DOI:
10.1039/D4RA07386J
(Paper)
RSC Adv., 2024,
14, 38105-38109
Ir-catalyzed reductive amination and transfer hydrogenation of diketones: access to β- and γ-amino alcohols†
Received
15th October 2024
, Accepted 15th November 2024
First published on 29th November 2024
Abstract
β- and γ-Amino alcohols are among the most significant structural motifs in pharmacologically active molecules and pharmaceuticals. Herein, a protocol for the construction of β- and γ-amino alcohols via reductive amination and transfer hydrogenation of diketones with aromatic amines is described. This reaction is performed by utilizing iridium complexes as catalysts and HCO2H as a hydrogen donor to deliver a library of β- and γ-amino alcohols under mild and operationally simple conditions. Successful scale-up performance was also conducted under standard conditions.
β- and γ-Amino alcohol backbones are extensively found in pharmacologically active molecules and pharmaceutical compounds. Amino alcohols also serve as key intermediates of various pharmacophores in medicine, cosmetics, materials science, organic synthesis and medicinal chemistry.1 For instance, metaraminol is frequently employed as a rescue drug in patients with shock, chloramphenicol shows potent antibacterial activity against Gram-negative bacteria, and indinavir and adrenaline are used to treat HIV-1 infections in adults and children (Scheme 1).2 Amino alcohols also serve as ligands in the field of catalytic organic synthesis.3 Therefore, it is of great significance to develop a new universal, atomic and step-economical strategy for the synthesis of amino alcohols in the rapid development of innovative drugs.4
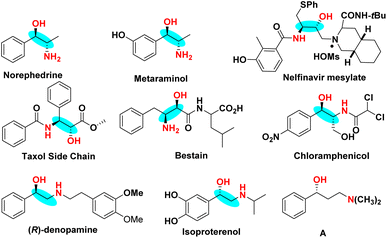 |
| Scheme 1 β- and γ-Amino alcohol motifs existing in biologically active molecules. | |
Ring-opening aminolysis of epoxides represents one of the classical strategies for constructing β-amino alcohols. Scandium,5 niobium,6 gadolinium,7 chromium,8 and organic molecules9 are employed as catalysts for the construction of β-amino alcohols. In addition, the Ru-catalyzed hydrogenation of α-amino ketones constitute an alternative effective strategy for β-amino alcohol synthesis.10 In 2019, Zhong's group reported a highly efficient Rh-catalyzed hydroxylation of alkenes to access β-amino alcohol compounds.11 In addition, the radical domino reaction12 and N-alkylation of amines with alcohols13 were employed for β-amino alcohol synthesis.
The hydrogenation of β-amino ketones14 could also be employed for γ-amino alcohol synthesis.15 In 2015, Zhang's group successfully reported the ruthenium complex-catalyzed hydrogenation of β-amino ketones to produce γ-amino alcohols.16 Furthermore, the hydroamination of allyl ketones17 and alcohols,18 ring-opening of epoxides with amines,2 oxidative amination of alkenes,19 addition or reduction of amino aldehydes or ketones,20 addition of an amino-carbon anion to a carbonyl compound,21 and the amine-allylation of alcohols22 were utilized as effective strategies for the formation of γ-amino alcohols. Although many encouraging achievements have been made in the synthesis of chiral β- and γ-amino alcohols, there are still some deficiencies in the substrate scope. Therefore, employing the easily accessible materials as substrates is still highly desirable for the production of β- and γ-amino alcohols.
In recent years, we have studied transfer hydrogenation reactions using N,N-iridium complexes as catalysts.23 Recently, Kuwata et al. developed an efficient asymmetric reductive amination of α-keto acids to access α-amino acids catalyzed by Cp*Ir catalysts bearing a chiral N-(2-picolyl)sulfonamidato ligand.24 Very recently, we also reported the protocol for N-aryl-substituted pyrrolidine synthesis via Ir-catalyzed successive reductive amination of diketones (Scheme 2a).25 Based on the successful formation of five-membered pyrrolidines with 2,5-diketones as substrates, the construction of four-membered or even the three-membered heterocyclic ring was also designed using 2,4-diketones or 2,3-diketones as substrates. However, this only produced β- and γ-amino alcohols, and no desired cyclic products were formed under standard conditions. Herein, we report on an Ir-catalyzed reductive amination and transfer hydrogenation of 2,4-diketones or 2,3-diketones to access β- and γ-amino alcohols (Scheme 2b). Various 2,4-diketones or 2,3-diketones and aromatic amines could be employed as substrates in this catalytic system, delivering the desired amino alcohols in moderate to excellent yields. This Ir-catalyzed reductive amination and transfer hydrogenation process offers an alternative protocol for β-and γ-amino alcohol synthesis.
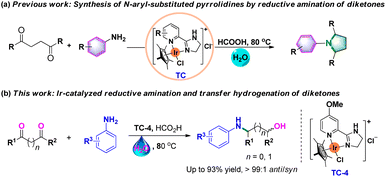 |
| Scheme 2 Ir-catalyzed difunctionalization of diketones. | |
We initiated our attempts at this Ir-catalyzed reductive amination and transfer hydrogenation of 2,3-butanedione (1a) with aniline (2a) as model substrates (Table 1). Preliminary reaction condition screens with TC-1–TC-6 as catalysts and 20.0 equivalent of HCO2H as the hydrogen donor in the presence of toluene afforded the β-amino alcohol 3aa in low yields (Table 1, entries 1–6). Based on our previous work21,22 showing that the reaction media would enhance the solubility of iridium complexes, we then investigated the influence of solvents (Table 1, entries 7–12). As anticipated, an increased yield of 80% was attained in the presence of H2O (Table 1, entry 12). To afford the optimal conditions, different quantities of HCO2H and other reaction times were further explored (Table 1, entries 13–19). Indeed, increasing the loading of HCO2H and prolonging the reaction time furnished the best yield of 93% (Table 1, entry 19). Decreasing the reaction temperature showed reduced performance (Table 1, entries 20–22). Control studies confirmed the necessity of the iridium complex and HCO2H for this reductive amination and transfer hydrogenation transformation (Table 1, entries 23 and 24).
Table 1 Optimization of the reaction conditionsa
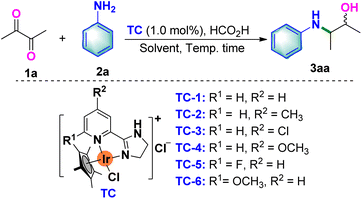
|
Entry |
Catalyst |
Solvent |
T (°C) |
HCO2H (equiv.) |
Time (h) |
Yieldb (%) |
Reaction conditions: a mixture of 1a (0.5 mmol, 1.0 equiv.), 2a (0.6 mmol, 1.1 equiv.), TC catalyst (1.0 mol%), HCO2H, and solvent (2.0 mL) was sealed in a 25.0 mL Schlenk tube under air. Yield was determined by NMR with dimethyl terephthalate as internal standard. Parenthesis is isolated yield based on 1a. |
1 |
TC-1 |
Toluene |
80 |
20 |
6 |
10 |
2 |
TC-2 |
Toluene |
80 |
20 |
6 |
11 |
3 |
TC-3 |
Toluene |
80 |
20 |
6 |
3 |
4 |
TC-4 |
Toluene |
80 |
20 |
6 |
15 |
5 |
TC-5 |
Toluene |
80 |
20 |
6 |
14 |
6 |
TC-6 |
Toluene |
80 |
20 |
6 |
4 |
7 |
TC-4 |
DMF |
80 |
20 |
6 |
27 |
8 |
TC-4 |
1,4-Dioxane |
80 |
20 |
6 |
n.d. |
9 |
TC-4 |
THF |
80 |
20 |
6 |
n.d |
10 |
TC-4 |
MeOH |
80 |
20 |
6 |
54 |
11 |
TC-4 |
Acetone |
80 |
20 |
6 |
n.d |
12 |
TC-4 |
H2O |
80 |
20 |
6 |
80 |
13 |
TC-4 |
H2O |
80 |
5 |
6 |
45 |
14 |
TC-4 |
H2O |
80 |
10 |
6 |
54 |
15 |
TC-4 |
H2O |
80 |
15 |
6 |
72 |
16 |
TC-4 |
H2O |
80 |
25 |
6 |
83 |
17 |
TC-4 |
H2O |
80 |
30 |
6 |
83 |
18 |
TC-4 |
H2O |
80 |
25 |
9 |
90 |
19 |
TC-4 |
H2O |
80 |
25 |
12 |
99 (93%)c |
20 |
TC-4 |
H2O |
rt |
25 |
12 |
87 |
21 |
TC-4 |
H2O |
60 |
25 |
12 |
97 |
22 |
TC-4 |
H2O |
100 |
25 |
12 |
99 |
23 |
— |
H2O |
80 |
25 |
12 |
— |
24 |
TC-4 |
H2O |
80 |
— |
12 |
— |
With the optimized reaction conditions in hand, we next probed the generality of the substrate scope. As showed in Table 2, 2,3-butanedione (1a) was capable of reductive amination with electron-withdrawing and electron-donating para-substituted aromatic amines (2b–2f) and transfer hydrogenation under standard conditions, furnishing the β-amino alcohol products 3ba–3fa in moderate yields and stereoselectivities. Meta-, ortho-, and di-substituted aromatic amines (2g–2i) also participated, producing the corresponding β-amino alcohols 3ga–3ia in similar moderate yields and stereoselectivities. Large block amines such as naphthylamine (2j), 4-cyclohexyl aniline (2k), and 5,6,7,8-tetrahydronaphthalen-2-amine (2l) were also tolerated in this catalytic system, providing the desirable reductive amination and transfer hydrogenation products of 3ja–3la. On the other hand, the more steric hindrance of 2,3-hexanedione (1b) gave same moderate yield and stereoselectivity of the corresponding product (3ab). Interestingly, aromatic diketone of 1-phenylpropane-1,2-dione (1c) was also a successful substrate, and showed improved stereoselectivity (anti/syn > 99
:
1) and excellent regioselectivity.
Table 2 Substrate scope of the Ir-catalyzed reductive amination and transfer hydrogenation of 2,3-diketones with aromatic aminesa,b,c
Reaction conditions: a mixture of 1 (0.5 mmol, 1.0 equiv.), 2 (0.6 mmol, 1.1 equiv.), TC-4 (1.0 mol%), HCO2H (12.5 mmol, 25.0 equiv.), and H2O (2.0 mL) were sealed in a 25.0 mL Schlenk tube under air at 80 °C for 12 h. Isolated yield based on 1. The value of the anti/syn ratio. |
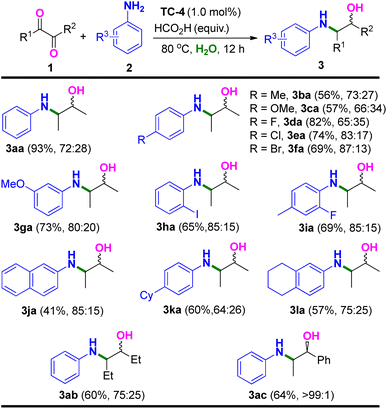 |
We next investigated the substrate scope with respect to the 1,3-diketones with aromatic amines (Table 3). The γ-amino alcohol of 4-(phenylamino)pentan-2-ol could also be prepared through this method (75% yield) using pentane-2,4-dione (4a) as the substrate. Moreover, para-substituted aromatic amines (2b–2f) could also be used in the reaction. However, products of 5da–5fa were formed in lower yields (32–42%) when the para-substituted aromatic amines bearing electron-withdrawing groups were used (2d–2f). A low yield was also observed for a meta-benzyl substituted aniline (2m). Pleasingly, 62% yield and moderate stereoselectivity of reductive amination and transfer hydrogenation product 5na were provided when 2,3-dihydro-1H-inden-5-amine (2n) was used in this reaction. Other aromatic and sterically more hindered diketones were also tolerated to produce 5ab and 5ac in moderate yields and excellent stereoselectivity (>99
:
1).
Table 3 Substrate scope of Ir-catalyzed reductive amination and transfer hydrogenation of 2,4-diketones with aromatic aminesa,b,c
Reaction conditions: a mixture of 4 (0.5 mmol, 1.0 equiv.), 2 (0.6 mmol, 1.1 equiv.), TC-4 (1.0 mol%), HCO2H (12.5 mmol, 25.0 equiv.), and H2O (2.0 mL) were sealed in a 25.0 mL Schlenk tube under air at 80 °C for 12 h. Isolated yield based on 4. The value of the anti/syn ratio. |
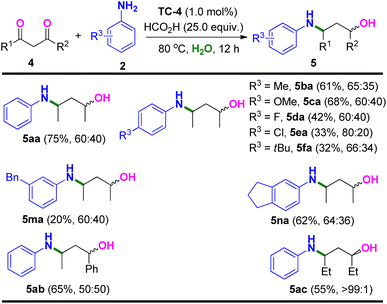 |
The robustness of this Ir-catalyzed reductive amination and transfer hydrogenation transformation was documented by performing the model reaction on a larger scale. As showcased in Scheme 3, 1.37 g of the product 3aa was afforded in 83% yield when 2,3-butanedione (1a) was loaded at a 10.0 mmol scale under standard conditions. With this successful large-scale performance, the follow-up asymmetric studies using chiral iridium complexes are underway and will be reported soon. In addition, the asymmetric Ir-catalyzed reduction amination of ketones with the model reaction was investigated under the optimized conditions (Scheme S1, ESI†). The desired reductive product 3aa was obtained using the chiral iridium complexes C1–C6 as catalysts, while a low enantioselectivity of the product was observed. The design and synthesis of more chiral iridium complexes are underway, and will be further applied in the asymmetric synthesis.
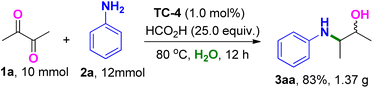 |
| Scheme 3 Large-scale synthesis of 3aa. | |
The proposed catalytic cycle of this Ir-catalyzed reductive amination and transfer hydrogenation is showcased in Scheme 4. On the one hand, the active Ir–H intermediate of Int-II was formed under the conditions of the iridium complex and HCO2H. On the other hand, the intermediate of imidone 6 was generated, which was subsequently reduced by Int-II to form the amino ketone 7. A more similar reduction of carbonyl by the active Ir–H was performed to furnish the desired product 5 and finish the catalytic cycle. Of note, it is possible that the transfer hydrogenation of the carbonyl group took place first, followed by the reductive amination process.
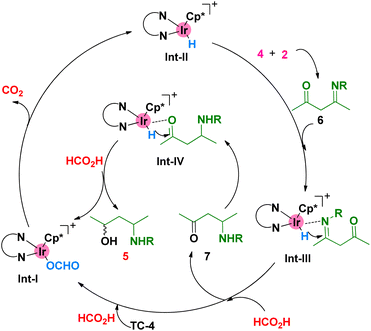 |
| Scheme 4 Proposed mechanism. | |
Conclusions
In summary, we report the reductive amination and transfer hydrogenation of diketones enabled by iridium complexes, facilitating access to diverse β- and γ-amino alcohols in moderate yields and stereoselectivities. The 2,3-diketones, 2,4-diketones and various substituted aromatic amines could be successfully employed in this system. The synthetic potential of this protocol was solidified by the large-scale performance.
Data availability
The data that support the findings of this study are available from the corresponding author upon reasonable request.
Conflicts of interest
There are no conflicts to declare.
Acknowledgements
This work was supported by the Fundamental Research Funds for Gannan Medical University (QD202019, QD202106, TD202310), and the Ganzhou Bureau of Science and Technology (2022-YB1402).
Notes and references
- P. Juszczyk, R. Kasprzykowska and A. S. Kołodziejczyk, Simple and efficient synthesis of chiral amino alcohols with an amino acid-based skeleton, Lett. Pept. Sci., 2003, 10, 79 CrossRef.
- J. L. Schwarz, R. Kleinmans, T. Paulisch and F. Glorius, 1,2-Amino alcohols via Cr/photoredox Dual-Catalyzed Addition of α-Amino Carbanion Equivalents to Carbonyls, J. Am. Chem. Soc., 2020, 142, 2168 CrossRef CAS PubMed.
-
(a) M. T. Reetz, M. W. Drewes and A. Schmitz, Stereoselective synthesis of β-amino alcohols from optically active α-amino acids, Angew. Chem., Int. Ed., 1987, 26, 1141 CrossRef;
(b) M. C. Wang, Z. K. Liu, S. Li, X. Ding, Y. Li and M. S. Tang, An experimental and theoretical study on free ligand conformational preferences and enantioselectivity relationship for the asymmetric addition of diethylzinc to benzaldehyde, Tetrahedron: Asymmetry, 2010, 21, 486 CrossRef CAS.
-
(a) C. E. O'Connell, K. A. Salvato, Z. Meng, B. A. Littlefield and C. E. Schwartz, Synthesis and evaluation of hapalosin and analogs as MDR-reversing agents, Bioorg. Med. Chem. Lett., 1999, 9, 1541 CrossRef PubMed;
(b) S. C. Bergmeier and D. M. Stanchina, Acylnitrene route to vicinal amino alcohols. Application to the synthesis of (−)-bestatin and analogues, J. Org. Chem., 1999, 64, 2852 CrossRef CAS PubMed;
(c) A. W. He and J. G. Cory, p53-independent anisomycin induced G1 arrest and apoptosis in L1210 cell lines, Anticancer Res., 1999, 19, 421 CAS;
(d) J. B. H. Tok and R. R. Rando, Simple aminols as aminoglycoside surrogates, J. Am. Chem. Soc., 1998, 120, 8279 CrossRef CAS;
(e) B. D. Feske, Bestatin: three decades of synthetic strategies, Curr. Org. Chem., 2007, 11, 483 CrossRef CAS;
(f) J. P. Michael, Indolizidine and quinolizidine alkaloids, Nat. Prod. Rep., 2008, 25, 139 RSC.
- E. Mai and C. Schneider, Scandium-bipyridine-catalyzed enantioselective aminolysis of meso-epoxides, Chem.–Eur. J., 2007, 13, 2729 CrossRef CAS PubMed.
- K. Arai, M. Salter, Y. Yamashita and S. Kobayashi, Enantioselective desymmetrization of meso epoxides with anilines catalyzed by a niobium complex of a chiral multidentate binol derivative, Angew. Chem., Int. Ed., 2007, 46, 955 CrossRef CAS PubMed.
- C. Wang and H. Yamamoto, Gadolinium-catalyzed regio-and enantioselective aminolysis of aromatic trans-2,3-epoxy sulfonamides, Angew. Chem., Int. Ed., 2015, 54, 8760 CrossRef CAS PubMed.
- R. Tak, M. Kumar, T. Menapara, M. K. Choudhary, R. I. Kureshy and N. U. H. Khan, Asymmetric catalytic syntheses of pharmaceutically important β-amino-α-hydroxyl esters by enantioselective aminolysis of methyl phenylglycidate, ChemCatChem, 2017, 9, 322 CrossRef CAS.
- M. Kumar, R. I. Kureshy, S. Saravanan, S. Verma, A. Jakhar, N. U. H. Khan and H. C. Bajaj, Unravelling a new class of chiral organocatalyst for asymmetric ring-opening reaction of meso epoxides with anilines, Org. Lett., 2014, 16, 2798 CrossRef CAS PubMed.
- J. H. Xie, S. Liu, W. L. Kong, W. J. Bai, X. C. Wang, L. X. Wang and Q. L. Zhou, Highly enantioselective and diastereoselective synthesis of chiral amino alcohols by ruthenium-catalyzed asymmetric hydrogenation of α-amino aliphatic ketones, J. Am. Chem. Soc., 2009, 131, 4222 CrossRef CAS PubMed.
- Y. Shi, Y. Wang, X. Lu, Y. Zhang, Y. Wu and F. Zhong, Rhodium-catalyzed aminohydroxylation of unactivated alkenes in aqueous media for the benign synthesis of 1, 2-amino alcohols, Green Chem., 2019, 21, 780 RSC.
- R. Spaccini, A. Ghilardi, N. Pastori, A. Clerici, C. Puna and O. Porta, Efficient radical domino approach to β-aminoalcohols from arylamines and alcohols triggered by Ti (III)/t-BuOOH, Tetrahedron, 2010, 66, 2044 CrossRef CAS.
- C. Wang, C. Chen, J. Han, J. Zhang, Y. Yao and Y. Zhao, Insight into O2-promoted
base-catalyzed N-alkylation of amines with alcohols, Eur. J. Org Chem., 2015, 2015, 2972–2977 CrossRef CAS.
-
(a) S. Sakuraba and K. Achiwa, Practical asymmetric synthesis of (R)-Fluoxetine hydrochloride catalyzed by (2S,4S)-4-dicyclohexylphosphino-2-diphenylphos-phinomethyl-1-(N-methyl carbamoyl) pyrrolidine-Rhodium complex1, Synlett, 1991, 1991, 689 CrossRef;
(b) S. Sakuraba and K. Achiwa, Efficient asymmetric hydrogenation of β-and γ-amino ketone derivatives leading to practical synthesis of fluoxetine and eprozinol, Chem. Pharm. Bull., 1995, 43, 748 CrossRef CAS;
(c) D. Liu, W. Gao, C. Wang and X. Zhang, Practical synthesis of enantiopure γ-amino alcohols by rhodium-catalyzed asymmetric hydrogenation of β-secondary-amino ketones, Angew. Chem., Int. Ed., 2005, 117, 1715 CrossRef;
(d) H. Geng, X. Zhang, M. Chang, L. Zhou, W. Wu and X. Zhang, Ruthenium-catalyzed asymmetric hydrogenation of β-keto-enamines: An efficient approach to chiral γ-amino alcohols, Adv. Synth. Catal., 2011, 353, 3039 CrossRef CAS;
(e) J. Wang, D. Liu, Y. Liu and W. Zhang, Asymmetric hydrogenation of β-amino ketones with the bimetallic complex RuPHOX-Ru as the chiral catalyst, Org. Biomol. Chem., 2013, 11, 3855 RSC.
-
(a) J. N. Zhou, Q. Fang, Y. H. Hu, L. Y. Yang, F. F. Wu, L. J. Xie and S. Li, Copper (II)-catalyzed enantioselective hydrosilylation of halo-substituted alkyl aryl and heteroaryl ketones: asymmetric synthesis of (R)-fluoxetine and (S)-duloxetine, Org. Biomol. Chem., 2014, 12, 1009 RSC;
(b) A. Träff, R. Lihammar and J. E. Bäckvall, A chemoenzymatic dynamic kinetic resolution approach to enantiomerically pure (R)-and (S)-duloxetine, J. Org. Chem., 2011, 76, 3917 CrossRef PubMed;
(c) Y. Gao and K. B. Sharpless, Asymmetric synthesis of both enantiomers of tomoxetine and fluoxetine. Selective reduction of 2, 3-epoxycinnamyl alcohol with Red-Al, J. Org. Chem., 1988, 53, 4081 CrossRef CAS;
(d) H. Kakei, T. Nemoto, T. Ohshima and M. Shibasaki, Efficient synthesis of chiral α-and β-hydroxy amides: Application to the synthesis of (R)-Fluoxetine, Angew. Chem., Int. Ed., 2004, 43, 317 CrossRef CAS PubMed;
(e) Y. Fujima, M. Ikunaka, T. Inoue and J. Matsumoto, Synthesis of (S)-3-(N-Methylamino)-1-(2-thienyl) propan-1-ol: revisiting Eli Lilly's resolution-racemization-recycle synthesis of duloxetine for its robust processes, Org. Process Res. Dev., 2006, 10, 905 CrossRef CAS;
(f) D. W. Robertson, J. H. Krushinski, R. W. Fuller and J. D. Leander, The absolute configurations and pharmacological activities of the optical isomers of fluoxetine, a selective serotonin-uptake inhibitor, J. Med. Chem., 1988, 31, 1412 CrossRef CAS PubMed.
- J. Wang, Y. Wang, D. Liu and W. Zhang, Asymmetric hydrogenation of β-secondary amino ketones catalyzed by a ruthenocenyl phosphino-oxazoline-ruthenium complex (RuPHOX-Ru): the Synthesis of γ-secondary amino alcohols, Adv. Synth. Catal., 2015, 357, 3262 CrossRef CAS.
- L. Wu, R. Jin, L. Li, X. Hu, T. Cheng and G. Liu, A Michael addition-asymmetric transfer hydrogenation one-pot enantioselective tandem process for syntheses of chiral γ-secondary amino alcohols, Org. Lett., 2017, 19, 3047 CrossRef CAS PubMed.
- F. Li, L. Long, Y. M. He, Z. Li, H. Chen and Q. H. Fan, Manganese-catalyzed asymmetric formal hydroamination of allylic alcohols: A remarkable macrocyclic ligand effect, Angew. Chem., Int. Ed., 2022, 134, e202202972 CrossRef.
- T. J. Donohoe, C. K. Callens, A. Flores, A. R. Lacy and A. H. Rathi, Recent developments in methodology for the direct oxyamination of olefins, Chem.–Eur. J., 2011, 17, 58 CrossRef CAS PubMed.
- O. K. Karjalainen and A. M. P. Koskinen, Diastereoselective synthesis of vicinal amino alcohols, Org. Biomol. Chem., 2012, 10, 4311 RSC.
- K. Li, X. Shao, L. Tseng and S. J. Malcolmson, 2-Azadienes as reagents for preparing chiral amines: Synthesis of 1,2-amino tertiary alcohols by Cu-catalyzed enantioselective reductive couplings with ketones, J. Am. Chem. Soc., 2018, 140, 598 CrossRef CAS PubMed.
- K. Spielmann, M. Xiang, L. A. Schwartz and M. J. Krische, Direct conversion of primary alcohols to 1,2-amino alcohols: Enantioselective Iridium-catalyzed carbonyl reductive coupling of phthalimido-allene via hydrogen auto-transfer, J. Am. Chem. Soc., 2019, 141, 14136 CrossRef CAS PubMed.
-
(a) L. Ouyang, Y. Liang, S. Wang, J. Liao and R. Luo, Access of arylmethanes via iridium-catalyzed deoxygenative cross-coupling of aryl ketones with anilines/phenols, J. Catal., 2024, 433, 115492 CrossRef CAS;
(b) R. Luo, S. Wang, Y. Liang, J. Tong, J. Liao and L. Ouyang, Ir-catalyzed selective reductive N-formylation and transfer hydrogenation of N-heteroarenes, Organometallics, 2024, 43, 2097 CrossRef CAS;
(c) L. Ouyang, R. Miao, Z. Yang and R. Luo, Iridium-catalyzed reductive amination of carboxylic acids, J. Catal., 2023, 418, 283 CrossRef CAS;
(d) Y. Wei, Y. Liang, R. Luo and L. Ouyang, Recent advances of Cp*Ir complexes for transfer hydrogenation: focus on formic acid/formate as hydrogen donors, Org. Biomol. Chem., 2023, 21, 7484 RSC;
(e) Y. Xia, S. Wang, R. Miao, J. Liao, L. Ouyang and R. Luo, Synthesis of N-alkoxy amines and hydroxylamines via the Iridium-catalyzed transfer hydrogenation of oximes, Org. Biomol. Chem., 2022, 20, 6394 RSC;
(f) L. Ouyang, Y. Xia, R. Miao, J. Liao and R. Luo, Iridium-catalyzed reductive etherification of α,β-unsaturated ketones and aldehydes with alcohols, Org. Biomol. Chem., 2022, 20, 2621 RSC;
(g) N. Luo, Y. Zhong, H. Shui and R. Luo, pH-Mediated selective synthesis of N-allylic alkylation or N-alkylation amines with allylic alcohols via an Iridium catalyst in water, J. Org. Chem., 2021, 86, 15509 CrossRef CAS PubMed;
(h) H. Wen, N. Luo, Q. Zhu and R. Luo, Amide iridium complexes as catalysts for transfer hydrogenation reduction of N-sulfonylimine, J. Org. Chem., 2021, 86(5), 3850 CrossRef CAS PubMed.
- T. Yajima, A. Katayama, T. Ito, T. Kawada, K. Yabushita, T. Yasuda, T. Ohta, T. Katayama, N. Utsumi, Y. Kayaki and S. Kuwata, Asymmetric reductive amination of α-keto acids using Ir-based hydrogen transfer catalysts: An access to unprotected unnatural α-amino acids, Org. Lett., 2024, 26, 1426 CrossRef CAS PubMed.
- J. Liao, J. Tong, L. Liu, L. Ouyang and R. Luo, Construction of N-aryl-substituted pyrrolidines by successive reductive amination of diketones via transfer hydrogenation, Molecules, 2024, 29, 2565 CrossRef CAS PubMed.
|
This journal is © The Royal Society of Chemistry 2024 |
Click here to see how this site uses Cookies. View our privacy policy here.