DOI:
10.1039/D4SC00601A
(Edge Article)
Chem. Sci., 2024,
15, 5730-5737
Aza-Achmatowicz rearrangement coupled with intermolecular aza-Friedel–Crafts enables total syntheses of uleine and aspidosperma alkaloids†
Received
25th January 2024
, Accepted 11th March 2024
First published on 14th March 2024
Abstract
Aspidosperma and uleine alkaloids belong to the large family of monoterpene indole alkaloids with diverse biological activities and thus have attracted extensive synthetic interest. Reported is the development of a new synthetic strategy that allows direct C3–C2′ linkage of indoles with functionalized 2-hydroxypiperidines to construct the core common to all aspidoserma and uleine alkaloids. Such indole-piperidine linkage is enabled by coupling aza-Achmatowicz rearrangement (AAR) with indoles via an intermolecular aza-Friedel–Crafts (iAFC) reaction. This AAR-iAFC reaction proceeds under mild acidic conditions with wide tolerance of functional groups (33 examples). The synthetic application of the AAR-iAFC method was demonstrated with collective total syntheses of 3 uleine-type and 6 aspidosperma alkaloids: (+)-3-epi-N-nor-dasycarpidone, (+)-3-epi-dasycarpidone, (+)-3-epi-uleine, 1,2-didehydropseudoaspidospermidine, 1,2-dehydroaspidospermidine, vincadifformine, winchinine B, aspidospermidine, and N-acetylaspidospermidine. We expect that this AAR-iAFC strategy is applicable to other monoterpene indole alkaloids with the C3–C2′ linkage of indoles and piperidines.
Introduction
The aspidosperma and uleine alkaloids (Scheme 1a and b) belong to the large family of monoterpenoid indole alkaloids (ca. 3000 members) with diverse biological activities and intricate skeletal diversity.1–7 They continue to influence the research fields of natural products, drug discovery, biochemistry, and especially organic chemistry (total synthesis and methodology development).8,9 Structurally, the direct covalent C3–C2′ linkage of indole (in blue) with piperidine (in red) characterizes this subclass of alkaloids and thus has sparked great interest from generations of organic chemists in developing novel and efficient synthetic strategies10–40 (Scheme 1c). For example, many innovative synthetic strategies for aspidosperma alkaloids have been developed, most of which can be classified into three categories: (i) intermolecular or intramolecular indolization of anilines with piperidine derivatives,41–50 (ii) late-stage piperidine formation from 1,2,3,4-tetrahydrocarbazoles,51–62 and (iii) intramolecular (or transannular) Mannich reaction of indoles with tethered piperidinium.63–72 It was interestingly noted that the intermolecular reaction of indoles with piperidine derivatives was first reported in 1955 but rarely explored for the total synthesis of aspidosperma (and uleine) alkaloids,73–75 probably due to the instability and unavailability of functionalized 2-hydroxypiperidines. In this article, we report the development of an intermolecular aza-Friedel-Crafts (iAFC) reaction76–78 of indoles with functionalized 2-hydroxypiperidines, which can be readily available from aza-Achmatowicz rearrangement and thus enables collective total synthesis of aspidosperma and uleine alkaloids (Scheme 1c(iv)).
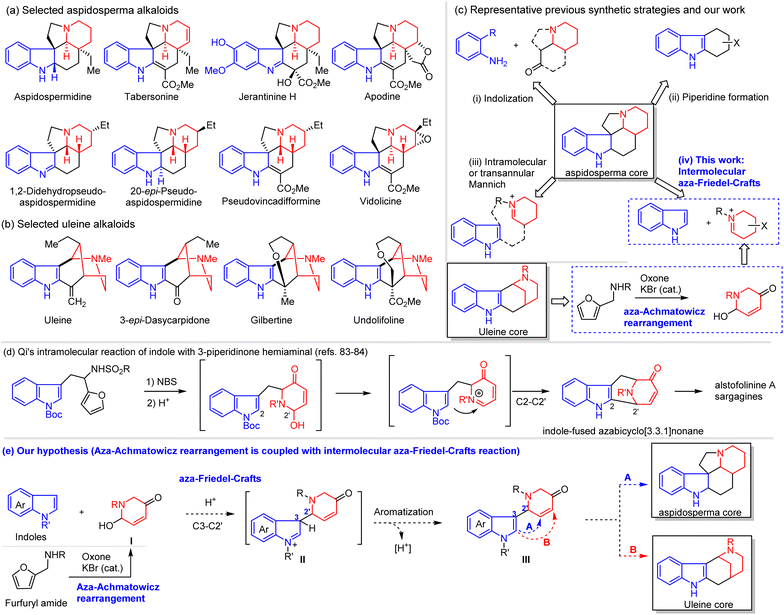 |
| Scheme 1 Representative members of aspidosperma and uleine alkaloids, selected synthetic strategies, and our hypothesis. | |
Aza-Achmatowicz rearrangement (AAR) represents one of the most facile entries into functionalized piperidines,79 and our research group has great interest in exploiting AAR for total syntheses of bioactive alkaloids.80–82 To extend the synthetic utility of rather unstable AAR products in total synthesis, we envisioned that the freshly prepared AAR products (functionalized 2-hydroxypiperidines) might react with indoles to enable a direct C3–C2′ coupling (iAFC) for aspidosperma and uleine alkaloids (Scheme 1e). We noticed that Qi et al.83,84 had reported an intramolecular reaction of indoles with AAR products (C2–C2′ coupling) to construct the indole-fused azabicyclo[3.3.1]nonane core and accomplished elegantly the total synthesis of alstofolinine A and sarpagine alkaloids (Scheme 1d). The challenge of this intramolecular reaction was well recognized by the authors due to limited substrates and oxidants (“upon exposure to oxidizing conditions (m-CPBA), the primary amine and the indole were preferentially oxidized to N-oxide and oxindole, respectively, prior to the oxidation of the furan”),83 which represents a significant limitation in synthetic applications. We believed that an intermolecular coupling version of indoles with AAR products could significantly expand the synthetic utility with the highest flexibility. Additionally, the restriction on NBS oxidation for aza-Achmatowicz rearrangement could be largely relaxed to use our green oxidation protocol (Oxone/KBr).85–87 If our hypothesis works, the coupling product III with C3–C2′ linkage could be used for the total synthesis of aspidosperma (path A) and uleine-type alkaloids (cyclization via path B, Scheme 1e).
Results and discussion
Methodology development: aza-Achmatowicz rearrangement is coupled with intermolecular aza-Friedel–Crafts (iAFC) reaction
We began our study with aza-Achmatowicz rearrangement of N-Boc-furfurylamine under our oxone/KBr (cat.) conditions85–87 to provide functionalized 2-hydroxypiperidine (2a, AAR product), which was unstable for storage and should be used immediately for the subsequent reaction with indoles (Table 1). Two indoles, 1a and 1b, were chosen to evaluate the reaction efficiency and functional group tolerance. Trifluoroacetic acid (TFA) was used first as the catalyst (5 mol%) for the iAFC reaction of indole88 (1a) and AAR product 2a under various solvents and the results were excellent with 78% to 88% yields of 3a derived from selective C3–C2′ coupling (entries 1–7). Notably, the C2–C2′ coupling product was not observed. It was noteworthy that the widely used Lewis acid BF3–Et2O gave a lower yield of 3a (60%, entry 8) while the unusual organic Brønsted acid pentacarbomethoxycyclopentadiene (PCCP)89–91 in toluene delivered slightly higher yield (90%, entry 9). Surprisingly, the iAFC reaction of indole 1b with AAR product 2a under various acidic conditions gave consistently lower yields of 3b (0–58%, entries 10–18), which might be due to the steric hindrance exerted by the C2-alkyl substituent. It was noted that the tert-butyldimethylsilyl (TBS) ether survived under these conditions and usually indole 1b was not fully consumed, which suggested that the decomposition of 2a occurred under acidic conditions with prolonged reaction time. Extensive experiments revealed that PCCP was the optimal protic acid as the catalyst with high reproducibility and best efficiency at 0.05–0.2 M concentration (58% yield of 3a, entry 18).
Table 1 Optimization of the reaction conditions of iAFCa
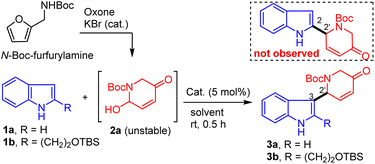
|
Entry |
1a/1b |
Catalyst |
Solvent |
Yield (%) |
Conditions: 1a/1b (0.1 mmol), 2a (0.12 mmol), acid (0.005 mmol), solvent (0.05–0.2 M), room temperature, 0.5–12 h. Yield was determined by 1H NMR analysis using dibromomethane as the internal standard. PCCP: pentacarbomethoxycyclopentadiene.
|
1 |
1a
|
TFA |
DCM |
82 |
2 |
1a
|
TFA |
PhMe |
88 |
3 |
1a
|
TFA |
MeOH |
78 |
4 |
1a
|
TFA |
DCE |
85 |
5 |
1a
|
TFA |
THF |
81 |
6 |
1a
|
TFA |
EtOAc |
86 |
7 |
1a
|
TFA |
CHCl3 |
88 |
8 |
1a
|
BF3–Et2O |
DCM |
60 |
9 |
1a
|
PCCP |
PhMe |
90 |
10 |
1b
|
TFA |
DCM |
35 |
11 |
1b
|
(−)-CSA |
DCM |
37 |
12 |
1b
|
F2CHCO2H |
DCM |
42 |
13 |
1b
|
pTSA·H2O |
DCM |
40 |
14 |
1b
|
ClCH2CO2H |
DCM |
Trace |
15 |
1b
|
Cl2CHCO2H |
DCM |
40 |
16 |
1b
|
Cl3CCO2H |
DCM |
24 |
17 |
1b
|
— |
HFIP |
10 |
18 |
1b
|
PCCP |
DCM |
58 |
Under the optimal conditions (PCCP or TFA in toluene), the scope of the iAFC reaction of indoles (1) with AAR products (2) was evaluated and the results are presented in Table 2. Generally, a wide variety of functionalized indoles could be employed for this iAFC with AAR products 2 in good to excellent yield and with excellent regioselectivity (C3–C2′ linkage). For example, substituents at the benzene moiety of indoles including halide (6-F, 5-Cl, 4-Br, and 6-Br) (3c–3f), methoxy (4-OMe, 5-OMe, 6-OMe, and 7-OMe) (3g–3j), acetoxyl (4-OAc and 5-OAc) (3k and 3l), alkyl (4-Me and 6-Me) (3o and 3p), and carbonyl (4-CHO, 7-CHO, and 5-CO2Me) (3q–3s) were well tolerated in the reaction. The only substituent that could not be tolerated was 5-OH on the indole (3n), while 4-hydroxylindole (4-OH) was a suitable substrate using TFA as the catalyst to provide 3m in 73% yield. We further examined indoles with nitrogen-containing substituents such as NO2 (3t), NHTs (3u), NMe2 (3v), and CN (3w) at C5 or C4 and found that only NMe2 at C5 was not able to deliver the desired coupling product (3v) which might be attributed to the basicity of the NMe2 group to form the ammonium salt with the protic acid catalyst, preventing the formation of the requisite cyclic iminium ion for iAFC. It was noted that the electron-withdrawing group (EWG) on the indole nitrogen such as N-Boc (3z) and N-Ts (3aa) deactivated the nucleophilicity of indole and thus iAFC did not occur, which was in contrast to the electron-donating group (EDG) such as N–Me (3x and 3ab 85% and 87%, respectively) and N-Bn (3y, 82%). Substitution at C2 indole was found to be possible for iAFC as exemplified by 1b in Table 1. To further understand such substitution effect, C2-Ph (3ac), C2-alkyl (3ad–3af), and C2-alkenyl (3ag) were employed for the iAFC with 2a and they proved efficient with 50–95% yield. The compatibility of C2 substitution was significant in terms of its potential application in total synthesis. Variation of AAR products for iAFC was also briefly examined and different EWG groups (N-Ts and N-Ns) on AAR products (3ah and 3ai) as well as C6′-allyl (3aj) were well tolerated in the iAFC reaction, yielding products in good to excellent yields. Finally, iAFC product 3a was reduced and then confirmed by X-ray diffraction analysis (3a′) (CCDC 2299125).
Table 2 Aza-Friedel–Crafts reaction of indoles (1) with AAR products (2)a
Conditions1(0.2 mmol), 2(0.24 mmol), PCCP (0.01 mmol), toluene (1 mL), room temperature, 0.5–2 h. Yields in parentheses were obtained using TFA (15 mol%) as the catalyst.
|
|
Total syntheses of uleine and aspidosperma alkaloids.
The iAFC reaction of indoles with AAR products not only greatly expanded the synthetic utility of AAR but also allowed access to the structural skeleton that could not be constructed by the intramolecular AFC reaction.83,84 To demonstrate such advantage of iAFC as compared to the intramolecular version, we undertook the total syntheses of uleine and aspidosperma alkaloids (Schemes 2 and 3). Our first synthetic targets were 3-epi-N-nor-dasycarpidone, 3-epi-dasycarpidone, and 3-epi-uleine. As depicted in Scheme 2, iAFC product 3a was obtained in >12 grams (90% yield) and used as the starting material for the synthesis of these three alkaloids. Protection of indole nitrogen with Boc2O provided compound 4 (85%), which was investigated for the kinetic resolution. While Corey–Bakshi–Shibata (CBS)92,93 reduction of enone 4 using (S)-(−)-2-methyl-CBS-oxazaborolidine and BH3·THF was not effective (<10% ee), Noyori asymmetric transfer hydrogenation94 with RuCl[(S,S)-TsDPEN](p-cymene) and HCO2H/Et3N could reduce the undesired enantiomer and the unreacted desired (+)-4 was obtained in 34% yield with 95% ee. Copper-mediated conjugate addition of ethyl Grignard to (+)-4 followed by acylation with ethyl cyanoformate delivered 1,3-dicarbonyl (−)-5 (60% for two steps) which was in equilibrium (tautomerization) with its enol form (−)-6. The enol (−)-6 was treated with triflate anhydride (Tf2O) and reduced with palladium catalysis to provide α,β-unsaturated ester (−)-8 in 71% yield. Palladium-catalyzed hydrogenation of the alkene was unexpectedly difficult, and we found that the conjugate reduction could be achieved by LiAlH4/CuI95 to generate (+)-9 in 37% yield (62% brsm). Removal of both N-Boc groups of (+)-9 with HCl and then KOH-promoted hydrolysis of ester gave carboxylic acid 10, which was subjected to polyphosphoric acid (PPA) for an intramolecular Friedel–Crafts reaction,96 furnishing (+)-3-epi-N-nor-dasycarpidone (11) (82%, two steps). However, the NMR data of our synthetic sample (11) were not in good agreement with those reported for natural product 3-epi-N-nor-dasycarpidone,97 which suggested that the structure of 3-epi-N-nor-dasycarpidone might be wrongly assigned. Reductive amination of 11 accomplished the synthesis of (+)-3-epi-dasycarpidone (12) in 60% yield, whose spectroscopic data were in well agreement with those of previously synthesized 3-epi-dasycarpidone.98–100 Finally, the synthesis of (+)-3-epi-uleine (13) was achieved in 44% yield by following Zhu's procedure.101
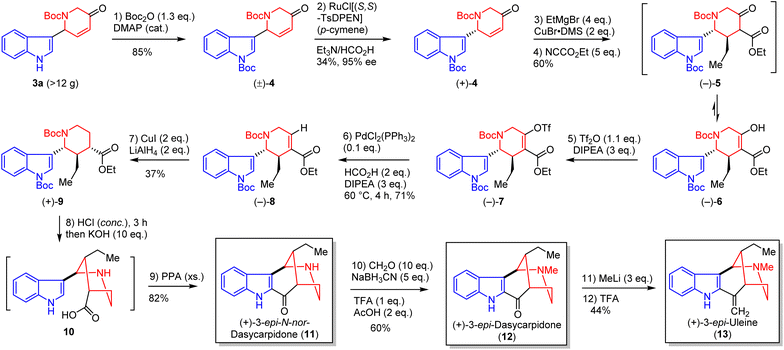 |
| Scheme 2 Total syntheses of uleine-type alkaloids. | |
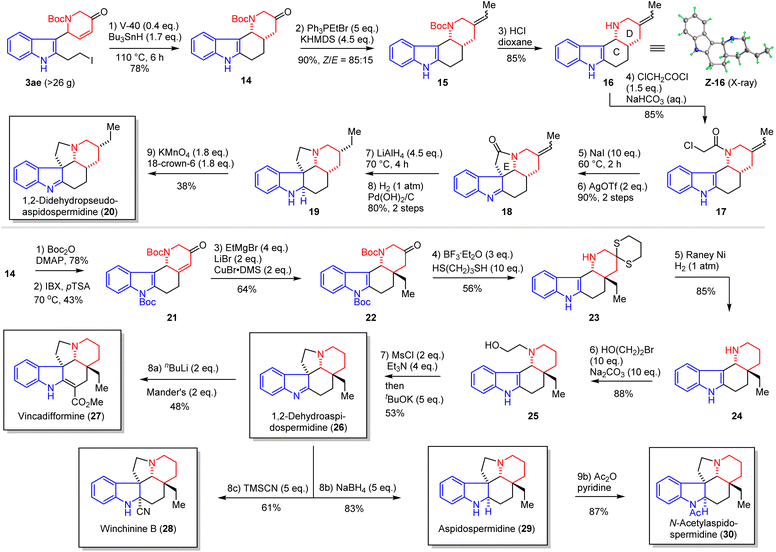 |
| Scheme 3 Total syntheses of aspidosperma alkaloids. | |
Next, we focused our efforts on the total synthesis of aspidosperma alkaloids by exploiting iAFC of indoles with AAR products. As depicted in Scheme 3, the iAFC product 3ae (Table 2) was obtained in >26 g from 3b and used as the starting material for the synthesis of all aspidosperma alkaloids. The 6-exo-trig cyclization of 3ae failed to occur with samarium iodide (SmI2)102–104 or palladium catalysis (Heck) but could be realized via a radical mechanism by treatment with 1,1′-azobis(cyclohexane-1-carbonitrile) (V-40) and tributyltin hydride105 under refluxed toluene to provide the tetracyclic core 14 (78% yield), which was common to all aspidosperma alkaloids. Wittig olefination of 14 gave a Z/E mixture of 15 (90%, Z/E = 85
:
15). Removal of N-Boc of 15 with HCl in dioxane afforded compound 16 (85%), in which the Z-isomer was suitable for single crystal X-ray diffraction analysis (CCDC 2299184) and thus confirmed the cis-fused CD ring. The E ring of 1,2-didehydropseudoaspidospermidine (20) was constructed by N-acylation of 16 with 2-chloroacetyl chloride (17, 85% yield) and then intramolecular SN2 substitution with assistance of AgOTf (90% yield). LiAlH4 reduction of indolenine 18 and palladium-catalyzed hydrogenation provided pseudoaspidospermidine (19, 80% yield), which was then oxidized by KMnO4 to furnish 1,2-didehydropseudoaspidospermidine (20) in 38% yield.106 Next, we employed 14 for the synthesis of other aspidosperma alkaloids. Protection of indole nitrogen of 14 as N-Boc was performed (78% yield), followed by direct desaturation of the tetracyclic ketone with 2-iodoxybenzoic acid107 (IBX, 43% yield), and conjugate addition of ethyl Grignard to 21 was facilitated with copper bromide and lithium bromide to provide 22 (64% yield) with the correct quaternary carbon center.
Deoxygenation of the ketone group of 22 was investigated with three approaches (Wolff–Kishner–Huang reduction, Bamford–Stevens reduction, and thioketalization/desulfurization) and thioketalization/desulfurizaiton was the most effective to give 24 in 48% yield over 2 steps. A well-developed 2-step sequence, N-alkylation and intramolecular SN2 substitution, was performed to furnish 1,2-dehydroaspidospermidine (26),14 which could serve as a common precursor to vincadifformine (27, 48% yield), winchinine B (28, 61% yield), aspidospermidine (29, 83% yield), and N-acetylaspidospermidine (30) (87%) in a single or two steps. All spectroscopic data of our 6 synthetic aspidosperma alkaloids were in good agreement with those reported for the natural alkaloids (see ESI†).
Conclusion
In summary, we have developed an efficient synthetic methodology to couple indoles and functionalized piperidines with C3–C2′ linkage for the synthesis of uleine and aspidosperma alkaloids. The coupling reaction is effectively promoted by Brønsted acid [H+: trifluoroacetic acid or 1,2,3,4,5-pentacarbomethoxycyclopentadiene (PCCP)] at room temperature with broad substrate scope (33 examples) and diverse functional group tolerance. As compared to previous intramolecular coupling (C2–C2′ linkage with bridged nitrogen), this intermolecular aza-Friedel–Crafts (iAFC) reaction greatly expands the synthetic utility of aza-Achmatowicz rearrangement (AAR) with high skeletal diversity, permitting access to different types of indole alkaloids. We demonstrated such advantage by using the AAR-iAFC coupling products as starting materials to accomplish the efficient total synthesis (7–12 steps) of 9 indole alkaloids consisting of two subclasses: uleine and aspidosperma, namely (+)-3-epi-N-nor-dasycarpidone, (+)-3-epi-dasycarpidone, (+)-3-epi-uleine, 1,2-didehydropseudoaspidospermidine, 1,2-dehydroaspidospermidine, vincadifformine, winchinine B, aspidospermidine, and N-acetylaspidospermidine. We expect that such high efficient coupling reaction (AAR-iAFC) will find wide application in total synthesis of other indole alkaloids and pave the way for medicinal chemistry and drug discovery.
Data availability
Synthetic procedures and complete characterization data for all new compounds can be found in the ESI.†
Author contributions
F. M., Y. L., K. A., H. Q. and Y. T. C. performed the synthetic experiments. Z. G. provided some suggestions on the work. R. T. conceptualized and directed the project and drafted the manuscript with assistance from all co-authors.
Conflicts of interest
The authors declare no competing interests.
Acknowledgements
This research was financially supported by the Research Grants Council of Hong Kong (C6026-19G, C6022-22W, 16306920, 16300921, 16308922, and 16304023).
Notes and references
- A. E. Mohammed, Z. H. Abdul-Hameed, M. O. Alotaibi, N. O. Bawakid, T. R. Sobahi, A. Abdel-Lateff and W. M. Alarif, Molecules, 2021, 26, 488 CrossRef CAS PubMed.
- D. Williams and V. De Luca, Phytochem. Rev., 2023, 22, 309–338 CrossRef CAS.
- M.-J. U. Ferreira and A. Paterna, Phytochem. Rev., 2019, 18, 971–987 CrossRef CAS.
- F. Omar, A. M. Tareq, A. M. Alqahtani, K. Dhama, M. A. Sayeed, T. B. Emran and J. Simal-Gandara, Molecules, 2021, 26, 2297 CrossRef CAS PubMed.
- S.-F. Teng, F.-R. Li, Q.-M. Cui, A. Khan, T. He, X.-D. Luo, Y.-P. Liu and G.-G. Cheng, Phytochem. Rev., 2023 DOI:10.1007/s11101-023-09871-2.
- J. Song, B. Zhang, M. Li and J. Zhang, Fitoterapia, 2023, 165, 105430 CrossRef CAS PubMed.
- L. Wang, S. Chen, X. Gao, X. Liang, W. Lv, D. Zhang and X. Jin, J. Enzyme Inhib. Med. Chem., 2023, 38, 2155639 CrossRef PubMed.
- S. Zhao, G. Sirasani and R. B. Andrade, Alkaloids: Chem. Biol., 2021, 86, 1–143 CAS.
- V. L. de Almeida, C. G. Silva, A. F. Silva, P. R. V. Campana, K. Foubert, J. C. D. Lopes and L. Pieters, J. Ethnopharmacol., 2019, 231, 125–140 CrossRef CAS PubMed.
-
J. M. Lopchuk, in Prog. Heterocycl. Chem., ed. G. W. Gribble and J. A. Joule, Elsevier, 2011, vol. 23, pp. 1–25 Search PubMed.
- N. Wang, X. Xiao, C.-X. Liu, H. Yao, N. Huang and K. Zou, Adv. Synth. Catal., 2022, 364, 2479–2501 CrossRef CAS.
- R. Tanifuji, A. Minami, H. Oguri and H. Oikawa, Nat. Prod. Rep., 2020, 37, 1098–1121 RSC.
- L. McMurray, E. M. Beck and M. J. Gaunt, Angew. Chem., Int. Ed., 2012, 51, 9288–9291 CrossRef CAS PubMed.
- S. A. Kozmin, T. Iwama, Y. Huang and V. H. Rawal, J. Am. Chem. Soc., 2002, 124, 4628–4641 CrossRef CAS PubMed.
- E. L. Campbell, A. M. Zuhl, C. M. Liu and D. L. Boger, J. Am. Chem. Soc., 2010, 132, 3009–3012 CrossRef CAS PubMed.
- J. M. Saya, E. Ruijter and R. V. A. Orru, Chem.–Eur. J., 2019, 25, 8916–8935 CrossRef CAS PubMed.
-
J. E. Saxton, in Alkaloids Chem. Biol., ed. G. A. Cordell, Academic Press, 1998, vol. 50, pp. 343–376 Search PubMed.
- H. Ohno and S. Inuki, Org. Biomol. Chem., 2021, 19, 3551–3568 RSC.
- X. Zeng, V. Shukla and D. L. Boger, J. Org. Chem., 2020, 85, 14817–14826 CrossRef CAS PubMed.
- O. Wagnières, Z. Xu, Q. Wang and J. Zhu, J. Am. Chem. Soc., 2014, 136, 15102–15108 CrossRef PubMed.
- L.-P. Zhao, P.-J. Li, L. Wang and Y. Tang, Angew. Chem., Int. Ed., 2022, 61, e202207360 CrossRef CAS PubMed.
- B. Horst, D. S. Verdoorn, S. Hennig, G. van der Heijden and E. Ruijter, Angew. Chem., Int. Ed., 2022, 61, e202210592 CrossRef CAS PubMed.
- Z. Xu, Q. Wang and J. Zhu, Chem. Soc. Rev., 2018, 47, 7882–7898 RSC.
- S. B. Jones, B. Simmons, A. Mastracchio and D. W. C. MacMillan, Nature, 2011, 475, 183–188 CrossRef CAS PubMed.
- L. Pan, C.-W. Zheng, G.-S. Fang, H.-R. Hong, J. Liu, L.-H. Yu and G. Zhao, Chem.–Eur. J., 2019, 25, 6306–6310 CrossRef CAS PubMed.
- H. Mizoguchi, H. Oikawa and H. Oguri, Nat. Chem., 2014, 6, 57–64 CrossRef CAS PubMed.
- C. Zheng and S.-L. You, Nat. Prod. Rep., 2019, 36, 1589–1605 RSC.
- B. Qin, Y. Wang, X. Wang and Y. Jia, Org. Chem. Front., 2021, 8, 369–383 RSC.
- B. Qin, Z. Lu and Y. Jia, Angew. Chem., Int. Ed., 2022, 61, e202201712 CrossRef CAS PubMed.
- M. Amat, M. Pérez, N. Llor, C. Escolano, F. J. Luque, E. Molins and J. Bosch, J. Org. Chem., 2004, 69, 8681–8693 CrossRef CAS PubMed.
- M. Amat, M. Pérez, N. Llor, M. Martinelli, E. Molins and J. Bosch, Chem. Commun., 2004, 1602–1603 RSC.
- M. Amat, C. Escolano, A. Gómez-Esqué, O. Lozano, N. Llor, R. Griera, E. Molins and J. Bosch, Tetrahedron: Asymmetry, 2006, 17, 1581–1588 CrossRef CAS.
- V. Semak, C. Escolano, C. Arróniz, J. Bosch and M. Amat, Tetrahedron: Asymmetry, 2010, 21, 2542–2549 CrossRef CAS.
- E. A. Peterson and E. N. Jacobsen, Angew. Chem., Int. Ed., 2009, 48, 6328–6331 CrossRef CAS PubMed.
- Y. Zhang, Z.-J. Yi, A.-M. Yang, J.-M. Guo, X. Li and B.-G. Wei, Org. Biomol. Chem., 2023, 21, 1303–1315 RSC.
- J. Song, Z.-J. Zhang and L.-Z. Gong, Angew. Chem., Int. Ed., 2017, 56, 5212–5216 CrossRef CAS PubMed.
- B. K. Corkey, S. T. Heller, Y.-M. Wang and F. D. Toste, Tetrahedron, 2013, 69, 5640–5646 CrossRef CAS PubMed.
- M. Amat, B. Checa, N. Llor, M. Pérez and J. Bosch, Eur. J. Org Chem., 2011, 898–907 CrossRef CAS.
- C. L. Martin, L. E. Overman and J. M. Rohde, J. Am. Chem. Soc., 2010, 132, 4894–4906 CrossRef CAS PubMed.
- E. Wenkert and T. Hudlicky, J. Org. Chem., 1988, 53, 1953–1957 CrossRef CAS.
- N. Wang and X. Jiang, Chem. Rec., 2021, 21, 295–314 CrossRef CAS PubMed.
- N. Wang, S. Du, D. Li and X. Jiang, Org. Lett., 2017, 19, 3167–3170 CrossRef CAS PubMed.
- G. Martin, P. Angyal, O. Egyed, S. Varga and T. Soós, Org. Lett., 2020, 22, 4675–4679 CrossRef CAS PubMed.
- R. Iyengar, K. Schildknegt, M. Morton and J. Aubé, J. Org. Chem., 2005, 70, 10645–10652 CrossRef CAS PubMed.
- S. Katahara, Y. Sugiyama, M. Yamane, Y. Komiya, T. Sato and N. Chida, Org. Lett., 2021, 23, 3058–3063 CrossRef CAS PubMed.
- J.-Y. Kim, C.-H. Suhl, J.-H. Lee and C.-G. Cho, Org. Lett., 2017, 19, 6168–6171 CrossRef CAS PubMed.
- M. M. Heravi, S. Rohani, V. Zadsirjan and N. Zahedi, RSC Adv., 2017, 7, 52852–52887 RSC.
- F. Reuß and P. Heretsch, Org. Lett., 2020, 22, 3956–3959 CrossRef PubMed.
- S. Varga, P. Angyal, G. Martin, O. Egyed, T. Holczbauer and T. Soós, Angew. Chem., Int. Ed., 2020, 59, 13547–13551 CrossRef CAS PubMed.
- B. Delayre, C. Piemontesi, Q. Wang and J. Zhu, Angew. Chem., Int. Ed., 2020, 59, 13990–13997 CrossRef CAS PubMed.
- R. Yang and F. G. Qiu, Angew. Chem., Int. Ed., 2013, 52, 6015–6018 CrossRef CAS PubMed.
- X.-L. Shen, R.-R. Zhao, M.-J. Mo, F.-Z. Peng, H.-B. Zhang and Z.-H. Shao, J. Org. Chem., 2014, 79, 2473–2480 CrossRef CAS PubMed.
- S. Zhao and R. B. Andrade, J. Am. Chem. Soc., 2013, 135, 13334–13337 CrossRef CAS PubMed.
- Z. Liu, X. Ju, S. Ma, C. Du, W. Zhang, H. Li, X. Wang, X. Xie and X. She, J. Org. Chem., 2019, 84, 14994–15000 CrossRef CAS PubMed.
- D. L. Cain, N. A. Anderson, D. B. Cordes, A. M. Z. Slawin and A. J. B. Watson, J. Org. Chem., 2022, 87, 15559–15563 CrossRef CAS PubMed.
- H. Ma, X. Xie, P. Jing, W. Zhang and X. She, Org. Biomol. Chem., 2015, 13, 5255–5259 RSC.
- W. Chen, X.-D. Yang, W.-Y. Tan, X.-Y. Zhang, X.-L. Liao and H. Zhang, Angew. Chem., Int. Ed., 2017, 56, 12327–12331 CrossRef CAS PubMed.
- L. Yang, S. Huang, R. Huang, A. Hou, S. Zhang, H. Su, X. Ding, B. Lin, M. Cheng and Y. Liu, Org. Lett., 2021, 23, 6471–6476 CrossRef CAS PubMed.
- J.-Y. Du, C. Zeng, X.-J. Han, H. Qu, X.-H. Zhao, X.-T. An and C.-A. Fan, J. Am. Chem. Soc., 2015, 137, 4267–4273 CrossRef CAS PubMed.
- H. Xu, H. Huang, C. Zhao, C. Song and J. Chang, Org. Lett., 2019, 21, 6457–6460 CrossRef CAS PubMed.
- Z. Li, S. Zhang, S. Wu, X. Shen, L. Zou, F. Wang, X. Li, F. Peng, H. Zhang and Z. Shao, Angew. Chem., Int. Ed., 2013, 52, 4117–4121 CrossRef CAS PubMed.
- D. Jiang, P. Tang, H. Xiong, S. Lei, Y. Zhang, C. Zhang, L. He, H. Qiu and M. Zhang, Angew. Chem., Int. Ed., 2023, 62, e202307286 CrossRef CAS PubMed.
- M. Mewald, J. W. Medley and M. Movassaghi, Angew. Chem., Int. Ed., 2014, 53, 11634–11639 CrossRef CAS PubMed.
- T. Sato, M. Yoritate, H. Tajima and N. Chida, Org. Biomol. Chem., 2018, 16, 3864–3875 RSC.
- F. De Simone, J. Gertsch and J. Waser, Angew. Chem., Int. Ed., 2010, 49, 5767–5770 CrossRef CAS PubMed.
- K. Yoshida, K. Okada, H. Ueda and H. Tokuyama, Angew. Chem., Int. Ed., 2020, 59, 23089–23093 CrossRef CAS PubMed.
- T. Kang, K. L. White, T. J. Mann, A. H. Hoveyda and M. Movassaghi, Angew. Chem., Int. Ed., 2017, 56, 13857–13860 CrossRef CAS PubMed.
- I.-S. Myeong, N. H. Avci and M. Movassaghi, Org. Lett., 2021, 23, 9118–9122 CrossRef CAS PubMed.
- P. W. Tan, J. Seayad and D. J. Dixon, Angew. Chem., Int. Ed., 2016, 55, 13436–13440 CrossRef CAS PubMed.
- M. V. Mijangos and L. D. Miranda, Org. Biomol. Chem., 2018, 16, 9409–9419 RSC.
- M. A. Toczko and C. H. Heathcock, J. Org. Chem., 2000, 65, 2642–2645 CrossRef CAS PubMed.
- Y. Han-ya, H. Tokuyama and T. Fukuyama, Angew. Chem., Int. Ed., 2011, 50, 4884–4887 CrossRef CAS PubMed.
- E. E. van Tamelen and G. G. Knapp, J. Am. Chem. Soc., 1955, 77, 1860–1862 CrossRef CAS.
- E. Wenkert, K. G. Dave, F. Haglid, R. G. Lewis, T. Oishi, R. V. Stevens and M. Terashima, J. Org. Chem., 1968, 33, 747–753 CrossRef CAS PubMed.
- E. Wenkert and T. Hudlicky, J. Org. Chem., 1988, 53, 1953–1957 CrossRef CAS.
- A. Hadj Mohamed and N. Masurier, Org. Chem. Front., 2023, 10, 1847–1866 RSC.
- S. Brunen, B. Mitschke, M. Leutzsch and B. List, J. Am. Chem. Soc., 2023, 145, 15708–15713 CrossRef CAS PubMed.
- Y.-S. Cheng, S.-H. Chan, G. A. Rao, R. Gurubrahamam and K. Chen, Adv. Synth. Catal., 2021, 363, 3502–3506 CrossRef CAS.
- F. van der Pijl, F. L. van Delft and F. P. J. T. Rutjes, Eur. J. Org Chem., 2015, 2015, 4811–4829 CrossRef CAS.
- F. Ma, C. He, E. Wang and R. Tong, Org. Lett., 2021, 23, 6583–6588 CrossRef CAS PubMed.
- W. F. Cheng, S. Ma, Y. T. Lai, Y. T. Cheung, K. Akkarasereenon, Y. Zhou and R. Tong, Angew. Chem., Int. Ed., 2023, 62, e202311671 CrossRef CAS PubMed.
- L. Liang, L.-D. Guo and R. Tong, Acc. Chem. Res., 2022, 55, 2326–2340 CrossRef CAS PubMed.
- L. Zhang, Y. Zhang, W. Li and X. Qi, Angew. Chem., Int. Ed., 2019, 58, 4988–4991 CrossRef CAS PubMed.
- Y. Zhang, L. Zhang and X. Qi, Angew. Chem., Int. Ed., 2023, 62, e202304435 CrossRef CAS PubMed.
- Z. Li and R. Tong, J. Org. Chem., 2016, 81, 4847–4855 CrossRef CAS PubMed.
- G. Zhao and R. Tong, Green Chem., 2019, 21, 64–68 RSC.
- G. Zhao and R. Tong, Tetrahedron, 2019, 75, 1669–1675 CrossRef CAS.
- T. Ahmad, S. Khan and N. Ullah, ACS Omega, 2022, 7, 35446–35485 CrossRef CAS PubMed.
- I. E. Mikhailov, G. A. Dushenko and V. I. Minkin, Russ. J. Org. Chem., 2021, 57, 1757–1808 CrossRef CAS.
- C. Yuan, J. Li and P. Li, ACS Omega, 2018, 3, 6820–6826 CrossRef CAS PubMed.
- C. D. Gheewala, B. E. Collins and T. H. Lambert, Science, 2016, 351, 961–965 CrossRef CAS PubMed.
- E. J. Corey, R. K. Bakshi and S. Shibata, J. Am. Chem. Soc., 1987, 109, 5551–5553 CrossRef CAS.
- E. J. Corey, R. K. Bakshi, S. Shibata, C. P. Chen and V. K. Singh, J. Am. Chem. Soc., 1987, 109, 7925–7926 CrossRef CAS.
- R. Noyori and S. Hashiguchi, Acc. Chem. Res., 1997, 30, 97–102 CrossRef CAS.
- E. C. Ashby, J. J. Lin and R. Kovar, J. Org. Chem., 1976, 41, 1939–1943 CrossRef CAS.
- M. Amat, S. Hadida, N. Llor, S. Sathyanarayana and J. Bosch, J. Org. Chem., 1996, 61, 3878–3882 CrossRef CAS PubMed.
- Z. E. Dos Santos Torres, E. R. Silveira, L. F. Rocha e Silva, E. S. Lima, M. C. De Vasconcellos, D. E. De Andrade Uchoa, R. B. Filho and A. M. Pohlit, Molecules, 2013, 18, 6281–6297 CrossRef CAS PubMed.
- T. Kametani and T. Suzuki, J. Org. Chem., 1971, 36, 1291–1293 CrossRef CAS.
- M. L. Bennasar, T. Roca and D. García-Díaz, J. Org. Chem., 2008, 73, 9033–9039 CrossRef CAS PubMed.
- P. Forns, A. Diez, M. Rubiralta, X. Solans and M. Font-Bardia, Tetrahedron, 1996, 52, 3563–3574 CrossRef CAS.
- B. Delayre, C. Fung, Q. Wang and J. Zhu, Helv. Chim. Acta, 2021, 104, e2100088 CrossRef CAS.
- D. J. Edmonds, D. Johnston and D. J. Procter, Chem. Rev., 2004, 104, 3371–3404 CrossRef CAS PubMed.
- M. M. Heravi and A. Nazari, RSC Adv., 2022, 12, 9944–9994 RSC.
- M. Szostak, N. J. Fazakerley, D. Parmar and D. J. Procter, Chem. Rev., 2014, 114, 5959–6039 CrossRef CAS PubMed.
- K. Hagiwara, T. Tabuchi, D. Urabe and M. Inoue, Chem. Sci., 2016, 7, 4372–4378 RSC.
- E. Wenkert, B. Porter, D. P. Simmons, J. Ardisson, N. Kunesch and J. Poisson, J. Org. Chem., 1984, 49, 3733–3742 CrossRef CAS.
- K. C. Nicolaou, T. Montagnon, P. S. Baran and Y. L. Zhong, J. Am. Chem. Soc., 2002, 124, 2245–2258 CrossRef CAS PubMed.
|
This journal is © The Royal Society of Chemistry 2024 |
Click here to see how this site uses Cookies. View our privacy policy here.