DOI:
10.1039/D4SC00522H
(Edge Article)
Chem. Sci., 2024,
15, 5211-5217
A multicomponent reaction for modular assembly of indole-fused heterocycles†
Received
24th January 2024
, Accepted 3rd March 2024
First published on 4th March 2024
Abstract
Indoles are privileged chemical entities in natural products and drug discovery. Indole-fused heterocycles, particularly seven-membered ones, have received increasing attention due to their distinctive chemical characteristics and wide spectrum of bioactivities. However, the synthetic access to these compounds is highly limited. Herein, we report a unique multicomponent reaction (MCR) for modular assembly of indole-fused seven-membered heterocycles. In this process, indole, formaldehyde and amino hydrochloride could assemble rapidly to yield indole-fused oxadiazepines, and another addition of sodium thiosulphate would furnish indole-fused thiadiazepines. The biological evaluation disclosed the promising anticancer activity of these compounds. Furthermore, this MCR could be applicable in the late-stage and selective modifications of peptides. Therefore, this work provides a powerful strategy for indole functionalization and valuable tool for construction of seven-membered heterocycles.
Introduction
Indole alkaloids are one of the largest classes of alkaloids in natural products. They possess diverse structural features and exhibit a wide spectrum of biological activities.1 Moreover, the indole moiety serves as a privileged structure scaffold in pharmaceutics and is present in a number of approved drugs.2 Given the prevalence of indole derivatives, the exploration of indole functionalization toward new scaffolds, chemical space expansion and new biological discoveries has gained considerable interest in chemistry and biology.3 In particular, indole-fused seven-membered heterocycles represent an important subunit of indole derivatives in pharmaceutics.4 For instance, indole-fused diazepine acts as a flexible core structure to fit differential targets in drug discovery, and there are a variety of candidates derived from this heterocycle, such as WAY-163909, serazapine, azepindole and staurosporine (Scheme 1A).5–8 Correspondingly, the synthesis approaches to these compounds attract much attention. Typically, Dinh reported a multi-step synthetic route and Greaney disclosed a Pd-catalyzed oxidative C–H coupling reaction to access these compounds (Scheme 1B).8,9a Besides, several alternative methods were also established.9 However, most of these methods rely on complex starting materials, tedious synthetic procedures and limited structural diversity, which very much hamper the chemical space exploration of indole-fused heterocycles.
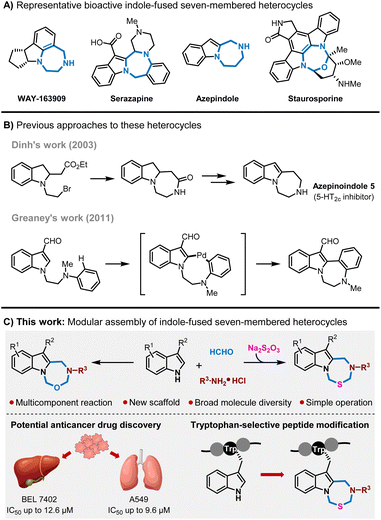 |
| Scheme 1 Indole-fused seven-membered heterocycles. | |
Multicomponent reactions (MCRs) are one-pot reactions that employ three or more reactants to form a single product which is incorporated with almost all the atoms from starting materials.10 MCRs have emerged as a powerful tool in chemistry and biology research, and have also demonstrated success in the construction of new chemical entities.11 With respect to indoles in MCRs, the current protocols mainly rely on the nucleophilicity of 3-position of indoles, while indole-based MCRs with new functionalization fashions and reaction modes for construction of new scaffolds remain to be investigated.12 Very recently, our group developed a series of Mannich-like MCRs of benzofurans and indoles, in which the MCR process occurred in both C(sp2)–H and benzylic C(sp3)–H to achieve an unprecedented alkylaminative cyclization to realize piperidine-fusion on benzofuran and indole frameworks.11e,12l,13 Inspired by these studies, we envisioned that the MCR process could occur on N–H and the adjacent 2-position of indoles simultaneously, and this might constitute new structured indole-fused heterocycles. Herein, we would like to report a multicomponent reaction for modular assembly of indole-fused seven-membered heterocycles (Scheme 1C).
Results and discussion
Initially, we commenced our investigation by employing 3-methylindole 1a, formaldehyde 2, and chloroethylamine hydrochloride 3a as model substrates. We conducted the reaction by mixing these substrates in one-pot in the solvent of acetic acid at 45 °C. Interestingly, a new product was formed in 37% yield (Table 1, entry 1) and the characterization identified this product as an intriguing indole-fused oxadiazepine, and this seven-membered heterocycle formation indicated a new multicomponent reaction occurrence. Furthermore, the structure of 4a was unambiguously confirmed by X-ray analysis. The next screening of solvents showed that the use of DMF, DMSO and MeOH would give low yields (entry 2, entry 5 and entry 7) while THF, CH3CN, and DCM were optimal to significantly improve the yield (THF, 75%; CH3CN, 63%; DCM, 70%; entries 3–4 and entry 6). The solvent mixture of DMF and CH3CN was also effective giving 4a in 65% yield (entry 8). Increasing the temperature to 60 °C gave a comparable yield (entry 9) while increasing to 70 °C would diminish the yield (entry 10). The following optimization by varying the amounts of 2 and 3a did not further improve the yields. In addition, conducting this reaction on a gram scale afforded 4a in 70% yield, thus underscoring the scalability of this process (see the ESI†).
Table 1 Reaction optimizationa
With the optimized reaction conditions in hand, we then investigated the generality of this multicomponent reaction. As shown in Fig. 1, a variety of amino hydrochlorides were varied in this process as amine building blocks, and numerous flexible aliphatic amino hydrochlorides with a diversity of substituents, including chloride, bromide, sulfone, trifluoromethyl, cyano, phthalimide, alkene, alkyne, (hetero)cyclic rings, thiophene and functionalized aryl rings, were found compatible to furnish the corresponding indole-fused oxadiazepines in good to excellent yields (4a–4t). Besides, diverse amino acid derivatives have been successfully utilized in this reaction sequence, allowing for seamless integration with 3-methylindoles 1 and formaldehyde 2 to yield the targeted compounds (4u–4aa). This process effectively incorporates the chirality and functionality of amino acids directly into products, thereby broadening the chemical diversity of indole-fused heterocycles and offering ample opportunity for biology exploration and lead compound optimization. The use of amino acid derivatives such as glycine, alanine, leucine, and methionine has proven highly effective in this MCR process, consistently producing indole-fused oxadiazepines with good to excellent yields (51–89%). Moreover, the structure of 4s was confirmed by X-ray analysis wherein the absolute configuration of the chiral center was determined to be identical with the starting amino building block, and it was reasonable that other amino acid derived oxadiazepines retained the same stereochemistry configuration. Aniline hydrochloride was also subjected to these MCRs and was not applicable. On the other hand, the scope of indoles was also investigated. As shown in Fig. 1, various substituted indoles with differential electron-donating and electron-withdrawing profiles and substitution positions were well compatible in this process, and those groups include fluoro, bromo, methoxy, alkene, alkyne, methyl, acetoxyl, ester, N-phthalamide, and amide, and this MCR process could convert these indoles to indole-fused oxadiazepines in moderate to good yields (4ab–4ap, 41–94%); and there were no significant differences in the yields for differential substitution while the electron-donating methoxy group on the indole ring would lead to slightly low yield probably due to their instability. Moreover, the structure of compound 4aj was determined by X-ray analysis. It should be noted that indoles 1o and 1p were tryptamine derivatives and melatonin respectively; thus this MCR protocol provides a distinct heterocycle-fused evolution for these bioactive molecules and would definitely be useful in drug discovery.
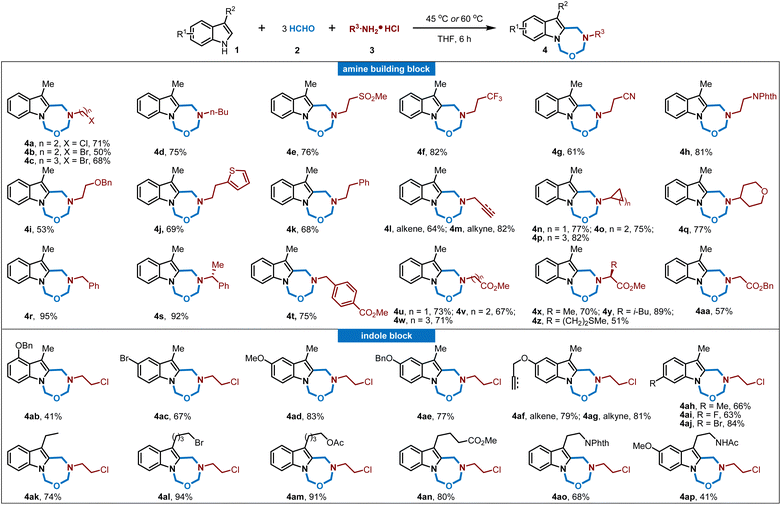 |
| Fig. 1 Scope for the multicomponent synthesis of indole-fused oxadiazepines. Reaction conditions: 1 (0.2 mmol), 2 (1.0 mmol), 3 (0.4 mmol), THF (2 mL), 45 °C or 60 °C, 6 hours. Isolated yields are given. Phth = phthalimide. | |
Encouraged by these results, we wondered whether this MCR strategy could be extended to the facile construction of other new structural indole-fused heterocycles. Based on the reaction mechanism understanding and various experimental attempts (for details, see the ESI†), we found that another addition of sodium thiosulfate 5 (Na2S2O3) to the mixture of 1, 2 and 3 with the treatment of aqueous hydrochloride solution would enable a new multicomponent reaction to deliver indole-fused thiadiazepine. Then the generality of this MCR was explored. As shown in Fig. 2, a wide range of amines bearing diverse functionalities, including flexible aliphatic amines with the substitution of chloro, sulfone, cyano, benzyloxy, heterocyclic rings, alkene, alkyne, cyclobutane and phenyl, were well applicable in this process to furnish the corresponding indole-fused thiadiazepine products in good to excellent yields (6a–6j), and the structure of 6a was unambiguously confirmed by single-crystal X-ray crystallography. Meanwhile, amino acid derivatives were also compatible in this process and assembled with indole 1, formaldehyde 2 and Na2S2O35 to rapidly achieve the products in good yields (6k–6n). The indole scope was also investigated wherein differential substitution and melatonin were explored, and the corresponding indole-fused thiadiazepine products were achieved in good to excellent yields (6o–6r). In addition, a gram-scale synthesis of 6a was conducted to demonstrate the scalability and practicality of this MCR (see the ESI†).
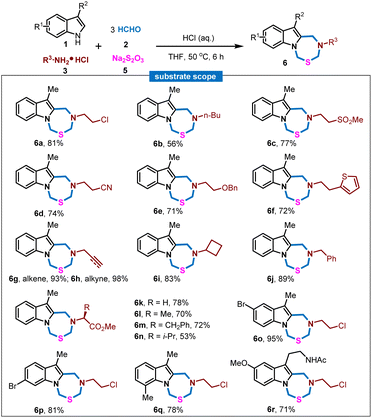 |
| Fig. 2 Scope for the multicomponent synthesis of indole-fused thiazepines. Conditions: 1 (0.2 mmol), 2 (1.0 mmol), 3 (0.4 mmol), Na2S2O35 (0.4 mmol), HCl (0.4 mmol, 12 N), THF (2 mL), 50 °C, 6 hours. Isolated yields are given. | |
To further gain insights into the mechanism, a series of control reactions and isotope-labeling reactions were conducted. First, compounds Int-1 and Int-2u were isolated and identified as intermediates. Then, Int-1, Int-2u and N-MOM protected indole 7 were individually subjected to the multicomponent reaction with formaldehyde 2 and methyl glycinate hydrochloride 3u, and the results showed that these MCRs could all furnish the product 4u in good yields (Fig. 3A). Besides, 1a was also subjected to a reaction with paraformaldehyde 2 and chloroethylamine hydrochloride 3a with an addition of 18O-labelled H2O, and the result showed that 4a could be formed in 59% yield coupled with high 18O-labelled incorporations (Fig. 3B). Based on these results, a plausible mechanism was proposed in Fig. 3C. Initially, indole 1a could react with formaldehyde 2 to give intermediate Int-1, and amino hydrochloride 3 could react with formaldehyde 2 to give iminium intermediate 8. Afterwards, an alkylamination would occur between Int-1 and 8 to deliver Int-2. Int-2 would further condense with formaldehyde to give Int-3; then water or H2S (in situ generated from Na2S2O3 and aqueous HCl) would undergo a bis-nucleophilic addition to Int-3 to give indole-fused seven-membered heterocycles, along with the release of Int-4 which further decomposed to formaldehyde 2 and iminium 8. When Na2S2O3 was replaced with Na2S, the MCRs would deliver the same products, thus indicating that H2S was the real nucleophilic reagent (for details, see the ESI†).14
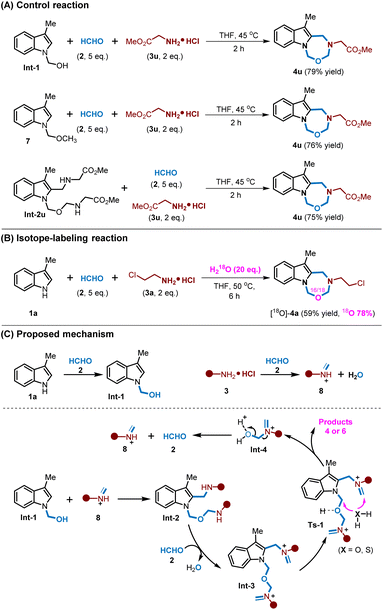 |
| Fig. 3 Mechanism investigation experiments and the proposed mechanism. | |
Since indole derivatives were privileged structures in pharmaceuticals, these indole-fused heterocyclic compounds were subsequently subjected to biological evaluation. At the beginning, cytotoxicity evaluation was conducted for these compounds in the GES-1 cell line (human) and 3T3 cell line (mouse) at a concentration of 10 μM and 20 μM, and the results revealed their low cytotoxicity (see ESI Fig. S7†). Then, these compounds were subjected to anti-proliferative evaluation against four human cancer cell lines, including A549 (lung cancer cell line), HCT116 (colon cancer cell line), BEL7402 (liver cancer cell line) and MDA-MB-231 (breast cancer cell line). As shown in Fig. 4A (for details, see ESI Fig. S8†), a number of compounds displayed potential inhibitory activities against BEL7402 cells, with IC50 values ranging from 12.6 μM to 23.9 μM. Moreover, compounds 6g and 6h exhibited good anti-proliferative activity against A549 cells (IC50 = 9.6 μM and IC50 = 11.7 μM). These results indicated that this class of indole-fused heterocycles has potential antitumor activities.
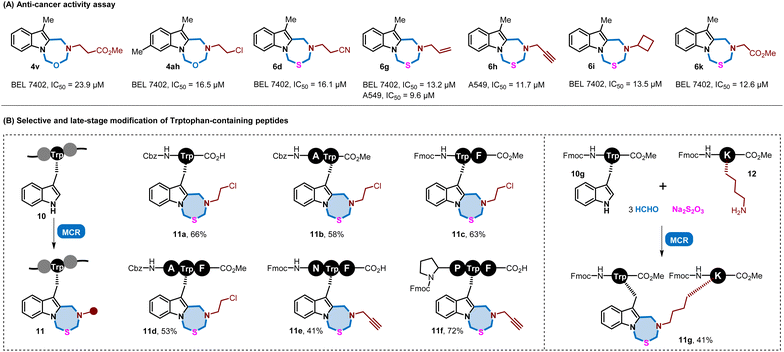 |
| Fig. 4 Application of these MCRs in biological activity assay and peptide chemistry. MCR = multicomponent reaction. | |
Meanwhile, the synthetic utility of this multicomponent reaction protocol was applied in peptide modification. Because of the natural advantage of mimicking the endogenous portion of the interacting proteins, peptides have gained increased attention.15 However, their drug discovery was plagued by their particularly poor physicochemical properties and in vivo stabilities.16 Chemical modification has been a robust tool to overcome the intrinsic limitations.17 In recent decades, the aromatic tryptophan (Trp) side chain has become an attractive target for late-stage modification for its essential role in peptides and proteins.12k,18 Given the successful implementation of these MCRs on tryptamine and melatonin toward modular assembly of indole-fused seven-membered heterocycles, we hypothesized that this protocol could be a selective and efficient method for tryptophan (Trp) containing peptides. Thus, we used Cbz-Trp-OH as a model substrate to react under standard conditions. As expected, the corresponding product 11a was obtained in satisfactory yield. The strategy was also tested on the dipeptide substrates Cbz-Ala-Trp-OMe and Cbz-Trp-Phe-OMe, and the results showed that moderate yields could be achieved regardless of whether the Trp residue is located at the C-terminal or N-terminal end (11b and 11c). Furthermore, this multicomponent reaction protocol was capable of tripeptide modification (11d–11f), displaying high reaction activity and compatibility with different amino acids. It should be noted that the modified Trp-containing peptides bearing an alkynyl moiety are promising building blocks for click reactions toward diverse derivatization. In addition, we employed Fmoc-Trp-OMe and Fmoc-Lys-OMe as indole and amine building blocks for these MCRs, and gratifyingly, these two peptides could be assembled to form the indole-fused heterocycles 11g in 41% yield. These results indicated that this multicomponent reaction could be a potent and practical modification strategy for peptides.
Conclusions
In conclusion, we have developed a modular assembly of indole-fused seven-membered heterocycles through multicomponent reactions. In this process, indole, formaldehyde and amino hydrochloride could assemble rapidly to achieve the construction of indole-fused oxadiazepines, while a further addition of Na2S2O3 would deliver thiadiazepines alternatively, and the reaction process featured mild reaction conditions, broad substrate scope and high efficiency. The control reaction and isotope-labelling reaction revealed an iterative assembly of these building blocks wherein a bis-nucleophilic addition was vital in this process. Biological evaluation disclosed that these compounds exhibit potent anti-proliferative activity, and this protocol could be applicable in the selective and late-stage modification of peptides. Therefore, this protocol provides a distinct and efficient approach for accessing indole-fused heterocycles and serves as an efficient peptide modification strategy and would be useful in organic synthesis, medicinal chemistry and chemical biology.
Data availability
Detailed synthetic procedures and complete characterization data for all new compounds can be found in the ESI.†
Author contributions
S. C. conceived and designed the experiments. S. C. and L. Z. directed the project. J. L. performed the experiments. H. N., W. Z., Z. L., and H. J. prepared some starting materials. J. L. and L. Z. analyzed the data. S. C. and L. Z. wrote the paper. All authors discussed the results and commented on the manuscript.
Conflicts of interest
There are no conflicts to declare.
Acknowledgements
We are grateful for financial support from the National Natural Science Foundation of China (22277106), the Natural Science Foundation of Zhejiang Province (Distinguished Young Scholar Program, LR23H300001), the National Program for Support of Top-notch Young Professionals (grant 2022), the Zhejiang Provincial Key R&D Program (2023C03118), and the joint funding of Zhejiang University-Huahai Pharmaceutical Co, Ltd. We are grateful to Zhenjun Mao (Department of Chemistry, Zhejiang University) and Jianyang Pan (Research and Service Center, College of Pharmaceutical Sciences, Zhejiang University) for performing NMR spectrometry for structure elucidation. We are grateful to Jiyong Liu (Department of Chemistry, Zhejiang University) for performing X-ray analysis.
Notes and references
-
(a) M. Somei and F. Yamada, Nat. Prod. Rep., 2005, 22, 73–103 RSC;
(b) R. S. Doody, S. I. Gavrilova, M. Sano, R. G. Thomas, P. S. Aisen, S. O. Bachurin, L. Seely and D. Hung, Lancet, 2008, 372, 207–215 CrossRef CAS PubMed;
(c) E. Stempel and T. Gaich, Acc. Chem. Res., 2016, 49, 2390–2402 CrossRef CAS PubMed;
(d) P. T. Singh and M. O. Singh, Mini-Rev. Med. Chem., 2018, 18, 9–25 CrossRef;
(e) Y. Wan, Y. Li, C. Yan, M. Yan and Z. Tang, Eur. J. Med. Chem., 2019, 183, 111691 CrossRef CAS;
(f) J. C. Reisenbauer, O. Green, A. Franchino, P. Finkelstein and B. Morandi, Science, 2022, 377, 1104–1109 CrossRef CAS PubMed;
(g) J. Woo, A. H. Christian, S. A. Burgess, Y. Jiang, U. F. Mansoor and M. D. Levin, Science, 2022, 376, 527–532 CrossRef CAS PubMed.
-
(a) J. Sun, H. Lou, S. Dai, H. Xu, F. Zhao and K. Liu, Phytochemistry, 2008, 69, 1405–1410 CrossRef CAS PubMed;
(b) T. V. Sravanthi and S. L. Manju, Eur. J. Pharm. Sci., 2016, 91, 1–10 CrossRef CAS PubMed;
(c) A. Dorababu, RSC Med. Chem., 2020, 11, 1335–1353 RSC;
(d) Y. Zhu, J. Zhao, L. Luo, Y. Gao, H. Bao, P. Li and H. Zhang, Eur. J. Med. Chem., 2021, 223, 113665 CrossRef CAS PubMed.
-
(a) L. S. Fernandez, M. S. Buchanan, A. R. Carroll, Y. J. Feng, R. J. Quinn and V. M. Avery, Org. Lett., 2009, 11, 329–332 CrossRef CAS PubMed;
(b) E. Ascic, J. F. Jensen and T. E. Nielsen, Angew. Chem., Int. Ed., 2011, 50, 5188–5191 CrossRef CAS PubMed;
(c) R. Vallakati and J. A. May, J. Am. Chem. Soc., 2012, 134, 6936–6939 CrossRef CAS;
(d) S. G. Dawande, B. S. Lad, S. Prajapati and S. Katukojvala, Org. Biomol. Chem., 2016, 14, 5569–5573 RSC;
(e) J. Dai, W. Dan, Y. Zhang and J. Wang, Eur. J. Med. Chem., 2018, 157, 447–461 CrossRef CAS PubMed;
(f) Q. Dan, S. A. Newmister, K. R. Klas, A. E. Fraley, T. J. McAfoos, A. D. Somoza, J. D. Sunderhaus, Y. Ye, V. V. Shende, F. Yu, J. N. Sanders, W. C. Brown, L. Zhao, R. S. Paton, K. N. Houk, J. L. Smith, D. H. Sherman and R. M. Williams, Nat. Chem., 2019, 11, 972–980 CrossRef CAS PubMed;
(g) X.-Y. Liu and Y. Qin, Acc. Chem. Res., 2019, 52, 1877–1891 CrossRef CAS;
(h) J.-K. Dai, W.-J. Dan and J.-B. Wan, Eur. J. Med. Chem., 2022, 229, 114057 CrossRef CAS PubMed.
-
(a) H. Mizoguchi, H. Oikawa and H. Oguri, Nat. Chem., 2014, 6, 57–64 CrossRef CAS;
(b) C. Sherer and T. J. Snape, Eur. J. Med. Chem., 2015, 97, 552–560 CrossRef CAS;
(c) R. Purgatorio, M. de Candia, M. Catto, A. Carrieri, L. Pisani, A. De Palma, M. Toma, O. A. Ivanova, L. G. Voskressensky and C. D. Altomare, Eur. J. Med. Chem., 2019, 177, 414–424 CrossRef CAS PubMed.
-
(a) B. E. Maryanoff and D. F. McComsey, J. Org. Chem., 1978, 43, 2733–2735 CrossRef CAS;
(b) B. E. Maryanoff, S. O. Nortey and J. F. Gardocki, J. Med. Chem., 1984, 27, 1067–1071 CrossRef CAS PubMed.
- R. J. Katz, P. S. Landau, M. Lott, A. Bystritsky, B. Diamond, R. Hoehn-Saric, M. Rosenthal and C. Weise, Biol. Psychiatry, 1993, 34, 41–44 CrossRef CAS.
-
(a) A. J. M. Disney, B. Kellam and L. V. Dekker, ChemMedChem, 2016, 11, 972–979 CrossRef CAS;
(b) K. M. Gayler, K. Kong, K. Reisenauer, J. H. Taube and J. L. Wood, ACS Med. Chem. Lett., 2020, 11, 2441–2445 CrossRef CAS;
(c) J. Zhang, A. Cordshagen, I. Medina, H. G. Nothwang, J. R. Wisniewski, M. Winklhofer and A.-M. Hartmann, PLoS One, 2020, 15, e0232967 CrossRef CAS PubMed.
- M. D. Ennis, R. L. Hoffman, N. B. Ghazal, R. M. Olson, C. S. Knauer, C. L. Chio, D. K. Hyslop, J. E. Campbell, L. W. Fitzgerald, N. F. Nichols, K. A. Svensson, R. B. McCall, C. L. Haber, M. L. Kagey and D. M. Dinh, Bioorg. Med. Chem. Lett., 2003, 13, 2369–2372 CrossRef CAS PubMed.
-
(a) D. G. Pintori and M. F. Greaney, J. Am. Chem. Soc., 2011, 133, 1209–1211 CrossRef CAS;
(b) L. D. Basanagoudar, C. S. Mahajanshetti and S. B. Dambal, Indian J. Chem., Sect. B: Org. Chem. Incl. Med. Chem., 1991, 30, 1014 Search PubMed;
(c) Y. Ohta, H. Chiba, S. Oishi, N. Fujii and H. Ohno, Org. Lett., 2008, 10, 3535 CrossRef CAS PubMed.
-
(a) A. Dömling, W. Wang and K. Wang, Chem. Rev., 2012, 112, 3083–3135 CrossRef PubMed;
(b)
J. Zhu, Multicomponent Reactions in Organic Synthesis, 2015 Search PubMed.
-
(a) S. J. Zuend, M. P. Coughlin, M. P. Lalonde and E. N. Jacobsen, Nature, 2009, 461, 968–970 CrossRef CAS PubMed;
(b) E. Ruijter, R. Scheffelaar and R. V. A. Orru, Angew. Chem., Int. Ed., 2011, 50, 6234–6246 CrossRef CAS PubMed;
(c) B. Huang, L. Zeng, Y. Shen and S. Cui, Angew. Chem., Int. Ed., 2017, 56, 4565–4568 CrossRef CAS PubMed;
(d) C. Wang, Z. Lai, H. Xie and S. Cui, Angew. Chem., Int. Ed., 2021, 60, 5147–5151 CrossRef CAS PubMed;
(e) Z. Lai, R. Wu, J. Li, X. Chen, L. Zeng, X. Wang, J. Guo, Z. Zhao, H. Sajiki and S. Cui, Nat. Commun., 2022, 13, 435 CrossRef CAS PubMed;
(f) X. Hu, L. Chen, H. Li, Q. Xu, X. Liu and X. Feng, ACS Catal., 2023, 13, 6675–6682 CrossRef CAS.
-
(a) M. Shiri, Chem. Rev., 2012, 112, 3508–3549 CrossRef CAS PubMed;
(b) N. Sarkar, A. Banerjee and S. G. Nelson, J. Am. Chem. Soc., 2008, 130, 9222–9223 CrossRef CAS PubMed;
(c) T. A. Cernak and T. H. Lambert, J. Am. Chem. Soc., 2009, 131, 3124–3125 CrossRef CAS PubMed;
(d) P. Galzerano, F. Pesciaioli, A. Mazzanti, G. Bartoli and P. Melchiorre, Angew. Chem., Int. Ed., 2009, 48, 7892–7894 CrossRef CAS PubMed;
(e) M. Terada, K. Machioka and K. Sorimachi, Angew. Chem., Int. Ed., 2009, 48, 2553–2556 CrossRef CAS PubMed;
(f) J. S. Alford and H. M. L. Davies, J. Am. Chem. Soc., 2014, 136, 10266–10269 CrossRef CAS PubMed;
(g) L. Jiang, W. Jin and W. Hu, ACS Catal., 2016, 6, 6146–6150 CrossRef CAS;
(h) O. Ghashghaei, M. Pedrola, F. Seghetti, V. V. Martin, R. Zavarce, M. Babiak, J. Novacek, F. Hartung, K. M. Rolfes, T. Haarmann-Stemmann and R. Lavilla, Angew. Chem., Int. Ed., 2021, 60, 2603–2608 CrossRef CAS PubMed;
(i) S. Yu, W. Chang, R. Hua, X. Jie, M. Zhang, W. Zhao, J. Chen, D. Zhang, H. Qiu, Y. Liang and W. Hu, Nat. Commun., 2022, 13, 7088 CrossRef CAS PubMed;
(j) R.-Y. Hua, S.-F. Yu, X.-T. Jie, H. Qiu and W.-H. Hu, Angew. Chem., Int. Ed., 2022, 61, e202213407 CrossRef CAS PubMed;
(k) S. Krajcovicova and D. R. Spring, Angew. Chem., Int. Ed., 2023, 62, e202307782 CrossRef CAS PubMed;
(l) J. Li, Z. Lai, W. Zhang, L. Zeng and S. Cui, Nat. Commun., 2023, 14, 4806 CrossRef CAS PubMed.
-
(a) M. Arend, B. Westermann and N. Risch, Angew. Chem., Int. Ed., 1998, 37, 1044–1070 CrossRef;
(b) M. Yamanaka, J. Itoh, K. Fuchibe and T. Akiyama, J. Am. Chem. Soc., 2007, 129, 6756–6764 CrossRef CAS PubMed;
(c) J. M. M. Verkade, L. J. C. v. Hemert, P. J. L. M. Quaedflieg and F. P. J. T. Rutjes, Chem. Soc. Rev., 2008, 37, 29–41 RSC;
(d) R. G. Arrayás and J. C. Carretero, Chem. Soc. Rev., 2009, 38, 1940–1948 RSC;
(e) A. Noble and J. C. Anderson, Chem. Rev., 2013, 113, 2887–2939 CrossRef CAS PubMed;
(f) J. Rostoll-Berenguer, G. Blay, J. R. Pedro and C. Vila, Adv. Synth. Catal., 2021, 363, 602–628 CrossRef CAS;
(g) M.-X. Pu, H.-Y. Guo, Z.-S. Quan, X. Li and Q.-K. Shen, J. Enzyme Inhib. Med. Chem., 2023, 38, 2235095 CrossRef PubMed.
- I. Klose, G. Di Mauro, D. Kaldre and N. Maulide, Nat. Chem., 2022, 14, 1306–1310 CrossRef CAS PubMed.
-
(a) B. J. Bruno, G. D. Miller and C. S. Lim, Ther. Delivery, 2013, 4, 1443–1467 CrossRef CAS PubMed;
(b) Q.-S. Du, N.-Z. Xie and R.-B. Huang, Med. Chem., 2015, 11, 235–247 CrossRef CAS PubMed.
-
(a) T. Uhlig, T. Kyprianou, F. G. Martinelli, C. A. Oppici, D. Heiligers, D. Hills, X. R. Calvo and P. Verhaert, EuPa Open Proteomics, 2014, 4, 58–69 CrossRef CAS;
(b) J. Iegre, J. S. Gaynord, N. S. Robertson, H. F. Sore, M. Hyvönen and D. R. Spring, Adv. Ther., 2018, 1, 1800052 CrossRef.
-
(a) T. A. Hill, N. E. Shepherd, F. Diness and D. P. Fairlie, Angew. Chem., Int. Ed., 2014, 53, 13020–13041 CrossRef CAS PubMed;
(b) E. V. Vinogradova, C. Zhang, A. M. Spokoyny, B. L. Pentelute and S. L. Buchwald, Nature, 2015, 526, 687–691 CrossRef CAS PubMed;
(c) D. S. Nielsen, N. E. Shepherd, W. Xu, A. J. Lucke, M. J. Stoermer and D. P. Fairlie, Chem. Rev., 2017, 117, 8094–8128 CrossRef CAS PubMed;
(d) L. Reguera and D. G. Rivera, Chem. Rev., 2019, 119, 9836–9860 CrossRef CAS PubMed.
-
(a) K. R. Schramma, L. B. Bushin and M. R. Seyedsayamdost, Nat. Chem., 2015, 7, 431–437 CrossRef CAS PubMed;
(b) Z. Bai, C. Cai, W. Sheng, Y. Ren and H. Wang, Angew. Chem., Int. Ed., 2020, 59, 14686–14692 CrossRef CAS PubMed;
(c) S. J. Tower, W. J. Hetcher, T. E. Myers, N. J. Kuehl and M. T. Taylor, J. Am. Chem. Soc., 2020, 142, 9112–9118 CrossRef CAS PubMed;
(d) R. Mao, S. Xi, S. Shah, M. J. Roy, A. John, J. P. Lingford, G. Gäde, N. E. Scott and E. D. Goddard-Borger, J. Am. Chem. Soc., 2021, 143, 12699–12707 CrossRef CAS PubMed;
(e) L. Liu, X. Fan, B. Wang, H. Deng, T. Wang, J. Zheng, J. Chen, Z. Shi and H. Wang, Angew. Chem., Int. Ed., 2022, 61, e202206177 CrossRef CAS PubMed;
(f) Y. Weng, X. Xu, H. Chen, Y. Zhang and X. Zhuo, Angew. Chem., Int. Ed., 2022, 61, e202206308 CrossRef CAS PubMed;
(g) J. A. C. Delgado, Y.-M. Tian, M. Marcon, B. König and M. W. Paixão, J. Am. Chem. Soc., 2023, 145, 26452–26462 CrossRef CAS PubMed;
(h) N. J. Kuehl and M. T. Taylor, J. Am. Chem. Soc., 2023, 145, 22878–22884 CrossRef CAS PubMed.
Footnote |
† Electronic supplementary information (ESI) available: Synthesis, characterization, control experiments, NMR, and crystallographic data. CCDC 2243493 (for 4a), 2243494 (for 4s), 2243505 (for 4aj) and 2249556 (for 6a). For ESI and crystallographic data in CIF or other electronic format see DOI: https://doi.org/10.1039/d4sc00522h |
|
This journal is © The Royal Society of Chemistry 2024 |
Click here to see how this site uses Cookies. View our privacy policy here.