DOI:
10.1039/D4SC03190C
(Edge Article)
Chem. Sci., 2024,
15, 13240-13249
Enantioconvergent and diastereoselective synthesis of atropisomeric hydrazides bearing a cyclic quaternary stereocenter through ternary catalysis†
Received
15th May 2024
, Accepted 27th June 2024
First published on 28th June 2024
Abstract
An efficient and highly enantioconvergent and diastereoselective ternary catalysis in a one-pot process is reported, which represents an integrated strategy for the synthesis of atropisomeric hydrazides with defined vicinal central and axial chirality from readily available racemic α-amino-ynones, azodicarboxylates, and Morita–Baylis–Hillman (MBH) carbonates. This method utilizes in situ-generated racemic pyrrolin-4-ones via hydroamination of racemic α-amino-ynones by AuCl catalysis as a novel and versatile C1 synthon, which engage commercially available azodicarboxylates to generate amination products in high yields and uniformly excellent enantioselectivities under the catalysis of a chiral phosphoric acid. Following amination, N-alkylation catalyzed by diastereoselective organocatalyst afforded axially chiral hydrazides with excellent diastereoselectivities (>98
:
2 dr). The synthetic utility of the amination products and axially chiral hydrazides was also demonstrated by their facile conversion to diverse molecules in high yields with excellent stereopurity. Density functional theory calculations were performed to understand the origin of diastereoselectivity.
Introduction
One emerging strategy for synthesizing complex chiral molecules is to use multiple catalysts to promote asymmetric tandem reactions, which features sequential activation of the starting materials or in situ-generated intermediates, with high levels of step- and atom-economy.1 Remarkable advances have been made with asymmetric cascade reactions using three combinations of two distinct catalysts (i.e., metal/metal, metal/organo, and organo/organo). These combinations enable enantio- and diastereoselective asymmetric ternary catalysis; however, the methods are quite limited.2 As illustrated in Scheme 1a, where ternary catalysis is shown to be a cascade process in which three catalytic cycles are involved, intermediate I is formed in the first catalytic transformation and engages in the second catalyzed reaction to afford intermediate II. This is then subjected to a third catalyzed transformation to deliver molecular complexity from simple starting materials. Racemic pyrrolin-4-one, which is easily accessed from readily available racemic α-amino-ynones under gold catalysis,3 may serve as a highly effective nucleophilic synthon for the ternary catalytic reaction. However, the functionalization of pyrrolin-4-ones using this strategy remains elusive (see Scheme 1b).
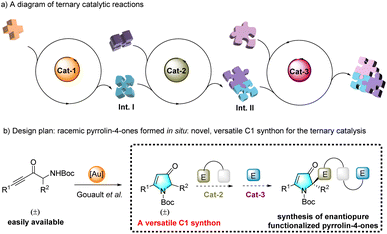 |
| Scheme 1 Ternary catalytic reactions for the efficient synthesis of complex chiral molecules. | |
The hydrazides are a class of highly valuable compounds. Some hydrazides, such as aza-peptide analog CGP 53820, are bioactive molecules, exhibiting potent inhibition of HIV-1 and HIV-2 protease. Others are used in environmentally benign insecticides (e.g., halofenozide and derivatives; Scheme 2(a)).4 However, several methods for the enantioselective synthesis of N–N axially chiral heterocyclic architectures have been reported. Nonetheless, the relevant studies focused only on their atroposelective creation.5 Recently, the elegant catalytic enantio- and diastereoselective synthesis of axially chiral hydrazides was achieved via two sequential asymmetric organocatalytic cycles in one pot (see Scheme 2b).6 However, synthesizing enantiopure N–N axially chiral hydrazides bearing a cyclic quaternary stereocenter requires multiple steps. Rinaldi et al. reported two such methods. The first employs an enantiopure ester substrate to control the installation of N–N axial chirality, obtaining the desired product in 17% yield in five steps with a low dr of 2.4
:
1 (see Scheme 2c, eqn (1)).7 The second method employs an enantiopure diastereomeric ester to afford an N–N axially chiral product in 36% yield over three steps and a low dr of 1.2
:
1 (Scheme 2c, eqn (2)).8 Notably, only azo dicarboxylates with a tert-butyl group (excessive steric hindrance) are used as the nitrogen source in both reactions. In summary, an efficient catalytic approach for the synthesis of optically pure atropisomeric hydrazides with a wide substrate scope is yet to be developed.
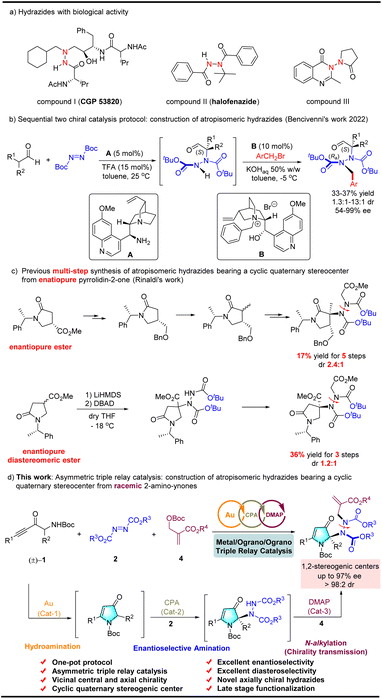 |
| Scheme 2 Bioactive molecules containing the hydrazides and synthesis of the optically active atropisomeric hydrazides bearing a cyclic quaternary stereocenter. | |
To improve the field of axially chiral entities9 and asymmetric relay catalytic reactions,10 we examined the possibility of constructing axially chiral hydrazides through ternary catalysis. Hence, this study reports an unprecedented example of ternary catalysis that integrates gold, Brønsted chiral phosphoric acid (CPA), and an organocatalyst (DMAP), and we demonstrate its effectiveness in the highly enantioconvergent and diastereoselective construction of atropisomeric hydrazides via a one-pot sequence involving an intramolecular hydroamination, an intermolecular asymmetric amination, and N-alkylation (see Scheme 2d).
Experimental section
We initially designed the enantioconvergent synthesis of 3 by employing the dual catalysis of achiral AuCl and CPA as a starting point for studying intramolecular hydroamination, followed by asymmetric amination to facilitate the subsequent development of annulation reactions. We surveyed the reaction between racemic α-amino-ynone (1a) and dibenzyl azodicarboxylate (2a)11 with AuCl12 and CPAs13via dual catalysis strategies.14 As listed in Table 1, various CPAs can enable the relay reaction to proceed smoothly and afford the desired product 3a in 5–86% yield at a high level (83–88%) of ee values (see Table 1, entries 1–5). Hence, the common commercially available CPA3 was used as the catalyst, and the desired enantioconvergent amination 3a was obtained in 86% yield and 88% ee (see Table 1, entry 3). Moreover, different solvents and molecular sieves were tested in the presence of CPA3 (see Table 1, entries 6–10). With the optimal choice of cyclohexane and 5 Å MS, an excellent ee of 93% was observed for 3a.
Table 1 Reaction condition screeninga
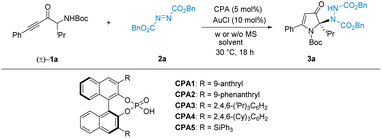
|
Entry |
CPA |
Solvent |
Yield 3ab (%) |
ee 3ac (%) |
The reaction was carried out with 1a (0.06 mmol, 1.2 equiv.), azo dicarboxylate 2a (0.05 mmol, 1.0 equiv.), AuCl (10 mol%), CPA (5 mol%) in solvent (1 mL) under a N2 atmosphere at 30 °C for 18 h.
Isolated yield of 3a with respect to 2a.
Determined by HPLC.
With additive 4 Å MS (50 mg).
With additive 5 Å MS (50 mg).
|
1 |
CPA1
|
Toluene |
78 |
83 |
2 |
CPA2
|
Toluene |
72 |
85 |
3 |
CPA3
|
Toluene |
86 |
88 |
4 |
CPA4
|
Toluene |
80 |
88 |
5 |
CPA5
|
Toluene |
<5 |
— |
6 |
CPA3
|
MTBE |
<5 |
— |
7 |
CPA3
|
THF |
<5 |
— |
8 |
CPA3
|
Cyclohexane |
90 |
91 |
9d |
CPA3
|
Cyclohexane |
90 |
92 |
10e |
CPA3
|
Cyclohexane |
93 |
93 |
Results and discussion
With reaction conditions optimized, we next examined the generality of the catalytic reaction (see Scheme 3), starting with a series of commercially available azodicarboxylates (i.e., DEAD, DIAD, and DBAD) reacting with racemic α-amino-ynone 1a to afford products (3a–3c). Good yields (92–96%) and excellent enantioselectives (97%) of products 3b and 3c were obtained for ethyl and isopropyl azodicarboxylates. The absolute configuration of 3b was unambiguously determined by single-crystal X-ray diffraction (XRD). The configurations of other products were assigned as analogies; however, when azo dicarboxylates with tert-butyl groups (excessive steric hindrance) were used as the nitrogen source, the target product was not observed.
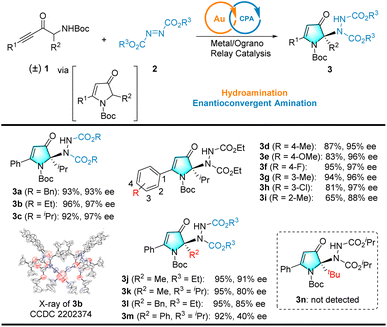 |
| Scheme 3 Scope of gold and CPA. | |
Variations in racemic α-amino-ynones 1 were explored next. Notably, the reaction can tolerate a wide variety of electron-donating or -withdrawing groups at different positions of the phenyl group. Furthermore, the yields and enantioselectivities of the corresponding products 3d–3i remained high. Alternatively, when using the less bulky racemic α-amino-ynones 1 bearing simple methyl or benzyl substituents, the reaction provided 3j–3l in high yields and 80–91% ee. The alkyl group was replaced with an aryl group that afforded product 3m with high yield and moderate enantioselectivity (40% ee). The R2 group was replaced with a tert-butyl group, which failed to provide the desired product 3n, presumably due to the size of the tert-butyl group. Fourteen additional examples are illustrated in Scheme S1 (see the ESI† for details). Consequently, this novel cascade reaction represents an atom- and step-economical approach.
We then turned our attention to the N-alkylation reaction of the intramolecular hydroamination/intermolecular amination reaction to create atropisomeric hydrazides (see Scheme 4).15 To our delight, the racemic 3k underwent an N-alkylation reaction in the presence of the Morita–Baylis–Hillman (MBH) carbonate 4a, achiral catalytic DMAP, and toluene to deliver an axially chiral hydrazide bearing a cyclic quaternary stereocenter 5 with 90% yield but only 1
:
1 dr (Scheme 4, eqn (1)). Nonetheless, the dr value can be improved to 2
:
1 when the solvent is switched to cyclohexane, which we used to prepare substrate 3via a relay catalytic reaction. Enantioenriched 3k was employed in the reaction, and the desired product 5 was obtained with the same enantiopurity. We speculate that the steric hindrance of the isopropyl groups may have affected diastereoselectivity. Notably, either racemic or enantiopure 3c was used as a substrate. Fortunately, a single diastereomer 6a was obtained at 92% yield, and high enantiopurity was retained without erosion (see Scheme 4, eqn (2)). Both CPA3 enantiomers were tested, and those corresponding to the product were obtained as single diastereomers. This result demonstrates that CPA is not effective for diastereoselectivity in N-alkylation with MBH carbonate (see Scheme 4, eqn (3)).
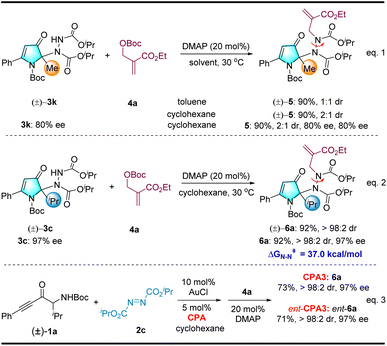 |
| Scheme 4 Investigation of axially chiral hydrazide and the effect of CPA. | |
The configurational stability of this new type of N–N-linked axially chiral compound was investigated both experimentally and computationally. Heating of product (6a) in toluene for 72 h at 150 °C led to no erosion of diastereopurity, which was corroborated by the high rotational barrier of 37.0 kcal mol−1 calculated for the N–N axis at 150 °C. Notably, the cyclohexane solvent used for the N-alkylation reaction was the same as that used for the AuCl/CPA relay catalytic reaction. Our next goal was to create axially chiral hydrazides bearing cyclic quaternary stereocenters via ternary catalysis. Representative optimization studies are provided in the ESI (see Table S1†).
Having optimized the reaction conditions, we turned to examine the generality of the ternary catalyst, by varying racemic α-amino-ynones first. A wide range of electron-donating or -withdrawing group substituents on the phenyl moiety at the 3- and 4-positions were tolerated to afford axially hydrazides 6a–6m in good yield and uniformly excellent diastereo- and enantioselectivity (see Scheme 5). Next, different MBH carbonate ester groups were evaluated, and methyl and allyl esters gave the desired products (6n and 6p) with moderate yields and excellent enantioselectivity. In contrast, bulkier isopropyl ester groups gave axially hydrazide 6o in moderate yield, but with slightly lower enantioselectivity (90% ee). Other branched alkyl groups (i.e., cyclopentyl and cyclohexyl) were introduced, and the corresponding products (6q and 6r) were obtained in a high yield with excellent enantioselectivity. A linear alkyl group (nPr) was also found to be compatible, yielding 6s in moderate yield and enantioselectivity but with a low dr (2
:
1). The α-amino-ynones 1 bearing the 4-nitrobenzyl substituent were also tested, and the reaction afforded 6t with 76% yield and 94% ee. The relative configuration of racemic 6t was determined by single-crystal XRD, and the absolute configuration of the quaternary carbon stereogenic center of 6 was determined to be R according to the X-ray structure analysis of 3b. To further confirm that the R,R-configuration product was the key experimental outcome, we compared the experimental electronic circular dichroism (ECD) spectra of enantiopure 6a with the calculated ECD spectra of the two stereoselective products (see the ESI†). The alkyl group was replaced by a phenyl group and afforded product 6u with high yield and moderate enantioselectivity (40% ee).
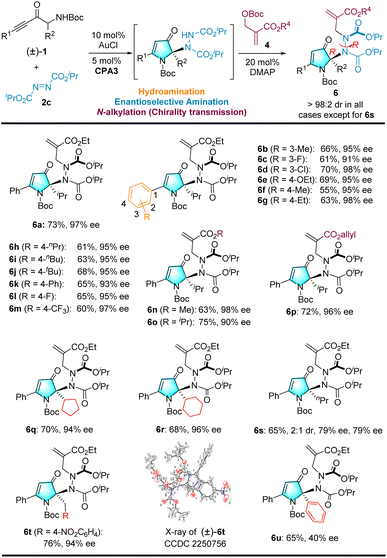 |
| Scheme 5 Scope of the atroposelective synthesis of hydrazides. | |
We hypothesized that two possible pathways exist for the AuCl/CPA relay catalytic reaction. Hence, to gain insights into the relay catalytic reaction pathway, control experiments were performed stepwise (see Scheme 6). First, racemic pyrrolin-4-one 7 was obtained as the product (90% yield) from racemic α-amino-ynone 1a under the catalysis of AuCl (10 mol%) without diethyl azodicarboxylate 2a or CPA3. Subsequently, treatment of 7 with diethyl azodicarboxylate 2a and chiral CPA3 (5 mol%) afforded an amination product 3b in 90% yield and 97% ee (see Scheme 6, eqn (1)). Additionally, the stepwise procedure delivered the desired products in lower two-step yields than our relay catalytic approach and efficiently avoided the loss of racemic pyrrolin-4-one intermediates during the additional purification. Alternatively, when the reaction was carried out with 1a and diethyl azodicarboxylate 2b in the presence of catalytic CPA3 (5 mol%), the desired product 8 was not observed (see Scheme 6, eqn (2)). These results demonstrated that the relay catalytic reaction followed the first pathway (see Scheme 6, eqn (1)).
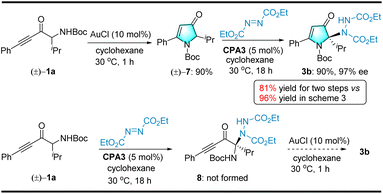 |
| Scheme 6 Control experiments. | |
To gain a better understanding of the reaction pathways, we monitored the kinetics of the reaction between 1a and 2b using 1H NMR spectroscopy (see Scheme 7). The kinetic profiles of this reaction clearly indicated that an essentially full conversion of 1a to the intermediate product 7 was observed within 1 h at 30 °C, which was then converted to the final product, 3b, in a remarkably high yield. Consequently, the two catalytic reactions were identified as relays.
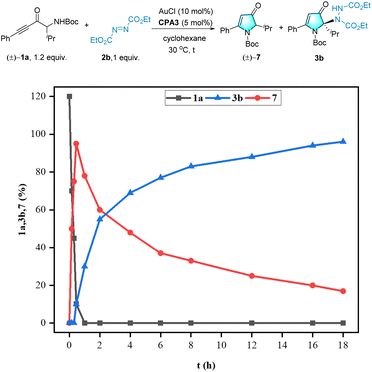 |
| Scheme 7
1H NMR studies revealed the two catalytic reactions. | |
Based on the above results, a plausible triple-relay catalytic reaction mechanism was proposed: (1) activation of racemic α-amino-ynones (1) by AuCl (viaI) yields intramolecular cyclization product II, and protonolysis of that then produces racemic pyrrolin-4-one 7. (2) Subsequently, 3-hydroxypyrroles were formed through enolization in the presence of CPA, and the CPA catalyst activated both reactants by forming dual H-bonds with 3-hydroxypyrroles (with their 3-hydroxy group) and azodicarboxylates, which underwent an additional reaction to provide the enantioenriched amination product 3, possessing a cyclic quaternary stereocenter, which is an enantio-determining step. (3) The first SN2′ reaction was triggered by the addition of the Lewis base catalyst DMAP to the MBH adduct, generating a good leaving group (OBoc), which grabbed the hydrogen from compound 3, delivering HOBoc (TBHC) and Int-V. TBHC decomposes into tBuOH and CO2. Later on, the second SN2′ reaction occurred through the addition of Int-V to the achiral pyridinium species, creating the N–N axis, and delivered the final products 6 with excellent diastereoseletivities and the organocatalyst (DMAP) to participate in the next catalytic cycle (Scheme 8).
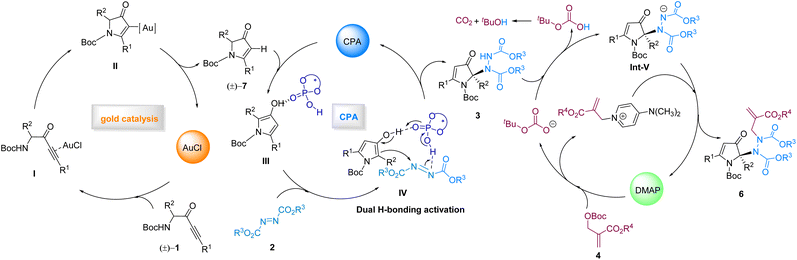 |
| Scheme 8 Proposed triple relay catalytic reaction mechanism. | |
To further demonstrate the utility of this methodology, we performed a one-mmol-scale reaction and synthetic transformations. As shown in Scheme 9(a), a representative one-mmol-scale reaction easily occurred to form product 3b in 95% yield and with excellent enantioselectivity, which demonstrated that this methodology can be utilized for synthesizing chiral pyrrolin-4-ones on a large scale. Relay catalytic products are tolerant of many functional groups and are valuable for versatile structural elaborations. Several illustrative transformations are illustrated in Scheme 9(b). As demonstrated, the Boc protecting group on 3b was easily removed under acidic conditions to generate free pyrrolin-4-one 9 in a moderate yield. Moreover, halogenation of 3b with NBS or iodine at 30 °C delivered fully-substituted pyrrolin-4-one 10 or 11 in 91 or 88% yield, respectively (see Scheme 9(b)). Brominated product 10 was coupled with phenylboronic acid to afford the desired product 12 with an 80% yield. Using this procedure, a wide range of boronic acids can be employed to afford various fully substituted pyrrolin-4-ones. Iodinated product 11 can be coupled with trimethylsilylacetylene to generate a cross-coupling product. The TMS group was efficiently removed to generate chiral terminal alkyne 13 in 75% yield over a two-step sequence in enantiopure form. Notably, the terminal alkynyl group in 13 is a versatile functional group,16 and the bromination of axially chiral hydrazide bearing a cyclic quaternary stereocenter 6a with NBS at 30 °C in DCM delivered the desired product 14 in 88% yield. Additionally, the hydrolysis of 6a at room temperature delivered free acid 15 in 84% yield (see Scheme 9(b)). Notably, in all of these transformations, excellent enantiopurity remained nearly without erosion.
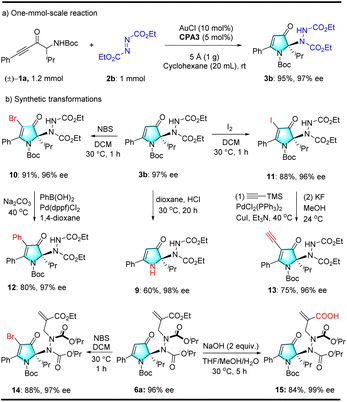 |
| Scheme 9 Product transformation. | |
Finally, we computationally investigated the N-alkylation of 3c in the presence of carbonate 4a and DMAP to form 6a to understand the origin of its high diastereoselectivity. Preliminary calculations demonstrated that the barrier of rotation around the N–N axis of 3c was 22.4 kcal mol−1 (see the ESI†), indicating that the axis was conformationally unstable and that the reaction diastereoselectivity was governed by the N-alkylation step. Subsequently, the entire reaction profile leading to the formation of RR-6a was calculated, and the results are illustrated in Scheme 10. The DMAP activation of 4a was calculated as a facile process with a barrier of 19.4 kcal mol−1. The generated INT2 contained a t-butyl carbonate anion, which serves as a Brønsted base to deprotonate the N–H moiety of 3c and turn it into a more potent nucleophile. The nucleophilic N–C bond formation, followed by the regeneration of the DMAP catalyst, yielded 6a. The highest-energy step was determined to be nucleophilic N–C bond formation with a ΔG295 value of 32.3 kcal mol−1.
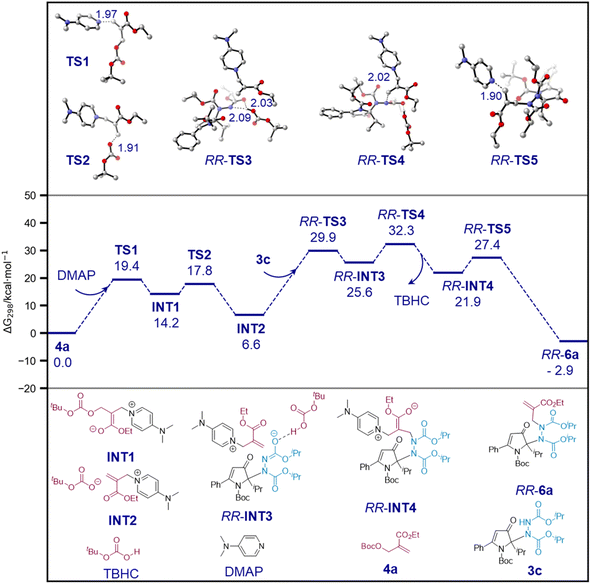 |
| Scheme 10 Calculated reaction profile of N-alkylation of 3c. ΔG298 values are reported in kcal mol−1 and distances in Å. | |
We then calculated the diastereoselectivity-determining transition states for N-alkylation of 3c and 3k, and the optimized structures are shown in Scheme 11. Although the calculated energy differences of 4.3 and 2.2 kcal mol−1 for 3c and 3k, respectively, would predict dr values much larger than those observed experimentally, there was evidence that this might be the consequence of the presence of numerous low-lying frequencies in the transition states. Hence, their treatment with quasi-harmonic approximation will lead to improved results. Furthermore, predictions based on enthalpy differences, which are less sensitive to low-lying frequencies, yielded improved results. Nevertheless, in all cases, our calculated results predicted better diastereoselectivity for 3c than 3k, which is in excellent agreement with the experimental results and can be attributed to the greater steric hindrance exerted by the i-Pr group of 3c. A very close H–H contact of 1.96 Å was observed between the i-Pr group of 3c and the C–H protons of the incoming electrophile. In comparison, the shortest H–H contact between the Me group of 3k and the electrophile was 2.56 Å.
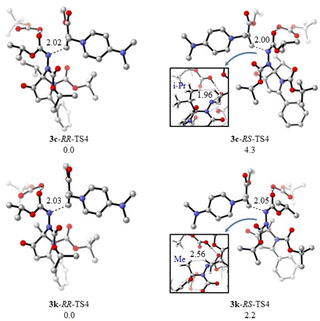 |
| Scheme 11 Diastereoselectivity of N-alkylation of 3c (top) and 3k (bottom). Enclosed are rotated structures showing the steric hindrance in 3c-RS-TS4. ΔG298 values are reported in kcal mol−1 and distances in Å. | |
Conclusions
We developed a catalytic, enantioconvergent, and diastereoselective synthesis of atropisomeric hydrazides from readily available starting materials. The cascade reaction proceeded via ternary catalysis involving gold(I) chloride, CPA, and DMAP, with all three catalytic cycles interwoven. A variety of racemic α-amino-ynones, azodicarboxylates, and MBH carbonates were successfully assembled in one pot in good yields and with excellent regioselectivities, which delivered the products with uniformly excellent control of central and axial chirality. DFT calculations revealed the origin of diastereoselectivity. The success of this work not only provides a useful strategy for the construction of contiguous cyclic quaternary central and N–N axial chirality in one pot but also represents the first example of a catalytic synthesis of optically pure N–N atropisomers via ternary catalysis. The development of new catalytic processes for accessing other challenging structures with different chiralities is currently under investigation.
Data availability
The data supporting this article have been uploaded as part of the ESI.†
Author contributions
S. L. conceived the project. X. W., S.-J. W., X. X. and H. A. performed the experiments and prepared the ESI.† H. Y. performed the DFT studies. S. L., Z. T., H. Y. and M. W. W. wrote the manuscript. All authors discussed the results.
Conflicts of interest
There are no conflicts to declare.
Acknowledgements
We are grateful for the generous financial support from the Program for Young Talents of Shaanxi Province (5113200043) and the Fundamental Research Funds for Central Universities.
Notes and references
- For selected reviews, see:
(a) L. F. Tietze, Domino Reactions in Organic Synthesis, Chem. Rev., 1996, 96, 115–136 CrossRef CAS;
(b) J. M. Lee, Y. Na, H. Han and S. Chang, Cooperative multi-catalyst systems for one-pot organic transformations, Chem. Soc. Rev., 2004, 33, 302–312 RSC;
(c) J. C. Wasilke, S. J. Obrey, R. T. Baker and G. C. Bazan, Concurrent Tandem Catalysis, Chem. Rev., 2005, 105, 1001–1020 CrossRef CAS PubMed;
(d) Z.-H. Shao and H.-B. Zhang, Combining Transition Metal Catalysis and Organocatalysis: A Broad New Concept for Catalysis, Chem. Soc. Rev., 2009, 38, 2745–2755 RSC;
(e) L. M. Am-brosini and T. H. Lambert, Multicatalysis: Advancing Synthetic Efficiency and Inspiring Discovery, ChemCatChem, 2010, 2, 1373–1380 CrossRef CAS;
(f) D. B. Ramachary and S. Jain, Sequential one-pot combination of multi-component and multi-catalysis cascade reactions: an emerging technology in organic synthesis, Org. Biomol. Chem., 2011, 9, 1277–1300 RSC;
(g) Z. T. Du and Z.-H. Shao, Combining Transition Metal Catalysis and Organocatalysis – an Update, Chem. Soc. Rev., 2013, 42, 1337–1378 RSC;
(h) D.-F. Chen, Z.-Y. Han, X.-L. Zhou and L.-Z. Gong, Asymmetric Organo-catalysis Combined with Metal Catalysis: Concept, Proof of Concept, and Beyond, Acc. Chem. Res., 2014, 47, 2365–2377 CrossRef CAS PubMed;
(i) T. L. Lohr and T. J. Marks, Orthogonal tandem catalysis, Nat. Chem., 2015, 7, 477–482 CrossRef CAS;
(j) Q.-J. Liang, Y.-H. Xu and T.-P. Loh, Multi-catalyst promoted asymmetric relay reactions, Org. Chem. Front., 2018, 5, 2765–2768 RSC;
(k) S. P. Sancheti, Urvashi, M. P. Shah and N. T. Patil, Ternary Catalysis: A Stepping Stone toward Multicatalysis, ACS Catal., 2020, 10, 3462–3489 CrossRef CAS;
(l)
B. A. Arndtsen and L.-Z. Gong, Topics in Current Chemistry Collections: Asymmetric Organocatalysis Combined with Metal Catalysis, Springer Nature, Cham, Switzerland, 2020 CrossRef;
(m) S. Martínez, L. Veth, B. Lainer and P. Dydio, Challenges and Opportunities in Multicatalysis, ACS Catal., 2021, 11, 3891–3915 CrossRef;
(n)
L.-Z. Gong, Asymmetric Organo-Metal Catalysis: Concepts, Principles, and Applications, Wiley-VCH, Weinheim, Germany, 2021 Search PubMed;
(o) D.-F. Chen and L.-Z. Gong, Organo/Transition-Metal Combined Catalysis Rejuvenates Both in Asymmetric Synthesis, J. Am. Chem. Soc., 2022, 144, 2415–2437 CrossRef CAS.
- For the enantio- and diastereoselective asymmetric triple relay catalysis, see:
(a) B. Simmons, A. M. Walji and D. W. C. MacMillan, Cycle-Specific Organocascade Catalysis: Application to Olefin Hydroamination, Hydro-oxidation, and Amino-oxidation, and to Natural Product Synthesis, Angew. Chem., Int. Ed., 2009, 48, 4349–4353 CrossRef CAS;
(b) W.-L. Yang, X.-Y. Shang, T. Ni, H. Yan, X. Luo, H. Zheng, Z. Li and W.-P. Deng, Diastereo- and Enantioselective Synthesis of Bisbenzannulated Spiroketals and Spiroaminals by Ir/Ag/Acid Ternary Catalysis, Angew. Chem., Int. Ed., 2022, 61, e202210207 CrossRef CAS . For the selected enantioselective asymmetric triple relay catalysis, see: ;
(c) X.-P. Yin, X.-P. Zeng, Y.-L. Liu, F.-M. Liao, J.-S. Yu, F. Zhou and J. Zhou, Asymmetric Triple Relay Catalysis: Enantioselective Synthesis of Spirocyclic Indolines through a One-Pot Process Featuring an Asymmetric 6π Electrocyclization, Angew. Chem., Int. Ed., 2014, 53, 13740–13745 CrossRef CAS;
(d) Z. Kang, W. Chang, X. Tian, X. Fu, W. Zhao, X. Xu, Y. Liang and W. Hu, Ternary Catalysis Enabled Three-Component Asymmetric Allylic Alkylation as a Concise Track to Chiral α,α-Disubstituted Ketones, J. Am. Chem. Soc., 2021, 143, 20818–20827 CrossRef CAS PubMed.
- N. Gouault, M. L. Roch, C. Cornée, M. David and P. Uriac, Synthesis of Substituted Pyrrolin-4-ones from Amino Acids in Mild Conditions via a Gold-Catalyzed Approach, J. Org. Chem., 2009, 74, 5614–5617 CrossRef CAS PubMed.
-
(a) J. P. Priestley, A. Fässler, J. Rösel, M. Tintelnot-Blomley, P. Strop and M. G. Grütter, Comparative analysis of the X-ray structures of HIV-1 and HIV-2 proteases in complex with CCP 53820, a novel pseudosym-metric inhibitor, Structure, 1995, 3, 381–389 CrossRef;
(b) M. J. Mulvihill, S. H. Sha-ber, B. S. MacDougall, C. Ajello, B. Martinez-Teipel, R. Joseph, D. V. Nguyen, D. G. Weaver, K. Chung, A. Gusev, J. M. Wierenga and W. D. Mathis, Synthesis of Insecticidally Active Halofenozide-[(Acyloxy)alkoxy]carbonyl and (Acyloxy)alkyl Derivatives, Synthesis, 2002, 53–58 CAS;
(c) S. Ke, T. Sun, Y. Liang and Z. Yang, Research Advance of Acylhydrazine Derivatives with Biological Activities, Chin. J. Org. Chem., 2010, 30, 1820–1830 CAS.
-
(a) G.-J. Mei, J. J. Wong, W. Zheng, A. A. Nangia, K. N. Houk and Y. Lu, Rational design and atroposelective synthesis of N–N axially chiral compounds, Chem, 2021, 7, 2743–2757 CrossRef CAS;
(b) G. Centonze, C. Portolani, P. Righi and G. Bencivenni, Enantioselective Strategies for The Synthesis of N–N Atropisomers, Angew. Chem., Int. Ed., 2023, 62, e202303966 CrossRef;
(c) J. Feng, C.-J. Lu and R.-R. Liu, Catalytic Asymmetric Synthesis of Atropisomers Featuring an Aza Axis, Acc. Chem. Res., 2023, 56, 2537–2554 CrossRef CAS;
(d) H.-H. Zhang, T.-Z. Li, S.-J. Liu and F. Shi, Catalytic Asymmetric Synthesis of Atropisomers Bearing Multipl Chiral Elements: An Emerging Field, Angew. Chem., Int. Ed., 2023, 62, e202311053 Search PubMed;
(e) X.-M. Wang, P. Zhang, Q. Xu, C.-Q. Guo, D.-B. Zhang, C.-J. Lu and R.-R. Liu, Enantioselective Synthesis of Nitrogen–Nitrogen Biaryl Atropisomers via Copper-Catalyzed Friedel–Crafts Alkylation Reaction, J. Am. Chem. Soc., 2021, 143, 15005–15010 CrossRef CAS PubMed;
(f) P. Zhang, Q. Xu, X.-M. Wang, J. Feng, C.-J. Lu, Y. Li and R.-R. Liu, Enantioselective Synthesis of N-N Bisindole Atropisomers, Angew. Chem., Int. Ed., 2022, 61, e202212101 CrossRef CAS;
(g) Y. Gao, L.-Y. Wang, T. Zhang, B.-M. Yang and Y. Zhao, Atroposelective Synthesis of 1,1′-Bipyrroles Bearing a Chiral N–N Axis: Chiral Phosphoric Acid Catalysis with Lewis Acid Induced Enantiodivergence, Angew. Chem., Int. Ed., 2022, 61, e202200371 CrossRef CAS;
(h) K.-W. Chen, Z.-H. Chen, S. Yang, S.-F. Wu, Y.-C. Zhang and F. Shi, Organocatalytic Atroposelective Synthesis of N–N Axially Chiral Indoles and Pyrroles by De Novo Ring Formation, Angew. Chem., Int. Ed., 2022, 61(No), e202116829 CrossRef CAS;
(i) W. Lin, Q. Zhao, Y. Li, M. Pan, C. Yang, G. Yang and X. Li, Asymmetric Synthesis of N–N Axially Chiral Compounds via Organocatalytic Atroposelective N-Acylation, Chem. Sci., 2022, 13, 141–148 RSC;
(j) Q. Xu, H. Zhang, F.-B. Ge, X.-M. Wang, P. Zhang, C.-J. Lu and R.-R. Liu, Cu(I)-Catalyzed Asymmetric Arylation of Pyrroles with Diaryliodonium Salts toward the Synthesis of N–N Atropisomers, Org. Lett., 2022, 24, 3138–3143 CrossRef CAS;
(k) L.-Y. Pu, Y.-J. Zhang, W. Liu and F. Teng, Chiral Phosphoric Acid-Catalyzed Dual-Ring Formation for Enantioselective Construc-tion of N–N Axially Chiral 3,3′-Bisquinazolinones, Chem. Commun., 2022, 58, 13131–13134 RSC;
(l) V. Hutskalova and C. Sparr, Control over Stereogenic N-N Axes by Pd-Catalyzed 5-endo-Hydroaminocyclizations, Synthesis, 2023, 55, 1770–1782 CrossRef CAS;
(m) Z.-H. Chen, T.-Z. Li, N.-Y. Wang, X.-F. Ma, S.-F. Ni, Y.-C. Zhang and F. Shi, Organocatalytic Enantioselective Synthesis of Axially Chiral N,N′-Bisindoles, Angew. Chem., Int. Ed., 2023, 62, e202300419 CrossRef CAS PubMed;
(n) L.-Y. Wang, J. Miao, Y. Zhao and B.-M. Yang, Chiral Acid-Catalyzed Atroposelective Indolization Enables Access to 1,1′-Indole-Pyrroles and Bisindoles Bearing a Chiral N–N Axis, Org. Lett., 2023, 25, 1553–1557 CrossRef CAS PubMed;
(o) W. Yao, C.-J. Lu, L.-W. Zhan, Y. Wu, J. Feng and R.-R. Liu, Enantioselective Synthesis of N–N Atropisomers by Palladium-Catalyzed C–H Functionalization of Pyrroles, Angew. Chem., Int. Ed., 2023, 62, e202218871 CrossRef CAS;
(p) S.-Y. Yin, Q. Zhou, C.-X. Liu, Q. Gu and S.-L. You, Enantioselective Synthesis of N–N Biaryl Atropisomers through Iridium(I)-Catalyzed C–H Alkylation with Acrylates, Angew. Chem., Int. Ed., 2023, 62, e202305067 CrossRef CAS PubMed;
(q) K. Balanna, S. Barik, S. Barik, S. Shee, N. Manoj, R. G. Gonnade and A. T. Biju, N-Heterocyclic Carbene-Catalyzed Atroposelective Synthesis of N–N Axially Chiral 3-Amino Quinazolinones, ACS Catal., 2023, 13, 8752–8759 CrossRef CAS;
(r) C. Wang and J. Sun, Atroposelective Synthesis of N–N Axially Chiral Bipyrroles via Rhodium-Catalyzed C–H Insertion Reaction, Org. Lett., 2023, 25, 4808–4812 CrossRef CAS PubMed;
(s) X. Zhu, H. Wu, Y. Wang, G. Huang, F. Wang and X. Li, Rhodium-Catalyzed Annulative Approach to N-N Axially Chiral Biaryls via C-H Activation and Dynamic Kinetic Transformation, Chem. Sci., 2023, 14, 8564–8569 RSC;
(t) Y. Wang, X. Zhu, D. Pan, J. Jing, F. Wang, R. Mi, G. Huang and X. Li, Rhodium-catalyzed enantioselective and diastereodivergent access to diaxially chiral heterocycles, Nat. Commun., 2023, 14, 4661 CrossRef CAS PubMed;
(u) T. Li, L. Shi, X. Wang, C. Yang, D. Yang, M.-P. Song and J.-L. Niu, Cobalt-catalyzed atroposelective C–H activation/annulation to access N–N axially chiral frameworks, Nat. Commun., 2023, 14, 5271 CrossRef CAS PubMed;
(v) C.-S. Wang, Q. Xiong, H. Xu, H.-R. Yang, T. Dang, X.-Q. Dong and C.-J. Wang, Organocatalytic Atroposelective Synthesis of Axially Chiral N,N′-Pyrroloindoles via De Novo Indole Formation, Chem. Sci., 2023, 14, 12091–12097 RSC;
(w) Q. Huang, Y. Li, C. Yang, W. Wu, J. Hai and X. Li, Atroposelective synthesis of N–N axially chiral pyrrolyl(aza)-quinolinone by de novo ring formation, Org. Chem. Front., 2024, 11, 726–734 RSC;
(x) F.-B. Ge, Q.-K. Yin, C.-J. Lu, X. Xuan, J. Feng and R.-R. Liu, Enantioselective Synthesis of Benzimidazole Atropisomers Featuring a N-N Axis, Chin. J. Chem., 2024, 42, 711–718 CrossRef CAS;
(y) C. Song, C. Pang, Y. Deng, H. Cai, X. Gan and Y. R. Chi, Catalytic N-Acylation for Access to N–N Atropisomeric N-Aminoindoles: Choice of Acylation Reagents and Mechanistic Insights, ACS Catal., 2024, 14, 6926–6935 CrossRef CAS;
(z) S. S. Ranganathappa, B. S. Dehury, G. K. Singh, S. Shee and A. T. Biju, Atroposelective Synthesis of N–N Axially Chiral Indoles and Pyrroles via NHC-Catalyzed Diastereoselective (3 + 3) Annulation Strategy, ACS Catal., 2024, 14, 6965–6972 CrossRef CAS.
- C. Portolani, G. Centonze, S. Luciani, A. Pellegrini, P. Righi, A. Mazzanti, A. Ciogli, A. Sorato and G. Bencivenni, Synthesis of Atropisomeric Hydrazides by One-Pot Sequential Enantioselective and Diastereoselective Catalysis, Angew. Chem., Int. Ed., 2022, 61, e202209895 CrossRef CAS PubMed.
- P. Amabili, A. Amici, A. Civitavecchia, B. Maggiore, M. Orena, S. Rinaldi and A. Tolomelli, Highly stable atropisomers by electrophilic amination of a chiral γ-lactam within the synthesis of an elusive conformationally restricted analogue of α-methylhomoserine, Amino Acid, 2016, 48, 461–478 CrossRef CAS PubMed.
- P. Amabili, A. Amici, G. Campisi, G. Guerra, M. Monari, M. Orena, F. Piccinelli, S. Rinaldi and A. Tolomelli, Synthesis of Enantiopure Isosteres of Amino Acids Containing a Quaternary Stereocenter: Experimental and Computational Evaluation of a Novel Class of Atropisomers, Eur. J. Org Chem., 2018, 6524–6536 CrossRef CAS.
-
(a) S.-C. Zhang, S. Liu, X. Wang, S.-J. Wang, H. Yang, L. Li, B. Yang, M. W. Wong, Y. Zhao and S. Lu, Enantioselective Access to Triaryl-2-pyrones with Monoaxial or Contiguous C–C Diaxes via Oxidative NHC Catalysis, ACS Catal., 2023, 13, 2565–2575 CrossRef CAS;
(b) S.-J. Wang, X. Wang, X. Xin, S. Zhang, H. Yang, M. W. Wong and S. Lu, Organocatalytic diastereo- and atroposelective construction of N–N axially chiral pyrroles and indoles, Nat. Commun., 2024, 15, 518 CrossRef CAS;
(c) X. Wang, S. Zhang, S.-J. Wang, H. An, X. Xin, H. Lin, Z. Tu and S. Lu, An Assembly of Pyrano[3,2-b]indol-2-ones via NHC-Catalyzed [3 + 3] Annulation of Indolin-3-ones with Ynals, Chin. J. Chem., 2024, 42, 1487–1492 CrossRef CAS.
- J. Jiang, X. Wang, S. Liu, S. Zhang, B. Yang, Y. Zhao and S. Lu, Enantioselective Cascade Annulation of α-Amino-ynones and Enals Enabled by Gold and Oxidative NHC Relay Catalysis, Angew. Chem., Int. Ed., 2022, 61, e202115464 CrossRef CAS PubMed.
-
(a) S. Yarlagadda, B. Ramesh, C. R. Reddy, L. Srinivas, B. Sridhar and B. V. S. Reddy, Organocatalytic Enantioselective Amination of 2-Substituted Indolin-3-ones: A Strategy for the Synthesis of Chiral α-Hydrazino Esters, Org. Lett., 2017, 19, 170–173 CrossRef CAS PubMed;
(b) J. Guo, Z.-H. Lin, K.-B. Chen, Y. Xie, A. S. C. Chan, J. Weng and G. Lu, Asymmetric amination of 2-substituted indolin-3-ones catalyzed by natural cinchona alkaloids, Org. Chem. Front., 2017, 4, 1400–1406 RSC;
(c) X. Kong, Q. Liu, Y. Chen, W. Wang, H.-F. Chen, W. Wang, S. Zhang, X. Chen and Z.-Y. Cao, Direct electrochemical synthesis of arenesulfonyl fluorides from nitroarenes: a dramatic ionic liquid effect, Green Chem., 2024, 26, 3435–3440 RSC.
- For selected recent reviews on gold chemistry, see:
(a) M. Bandini, Gold-catalyzed decorations of arenes and heteroarenes with C–C multiple bonds, Chem. Soc. Rev., 2011, 40, 1358–1367 RSC;
(b) A. Corma, A. Leyva-Pérez and M. J. Sabater, Gold-Catalyzed Carbon–Heteroatom Bond-Forming Reactions, Chem. Rev., 2011, 111, 1657–1712 CrossRef CAS PubMed;
(c) A. S. K. Hashmi and M. Rudolph, Gold catalysis in total synthesis-an update, Chem. Soc. Rev., 2012, 41, 2448–2462 RSC;
(d) L. Liu and G. B. Hammond, Recent advances in the isolation and reactivity of organogold complexes, Chem. Soc. Rev., 2012, 41, 3129–3139 RSC;
(e) A. S. K. Hashmi, Dual Gold Catalysis, Acc. Chem. Res., 2014, 47, 864–876 CrossRef CAS;
(f) L. Zhang, A Non-Diazo Approach to α-Oxo Gold Carbenes via Gold-Catalyzed Alkyne Oxidation, Acc. Chem. Res., 2014, 47, 877–888 CrossRef CAS PubMed;
(g) C. Obradors and A. M. Echavarren, Gold-Catalyzed Rearrangements and Beyond, Acc. Chem. Res., 2014, 47, 902–912 CrossRef CAS PubMed;
(h) D. Qian and J. Zhang, Gold-catalyzed cyclopropanation reactions using a carbenoid precursor toolbox, Chem. Soc. Rev., 2015, 44, 677–698 RSC;
(i) W. Zi and F. D. Toste, Recent advances in enantioselective gold catalysis, Chem. Soc. Rev., 2016, 45, 4567–4589 RSC;
(j) A. Fgrstner, Gold Catalysis for Heterocyclic Chemistry: A Representative Case Study on Pyrone Natural Products, Angew. Chem., Int. Ed., 2018, 57, 4215–4233 CrossRef;
(k) F.-L. Hong and L.-W. Ye, Transition Metal-Catalyzed Tandem Reactions of Ynamides for Divergent N-Heterocycle Synthesis, Acc. Chem. Res., 2020, 53, 2003–2019 CrossRef CAS;
(l) D. Campeau, D. F. León Rayo, A. Mansour, K. Muratov and F. Gagosz, Gold-Catalyzed Reactions of Specially Activated Alkynes, Allenes, and Alkenes, Chem. Rev., 2021, 121, 8756–8867 CrossRef CAS;
(m) Z. Zheng, X. Ma, X. Cheng, K. Zhao, K. Gutman, T. Li and L. Zhang, Homogeneous Gold-Catalyzed Oxidation Reactions, Chem. Rev., 2021, 121, 8979–9038 CrossRef CAS PubMed.
- For reviews of CPAs, see:
(a) T. Akiyama, Stronger brønsted acids, Chem. Rev., 2007, 107, 5744–5758 CrossRef CAS;
(b) M. Terada, Binaphthol derived phosphoric acid as a versatile catalyst for enantioselective carbon–carbon bond forming reactions, Chem. Commun., 2008, 4097–4112 RSC;
(c) M. Terada, Chiral phosphoric acids as versatile catalysts for enanti-oselective transformations, Synthesis, 2010, 1929–1982 CrossRef CAS;
(d) M. Rueping, A. Kuenkel and I. Atodiresei, Chiral Brønsted acids in enantioselective carbonyl activations-activation modes and applications, Chem. Soc. Rev., 2011, 40, 4539–4549 RSC;
(e) J. Yu, F. Shi and L.-Z. Gong, Brønsted-Acid-Catalyzed Asymmetric Multicomponent Reactions for the Facile Synthesis of Highly Enantioenriched Structurally Diverse Nitrogenous Heterocycles, Acc. Chem. Res., 2011, 44, 1156–1171 CAS;
(f) D. Parmar, E. Sugiono, S. Raja and M. Rueping, Complete Field Guide to Asymmetric BINOL-Phosphate Derived Brønsted Acid and Metal Catalysis: History and Classification by Mode of Activation; Brønsted Acidity, Hydrogen Bonding, Ion Pairing, and Metal Phosphates, Chem. Rev., 2014, 114, 9047–9153 CAS;
(g) D. Parmar, E. Sugiono, S. Raja and M. Rueping, Addition and Correction to Complete Field Guide to Asymmetric BINOL-Phosphate Derived Brønsted Acid and Metal Catalysis: History and Classification by Mode of Activation; Brønsted Acidity, Hydrogen Bonding, Ion Pairing, and Metal Phosphates, Chem. Rev., 2017, 117, 10608–10620 CrossRef CAS PubMed;
(h) Z.-L. Xia, Q.-F. Xu, C. Zheng and S.-L. You, Chiral phosphoric acid-catalyzed asymmetric dearomatization reactions, Chem. Soc. Rev., 2020, 49, 286–300 RSC;
(i) S. Li, S.-H. Xiang and B. Tan, Chiral Phosphoric Acid Creates Promising Opportunities for Enantioselective Photoredox Catalysis, Chin. J. Chem., 2020, 38, 213–214 CAS;
(j) X. Lin, L. Wang, Z. Han and Z. Chen, Chiral Spirocyclic Phosphoric Acids and Their Growing Applications, Chin. J. Chem., 2021, 39, 802–824 CrossRef CAS.
- For a review on the gold/CPA dual asymmetric catalysis reaction: P.-S. Wang, D.-F. Chen and L.-Z. Gong, Recent Progress in Asymmetric Relay Catalysis of Metal Complex with Chiral Phosphoric Acid, Top. Curr. Chem., 2020, 378, 9 Search PubMed . For gold(I)/CPA relay catalysis, see: Z.-Y. Han, H. Xiao, X.-H. Chen and L.-Z. Gong, Consecutive Intramolecular Hydroamination/Asymmetric Transfer Hydrogenation under Relay Catalysis of an Achiral Gold Complex/Chiral Brønsted Acid Binary System, J. Am. Chem. Soc., 2009, 131, 9182–9183 CrossRef CAS PubMed; M. E. Muratore, C. A. Holloway, A. W. Pilling, R. I. Storer, G. Trevitt and D. J. Dixon, Enantioselective Brønsted Acid-Catalyzed N-Acyliminium Cyclization Cascades, J. Am. Chem. Soc., 2009, 131, 10796–10797 CrossRef PubMed; Z.-Y. Han, D.-F. Chen, Y.-Y. Wang, R. Guo, P.-S. Wang, C. Wang and L.-Z. Gong, Hybrid Metal/Organo Relay Catalysis Enables Enynes To Be Latent Dienes for Asymmetric Diels–Alder Reaction, J. Am. Chem. Soc., 2012, 134, 6532–6535 CrossRef; H. Wu, Y.-P. He and L.-Z. Gong, Direct Access to Enantioenriched Spiroacetals through Asymmetric Relay Catalytic Three-Component Reaction, Org. Lett., 2013, 15, 460–463 CrossRef PubMed; F. Zhao, N. Li, Y.-F. Zhu and Z.-Y. Han, Enantioselective Construction of Functionalized Tetrahydrocarbazoles Enabled by Asymmetric Relay Catalysis of Gold Complex and Chiral Brønsted Acid, Org. Lett., 2016, 18, 1506–1509 CrossRef PubMed; H. Wei, M. Bao, K. Dong, L. Qiu, B. Wu, W. Hu and X. Xu, Enantioselective Oxidative Cyclization/Mannich Addition Enabled by Gold(I)/Chiral Phosphoric Acid Cooperative Catalysis, Angew. Chem., Int. Ed., 2018, 57, 17200–17204 CrossRef PubMed; S. Zhou, X. Liu, W. Hu, Z. Ke and X. Xu, Enantioselective Oxidative Multi-Functionalization of Terminal Alkynes with Nitrones and Alcohols for Expeditious Assembly of Chiral α-Alkoxy-β-amino-ketones, J. Am. Chem. Soc., 2021, 143, 14703–14711 CrossRef; X.-S. Liu, Z. Tang, Z.-Y. Si, Z. Zhang, L. Zhao and L. Liu, Enantioselective para-C(sp2)–H Functionalization of Alkyl Benzene Derivatives via Cooperative Catalysis of Gold/Chiral Brønsted Acid, Angew. Chem., Int. Ed., 2022, 61, e202208874 CrossRef.
- S. Lu, S. V. H. Ng, K. Lovato, J.-Y. Ong, S. B. Poh, X. Q. Ng, L. Kürti and Y. Zhao, Practical access to axially chiral sulfonamides and biaryl amino phenols via organocatalytic atroposelective N-alkylation, Nat. Commun., 2019, 10, 3061 Search PubMed.
-
(a) J. S. Moore, Shape-Persistent Molecular Architectures of Nanoscale Dimension, Acc. Chem. Res., 1997, 30, 402–413 CrossRef CAS;
(b) A. D. Finke, D. E. Gross, A. Han and J. S. Moore, Engineering Solid-State Morphologies in Carbazole–Ethynylene Macrocycles, J. Am. Chem. Soc., 2011, 133, 14063–14070 CrossRef CAS.
|
This journal is © The Royal Society of Chemistry 2024 |
Click here to see how this site uses Cookies. View our privacy policy here.