl hydrazides has rarely been reported, especially in aqueous phase.
As a common topical disinfectant, iodophor (povidone–iodine in water) is widely used in our daily life. It is inexpensive, commercially available and not harmful to the environment. However, iodophor has rarely been used as a catalyst in organic chemistry. For the sake of environmental benignity and our continuous research interests,23,24 we present herein the iodophor-catalyzed sulfenylation of indoles with sulfonyl hydrazides for the synthesis of 3-sulfenylindoles in aqueous phase under mild conditions.
Results and discussion
The reaction of indole (1a) with benzenesulfonyl hydrazide (2a) in iodophor and open to air was chosen as a model reaction to screen for the optimal reaction conditions, and the results are summarized in Table 1. Initially, 1a was treated with 2a in a 1
:
1 ratio in 2 mL iodophor (0.04 mmol I2) at 100 °C for 12 h to give the target product 3a in 45% yield (Table 1, entry 1). The same reaction in an N2 atmosphere gave a yield of only 19% (Table 1, entry 2). The result implied that air played an important role in the course of the reaction. Increasing the amount of 2a improved the yield of product 3a to varying degrees (Table 1, entries 2–5). The main reason for this is probably that 2a is less soluble in water. It was found that 1
:
3 was the optimal ratio of 1a and 2a, affording product 3a in 61% yield. Revealing excess sulfonyl hydrazides could increase the yield of 3-sulfenylindoles. The temperature variations suggested that 110 °C was optimal, giving 80% yield of 3a (Table 1, entries 6–8). Reducing the I2 loading to 0.02 mmol resulted in a much lower yield (53%) (Table 1, entry 9). Furthermore, the efficiency of the reaction was obviously low with the extension of the reaction time and only 61% yield was obtained (Table 1, entry 10). In comparison, a relatively lower yields were obtained when the reactions of 1a and 2a in a ratio of 1
:
2.5 or 1
:
3.5 at 110 °C (Table 1, entries 11 and 12). Finally, the optimized reaction conditions were chosen as follows: indole (1a) (1 equiv.) with benzenesulfonyl hydrazide (2a) (3 equiv.) and 2 mL iodophor (0.04 mmol I2) at 110 °C for 12 h.
Table 1 Optimization of reaction conditionsa
The scope of indoles 1 and sulfonyl hydrazides 2 was explored respectively under optimal conditions. First, a series of 4-substituted aryl sulfonyl hydrazides bearing either electron-deficient groups (F, Br, NO2, CF3 and OCF3) or electron-rich groups (Me and OMe) were treated with indole 1a to afford corresponding 3-phenylthioindoles in good yields (3b–3h). In comparison, the substitution effect of electron absorbing groups (3b–3f) is slightly better than that of electron-rich groups (3g and 3h). As additional experiments, the sulfenylation for the synthesis of 3c and 3g have been investigated for 18 h to give 3c in 83% yield and 3g in 62% yield respectively. The yields of 3c and 3g have been improved to different degrees, but the electron-withdrawing group still gave a higher yield. A series of 2- or 3-substituted aryl sulfonyl hydrazides 2 were then treated with 1a to give the desired products 3i–3n in 66–75% yield. It should be noted that the different positions of the functional groups on the phenyl ring had little influence on the sulfenylation. These results suggest that the synthetic strategy has a high tolerance to different functional groups of aryl sulfonyl hydrazides 2 (Table 2).
Table 2 Iodophor-catalyzed sulfenylation of indole (1a) with aryl sulfonyl hydrazides (2)a,b
Reaction conditions: 1a (0.3 mmol), 2 (0.9 mmol), iodophor (2 mL), under air, 110 °C, 12 h. Isolated yield. Reaction time for 18 h. |
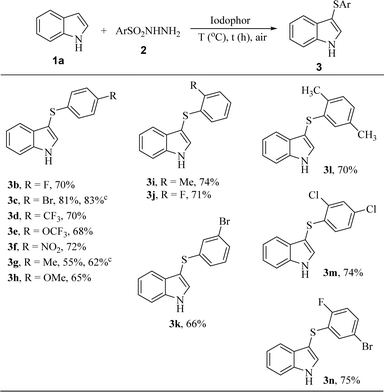 |
Subsequently, the substrate scope of indoles 1 was investigated. Various aryl-substituted indoles were treated with benzenesulfonyl hydrazide (2a) under the optimal conditions. Either electron withdrawing groups or electron donating groups on the N-1 (4l), C-2 (4j and 4k), C-4 (4a–4c), C-5 (4f and 4g), C-6 (4h and 4i) and C-7 (4d and 4e) afforded the desired 3-arylthioindoles in moderate to good yields. The results showed that the protocol is highly tolerant to different functional groups of indoles. Among them, electron-rich groups (Me) on the C-5 (4f) slightly influenced the reactivity with the lower yield of 57%. In addition, the sulfenylation of biheterocyclic 1H-pyrrolo[2,3-b]pyridine was investigated to afford the target product 4m in 66% (Table 3).
Table 3 Iodophor-catalyzed sulfenylation of indoles (1) with benzenesulfonyl hydrazide (2a)a,b
Reaction conditions: 1 (0.3 mmol), 2a (0.9 mmol), iodophor (2 mL), under air, 110 °C, 12 h. Isolated yield. |
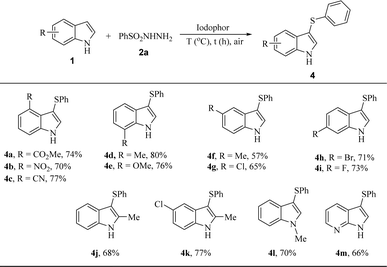 |
Based on our previous work24 and previous literature,13,21 a plausible mechanism for the iodophor-mediated 3-sulfenylation of indoles with sulfonyl hydrazides is illustrated in Scheme 1. First, benzenesulfonyl hydrazide (2a) was initiated by iodophor to afford intermediates PhSSPh (major) and PhSO2SPh (minor). On the one hand, PhSI derived from PhSSPh in the presence of I2 could react with 1a to give the intermediate I. Subsequently I rapidly transformed to product 3a by releasing a molecule of HI. On the other hand, PhSO2SPh could react with 1a without I2 to give the corresponding product 3a through intermediate II. To further prove the mechanism, the control experiments were carried out. Self-coupling of benzenesulfonohydrazide occurred under optimal conditions to give the corresponding product PhSSPh in 64% yield (Scheme 2a). PhSSPh was then treated with indole 1a under optimal conditions to give the desired product 3a in 78% yield (Scheme 2b). The results of the control experiments supported the proposed mechanism.
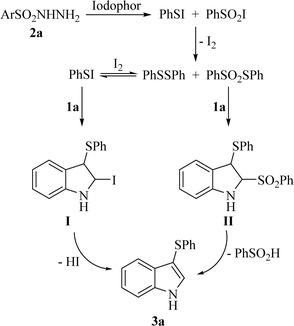 |
| Scheme 1 Proposed mechanism for 3-sulfenylation of indoles. | |
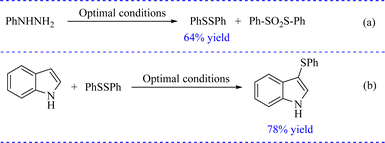 |
| Scheme 2 Control experiments (a) and (b) for proposed mechanism. | |
The gram-scale reaction was also performed by the reaction of 10 mmol indole (1a) with 30 mmol benzenesulfonyl hydrazide (2a) in 20 mL iodophor under the optimal reaction conditions. 1.5 g of 3a was obtained in 67% yield (Scheme 3). Moreover, diverse 3-sulfenylindoles can be obtained by the functionalization of 3a. For example, 3a was readily oxidized by Fe(NO3)3·9H2O to give the corresponding product 4n in trifluoroacetic acid (TFA). This gram-scale reaction demonstrated the practical applicability of this new protocol for the synthesis of diverse 3-sulfenylindoles.
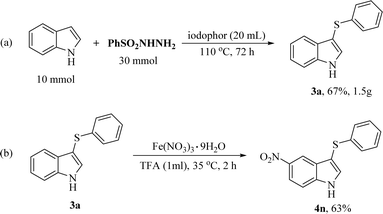 |
| Scheme 3 Gram-scale reaction of 3a and its derivatization. | |
Conclusions
In conclusion, we have developed an eco-friendly, mild and effective iodophor-catalyzed sulfenylation of indoles with sulfonyl hydrazides for the synthesis of 3-sulfenylindoles in aqueous phase. Iodophor as catalyst and solvent is inexpensive, commercially available and environment friendly. In addition, this approach has the high tolerance to various functional groups including electron withdrawing groups or electron donating groups. A plausible mechanism for the iodophor-mediated 3-sulfenylation of indoles with sulfonyl hydrazides has been proposed. In addition, the gram-scale sulfenylation of indole with benzenesulfonyl hydrazide offers the possibility for the synthesis of various 3-sulfenylindoles in medicinal chemistry and materials science.
Experimental section
General methods
Unless otherwise stated, all reactions were carried out in Schlenk tubes. Melting points were determined using a melting point apparatus and are uncorrected. Chemicals were purchased commercially and were used without further purification. Column chromatography was performed on Qingdao Ocean Chemical silica gel (200–300 mesh). 1H NMR and 13C NMR spectra were recorded on a Bruker Avance III HD 400 MHz spectrometer in CDCl3 with tetramethylsilane (TMS) as the internal standard. High resolution mass spectrometry (HRMS) was performed on a Thermo Scientific LTQ Orbitrap XL mass spectrometer, Thermo Fisher Q Exactive.
General procedure for iodophor-catalyzed sulfenylation of indoles with sulfonyl hydrazides
Indole 1a (0.3 mmol) and benzenesulfonyl hydrazide 2a (0.9 mmol) were introduced in a sealed 10 mL reaction tube, and 2 mL iodophor (0.04 mmol I2) (5% solution of the povidone–iodine in water) was added. The reaction was then continued at 110 °C for 12 h. When the reaction finished, the reaction mixture was cooled to room temperature. Saturated salt solution (10 mL) was then added and extracted with dichloromethane (3 × 10 mL). The combined organic layers were dried over anhydrous Na2SO4, and the organic solvent was evaporated on a rotatory evaporator. The crude was purified by flash chromatography on silica gel (PE/EtOAc) to give the corresponding products 3a.
Procedure for the gram-scale reaction of 3a and its derivatization
Indole 1a (10 mmol) and benzenesulfonyl hydrazide 2a (30 mmol) were introduced in a sealed 100 mL round-bottomed flask, and 20 mL iodophor was added. The reaction mixture was then stirred continuously at 110 °C for 72 h. After the reaction finished, the reaction mixture was cooled to room temperature and extracted with dichloromethane (5 × 10 mL). The combined organic layers were washed with water. The organic phase was then separated and dried over anhydrous Na2SO4 and filtered. Subsequently the organic solvent was evaporated on a rotatory evaporator. The crude was purified by flash chromatography on silica gel (PE/EtOAc) to give the corresponding products 3a in 67% yield (1.5 g).
A mixture of 3a (3 mmol) and Fe(NO3)3·H2O (1.5 mmol) in TFA (1 mL) was stirred for 2 h at 35 °C. After the completion of the reaction, the reaction mixture was neutralized with sodium bicarbonate solution and extracted with ethyl acetate (20 mL). The organic phase was separated and dried over Na2SO4 and filtered. The filtrate was concentrated and the resulting residue was purified by flash column chromatography on silica gel with PE/EtOAc as eluent to provide the product 4R in 63% yield.
Data availability
The data supporting this article have been included as part of the ESI.†
Conflicts of interest
There are no conflicts to declare.
Acknowledgements
We gratefully acknowledge the financial support of this work by Shanxi Provincial Basic Research Program (No. 20210302124467), Science and Technology Innovation Talents Program of Shihezi University (No. ZG010603), Innovation Development Program of Shihezi University (CXFZ202204) and Research Initiation Fund of Shanxi Agricultural University 2021BQ11.
References
-
(a) R. J. Sundberg, Indoles, Elsevier, 1996 Search PubMed;
(b) R. Ragno, A. Coluccia, G. La Regina, G. De Martino, F. Piscitelli, A. Lavecchia, E. Novellino, A. Bergamini, C. Ciaprini, A. Sinistro, G. Maga, E. Crespan, M. Artico and R. Silvestri, J. Med. Chem., 2006, 49, 3172 CrossRef CAS PubMed;
(c) A. J. Kochanowska-Karamyan and M. T. Hamann, Chem. Rev., 2010, 110, 4489 CAS;
(d) D. Crich and A. Banerjee, Acc. Chem. Res., 2007, 40, 151 CAS.
- R. Ragno, A. Coluccia, G. La Regina, G. De Martino, F. Piscitelli, A. Lavecchia, E. Novellino, A. Bergamini, C. Ciaprini, A. Sinistro, G. Maga, E. Crespan, M. Artico and R. Silvestri, J. Med. Chem., 2006, 49, 3172 CAS.
- G. La Regina, M. C. Edler, A. Brancale, S. Kandil, A. Coluccia, F. Piscitelli, E. Hamel, G. De Martino, R. Matesanz, J. F. Díaz, A. I. Scovassi, E. Prosperi, A. Lavecchia, E. Novellino, M. Artico and R. Silvestri, J. Med. Chem., 2007, 50, 2865 CAS.
-
(a) J. P. Berger, T. W. Doebber, M. Leibowitz, D. E. Moller, R. T. Mosley, R. L. Tolman, J. Ventre, B. B. Zhang and G. Zhou, WO0130343, 2001;
(b) V. S. N. Ramakrishna, V. S. Shirsath, R. S. Kambhampati, S. Vishwakarma, N. V. Kandikere, S. Kota and V. Jasti, WO2007020653, 2007.
- C. D. Funk, Nat. Rev. Drug Discovery, 2005, 4, 664 CAS.
- P. C. Unangst, D. T. Connor, S. R. Stabler, R. J. Weikert, M. E. Carethers, J. A. Kennedy, D. O. Thueson, J. C. Chestnut, R. L. Adolphson and M. C. Conroy, J. Med. Chem., 1989, 32, 1360 CAS.
-
(a) G. De Martino, M. C. Edler, G. La Regina, A. Coluccia, M. C. Barbera, D. Barrow, R. I. Nicholson, G. Chiosis, A. Brancale, E. Hamel, M. Artico and R. Silvestri, J. Med. Chem., 2006, 49, 947 CAS;
(b) Y. Maeda, M. Koyabu, T. Nishimura and S. Uemura, J. Org. Chem., 2004, 69, 7688 CAS.
-
(a) F. Zhao, N. Li, Y. F. Zhu and Z. Y. Han, Org. Lett., 2016, 18, 1506 CAS;
(b) J. Z. Huang, C. L. Zhang, Y. F. Zhu, L. L. Li, D. F. Chen, Z. Y. Han and L. Z. Gong, Chem.–Eur. J., 2015, 21, 8389 CAS.
-
(a) D. Equbal, R. S. Saima, A. G. Lavekar and A. K. Sinha, J. Org. Chem., 2019, 84, 2660 CAS;
(b) A. Ghosh, M. Lecomte, S. H. Kim-Lee and A. T. Radosevich, Angew. Chem., Int. Ed., 2019, 58, 2864 CAS;
(c) Y. Z. He, J. Jiang, W. H. Bao, W. Deng and J. Xiang, Tetrahedron Lett., 2017, 58, 4583 CAS;
(d) S. E. Denmark and G. L. Beutner, Angew. Chem., Int. Ed., 2008, 47, 1560 CAS.
-
(a) T. Hostier, V. Ferey, G. Ricci, D. G. Pardo and J. Cossy, Chem. Commun., 2015, 51, 13898 CAS;
(b) E. Marcantoni, R. Cipolletti, L. Marsili, S. Menichetti, R. Properzi and C. Viglianisi, Eur. J. Org Chem., 2013, 132 CAS;
(c) C. C. Silveira, S. R. Mendes, L. Wolf and G. M. Martins, Tetrahedron Lett., 2010, 51, 2014 CAS;
(d) M. Tudge, M. Tamiya, C. Savarin and G. R. Humphrey, Org. Lett., 2006, 8, 565 CrossRef CAS PubMed.
-
(a) X. Zhao, A. Wei, B. Yang, T. J. Li, Q. Li, D. Qiu and K. Lu, J. Org. Chem., 2017, 82, 9175 CrossRef CAS PubMed;
(b) X. Zhao, X. Zheng, M. M. Tian, J. Sheng, Y. F. Tong and K. Lu, Tetrahedron, 2017, 73, 7233 CrossRef CAS;
(c) C. R. Liu and L. H. Ding, Org. Biomol. Chem., 2015, 13, 2251 RSC;
(d) F. H. Xiao, H. Xie, S. W. Liu and G. J. Deng, Adv. Synth. Catal., 2014, 356, 364 CrossRef CAS;
(e) H. H. Rao, P. Wang, J. C. Wang, Z. F. Li, X. Z. Sun and S. L. Cao, RSC Adv., 2014, 4, 49165 RSC.
-
(a) D. L. Kong, T. Huang, M. Liang, M. S. Wu and Q. Lin, Org. Biomol. Chem., 2019, 17, 830 RSC;
(b) F. C. Bai, S. Zhang, L. Wei and Y. Y. Liu, Asian J. Org. Chem., 2018, 7, 371 CAS;
(c) R. Ohkado, T. Ishikawa and H. Iida, Green Chem., 2018, 20, 984 CAS;
(d) P. Choudhury, B. Roy and B. Basu, Asian J. Org. Chem., 2017, 6, 1569 CrossRef CAS;
(e) S. Malik, D. Equbal, A. G. Lavekar and A. K. Sinha, Org. Biomol. Chem., 2016, 14, 6111 RSC.
-
(a) T. Benchawan and R. Saeeng, Eur. J. Org Chem., 2022, 2022, e202201388 Search PubMed;
(b) S. Thurow, F. Penteado, G. Perin, D. Alves, C. Santi, B. Monti, C. H. Schiesser and E. J. Lenardao, Org. Chem. Front., 2018, 5, 1983 CAS;
(c) L. M. Ye, J. Chen, P. Mao, X. J. Zhang and M. Yan, Tetrahedron Lett., 2017, 58, 2743 CAS;
(d) P. Sang, Z. K. Chen, J. W. Zou and Y. H. Zhang, Green Chem., 2013, 15, 2096 CAS;
(e) L. H. Zou, J. Reball, J. Mottweiler and C. Bolm, Chem. Commun., 2012, 48, 11307 CAS;
(f) W. L. Ge and Y. Y. Wei, Green Chem., 2012, 14, 2066 CAS.
-
(a) M. Matsugi, K. Murata, H. Nambu and Y. Kita, Tetrahedron Lett., 2001, 42, 1077 CAS;
(b) M. Matsugi, K. Murata, K. Gotanda, H. Nambu, G. Anilkumar, K. Matsumoto and Y. Kita, J. Org. Chem., 2001, 66, 2434 CAS.
-
(a) G. Kumaraswamy, R. Raju and V. Narayanarao, RSC Adv., 2015, 5, 22718 CAS;
(b) M. Chen, Z. T. Huang and Q. Y. Zheng, Chem. Commun., 2012, 48, 11686 CAS;
(c) Q. Wu, D. B. Zhao, X. R. Qin, J. B. Lan and J. S. You, Chem. Commun., 2011, 47, 9188 CAS.
-
(a) S. K. Rastogi, R. Singh, S. Kumar, A. K. Mishra, M. B. Ahirwar, M. M. Deshmukh, A. K. Sinha and R. Kumar, Org. Biomol. Chem., 2023, 21, 838 CAS;
(b) Z. Li, L. C. Hong, R. T. Liu, J. Z. Shen and X. G. Zhou, Tetrahedron Lett., 2011, 52, 1343 CAS;
(c) Y. J. Guo, R. Y. Tang, J. H. Li, P. Zhong and X. G. Zhang, Adv. Synth. Catal., 2009, 351, 2615 CAS.
-
(a) Y. Chen, C. H. Cho, F. Shi and R. C. Larock, J. Org. Chem., 2009, 74, 6802 CAS;
(b) Y. Chen, C. H. Cho and R. C. Larock, Org. Lett., 2008, 11, 173 CrossRef PubMed.
-
(a) S. T. Zhao, K. J. Chen, L. Zhang, W. G. Yang and D. Y. Huang, Adv. Synth. Catal., 2020, 362, 3516 CrossRef CAS;
(b) Z. H. Shao and H. B. Zhang, Chem. Soc. Rev., 2012, 41, 560 RSC;
(c) J. Barluenga and C. Valds, Angew. Chem., 2011, 123, 7626 (Angew. Chem., Int. Ed., 2011, 50, 7486) CrossRef.
-
(a) C. Wang, Q. Y. Peng, Y. Wu, Y. L. He, X. H. Zheng, H. B. Wang, J. M. Xin, Q. He, J. Xie and L. Tang, RSC Adv., 2024, 14, 4587 RSC;
(b) X. Q. Li, X. S. Xu and C. Zhou, Chem. Commun., 2012, 48, 12240 RSC;
(c) T. Taniguchi, A. Idota and H. Ishibashi, Org. Biomol. Chem., 2011, 9, 3151 RSC;
(d) I. V. Ukrainets, A. A. Tkach, V. V. Kravtsova and S. V. Shishkina, Chem. Heterocycl. Compd., 2008, 44, 677 CrossRef CAS.
- F. L. Yang and S. K. Tian, Angew. Chem., Int. Ed., 2013, 52, 4929 CrossRef CAS PubMed.
- Y. Yang, S. Zhang, L. Tang, Y. B. Hu, Z. G. Zha and Z. Y. Wang, Green Chem., 2016, 18, 2609 RSC.
- R. Rahaman, N. Devi, K. Sarma and P. Barman, RSC Adv., 2016, 6, 10873 RSC.
- J. Zhang, Z. Wang, L. J. Chen, Y. Liu, P. Liu and B. Dai, RSC Adv., 2018, 8, 41651 CAS.
- Y. T. Yuan, J. He, X. W. Ma, S. Han and Y. Liu, Molecules, 2024, 29, 2411 CAS.
|
This journal is © The Royal Society of Chemistry 2024 |
Click here to see how this site uses Cookies. View our privacy policy here.