DOI:
10.1039/D4SC02002B
(Edge Article)
Chem. Sci., 2024,
15, 10477-10490
How does ferrocene correlate with ferroptosis? Multiple approaches to explore ferrocene-appended GPX4 inhibitors as anticancer agents†
Received
26th March 2024
, Accepted 31st May 2024
First published on 31st May 2024
Abstract
Ferroptosis has emerged as a form of programmed cell death and exhibits remarkable promise for anticancer therapy. However, it is challenging to discover ferroptosis inducers with new chemotypes and high ferroptosis-inducing potency. Herein, we report a new series of ferrocenyl-appended GPX4 inhibitors rationally designed in a “one stone kills two birds” strategy. Ferroptosis selectivity assays, GPX4 inhibitory activity and CETSA experiments validated the inhibition of novel compounds on GPX4. In particular, the ROS-related bioactivity assays highlighted the ROS-inducing ability of 17 at the molecular level and their ferroptosis enhancement at the cellular level. These data confirmed the dual role of ferrocene as both the bioisostere motif maintaining the inhibition capacity of certain molecules with GPX4 and also as the ROS producer to enhance the vulnerability to ferroptosis of cancer cells, thereby attenuating tumor growth in vivo. This proof-of-concept study of ferrocenyl-appended ferroptosis inducers via rational design may not only advance the development of ferroptosis-based anticancer treatment, but also illuminate the multiple roles of the ferrocenyl component, thus opening the way to novel bioorganometallics for potential disease therapies.
Introduction
Ferroptosis was defined in 2012 as an iron-dependent form of programmed cell death caused by increased cellular reactive oxygen species (ROS) and lipid peroxidation (LPO).1 Lipid metabolism, ROS biology, and iron regulation have been summarized as intersections from the centrality of the framework of ferroptosis.2 In the past few decades, ferroptosis has been proven to engage in human diseases including cancer, neurodegeneration and ischemic disease.3–9 Notably, accumulating evidence shows that ferroptosis plays important roles in cancer occurrence, development and metastasis, which highlights its great potential for the treatment of refractory tumors.10 A deeper understanding of the epithelial–mesenchymal transition (EMT) in metastasis underscores the critical role of glutathione peroxidase 4 (GPX4) in cancer cells undergoing EMT; it is the only peroxidase in mammals capable of reducing lipid hydroperoxides.11,12 Indeed, when GPX4 function is impaired, lipid peroxidation causes ferroptosis which is a tumor-suppressive process. Moreover, further research suggested that blocking GPX4 function or decreasing the protein level of GPX4 can suppress tumor growth.13 Thus, aggressive neoplastic diseases might be treated through the use of GPX4 inhibitors.
Iron homeostasis composes the essential part of ferroptosis which is mainly mediated via the Fenton chain reaction.14,15 Therefore, elevated levels of iron can increase the vulnerability to ferroptosis. The traditional Fenton system composed of H2O2 and Fe2+ has been intensively investigated and used in cancer treatment. The ferrocenyl group is perhaps the most widely studied organometallic moiety and enables the generation of ROS, which has contributed to its wide-spread exploration for use in medicinal chemistry.16 The electron donor–acceptor ability of the central iron atom endows ferrocene with good redox characteristics and high catalytic capacity as an excellent Fenton catalyst, which effectively enhances the biological behavior of the original drugs.17–20 Considering the high level of interest in ferroptosis, whose impetus towards the formation of lipid hydroperoxides arises from the iron-catalyzed Fenton reaction, exploration of the effect of ferrocene on the framework of ferroptosis (Fig. 1A) could be a valuable contribution. Thus far, however, comprehensive investigation of ferrocene in terms of ferroptosis inducers has been rather limited, and reported only for iron/ferrocene-based inorganic complexes or nanomaterials.21–24
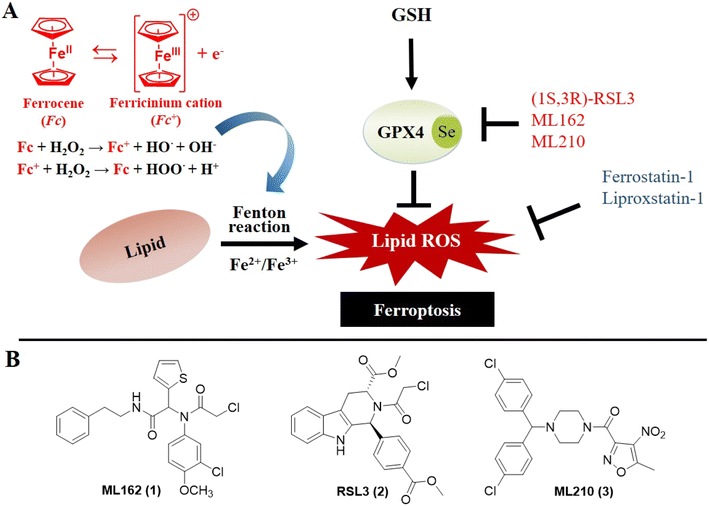 |
| Fig. 1 (A) Illustration of the basis of developing ferrocene-based ferroptosis inducers. (B) Typical small molecule ferroptosis inducers. | |
In recent years, transition metal bioorganometallic chemistry has gradually come to the fore regarding its potential for the development of metallodrugs to circumvent the drawbacks25–31 associated with platinum complexes.32–37 Amongst the organometallic complexes that can be used as antitumoral agents, ferrocene occupies a privileged position as a compact, stable, non-toxic metallocene not only acting as a bioisostere for aryl/heteroaryl rings but also interfering in cellular redox homeostasis.17,18,38–41 Initially, a library of ferrocene-based compounds (>100 entries) was established and then used to screen for ferroptosis inducers. Unfortunately, none of these random ferrocenyl-bearing entities could be characterized as ferroptosis inducers, although some have moderate cytotoxicities. These results indicated that regular ferrocenyl entities may not be able to induce ferroptosis significantly, which contradicted the conservative perception of ferrocene for ferroptosis and drove us towards the rational design of ferrocene-based ferroptosis inducers.
Thus, a “one stone kills two birds” strategy has been developed in this paper integrating the ferrocene fragment into typical ferroptosis inducers, such as the widely used GPX4 inhibitors (ML162, RSL3 and ML210, Fig. 1B). We here describe multiple approaches towards the development of ferrocene-appended GPX4 inhibitors as anticancer agents, including structure-based drug design (SBDD), ferroptosis-targeted phenotypic evaluation and other molecular and cellular investigations. The ferroptosis selectivity assays, GPX4 inhibitory activity and CETSA experiments validated the inhibition of these novel compounds on GPX4. The ROS-related bioactivity assays emphasized the ROS-inducing ability of selected compounds at the molecular level and their ferroptosis enhancement at the cellular level. These data confirmed the dual role of ferrocene as both the bioisostere fragment maintaining the binding capacity of certain molecules with GPX4 and also as the ROS producer enhancing the vulnerability to ferroptosis of cancer cells, thereby attenuating tumor growth in vivo. This work describes a proof-of-concept study of ferrocene-appended dual-function ferroptosis inducers for cancer therapies via a rational design procedure, and consummated the potential application of ferrocene in ferroptosis-targeted drug development.
Results and discussion
Ferrocene-based organometallic compound library construction and screening
The bioorganometallic community has explored the use of ferrocenyl compounds as inhibitors or as auxiliary groups to augment the efficacy of existing organic moieties, and some of them, such as ferroquine,42 have entered clinical trials. In an initial approach to explore the potential of ferrocenyl compounds as ferroptosis inducers, an in-house ferrocene-based compound library was constructed, consisting of simple mono- or di-substituted ferrocenes, known ferrocene-based drug candidates such as the ferrocifen derivatives developed in our own laboratories,43–46 aminoferrocene analogues from the Mokhir group40,47 and ferroquine, as well as several chiral ferrocene derivatives reported by the You group48–50 (Fig. 2A). Approximately 10% of the library was purchased from commercial sources and used without further modification, while the majority of the remaining complexes were either generous gifts from the original laboratory, or were prepared according to literature procedures. Initially, a primary screening was carried out for ferrocene complexes at two concentrations (1 and 10 μM) on ferroptosis-sensitive HT1080 cells, with or without ferrostatin-1 (fer-1), a known specific ferroptosis inhibitor.1 For the complexes having moderate antiproliferative activities, their IC50 values were further tested to double check their ferroptosis selectivity. Unfortunately, although some of these organometallics can inhibit proliferation of HT1080 cells at the micromolar level, these cytotoxicities cannot be reversed significantly by the ferroptosis inhibitor fer-1, as shown in Fig. S1.† These results indicated that the antiproliferative effect of the tested ferrocene complexes may not be relevant to ferroptosis, even though some molecules have been claimed to produce ROS significantly at both the molecular and cellular levels including ferrocifen and amino-ferrocene derivatives.40,51 These observations suggested there is no immediate connection between ferrocene and ferroptosis, which highlights its unique mechanism.
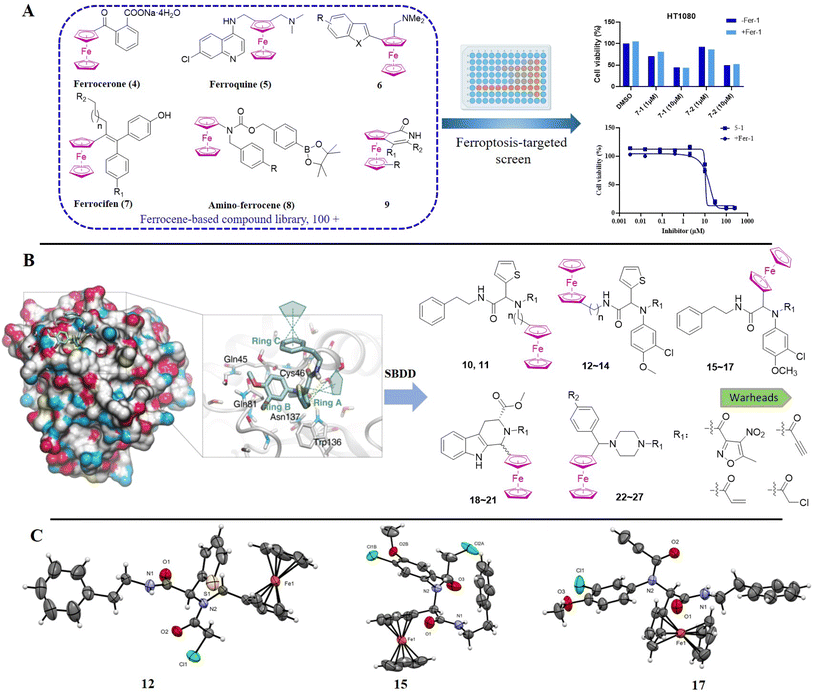 |
| Fig. 2 (A) Illustration of the ferrocene-based organometallic compound library construction and screening. (B) Structure-based drug design (SBDD) of ferrocene-appended GPX4 inhibitors. (C) Molecular structures of the ferrocene complexes 12, 15 and 17 with thermal ellipsoids shown at 50% probability. | |
Rational design and synthesis of ferrocene-appended GPX4 inhibitors
Apparently, the currently available ferrocene-based chemical entities could not induce ferroptosis, which prompted us to explore the effect of ferrocene in terms of ferroptosis via a rational design strategy. Inhibition of GPX4 with small molecules has been established as a pharmacological stimulator of ferroptosis, which can achieve durable responses in a range of cancer types supported by evidence both in vitro and in vivo.52,53 The most well-known and potent GPX4 inhibitors involve two covalent binders RSL3 and ML162 having chemically reactive chloroacetamides as warheads, as well as an additional prodrug, ML210, that is converted to a nitrile oxide reactant in cells.54 In a preliminary analysis of the cocrystalline structure of GPX4 with its covalent inhibitor ML162 in a monomer model,55 we noted that the solvent-exposed area of the ligand ML162 exceeds 60%, especially in terms of rings A and C (Fig. 2B). Assuming that the under-occupied lipophilic pocket around rings A and C may be able to accommodate the ferrocenyl sandwich motif, this could provide an approach towards targeting GPX4 by ferrocene-appended GPX4 inhibitors.
To this end, 18 covalent GPX4 inhibitors with a ferrocenyl substituent incorporated at varied positions were synthesized so as to replace the aromatic ring of ML162, RSL3 and ML210, as shown in Fig. 2B. Efficient syntheses of the designed ferrocenyl dipeptide analogues 10–17 proceeded in moderate to good yields, each via a Ugi four-component reaction from a diversity of reactants, aryl aldehydes, amines, carboxylic acids and isocyanides (Scheme S2†). The synthesis of ferrocenyl RSL3 derivatives 18–21 was accomplished by Pictet–Spengler condensation of tryptophan analogues with formylferrocene, followed by an amide coupling (Scheme S3†). It took several steps to prepare the third series, and ferrocenyl compounds 22–27 were each obtained in a multi-step process (Scheme S4†). Benzoylferrocene derivatives were first prepared by a Friedel–Crafts acylation and then reduced, using sodium borohydride, to the corresponding alcohols FC-2a/2b. Treatment of FC-2a/2b with acetic anhydride provided the ester analogues, and was followed by treatment with excess piperazine in refluxing acetonitrile to afford FC-3a/3b. Coupling of secondary amines FC-3a/3b with carboxylic acids or acyl chlorides in dichloromethane delivered the final compounds 22–27. The molecular structures of 12, 15 and 17 were unequivocally established by X-ray crystallography, as illustrated in Fig. 2C and Tables S5–S8.†
In vitro anticancer evaluations and cellular uptake measurements
As mentioned above, a preliminary screening on ferroptosis-sensitive HT1080 cells, with or without fer-1, has been routinely used to evaluate the anticancer activity of our molecules (as shown in Table 1). Encouragingly, in the case of ML162 analogues, the designed compound 15 with a ferrocenyl group instead of 2-thienyl showed comparable antitumor activity and ferroptosis selectivity to that of ML162. However, introducing the ferrocenyl group at either the R2 or R4 position on the dipeptide scaffold of ML162 seems to have a negative influence on both the antitumor activity and the ferroptosis selectivity. Compounds 10–14 displayed only moderate cytotoxicity on HT1080 cells without significant ferroptosis selectivity. More interestingly, the ferroptosis-inducing ability of dipeptide analogues bearing a ferrocenyl group can be modulated by changing the covalent warhead. Compound 17 possessing a propiolamide as its warhead showed a large improvement in antitumoral activity on HT1080 with IC50 values at the nanomolar level as well as excellent ferroptosis selectivity. However, replacing chloroacetamide with acrylamide, as in 16, resulted in a very dramatic loss of activity relative to that of ML162. The best combination therefore appears to be a ferrocenyl group at the R3 position and a propiolamide as the warhead.
Table 1 Antitumor activities and ferroptosis selectivity of target compounds towards HT1080 cells
Comp. |
R1 |
R2 |
R3 |
R4 |
HT1080, IC50a (μM) |
Selectivityb |
−fer-1 |
+fer-1 |
Values are expressed as the mean of three independent experiments ± SD.
Selectivity: HT1080 IC50 with fer-1/HT1080 IC50 without fer-1.
|
|
10
|
|
|
|
|
1.044 ± 0.201 |
1.699 ± 0.014 |
1.6 |
11
|
|
|
|
|
4.980 ± 0.954 |
6.732 ± 1.228 |
1.4 |
12
|
|
|
|
|
2.520 ± 0.290 |
1.749 ± 0.238 |
0.7 |
13
|
|
|
|
|
4.866 ± 1.087 |
2.982 ± 0.667 |
0.6 |
14
|
|
|
|
|
1.449 ± 0.069 |
4.068 ± 0.520 |
2.8 |
15
|
|
|
|
|
0.043 ± 0.007 |
2.772 ± 0.231 |
64.5 |
16
|
|
|
|
|
8.510 ± 1.371 |
9.391 ± 0.459 |
1.1 |
17
|
|
|
|
|
0.007 ± 0.001 |
1.486 ± 0.193 |
212.3 |
ML162
|
0.036 ± 0.013 |
2.200 ± 1.320 |
61.1 |
In contrast to the diverse role of ferrocene in the antitumor behavior of ML162, incorporation of the ferrocenyl unit in RSL3 and ML210 by replacing the phenyl with ferrocenyl generally triggers a global loss of activity (as shown in Table S9†). In particular, in the analogues of ML210, all synthesized compounds bearing ferrocene show diminished ferroptosis-inducing activity, even with varied covalent warheads. One should note that, in the RSL3 series, only ferrocenyl complex 21 possessing a propiolamide warhead exhibited approximately two-fold decreased antitumor activity, and five-fold reduced ferroptosis selectivity, compared to that of RSL3. These data emphasized the complexity of the effect of ferrocene on these three classical ferroptosis inducers in terms of their antitumoral activities and ferroptosis selectivity.
Inspired by the above results, we next attempted to evaluate the cytotoxicity effects of certain molecules on more cancers including ferroptosis-susceptible human renal cell carcinoma (OS-RC2), triple negative mouse breast cancer (TNBC 4T1), as well as two non-tumorigenic cell lines (L02 and MCF-10A), as shown in Table 2. OS-RC2 cells display high vulnerability to ferroptosis and are widely used to evaluate the specificity of compounds to ferroptosis, and the inhibitory activities of compounds against the GPX4 enzyme were evaluated on this cell line at 1 μM. Gratifyingly, ferrocene-appended compounds 15 and 17 exhibited outstanding ferroptosis selectivity and potent inhibition of GPX4 function, which was validated upon the exceptional reversal of cytotoxicity by the ferroptosis inhibitor. These data were in line with their antitumoral behaviors on the aforementioned ferroptosis-sensitive HT1080 cells. It is interesting to see that compounds 12 and 13, each with a ferrocene group at the R4 position on the ML162 scaffold, bring about more vulnerability on OS-RC2 cells than HT1080 cells, and these two compounds also possess certain inhibitory activity on the GPX4 enzyme. This indicated that the growth of OS-RC2 may be more dependent on the function of GPX4. This phenomenon has also been observed for the ferrocene-appended RSL analogue 21 which has remarkable ferroptosis-inducing ability on OS-RC2 with a selectivity of 103.9 superior to that of HT1080. An obvious decrease in the cytotoxic activity was observed in the case of active derivatives on TNBC 4T1 cells, which indicated the distinctive damage wrought by ferroptosis on multiple cancer cells. On the other hand, compounds 15 and 17 display weaker cytotoxicity on human normal cells of L02 and MCF-10A compared to that of ML162, and this criterion indicates that they may be good candidates for drug development.
Table 2 Antitumor activities and ferroptosis selectivity of selected compounds on other cell lines: human renal cell carcinoma (OS-RC2), triple negative mouse breast cancer (4T1), normal liver cells (L-02) and normal breast cells (MCF-10A)
Comp. |
OS-RC2, IC50a (μM) |
Selectivityb |
GPX4 inhibition (%) at 1.0 μM |
4T1 IC50 (μM) |
L02 IC50 (μM) |
MCF-10A IC50 (μM) |
−fer-1 |
+fer-1 |
Values are expressed as the mean of three independent experiments ± SD.
Selectivity: OS-RC2 IC50 with fer-1/OS-RC2 IC50 without fer-1.
|
12
|
0.055 ± 0.017 |
10.16 ± 0.28 |
185 |
25.40 ± 4.24 |
0.634 ± 0.074 |
4.98 ± 0.87 |
NT |
13
|
0.027 ± 0.003 |
2.42 ± 0.70 |
89.6 |
42.37 ± 1.93 |
0.192 ± 0.008 |
14.87 ± 5.78 |
7.81 ± 0.49 |
15
|
0.023 ± 0.005 |
7.687 ± 0.030 |
334.2 |
34.02 ± 4.94 |
0.169 ± 0.008 |
15.38 ± 4.35 |
7.79 ± 0.44 |
17
|
0.009 ± 0.001 |
6.917 ± 1.211 |
768.6 |
49.16 ± 4.60 |
0.095 ± 0.006 |
3.54 ± 0.56 |
7.32 ± 0.30 |
18
|
4.805 ± 0.364 |
4.281 ± 0.275 |
0.9 |
17.88 ± 1.03 |
NT |
4.04 ± 0.79 |
NT |
19
|
6.584 ± 0.442 |
4.023 ± 0.307 |
0.6 |
12.80 ± 2.67 |
NT |
9.98 ± 2.69 |
NT |
20
|
26.320 ± 0.930 |
25.560 ± 0.515 |
0.9 |
17.60 ± 11.13 |
12.930 |
15.76 ± 2.78 |
NT |
21
|
0.028 ± 0.003 |
2.909 ± 0.589 |
103.9 |
15.74 ± 0.34 |
2.63 ± 0.6 |
4.27 ± 1.55 |
6.77 ± 0.11 |
ML162
|
0.021 ± 0.009 |
2.574 ± 0.49 |
122.3 |
45.02 ± 1.40 |
0.516 ± 0.059 |
1.20 ± 0.26 |
2.93 ± 0.12 |
RSL3
|
0.024 ± 0.004 |
0.69 ± 0.32 |
28.8 |
46.14 ± 5.44 |
0.34 ± 0.062 |
0.89 ± 0.13 |
16.93 ± 1.40 |
To comprehensively understand the synergistic effects between ferrocene and GPX4 inhibitors on these dual-function inducers, we then explored whether a simple combination of ferrocene compounds and GPX4 inhibitors could enhance ferroptosis induction and antitumor effects. As shown in Fig. S10,† the combination of ferrocene with ML162 only resulted in identical inhibition compared with ML162 alone on HT1080 cells; these antitumor effects are obviously weaker than those of the ferrocene-appended GPX4 inhibitor, 17. These results acknowledged that the synergies of ferrocene and GPX4 inhibitors exert stronger ferroptosis inducing activities. In addition, ferrocenyl groups have been recognised as important hydrophobic organometallic units to improve the penetration of the cell membrane in terms of biologically active compounds;56 the improvement of this effect is of interest to investigate for the compounds herein. The cellular uptake efficiency of ML162 and 17 were examined by the LC-MS/MS method (Fig. S11†), which demonstrates that both complexes accumulate rapidly within cells on a time scale of hours. The internalization of 17 is significantly fast compared with that of ML162 and leads to higher concentration inside of cells. As a consequence, the higher cytotoxicity of 17 may at least partly emerge from its high cellular accumulation due to the lipophilic ferrocenyl group.
Cell death and EMT process studies
To investigate the ferroptosis selectivity of ferrocene-appended GPX4 inhibitors, their modes of cell death were explored by employing the apoptosis inhibitor Z-VAD-FMK, the necrosis inhibitor necrostatin-1 (Nec-1), the autophagy inhibitor Wortmannin and the typical ferroptosis inhibitors fer-1 and deferoxamine (DFO). As shown in Fig. 3A, the percentage of cell viability of OS-RC2 upon combining the ferrocene-appended molecule 17 and the apoptosis inhibitor Z-VAD-FMK remained unchanged, indicating that 17 could not induce cell apoptosis at the current concentration. Moreover, the co-treatment of 17 with Nec-1 and Wortmannin, respectively, did not significantly thwart the cellular death of OS-RC2 induced by these two compounds. This demonstrated that the cellular death caused by 17 may not be attributable to necrosis and autophagy. In contrast, when combined with typical ferroptosis inhibitors fer-1 and DFO, the cytotoxicity of 17 was obviously reversed. Of particular note are the higher EC50 values of ferroptosis inhibitors to reverse the cellular death posed by 17 compared to that of ML162 (Table S10†); these observations confirmed that the potent antiproliferative activities of 17 were achieved mainly by inducing ferroptosis of cancer cells, and also underscored its remarkable ferroptosis selectivity.
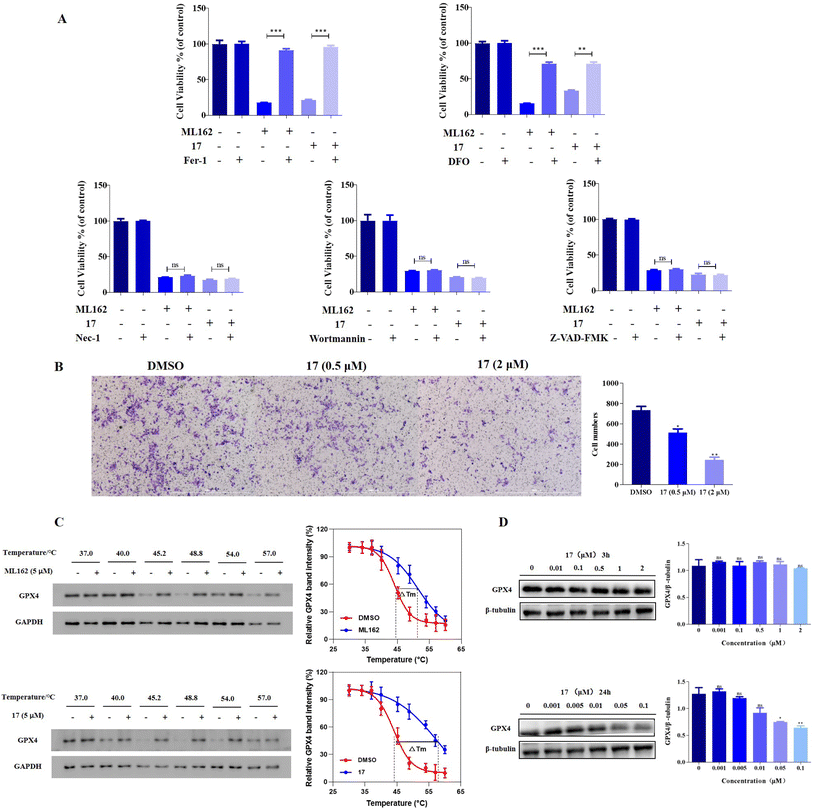 |
| Fig. 3 (A) The percentage of cell viability after treatment of ML162 or 17 (0.4 μM) with Z-VAD-FMK (10 μM), Nec-1 (10 μM), Wortmannin (30 μM), Fer-1 (2 μM), and DFO (30 μM); analysis results represented the mean ± SD. (B) Transwell assays were used to detect the invasion of cancer cells after treatment of 17 (0.5 and 2 μM). (C) CETSA experiments were performed on OS-RC-2 cells treated with DMSO, ML162 and 17 (5 μM) for 4 h. The protein levels were analyzed by western blotting after heating at different temperatures (30–60 °C), and the apparent Tagg values for GPX4 in OS-RC-2 cells in the absence and presence of ML162 and 17 were 44.09 °C to 51.42 °C, and 44.05 to 58.00 °C, respectively. (D) Western blot analysis of GPX4 levels in OS-RC-2 cells following treatment of 17 in 3 and 24 h. *P < 0.05, **P < 0.01, and ***P < 0.001 versus the control groups. | |
EMT is connected to carcinogenesis, invasiveness, metastasis, and therapeutic resistance in cancer. Increasing evidence shows that cancer cells undergoing EMT are vulnerable to ferroptotic cell death.11,12 Thus, the effect of the ferroptosis-inducing agent 17 on the migration and invasion of cancer cells was examined. Through a wound-healing assay, it was found that those compounds significantly prevented the migration of 4T1 cells time-dependently, especially in the case of 17 (Fig. S11†). Similarly, transwell assays revealed that this molecule disrupts the invasion of tumor cells dose-dependently (Fig. 3B). These results indicated the superior capacity of ferrocene-appended ferroptosis inducers to block the migratory and invasive properties of 4T1 cells, denoting their enormous promise for the treatment of refractory cancer metastasis, taking into account that TNBC 4T1 cells have high invasiveness, and approximately half of the TNBC cases will have distant metastasis.57
GPX4 CETSA and western blotting evaluations
Since certain ferrocene-appended molecules exhibited high selectivity for ferroptosis and remarkable GPX4 inhibition activities, the effect of 17 on an intact GPX protein was further determined, and the results are shown in Fig. 3C and D. The ability of 17 for binding GPX4 was first validated by the cellular thermal shift assay (CETSA) considering that the stability of the protein is changed when it is bound with the molecule. It was found that the aggregation temperature (Tagg) of GPX4 was improved by ∼14 °C in OS-RC2 cells after treatment of 17, whereas the Tagg in the case of ML162 increased by ∼7 °C, indicating the stronger interaction of 17 with GPX4 in the cells. The increased thermal stability of GPX4 is in line with that of ML210 and other alkyne analogues on the stabilization of GPX4 reported previously,54 suggesting that they may bind to GPX4 in a similar way. Meanwhile, the expression level of GPX4 was further investigated by western blot, and it is noteworthy that there was no obvious difference in the protein level of GPX4 compared to that of the control group within 3 h of 17 treatment (Fig. 3D). However, the down-regulation of GPX4 was evident after 24 h treatment. One could speculate that the potent ferroptosis-inducing ability of ferrocene-based ML162 analogues may result from both the inhibition and downgrading of GPX4 during long-term cellular incubation. These results accentuate the complex effects of covalent inhibitors on GPX4.
LPO related bioactivity evaluations
It is well known that certain ferrocene complexes can induce the formation of ROS via a Fenton type reaction. Therefore, the ability of ferrocene-appended GPX4 inhibitor 17 to yield ROS was first evaluated under physiological conditions by the widely used fluorescent ROS indicator, 2′,7′-dichlorofluorescein diacetate (DCFH-DA). As shown in Fig. 4A, a known H2O2-induced activation method was carried out to monitor the ROS generation by using selected molecules in buffer,47 containing FeSO4 as a positive control. It is evident that 17 can facilitate the conversion of H2O2 to OH˙ and increase the production of ROS in a time-dependent manner, reflected by the OH˙-mediated oxidation of nonfluorescent 2′,7′-dichlorofluorescein (DCFH) to fluorescent 2′,7′-dichlorofluorescein (DCF). In contrast, the pure organic compound ML162 did not exhibit obvious catalytic efficiency for yielding ROS. Unrestricted lipid peroxidation is a hallmark of ferroptosis, which can be evaluated in cells by multiple approaches. As a metabolite of LPOs, the level of MDA is commonly used as a characteristic index for the determination of LPOs. As illustrated in Fig. 4B, 17 improved the contents of MDA in cells dose-dependently and more prominently than ML162 at the same concentration, which corresponds to the phenomenon observed at the molecular level.
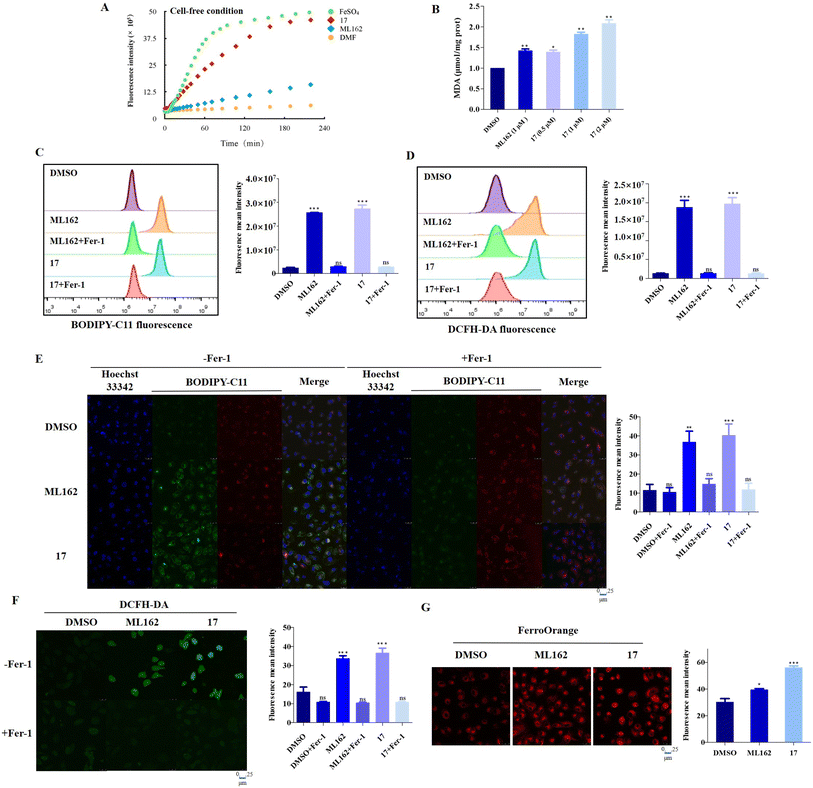 |
| Fig. 4 (A) Monitoring of the generation of ROS of corresponding compounds (100 μM) using fluorescence spectroscopy in combination with ROS-sensitive DCFH-DA (λex = 501 nm and λem = 531 nm). (B) OS-RC2 cells were treated with ML162 (1 μM) and 17 (0.5, 1, and 2 μM) for 4 h, and then the content of MDA was determined. (C) Flow cytometry analysis for intracellular LPO by C11-BODIPY of OS-RC-2 cells treated with DMSO, ML162 and 17 (0.5 μM) with and without fer-1 (1.5 μM) respectively. (D) Flow cytometry analysis for intracellular ROS by DCFH-DA of OS-RC-2 cells treated with DMSO, ML162 and 17 (0.5 μM) with and without fer-1 (1.5 μM) respectively. (E) Representative confocal images and analysis of C11-BODIPY staining of OS-RC-2 cells after incubation with ML162 and 17 (0.5 μM) with and without fer-1 (1.5 μM) respectively. (F) Representative confocal images and analysis of DCFH-DA staining of OS-RC-2 cells after incubation with ML162 and 17 (0.5 μM) with and without fer-1 (1.5 μM) respectively. (G) Intracellular Fe2+ was visualized with the FerroOrange fluorescent probe. OS-RC-2 cells were treated with ML162 and 17 (0.5 μM) for 4 h, and the content of intracellular Fe2+ was determined. *P < 0.05, **P < 0.01, and ***P < 0.001 versus the control groups. | |
Accordingly, the fluorescent probes C11-BODIPY and DCFH-DA were employed to investigate the intracellular ROS and LPOs via flow cytometry and confocal laser scanning microscopy (CLSM). As illustrated in Fig. 4C and D, 17 could significantly induce the accumulation of LPOs slightly more than ML162 and this trend could be reversed by fer-1 totally according to the flow cytometry analysis, which emphasized its remarkable ferroptosis selectivity. In agreement with this, the oxidative fluorescence intensity (green) of C11-BODIPY and DCFH-DA in OS-RC2 cells significantly increased upon treatment with 17 (Fig. 4E and F). Considering the iron dependence which can make cancer cells more vulnerable to ferroptosis, the overall level of cellular ferrous iron was detected by Fe2+-sensitive FerroOrange staining. As shown in Fig. 4G, the images of FerroOrange staining intuitively illustrated that a notable fluorescence intensity of the cells after treatment with 17 is found to be significantly higher than that of cells incubated with ML162, suggesting that 17 can increase the level of cellular ferrous iron. Overall, these results verified by the increasing LPOs and Fe2+, lead to a consistent conclusion that ferrocene-appended GPX4 inhibitors could significantly induce the accumulation of LPOs and subsequently engender severe ferroptosis of cancer cells.
Molecular docking
The molecular simulation of small molecular ligands with GPX4 has been challenging due to the flat surface surrounding the active site of GPX4,58 and thus a template-based docking by Watvina was carried out. According to the atomic types and their contribution to ligand–receptor interaction, we first generate a template-containing mapping of heavy atoms, aromatic atoms and hydrogen bond receptors (Fig. S12†). Using the genetic algorithm for global searching and BFGS for local refinement in Watvina, the conformation with the highest template matching score of docked ferrocene derivatives was used for further analysis. As shown in Fig. 5, the selected ligands 12, 13, 15 and 17 docked well to the ML162 space with a paired atom RMSD of less than 0.5 Å. These four organometallics enabled the formation of two key hydrogen bonds with residues of GPX4 like that of ML162. One of the cyclopentadienyl rings of 15 or 17 occupied the same hydrophobic space as that of ML162, and the resulting conformation of 17 revealed that it filled the active pocket better than compound 15. In the case of 12 and 13, the ferrocene groups are placed in lieu of the benzene (ring C) part in ML162 (Fig. 2B, 5C and D). Since ring C occupied by ferrocene is flexible and distal from the binding pocket, 12 and 13 may exhibit weak binding activity with GPX4. As for compounds 10 and 11 with the ferrocenyl group sited at the very cramped ring B part (Fig. 2B), they were expected to lose the capacity of conjugating GPX4. Indeed, these docking data, which held the binding mode of covalent inhibitors with GPX4, concur well with their GPX4 inhibitory activities and thus provided a reasonable interpretation.
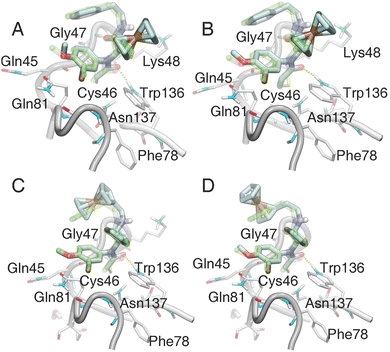 |
| Fig. 5 The binding models of ferrocenyl ligands in the GPX4 crystal structure (PDB code 6HKQ). Compounds 15 (A), 17 (B), 12 (C), and 13 (D) were docked to GPX4 by Watvina with the template-based method; then all the structures were minimized with the xtb program. The original ligand ML162 was shown in green transparent stick mode, and ferrocenyl ligands in cyan stick mode. The hydrogen bonds were represented with a yellow dashed line by VMD software. | |
In vivo profiling of ferrocene-based ferroptosis inducers
Considering the excellent antitumor activities of 17 on two ferroptosis sensitive cell lines, the OS-RC2 xenograft model was established in BALB/C nude mice to assess its in vivo efficacy. The tested compounds were administered by intraperitoneal injection every three days for approximately 4 weeks (Fig. 6). The tumor growth inhibition (TGI) values of 17-treated groups were 44%@10 mg kg−1 and 56%@20 mg kg−1, which showed that 17 could suppress the progression of the renal tumor with a significant dose-dependent effect. It is noteworthy that 17 displayed prominent antitumor activities in vivo compared to ML162 in the same case of delivering a high dose of drugs. Additionally, there was no obvious difference in body weight of the 17 treatment groups compared with the control group. Furthermore, hematoxylin and eosin (H&E) staining analysis of the major organs was carried out to investigate the safety profile of ML162 and 17, which indicated that there was no apparent toxic damage observed in major organs such as the heart, liver, spleen, lung, and kidney. Subsequent immunohistochemical analysis and western blot studies showed that 17 evidently reduced the level of the GPX4 protein in tumor tissues, revealing that ferrocenyl complex 17 effectively induced ferroptosis in vivo. These results emphasized the superior potential of ferrocene-based ferroptosis inducers as anticancer drug candidates for further research.
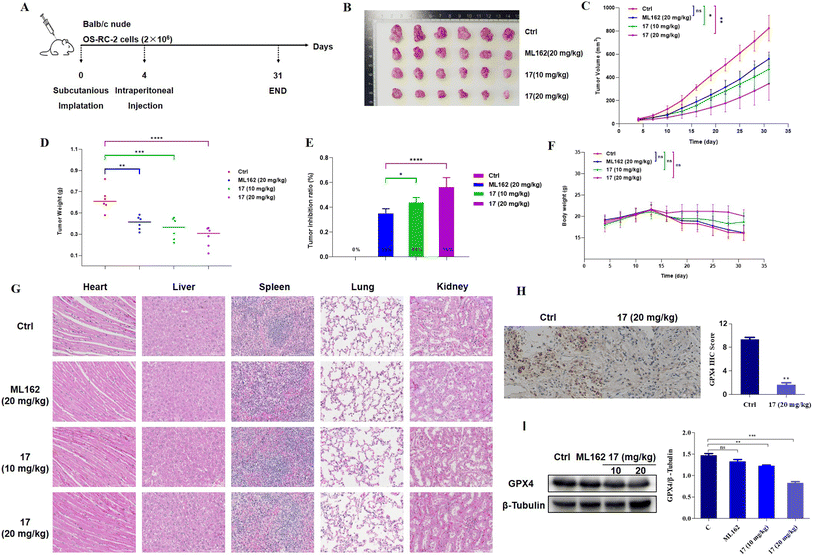 |
| Fig. 6 (A) In vivo antitumor activity of ML162 and 17 in a human OS-RC2 renal carcinoma model (BALB/c nude mice, given medicine every three days, n = 6). (B) Picture of excised tumors from the different groups after the treatment; (C) tumor volume curves during the treatment period; (D) tumor weights after treatment; (E) tumor inhibition ratio after the treatment period. (F) Body weight curves of different groups after the treatment; (G) H&E staining of the major organs of mice after treatment with compounds. (H) Anti-GPX4 immunohistochemistry images and analysis from the OS-RC-2 xenograft model. (I) Western blot analysis of GPX4 levels in tumor tissue following treatment of the corresponding compounds. *P < 0.05, **P < 0.01, ***P < 0.001 and ****P < 0.0001 versus the control groups. | |
RNA-seq analysis
To further illustrate the impact of 17 on the gene expression pattern, we employed the NovaSeq 6000 PE150 platform (Illumina Inc., CA, USA) to analyze gene expression differences (DEGs) between the extracts of tumor tissues from OS-RC-2 xenograft model rats treated with 17 (20 mg kg−1) and normal control rats. A volcano plot illustrated significant differences in mRNA expression patterns between the two groups (Fig. 7A and Table S13†). Utilizing the Limma package, we identified differential genes under the condition p-value < 0.05 & |log
FC| > 1, resulting in 790 differentially expressed genes (324 up-regulated and 466 down-regulated).
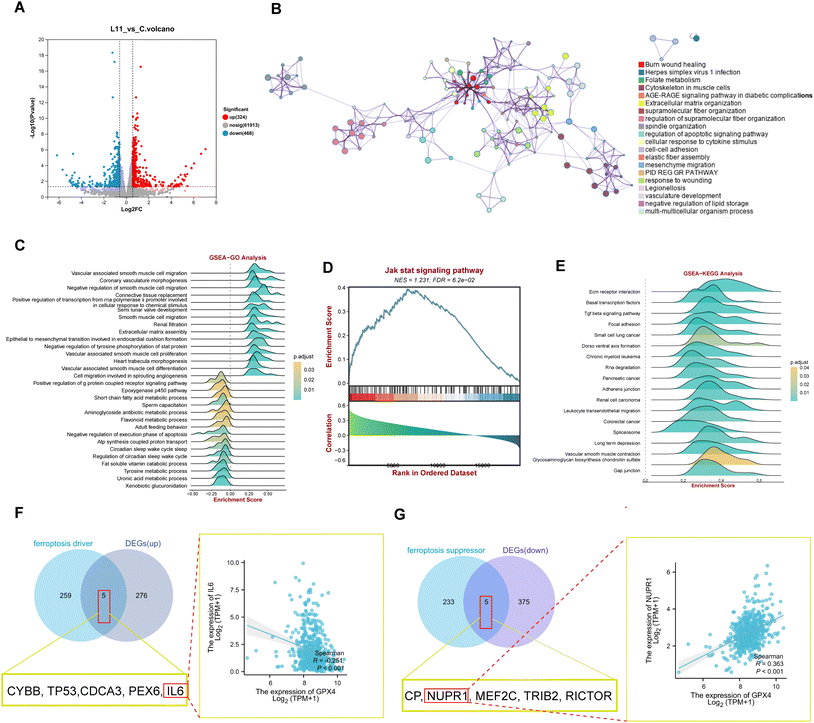 |
| Fig. 7 RNA-seq analysis of samples from OS-RC-2 xenograft model rats treated with 17 (20 mg kg−1) and the control. (A) Volcano plot of all DEGs; red indicates upregulation, whereas blue indicates downregulation. (B) Protein–protein interaction (PPI) networks constructed by Metascape and the protein function were enriched. (C) GO term enrichment analysis of DEGs from the 17 (20 mg kg−1) treatment group. (D) GSEA analysis of DEGs in the JAK-STAT signaling pathway. (E) KEGG enrichment analysis of DEGs from the 17 (20 mg kg−1) treatment group. (F and G) The genes as the driver and suppressor of ferroptosis overlap with DEGs that are up-regulated and down-regulated by using a Venn diagram. | |
Subsequently, we constructed a PPI network of the 790 DEGs using Metascape. This analysis revealed enrichment in ferroptosis-related signaling pathways and cell phenotypes, including folate metabolism, the AGE-RAGE signaling pathway in diabetic composites, negative regulation of lipid storage, and mesenchymal migration (Fig. 7B). Further insight into the biological functions and signaling pathways associated with ferroptosis was gained through GO and KEGG analyses (Fig. 7C–E). These analyses demonstrated the involvement of DEGs in multiple biological processes related to iron homeostasis, such as cyclooxygenase and G protein-coupled receptor signaling pathways. Notably, the activation of multiple inflammation-related signaling pathways leading to ferroptosis was observed, with DEGs enriched in the inflammatory signaling pathway Jak-stat, as indicated by GSVA analysis (Fig. 7D). KEGG analysis also highlighted involvement in pathways related to iron and lipid metabolism, including short-chain fatty acid metabolism and tyrosine metabolism (Fig. 7E). To explore the correlation of 17 with ferroptosis-related pathways, we examined the overlapping genes between the ferroptosis driver and DEGs, or ferroptosis suppressor genes and DEGs after 17 treatment. The results revealed that 17 upregulated ferroptosis driver genes (CYBB, TP53, CDCA3, PEX6, and IL6) and downregulated suppressor genes (CP, NUPR1, MEF2C, TRIB2, RICTOR, including GPX4). The correlation analysis showed a significant negative correlation between the driving gene IL6 and GPX4 (Fig. 7F) and a significant positive correlation with UPR1 and GPX4 (Fig. 7G), indicating the targeted inhibition of GPX4 function by 17 to induce ferroptosis in renal cell carcinoma in vivo.
Conclusions
According to the ferroptosis-based phenotypic screening of our in-house organometallic compound library, the usual ferrocene complexes did not possess visible ferroptosis-inducing activities, which contradicted the conventional understanding of ferrocene for ferroptosis. As a result, a series of ferrocene-appended GPX4 inhibitors has been rationally designed by a one-stone-two-birds strategy for the first time. Distinct from the known bioferrocene species, only using the ferrocenyl group as an auxiliary fragment for existing organic moieties, or merely taking advantage of the redox behaviors of ferrous iron to generate ROS, complex 17 can both inhibit GPX4 effectively and catalyze the Fenton-like reaction to yield ROS, and consequently result in stronger antiproliferative activity and higher ferroptosis selectivity than the corresponding organic compound ML162 (Fig. 8). Therefore, as a proof-of-concept study, we successfully achieved the goal to use a single molecule to modulate the two main pathways of ferroptosis, iron homeostasis and the antioxidant defense system. In particular, cellular CETSA experiments confirmed the strong interaction of 17 with an intact GPX4 protein, and this result was in accordance with its capability to disrupt the function of GPX4 in OS-RC2 cells. Moreover, the significant ROS-inducing ability of 17 at both the molecular level and at the cellular level acknowledged the ferroptosis enhancement of the ferrocene-appended GPX4 inhibitor. Subsequent in vivo experiments demonstrated that 17 is a potent attenuator of the growth of renal tumors, and ferroptosis is pronounced to be involved in this inhibition process, supported by the GPX4 content analysis and RNA-seq study of tumor tissues. In conclusion, we present here a comprehensive study of rationally designed ferrocene-appended ferroptosis inducers, not only to promote the development of ferroptosis-based anticancer treatments, but also to provide new insight into the design of novel bioorganometallics based on ferrocene for potential disease therapies.
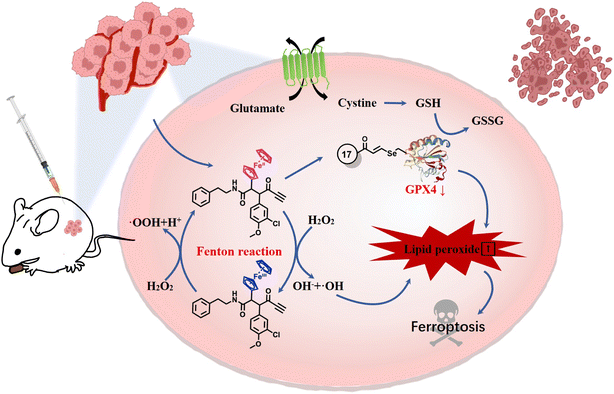 |
| Fig. 8 Proposed mechanisms of ferrocene-appended ferroptosis inducers. | |
Data availability
The data that support the findings of this study are available in the ESI† or on request from the corresponding author.
Author contributions
W. L., J. Y., J. W. and X. F. contributed equally to this manuscript. Y. W. and J. L. designed the study. W. L., J. W., H. W. and Q. Z. performed the synthesis and characterization of the chemical compounds. J. Y., X. F., Y. X., X. Z. and X. Qi performed the in vitro and in vivo anticancer evaluation of the compounds. X. X. performed the docking calculation work. X. L. and X. Qiu contributed to the target protein binding affinity evaluation. P. P., Q. G., G. J. and S. Y. contributed to the construction of the ferrocene-based compound library. J. B.-C. and M. J. M. prepared the crystal figure. Y. W., J. L. and X. Qiu supervised the study. Y. W., J. L. and M. J. M. wrote the manuscript. All authors participated in valuable discussion and manuscript correction.
Conflicts of interest
Y. W., J. L., J. W., W. L., H. W. and X. Z. are inventors on a patent application related to this work.
Acknowledgements
Y. W. received funding from Qingdao Marine Science and Technology (2022QNLM030003-2), Taishan Scholar Youth Expert Program in Shandong Province (tsqn202103035) and the Distinguished Young Scholars of Shandong Provincial Natural Science Foundation (Overseas, 2022HWYQ-069), andX. Q. is thankful for the support of the Fundamental Research Funds for the Central Universities of Ocean University of China (No. 202262015).
References
- S. J. Dixon, K. M. Lemberg, M. R. Lamprecht, R. Skouta, E. M. Zaitsev, C. E. Gleason, D. N. Patel, A. J. Bauer, A. M. Cantley, W. S. Yang, B. Morrison III and B. R. Stockwell, Ferroptosis: An Iron-Dependent Form of Nonapoptotic Cell Death, Cell, 2012, 149(5), 1060–1072 CrossRef CAS PubMed.
- K. Hadian and B. R. Stockwell, SnapShot: Ferroptosis, Cell, 2020, 181(5), 1188 CrossRef CAS PubMed.
- B. R. Stockwell, Ferroptosis turns 10: Emerging mechanisms, physiological functions, and therapeutic applications, Cell, 2022, 185(14), 2401–2421 CrossRef CAS PubMed.
- X. Fang, H. Wang, D. Han, E. Xie, X. Yang, J. Wei, S. Gu, F. Gao, N. Zhu, X. Yin, Q. Cheng, P. Zhang, W. Dai, J. Chen, F. Yang, H.-T. Yang, A. Linkermann, W. Gu, J. Min and F. Wang, Ferroptosis as a target for protection against cardiomyopathy, Proc. Natl. Acad. Sci. U. S. A., 2019, 116(7), 2672–2680 CrossRef CAS PubMed.
- W.-Y. Sun, V. A. Tyurin, K. Mikulska-Ruminska, I. H. Shrivastava, T. S. Anthonymuthu, Y.-J. Zhai, M.-H. Pan, H.-B. Gong, D.-H. Lu, J. Sun, W.-J. Duan, S. Korolev, A. Y. Abramov, P. R. Angelova, I. Miller, O. Beharier, G.-W. Mao, H. H. Dar, A. A. Kapralov, A. A. Amoscato, T. G. Hastings, T. J. Greenamyre, C. T. Chu, Y. Sadovsky, I. Bahar, H. Bayır, Y. Y. Tyurina, R.-R. He and V. E. Kagan, Phospholipase iPLA2β averts ferroptosis by eliminating a redox lipid death signal, Nat. Chem. Biol., 2021, 17(4), 465–476 CrossRef CAS PubMed.
- R. Skouta, S. J. Dixon, J. Wang, D. E. Dunn, M. Orman, K. Shimada, P. A. Rosenberg, D. C. Lo, J. M. Weinberg, A. Linkermann and B. R. Stockwell, Ferrostatins Inhibit Oxidative Lipid Damage and Cell Death in Diverse Disease Models, J. Am. Chem. Soc., 2014, 136(12), 4551–4556 CrossRef CAS PubMed.
- J. P. Friedmann Angeli, M. Schneider, B. Proneth, Y. Y. Tyurina, V. A. Tyurin, V. J. Hammond, N. Herbach, M. Aichler, A. Walch, E. Eggenhofer, D. Basavarajappa, O. Rådmark, S. Kobayashi, T. Seibt, H. Beck, F. Neff, I. Esposito, R. Wanke, H. Förster, O. Yefremova, M. Heinrichmeyer, G. W. Bornkamm, E. K. Geissler, S. B. Thomas, B. R. Stockwell, V. B. O'Donnell, V. E. Kagan, J. A. Schick and M. Conrad, Inactivation of the ferroptosis regulator Gpx4 triggers acute renal failure in mice, Nat. Cell Biol., 2014, 16(12), 1180–1191 CrossRef CAS PubMed.
- J. Li, J. Liu, Z. Zhou, R. Wu, X. Chen, C. Yu, B. Stockwell, G. Kroemer, R. Kang and D. Tang, Tumor-specific GPX4 degradation enhances ferroptosis-initiated antitumor immune response in mouse models of pancreatic cancer, Sci. Transl. Med., 2023, 15(720), eadg3049 CrossRef CAS PubMed.
- X. Jiang, B. R. Stockwell and M. Conrad, Ferroptosis: mechanisms, biology and role in disease, Nat. Rev. Mol. Cell Biol., 2021, 22(4), 266–282 CrossRef PubMed.
- C. Mao, X. Liu, Y. Zhang, G. Lei, Y. Yan, H. Lee, P. Koppula, S. Wu, L. Zhuang, B. Fang, M. V. Poyurovsky, K. Olszewski and B. Gan, DHODH-mediated ferroptosis defence is a targetable vulnerability in cancer, Nature, 2021, 593(7860), 586–590 CrossRef CAS PubMed.
- M. J. Hangauer, V. S. Viswanathan, M. J. Ryan, D. Bole, J. K. Eaton, A. Matov, J. Galeas, H. D. Dhruv, M. E. Berens, S. L. Schreiber, F. McCormick and M. T. McManus, Drug-tolerant persister cancer cells are vulnerable to GPX4 inhibition, Nature, 2017, 551(7679), 247–250 CrossRef CAS PubMed.
- V. S. Viswanathan, M. J. Ryan, H. D. Dhruv, S. Gill, O. M. Eichhoff, B. Seashore-Ludlow, S. D. Kaffenberger, J. K. Eaton, K. Shimada, A. J. Aguirre, S. R. Viswanathan, S. Chattopadhyay, P. Tamayo, W. S. Yang, M. G. Rees, S. Chen, Z. V. Boskovic, S. Javaid, C. Huang, X. Wu, Y. Y. Tseng, E. M. Roider, D. Gao, J. M. Cleary, B. M. Wolpin, J. P. Mesirov, D. A. Haber, J. A. Engelman, J. S. Boehm, J. D. Kotz, C. S. Hon, Y. Chen, W. C. Hahn, M. P. Levesque, J. G. Doench, M. E. Berens, A. F. Shamji, P. A. Clemons, B. R. Stockwell and S. L. Schreiber, Dependency of a therapy-resistant state of cancer cells on a lipid peroxidase pathway, Nature, 2017, 547(7664), 453–457 CrossRef CAS PubMed.
- I. Ingold, C. Berndt, S. Schmitt, S. Doll, G. Poschmann, A. Roveri, X. Peng, F. P. Freitas, M. Aichler, M. Jastroch, F. Ursini, E. S. J. Arnér, N. Fradejas-Villar, U. Schweizer, H. Zischka, J. P. F. Angeli and M. Conrad, Selenium utilization by GPX4 was an evolutionary requirement to prevent hydroperoxide-induced ferroptosis, Free Radical Biol. Med., 2017, 112, 24 CrossRef.
- B. Hassannia, P. Vandenabeele and T. Vanden Berghe, Targeting Ferroptosis to Iron Out Cancer, Cancer Cell, 2019, 35(6), 830–849 CrossRef CAS PubMed.
- X. Chen, C. Yu, R. Kang and D. Tang, Iron Metabolism in Ferroptosis, Front. Cell Dev. Biol., 2020, 8, 590226 CrossRef PubMed.
- M. Patra and G. Gasser, The medicinal chemistry of ferrocene and its derivatives, Nat. Rev. Chem., 2017, 1(9), 0066 CrossRef CAS.
- G. Jaouen, A. Vessieres and S. Top, Ferrocifen type anti cancer drugs, Chem. Soc. Rev., 2015, 44(24), 8802–8817 RSC.
- B. Sharma and V. Kumar, Has Ferrocene Really Delivered Its Role in Accentuating the Bioactivity of Organic Scaffolds?, J. Med. Chem., 2021, 64(23), 16865–16921 CrossRef CAS PubMed.
- R. Wang, H. Chen, W. Yan, M. Zheng, T. Zhang and Y. Zhang, Ferrocene-containing hybrids as potential anticancer agents: Current developments, mechanisms of action and structure-activity relationships, Eur. J. Med. Chem., 2020, 190, 112109 CrossRef CAS PubMed.
- A. Vessières, Y. Wang, M. J. McGlinchey and G. Jaouen, Multifaceted chemical behaviour of metallocene (M = Fe, Os) quinone methides. Their contribution to biology, Coord. Chem. Rev., 2021, 430, 213658 CrossRef.
- W.-J. Wang, Y.-Y. Ling, Y.-M. Zhong, Z.-Y. Li, C.-P. Tan and Z.-W. Mao, Ferroptosis-Enhanced Cancer Immunity by a Ferrocene-Appended Iridium(III) Diphosphine Complex, Angew. Chem., Int. Ed., 2022, 61(16), e202115247 CrossRef CAS PubMed.
- J. Li, Q. Zong, Y. Liu, X. Xiao, J. Zhou, Z. Zhao and Y. Yuan, Self-catalyzed tumor ferroptosis based on ferrocene conjugated reactive oxygen species generation and a responsive polymer, Chem. Commun., 2022, 58(20), 3294–3297 RSC.
- X. Zhang, Y. Ding, Z. Zhang, Y. Ma, X. Sun, L. Wang, Z. Yang and Z.-W. Hu, In Situ Construction of Ferrocene-Containing Membrane-Bound Nanofibers for the Redox Control of Cancer Cell Death and Cancer Therapy, Nano Lett., 2023, 23(16), 7665–7674 CrossRef CAS PubMed.
- J. Sagasser, B. N. Ma, D. Baecker, S. Salcher, M. Hermann, J. Lamprecht, S. Angerer, P. Obexer, B. Kircher and R. Gust, A New Approach in Cancer Treatment: Discovery of Chlorido[N,N′-disalicylidene-1,2-phenylenediamine]iron(III) Complexes as Ferroptosis Inducers, J. Med. Chem., 2019, 62(17), 8053–8061 CrossRef CAS PubMed.
- M. Lv, Y. Zheng, J. Wu, Z. Shen, B. Guo, G. Hu, Y. Huang, J. Zhao, Y. Qian, Z. Su, C. Wu, X. Xue, H. K. Liu and Z. W. Mao, Evoking Ferroptosis by Synergistic Enhancement of a Cyclopentadienyl Iridium-Betulin Immune Agonist, Angew. Chem., Int. Ed., 2023, 62(48), e202312897 CrossRef CAS PubMed.
- B. Rosenberg and L. Vancamp, Successful Regression of Large Solid Sarcoma 180-Tumors by Platinum Compounds, Cancer Res., 1970, 30(6), 1799–1802 CAS.
- J. J. Wilson and S. J. Lippard, Synthetic Methods for the Preparation of Platinum Anticancer Complexes, Chem. Rev., 2014, 114(8), 4470–4495 CrossRef CAS PubMed.
- T. Yang, S. Zhang, H. Yuan, Y. Wang, L. Cai, H. Chen, X. Wang, D. Song, X. Wang, Z. Guo and X. Wang, Platinum-Based TREM2 Inhibitor Suppresses Tumors by Remodeling the Immunosuppressive Microenvironment, Angew. Chem., Int. Ed., 2023, 62(2), e202213337 CrossRef CAS PubMed.
- X. Wang, X. Wang, S. Jin, N. Muhammad and Z. Guo, Stimuli-Responsive Therapeutic Metallodrugs, Chem. Rev., 2019, 119(2), 1138–1192 CrossRef CAS PubMed.
- X. Zhao, J. Zhang, W. Zhang, Z. Guo, W. Wei, X. Wang and J. Zhao, A chiral fluorescent Ir(iii) complex that targets the GPX4 and ErbB pathways to induce cellular ferroptosis, Chem. Sci., 2023, 14(5), 1114–1122 RSC.
- R. Lengacher, Y. Wang, H. Braband, O. Blacque, G. Gasser and R. Alberto, Organometallic small molecule kinase inhibitors – direct incorporation of Re and 99mTc into Opaganib®, Chem. Commun., 2021, 57(98), 13349–13352 RSC.
- S. M. Meier-Menches, C. Gerner, W. Berger, C. G. Hartinger and B. K. Keppler, Structure–activity relationships for ruthenium and osmium anticancer agents – towards clinical development, Chem. Soc. Rev., 2018, 47(3), 909–928 RSC.
- X. Wang, M. d. G. Jaraquemada-Peláez, C. Rodríguez-Rodríguez, Y. Cao, C. Buchwalder, N. Choudhary, U. Jermilova, C. F. Ramogida, K. Saatchi, U. O. Häfeli, B. O. Patrick and C. Orvig, H4octox: Versatile Bimodal Octadentate Acyclic Chelating Ligand for Medicinal Inorganic Chemistry, J. Am. Chem. Soc., 2018, 140(45), 15487–15500 CrossRef CAS PubMed.
- H. Huang, S. Banerjee, K. Qiu, P. Zhang, O. Blacque, T. Malcomson, M. J. Paterson, G. J. Clarkson, M. Staniforth, V. G. Stavros, G. Gasser, H. Chao and P. J. Sadler, Targeted photoredox catalysis in cancer cells, Nat. Chem., 2019, 11(11), 1041–1048 CrossRef CAS PubMed.
- J.-J. Zhang, M. A. Abu el Maaty, H. Hoffmeister, C. Schmidt, J. K. Muenzner, R. Schobert, S. Wölfl and I. Ott, A Multitarget Gold(I) Complex Induces Cytotoxicity Related to Aneuploidy in HCT-116 Colorectal Carcinoma Cells, Angew. Chem., Int. Ed., 2020, 59(38), 16795–16800 CrossRef CAS PubMed.
- W. D. J. Tremlett, D. M. Goodman, T. R. Steel, S. Kumar, A. Wieczorek-Błauż, F. P. Walsh, M. P. Sullivan, M. Hanif and C. G. Hartinger, Design concepts of half-sandwich organoruthenium anticancer agents based on bidentate bioactive ligands, Coord. Chem. Rev., 2021, 445, 213950 CrossRef CAS.
- G. Moreno-Alcántar and A. Casini, Bioinorganic supramolecular coordination complexes and their biomedical applications, FEBS Lett., 2023, 597(1), 191–202 CrossRef PubMed.
- D. R. van Staveren and N. Metzler-Nolte, Bioorganometallic Chemistry of Ferrocene, Chem. Rev., 2004, 104(12), 5931–5986 CrossRef CAS PubMed.
- K. Kowalski, Organometallic nucleosides—Synthesis, transformations, and applications, Coord. Chem. Rev., 2021, 432, 213705 CrossRef CAS.
- H.-G. Xu, M. Schikora, M. Sisa, S. Daum, I. Klemt, C. Janko, C. Alexiou, G. Bila, R. Bilyy, W. Gong, M. Schmitt, L. Sellner and A. Mokhir, An Endoplasmic Reticulum Specific Pro-amplifier of Reactive Oxygen Species in Cancer Cells, Angew. Chem., Int. Ed., 2021, 60(20), 11158–11162 CrossRef CAS PubMed.
- J. Yan, K. Yue, X. Fan, X. Xu, J. Wang, M. Qin, Q. Zhang, X. Hou, X. Li and Y. Wang, Synthesis and bioactivity evaluation of ferrocene-based hydroxamic acids as selective histone deacetylase 6 inhibitors, Eur. J. Med. Chem., 2023, 246, 115004 CrossRef CAS PubMed.
- T. Stringer, L. Wiesner and G. S. Smith, Ferroquine-derived polyamines that target resistant Plasmodium falciparum, Eur. J. Med. Chem., 2019, 179, 78–83 CrossRef CAS PubMed.
- Y. Wang, P. Pigeon, W. Li, J. Yan, P. M. Dansette, M. Othman, M. J. McGlinchey and G. Jaouen, Diversity-oriented synthesis and bioactivity evaluation of N-substituted ferrocifen compounds as novel antiproliferative agents against TNBC cancer cells, Eur. J. Med. Chem., 2022, 234, 114202 CrossRef CAS PubMed.
- Y. Wang, P. Pigeon, S. Top, J. Sanz García, C. Troufflard, I. Ciofini, M. J. McGlinchey and G. Jaouen, Atypical Lone Pair–π Interaction with Quinone Methides in a Series of Imido-Ferrociphenol Anticancer Drug Candidates, Angew. Chem., Int. Ed., 2019, 58(25), 8421–8425 CrossRef CAS PubMed.
- Y. Wang, P. Pigeon, M. J. McGlinchey, S. Top and G. Jaouen, Synthesis and antiproliferative evaluation of novel hydroxypropyl-ferrociphenol derivatives, resulting from the modification of hydroxyl groups, J. Organomet. Chem., 2017, 829, 108–115 CrossRef CAS.
- H. Wang, X. Fan, P.-P. Xie, S. Yang, P. Pigeon, Y. Xiong, S. Gai, X. Qi, J. Wang, Q. Zhang, W. Li, H. Qian, M. J. McGlinchey, G. Jaouen, C. Zheng and Y. Wang, Deciphering the Diversified Metabolic Behavior of Hydroxyalkyl Ferrocidiphenols as Anticancer Complexes, J. Med. Chem., 2024, 67(2), 1209–1224 CrossRef CAS PubMed.
- H. Hagen, P. Marzenell, E. Jentzsch, F. Wenz, M. R. Veldwijk and A. Mokhir, Aminoferrocene-Based Prodrugs Activated by Reactive Oxygen Species, J. Med. Chem., 2012, 55(2), 924–934 CrossRef CAS PubMed.
- D.-W. Gao, Q. Gu and S.-L. You, An Enantioselective Oxidative C–H/C–H Cross-Coupling Reaction: Highly Efficient Method To Prepare Planar Chiral Ferrocenes, J. Am. Chem. Soc., 2016, 138(8), 2544–2547 CrossRef CAS PubMed.
- Z.-J. Cai, C.-X. Liu, Q. Gu, C. Zheng and S.-L. You, PdII-Catalyzed Regio- and Enantioselective Oxidative C−H/C−H Cross-Coupling Reaction between Ferrocenes and Azoles, Angew. Chem., Int. Ed., 2019, 58(7), 2149–2153 CrossRef CAS PubMed.
- C.-X. Liu, W.-W. Zhang, P. Yang, F. Zhao, Z. Feng, Q. Wang, S.-Z. Zhang, Q. Gu and S.-L. You, Pd-catalyzed asymmetric oxidative C-H/C-H cross-coupling reaction between dialkylaminomethylferrocenes and indolizines, Chem Catal., 2022, 2(1), 102–113 CrossRef CAS.
- P. Pigeon, Y. Wang, S. Top, F. Najlaoui, M. C. Garcia Alvarez, J. Bignon, M. J. McGlinchey and G. Jaouen, A New Series of Succinimido-ferrociphenols and Related Heterocyclic Species Induce Strong Antiproliferative Effects, Especially against Ovarian Cancer Cells Resistant to Cisplatin, J. Med. Chem., 2017, 60(20), 8358–8368 CrossRef CAS PubMed.
- H. Liu, F. Forouhar, A. J. Lin, Q. Wang, V. Polychronidou, R. K. Soni, X. Xia and B. R. Stockwell, Small-molecule allosteric inhibitors of GPX4, Cell Chem. Biol., 2022, 29(12), 1680–1693 CrossRef CAS PubMed.
- C. Xu, Z. Xiao, J. Wang, H. Lai, T. Zhang, Z. Guan, M. Xia, M. Chen, L. Ren, Y. He, Y. Gao and C. Zhao, Discovery of a Potent Glutathione Peroxidase 4 Inhibitor as a Selective Ferroptosis Inducer, J. Med. Chem., 2021, 64(18), 13312–13326 CrossRef CAS PubMed.
- J. K. Eaton, L. Furst, R. A. Ruberto, D. Moosmayer, A. Hilpmann, M. J. Ryan, K. Zimmermann, L. L. Cai, M. Niehues, V. Badock, A. Kramm, S. Chen, R. C. Hillig, P. A. Clemons, S. Gradl, C. Montagnon, K. E. Lazarski, S. Christian, B. Bajrami, R. Neuhaus, A. L. Eheim, V. S. Viswanathan and S. L. Schreiber, Selective covalent targeting of GPX4 using masked nitrile-oxide electrophiles, Nat. Chem. Biol., 2020, 16(5), 497–506 CrossRef CAS PubMed.
- D. Moosmayer, A. Hilpmann, J. Hoffmann, L. Schnirch, K. Zimmermann, V. Badock, L. Furst, J. K. Eaton, V. S. Viswanathan, S. L. Schreiber, S. Gradl and R. C. Hillig, Crystal structures of the selenoprotein glutathione peroxidase 4 in its apo form and in complex with the covalently bound inhibitor ML162, Acta Crystallogr., Sect. D: Struct. Biol., 2021, 77(Pt 2), 237–248 CrossRef CAS PubMed.
- Y. Wang, F. Heinemann, S. Top, A. Dazzi, C. Policar, L. Henry, F. Lambert, G. Jaouen, M. Salmain and A. Vessieres, Ferrocifens labelled with an infrared rhenium tricarbonyl tag: synthesis, antiproliferative activity, quantification and nano IR mapping in cancer cells, Dalton Trans., 2018, 47(29), 9824–9833 RSC.
- L. Yin, J.-J. Duan, X.-W. Bian and S.-C. Yu, Triple-negative breast cancer molecular subtyping and treatment progress, Breast Cancer Res., 2020, 22(1), 61 CrossRef PubMed.
- A. Borchert, J. Kalms, S. R. Roth, M. Rademacher, A. Schmidt, H. G. Holzhutter, H. Kuhn and P. Scheerer, Crystal structure and functional characterization of selenocysteine-containing glutathione peroxidase 4 suggests an alternative mechanism of peroxide reduction, Biochim. Biophys. Acta, Mol. Cell Biol. Lipids, 2018, 1863(9), 1095–1107 CrossRef CAS PubMed.
Footnotes |
† Electronic supplementary information (ESI) available: Experimental procedures for syntheses and biological evaluation, supplementary figures, schemes and tables, computational methods and details, cif files and X-ray data of 12, 15 and 17. CCDC 2310688–2310690. For ESI and crystallographic data in CIF or other electronic format see DOI: https://doi.org/10.1039/d4sc02002b |
‡ These authors contributed equally. |
|
This journal is © The Royal Society of Chemistry 2024 |
Click here to see how this site uses Cookies. View our privacy policy here.