DOI:
10.1039/D3SC06600B
(Edge Article)
Chem. Sci., 2024,
15, 3249-3254
Borylated cyclobutanes via thermal [2 + 2]-cycloaddition†
Received
8th December 2023
, Accepted 17th January 2024
First published on 25th January 2024
Abstract
A one-step approach to borylated cyclobutanes from amides of carboxylic acids and vinyl boronates is elaborated. The reaction proceeds via the thermal [2 + 2]-cycloaddition of in situ-generated keteniminium salts.
Introduction
Small aliphatic rings attract considerable attention in contemporary research.1 For example, the cyclobutane ring is common within modern bioactive compounds2 and can be found in the structures of at least ten market-approved drugs.3 This motivated substantial development of cyclobutyl boronate chemistry during the past decade owing to the fact that the carbon–boron bond provides an excellent site for functionalization.4 The known approaches to the preparation of cyclobutyl boronates include the C–H activation of cyclobutanes,5 electrocyclization,6 functionalization of bicyclo[1.1.0]butanes,7 borylation8 and hydrogenation of cyclobutenes,9 along with other methods.10,11
The most frequently used approach is a [2 + 2]-cycloaddition. Strikingly, while the photochemical version of this transformation has been elaborated in numerous studies by Hollis, Bach, Hiemstra, Grygorenko, Yoon, Swierk with Brown, Romanov-Michailidis with Knowles, and Fürstner,12 the thermal approach remained underdeveloped for unclear reasons (Scheme 1). We found only a single example in the literature on non-catalyzed thermal [2 + 2]-cycloaddition between alkene 1 and ketene 2 (Scheme 1). In 1969, Fish demonstrated that heating this mixture in a sealed vial for 15 days afforded the target cyclobutane 3 in 23% yield.13 Also, recently Brown showed an example of a thermal [2 + 2]-cycloaddition between a borylated alkene and an allene that required, however, Lewis acid catalysis.14
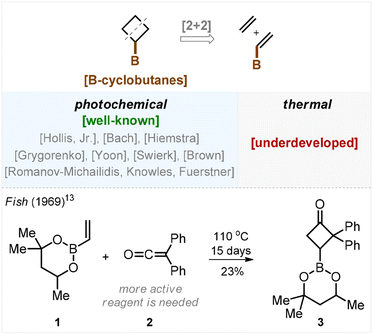 |
| Scheme 1 Retrosynthetic disconnection of borylated cyclobutanes via a [2 + 2]-reaction: photochemical vs. thermal strategies. | |
In this work, we developed a one-step approach to borylated cyclobutanes by thermal [2 + 2]-cycloaddition between vinyl boronates and in situ-generated keteniminium salts.
Results and discussion
From the pioneering study of Fish (Scheme 1),13 it seemed reasonable to assume that vinyl boronates are amenable to non-catalytic thermal [2 + 2] cycloadditions; however, a more active partner than a ketene was needed. We turned our attention to keteniminium salts that are known to be more active than ketenes.15 Moreover, the [2 + 2]-cycloaddition of keteniminium salts with alkenes has been reported.16,17 Despite the substantial recent progress in keteniminium chemistry,15 we found no literature mentioning the reaction of borylated alkenes with keteniminium salts. Initially, we suspected that the conditions for their generation, which typically involved treatment with triflic anhydride,17 do not tolerate the Bpin group and the latter might decompose. Nonetheless, we decided to examine the feasibility of this transformation.
The model reaction between N,N-dimethylacetamide and vinyl Bpin did not produce the desired product 4 even at trace amounts when performed in refluxed dichloroethane. Lowering the temperature to 60 °C or increasing the number of keteniminium equivalents from 1.2 to 3, 4, and 5 were as unsuccessful (Scheme 2, part limitations). Only the starting vinyl Bpin along with unidentified side products was detected in the reaction mixture. We were quite discouraged by the futility of our initial efforts, yet we attempted another reaction involving the homologous N,N-dimethylpropanamide. Serendipitously, the reaction worked out. Activation of the amide with (CF3SO2)2O/collidine (in situ formation of the keteniminium salt) followed by its reaction with vinyl Bpin produced the desired product 5 (Scheme 2).
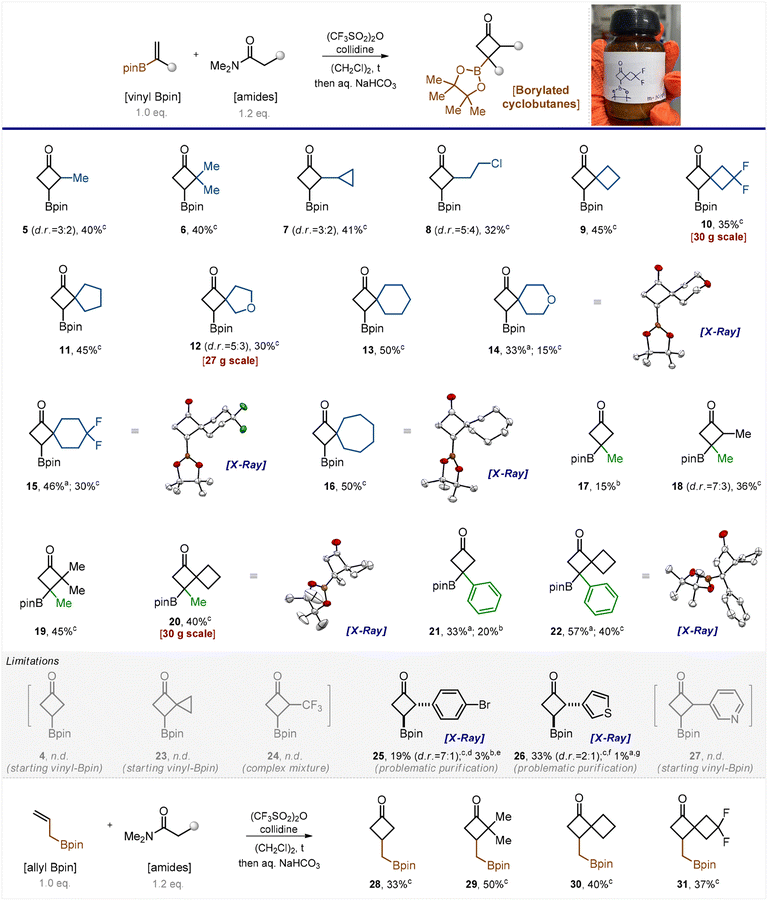 |
| Scheme 2 Reaction conditions: (i) vinyl Bpin/allyl Bpin (1.0 equiv.), amide (1.2 equiv.), triflic anhydride (1.4 equiv.), collidine or lutidine (1.4 equiv.), 1,2-dichloroethane, reflux, and 16 h; (ii) aqueous NaHCO3; (iii) purification (vacuum distillation or column chromatography). The scale of the synthesis: a 100–500 mg; b 1–7 g; c 10–50 g of the isolated product. d Product 25 (d.r. = 7 : 1) was obtained with ca. 70% purity. e Additional purification by column chromatography provided the pure product 25 as a single diastereomer in 3% yield. f Product 26 (d.r. = 2 : 1) was obtained with ca. 50% purity. g Additional purification by column chromatography provided the pure product 26 as a single diastereomer in 1% yield. X-ray crystal structures of compounds 14–16, 20, and 22 are shown as thermal ellipsoids at a 50% probability level; carbon – white, oxygen – red, boron – brown, and fluorine – green; hydrogen atoms are omitted for clarity. | |
After short optimization of the reaction conditions, we found that performing the reaction in refluxing dichloroethane for 12 hours produced excellent conversion of the starting vinyl boronate (see Table S1 in the ESI†). Thus, we examined the reaction scope by taking various amide counterparts and obtained borylated cyclobutanes 5–16 in decent yields (Scheme 2). The reaction was found compatible with the presence of the cyclopropyl ring (7), the active chlorine atom (8), and the gem-difluoro motif (10 and 15) in the product substances. Substituted alkenes, CH2
C(Me)-Bpin and CH2
C(Ph)-Bpin, gave the desired borylated cyclobutanes 17–22 as well. Products 5, 7, 8, 12, and 18 were obtained as inseparable mixtures of two diastereomers. The structure of products 14–16, 20 and 22 was confirmed by X-ray crystallographic analysis.18
The developed reaction showed few limitations, however. The keteniminium salt obtained from N,N-dimethylacetamide reacted with substituted vinyl boronates (products 17 and 21) but failed to react with vinyl Bpin (4). Attempts towards the synthesis of compounds 23, 24 and 27 failed as well. Analysis of the reaction mixture revealed either the presence of unreacted vinyl Bpin (23, 27) or the formation of a complex mixture (24). Some products, such as 25 and 26, were obtained in low isolated yields because they required rather tedious purification. The purification led to isolation of a single diastereomer in each case, and the trans-configuration of compounds 25 and 26 was revealed by X-ray crystallography.18
The analogous reaction between amides of carboxylic acids and the homologous CH2
CH–CH2-Bpin also produced the desired products 28–31 (Scheme 2).
It is important to note that the reaction demonstrated good performance on milligram, gram, and even multigram scales (10, 12, and 20). When carrying out the reaction on a small scale, we purified products by silica gel column chromatography. On a gram-to-multigram scale, we isolated the products by distillation under reduced pressure, which is more practical. Despite the seeming simplicity of the current approach to borylated cyclobutanes, to the best of our knowledge, none of the obtained products depicted in Scheme 2 has been reported in the literature.
Somewhat unexpectedly, the reaction between β,β-disubstituted vinyl boronate 32 and N,N-dimethylacetamide produced ketone 33 rather than the borylated cyclobutane 34 (Scheme 3). While we did not examine the exact mechanism of this transformation,19 we found a fairly reasonable explanation for the observed outcome. We reasoned that the bulky C
NMe2 moiety approached the tertiary rather than quaternary carbon of the amide in the course of the cycloaddition probably due to steric reasons (Scheme 3, proposed explanation). Effectively, this steered the reaction towards the formation of the intermediate compound 35, which is related to the class of unstable α-borylated ketones20 prone to hydrolytic protodeborylation, thus producing ketone 33.
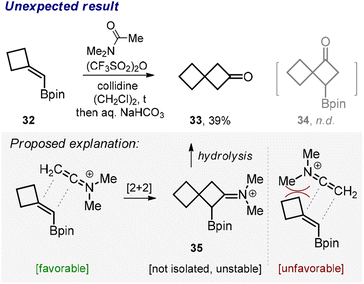 |
| Scheme 3 Unexpected synthesis of ketone 33. | |
Next, we performed transformations of the obtained products. For example, despite the failed attempt to direct synthesis of cyclobutane 23 from amide 36 (Scheme 2, limitations), we were able to obtain compound 23 by an intramolecular cyclization of the previously synthesized chloride 8 in 84% yield (Scheme 4a). From ketone 6, the corresponding amino boronate 37 was synthesized in two steps (Scheme 4b). The subsequent N-protection provided N-Boc amino boronate 38, which represents a useful medicinal chemistry precursor. Analogously, the N-Boc amino boronate 40 was obtained from ketone 9via amine 39.
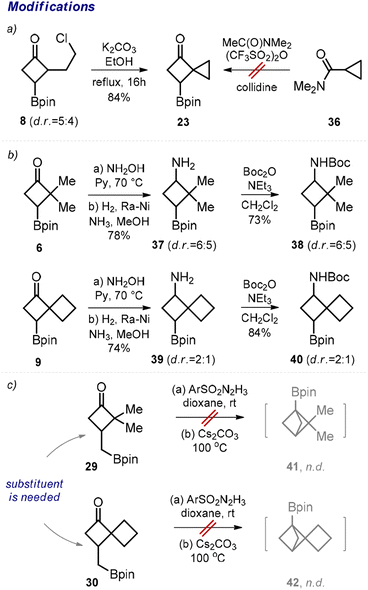 |
| Scheme 4 Modifications of borylated cyclobutanes. (a) Synthesis of borylated cyclobutane 23. (b) Synthesis of N-Boc amino boronates 38 and 40. (c) An attempted synthesis of bicyclo[1.1.1]pentanes 41 and 42. | |
In 2021, Qin and colleagues developed an elegant intramolecular coupling towards multi-substituted bicycloalkyl boronates.11a The corresponding starting materials were synthesized in multiple steps. In this work, we obtained ketones 29 and 30 in one step, and we also attempted their cyclization into the desired bicyclo[1.1.1]pentanes 41 and 42 (Scheme 4c). Unfortunately, under the original conditions reported by the Qin group, the formation of the desired products was not observed. Our unsuccessful efforts corroborate the seminal conclusion that the presence of an additional substituent at the cyclobutane ring is crucial for the formation of bicyclo[1.1.1]pentane in this reaction.11a
Finally, we performed a few other representative modifications. The reaction of pinacol boronates 6, 9, and 13 with KHF2 produced potassium trifluoroborates 6a, 9a, and 13a (Scheme 5). The reduction of the ketone group in 13 with NaBH4 gave alcohol 43. The oxidative cleavage of the Bpin group in the latter substance gave diol 44. We assume that similar modifications could be performed with the other obtained Bpin ketones following our protocols.
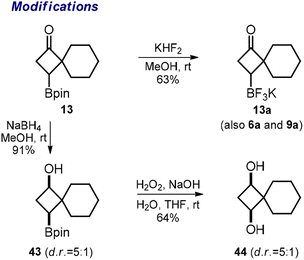 |
| Scheme 5 Synthesis of potassium trifluoroborate salts 6a, 9a, and 13; and diol 44. | |
Conclusions
Here, we elaborated a thermal [2 + 2]-cycloaddition between vinyl boronates and in situ generated keteniminium salts. This practical approach allows the preparation of borylated cyclobutanes in one step. The obtained compounds can be used in the syntheses of various functionalized cyclobutanes.
Data availability
The ESI† contains method description, product characterization data, and NMR spectra.
Author contributions
O. P. D. and P. K. M. designed the project. K. P., O. P., S. S., K. G., and O. P. D. carried out experiments. V. K. analysed the data. V. K. and P. K. M. wrote the manuscript and all authors provided comments.
Conflicts of interest
The authors are employees of a chemical supplier Enamine.
Acknowledgements
The authors are grateful to Prof. A. A. Tolmachov for the support and to Dr S. Shishkina for the X-Ray analysis. This project has received funding from the European Research Council (ERC) under the European Union's Horizon 2020 research and innovation program (grant agreement No. 101000893 – BENOVELTY).
Notes and references
- M. R. Bauer, P. Di Fruscia, S. C. C. Lucas, I. N. Michaelides, J. E. Nelson, R. I. Storer and B. C. Whitehurst, Put a ring on it: application of small aliphatic rings in medicinal chemistry, RSC Med. Chem., 2021, 12, 448–471 RSC.
- M. R. van der Kolk, M. A. C. H. Janssen, F. P. J. T. Rutjes and D. Blanco-Ania, Cyclobutanes in Small-Molecule Drug Candidates, ChemMedChem, 2022, 17, e202200020 CrossRef CAS PubMed.
-
Chemical structure search drugbank online, https://go.drugbank.com/structures/search/small_molecule_drugs/structure, accessed December 2023, filters used: “substucture”+“approved”+”vet approved”.
-
(a) R. J. Armstrong and V. Aggarwal, 50 Years of Zweifel Olefination: a Transition-Metal-Free Coupling, Synthesis, 2017, 49, 3323–3336 CrossRef CAS;
(b) C. Sandford and V. K. Aggarwal, Stereospecific Functionalizations and Transformations of Secondary and Tertiary Boronic Esters, Chem. Commun., 2017, 53, 5481–5494 RSC;
(c) J. W. B. Fyfe and A. J. B. Watson, Recent Developments in Organoboron Chemistry: Old Dogs, New Tricks, Chem, 2017, 3, 31–55 CrossRef CAS.
-
(a) J. He, Q. Shao, Q. Wu and J.-Q. Yu, Pd(II)-Catalyzed Enantioselective C(sp3)-H Borylation, J. Am. Chem. Soc., 2017, 139, 3344–3347 CrossRef CAS PubMed;
(b) R. Murakami, K. Tsunoda, T. Iwai and M. Sawamura, Stereoselective C-H Borylations of Cyclopropanes and Cyclobutanes with Silica-Supported Monophosphane-Ir Catalysts, Chem.–Eur. J., 2014, 20, 13127–13131 CrossRef CAS PubMed;
(c) X. Chen, L. Chen, H. Zhao, Q. Gao, Z. Shen and S. Xu, Iridium-Catalyzed Enantioselective C(sp3)–H Borylation of Cyclobutanes, Chin. J. Chem., 2020, 38, 1533–1537 CrossRef CAS;
(d) Q. Gao and S. Xu, Site- and Stereoselective C(sp3)−H Borylation of Strained (Hetero)Cycloalkanols Enabled by Iridium Catalysis, Angew. Chem., Int. Ed., 2023, 62, e202218025 CrossRef CAS PubMed.
- Z. X. Giustra, X. Yang, M. Chen, H. F. Bettinger and S.-Y. Liu, Accessing 1,2-Substituted Cyclobutanes Through 1,2-Azaborine Photoisomerization, Angew. Chem., Int. Ed., 2019, 58, 18918–18922 CrossRef CAS PubMed.
-
(a) M. Silvi and V. K. Aggarwal, Radical Addition to Strained σ-Bonds Enables the Stereocontrolled Synthesis of Cyclobutyl Boronic Esters, J. Am. Chem. Soc., 2019, 141, 9511–9515 CrossRef CAS PubMed;
(b) A. Fawcett, T. Biberger and V. K. Aggarwal, Carbopalladation of C−C σ-Bonds Enabled by Strained Boronate Complexes, Nat. Chem., 2019, 11, 117–122 CrossRef CAS PubMed;
(c) J. Michalland, N. Casaretto and S. Z. Zard, A Modular Access to 1,2- and 1,3-Disubstituted Cyclobutylboronic Esters by Consecutive Radical Additions, Angew. Chem., Int. Ed., 2022, 61, e202113333 CrossRef CAS PubMed.
-
(a) L. Brener and H. C. Brown, Hydroboration. 47. Unique Stereospecificity of the Hydroboration of 1, 3-Dimethylcycloalkenes with 9-Borabicyclo [3.3.1] Nonane, J. Org. Chem., 1977, 42, 2702–2704 CrossRef CAS;
(b) M. Guisan-Ceinos, A. Parra, V. Martin-Heras and M. Tortosa, Enantioselective Synthesis of Cyclobutylboronates via a Copper-Catalyzed Desymmetrization Approach, Angew. Chem., Int. Ed., 2016, 55, 6969–6972 CrossRef CAS PubMed;
(c) H. A. Clement, M. Boghi, R. M. McDonald, L. Bernier, J. W. Coe, W. Farrell, C. J. Helal, M. R. Reese, N. W. Sach, J. C. Lee and D. G. Hall, High-Throughput Ligand Screening Enables the Enantioselective Conjugate Borylation of Cyclobutenones to Access Synthetically Versatile Tertiary Cyclobutylboronates, Angew. Chem., Int. Ed., 2019, 58, 18405–18409 CrossRef CAS PubMed;
(d) A. K. Simlandy, M.-Y. Lyu and M. K. Brown, Catalytic Arylboration of Spirocyclic Cyclobutenes: Rapid Access to Highly Substituted Spiro[3.n]alkanes, ACS Catal., 2021, 11, 12815–12820 CrossRef CAS PubMed;
(e) L. Nóvoa, L. Trulli, A. Parra and M. Tortosa, Stereoselective Diboration of Spirocyclobutenes: A Platform for the Synthesis of Spirocycles with Orthogonal Exit Vectors, Angew. Chem., Int. Ed., 2021, 60, 11763–11768 CrossRef PubMed;
(f) L. Novoa, L. Trulli, I. Fernandez, A. Parra and M. Tortosa, Regioselective Monoborylation of Spirocyclobutenes, Org. Lett., 2021, 23, 7434–7438 CrossRef CAS PubMed;
(g) J. Proessdorf, C. Jandl, T. Pickl and T. Bach, Synthesis of Boronates with a Protoilludane Skeleton, Synthesis, 2023, 55, 2311–2318 CrossRef CAS;
(h) M. Cui, Z.-Y. Zhao and M. Oestreich, Boosting the Enantioselectivity of Conjugate Borylation of α,β-Disubstituted Cyclobutenones with Monooxides of Chiral C2-Symmetric Bis(phosphine) Ligands, Chem.–Eur. J., 2022, 62, e202202163 CrossRef PubMed.
- M. M. Parsutkar, V. V. Pagar and T. V. RajanBabu, Catalytic Enantioselective Synthesis of Cyclobutenes From Alkynes and Alkenyl Derivatives, J. Am. Chem. Soc., 2019, 141, 15367–15377 CrossRef CAS PubMed.
-
(a) D. P. Hari, J. C. Abell, V. Fasano and V. K. Aggarwal, Ring-Expansion Induced 1,2-Metalate Rearrangements: Highly Diastereoselective Synthesis of Cyclobutyl Boronic Esters, J. Am. Chem. Soc., 2020, 142, 5515–5520 CrossRef CAS PubMed;
(b) T. R. McDonald and S. A. L. Rousseaux, Synthesis of 3-borylated cyclobutanols from epihalohydrins or epoxy alcohol derivatives, Chem. Sci., 2023, 14, 963–969 RSC;
(c) P. Dominguez-Molano, R. Weeks, R. J. Maza, J. J. Carbo and E. Fernández, Boron-Copper 1,3-Rearrangement: the New Concept Behind the Boryl Migration from C(sp2) in Alkenyl Boranes to C(sp3), Angew. Chem., Int. Ed., 2023, 62, e202304791 CrossRef CAS PubMed.
- Borylated bicyclo[1.1.1]pentanes:
(a) Y. Yang, J. Tsien, J. M. E. Hughes, B. K. Peters, R. R. Merchant and T. Qin, An intramolecular coupling approach to alkyl bioisosteres for the synthesis of multisubstituted bicycloalkyl boronates, Nat. Chem., 2021, 13, 950–955 CrossRef CAS PubMed;
(b) Y. Yang, J. Tsien, R. Dykstra, S.-J. Chen, J. B. Wang, R. R. Merchant, J. M. E. Hughes, B. K. Peters, O. Gutierrez and T. Qin, Programmable late-stage functionalization of bridge-substituted bicyclo[1.1.1]pentane bis-boronates, Nat. Chem., 2023 DOI:10.1038/s41557-023-01342-7;
(c) S. Kim, H. Oh, W. Dong, J. Majhi, M. Sharique, B. Matsuo, S. Keess and G. A. Molander, Metal-Free Photoinduced Acylboration of [1.1.1]Propellane via Energy Transfer Catalysis, ACS Catal., 2023, 13, 9542–9549 CrossRef CAS;
(d) W. Dong, E. Yen-Pon, L. Li, A. Bhattacharjee, A. Jolit and G. A. Molander, Exploiting the sp2 character of bicyclo[1.1.1]pentyl radicals in the transition-metal-free multi-component difunctionalization of [1.1.1]propellane, Nat. Chem., 2022, 14, 1068–1077 CrossRef CAS PubMed;
(e) I. F. Yu, J. L. Manske, A. Diéguez-Vázquez, A. Misale, A. E. Pashenko, P. K. Mykhailiuk, S. V. Ryabukhin, D. M. Volochnyuk and J. F. Hartwig, Catalytic undirected borylation of tertiary C–H bonds in bicyclo[1.1.1]pentanes and bicyclo[2.1.1]hexanes, Nat. Chem., 2023, 15, 685–693 CrossRef CAS PubMed.
-
(a) W. G. Hollis Jr., W. C. Lappenbusch, K. A. Everberg and C. M. Woleben, The Use of Alkenylboronate Esters in [2 + 2] Enone-Olefin Photocycloadditions, Tetrahedron Lett., 1993, 34, 7517–7520 CrossRef;
(b) S. C. Coote and T. Bach, Enantioselective Intermolecular [2 + 2] Photocycloadditions of Isoquinolone Mediated by a Chiral Hydrogen-Bonding Template, J. Am. Chem. Soc., 2013, 135, 14948–14951 CrossRef CAS PubMed;
(c) R. A. Kleinnijenhuis, B. J. J. Timmer, G. Lutteke, J. M. M. Smits, R. de Gelder, J. H. van Maarseveen and H. Hiemstra, Formal Synthesis of Solanoeclepin a: Enantioselective Allene Diboration and Intramolecular [2 + 2] Photocycloaddition for the Construction of the Tricyclic Core, Chem.–Eur. J., 2016, 22, 1266–1269 CrossRef CAS PubMed;
(d) O. P. Demchuk, O. V. Hryshchuk, B. V. Vashchenko, A. V. Kozytskiy, A. V. Tymtsunik, I. V. Komarov and O. O. Grygorenko, Photochemical [2 + 2] Cycloaddition of Alkenyl Boronic Derivatives: an Entry Into 3-Azabicyclo[3.2.0]Heptane Scaffold, J. Org. Chem., 2020, 85, 5927–5940 CrossRef CAS PubMed;
(e) S. O. Scholz, J. B. Kidd, L. Capaldo, N. E. Flikweert, R. M. Littlefield and T. P. Yoon, Construction of Complex Cyclobutane Building Blocks by Photosensitized [2 + 2] Cycloaddition of Vinyl Boronate Esters, Org. Lett., 2021, 23, 3496–3501 CrossRef CAS PubMed;
(f) Y. Liu, D. Ni, B. G. Stevenson, V. Tripathy, S. E. Braley, K. Raghavachari, J. R. Swierk and M. K. Brown, Photosensitized [2 + 2]-Cycloadditions of Alkenylboronates and Alkenes, Angew. Chem., Int. Ed., 2022, 61, e202200725 CrossRef CAS PubMed;
(g) P. R. D. Murray, W. M. M. Bussink, G. H. M. Davies, F. W. van der Mei, A. H. Antropow, J. T. Edwards, L. A. D'Agostino, J. M. Ellis, L. G. Hamann, F. Romanov-Michailidis and R. R. Knowles, Intermolecular Crossed [2+2] Cycloaddition Promoted by Visible-Light Triplet Photosensitization: Expedient Access to Polysubstituted 2-Oxaspiro[3.3]Heptanes, J. Am. Chem. Soc., 2021, 143, 4055–4063 CrossRef CAS PubMed;
(h) Y. Liu, D. Ni and M. K. Brown, Boronic Ester Enabled [2 + 2]-Cycloadditions by Temporary Coordination: Synthesis of Artochamin J and Piperarborenine B, J. Am. Chem. Soc., 2022, 144, 18790–18796 CrossRef CAS PubMed;
(i) S. M. Spohr and A. Fürstner, Studies toward Providencin: The Furanyl-Cyclobutanol Segment, Org. Lett., 2023, 25, 1536–1540 CrossRef CAS PubMed.
- R. H. Fish, The Cycloaddition of Diphenylketene to 2-Vinyl-4,6,6-trimethyl-1,3,2-dioxaborinane, J. Org. Chem., 1969, 34, 1127–1128 CrossRef CAS.
- M. L. Conner and M. K. Brown, Synthesis of 1,3-Substituted Cyclobutanes by Allenoate-Alkene [2 + 2] Cycloaddition, J. Org. Chem., 2016, 81, 8050–8060 CrossRef CAS PubMed.
-
(a) C. Madelaine, V. Valerio and N. Maulide, Revisiting Keteniminium Salts: More than the Nitrogen Analogs of Ketenes, Chem.–Asian J., 2011, 6, 2224–2239 CrossRef CAS PubMed;
(b) G. Evano, M. Lecomte, P. Thilmany and C. Theunissen, Keteniminium Ions: Unique and Versatile Reactive Intermediates for Chemical Synthesis, Synthesis, 2017, 49, 3183–3214 CrossRef CAS.
-
(a) J. Marchand-Brynaert and L. Ghosez, Cycloadditions of Keteneimmonium Cations to Olefins and Dienes. A new Synthesis of Four-Membered Rings, J. Am. Chem. Soc., 1972, 94, 2870–2872 CrossRef CAS;
(b) A. Sidani, J. Marchand-Brynaert and L. Ghosez, A Convenient Procedure for the Synthesis of Cyclobutanones, Angew. Chem., Int. Ed., 1974, 13, 267 CrossRef.
- J.-B. Falmagne, J. Escudero, S. Taleb-Sahraoui and L. Ghosez, Cyclobutanone and Cyclobutenone Derivatives by Reaction of Tertiary Amides with Alkenes or Alkynes, Angew. Chem., Int. Ed., 1981, 20, 879–880 CrossRef.
- Cambridge Crystallographic Data Centre (CCDC) deposition numbers: 2312008 (14), 2312007 (15), 2312010 (16), 2312009 (20), 2312011 (22), 2321052 (25), and 2321392 (26).
- H. Saimoto, C. Houge, A.-M. Hesbain-Frisque, A. Mockel and L. Ghosez, Nonstereospecificity in the cycloadditions of keteneiminium salts to olefins. Evidence for a stepwise mechanism, Tetrahedron Lett., 1983, 24, 2251–2254 CrossRef CAS.
- Z. He, A. Zajdlik and A. K. Yudin, α-Borylcarbonyl compounds: from transient intermediates to robust building blocks, Dalton Trans., 2014, 43, 11434–11451 RSC.
|
This journal is © The Royal Society of Chemistry 2024 |
Click here to see how this site uses Cookies. View our privacy policy here.