DOI:
10.1039/D4SC05111D
(Edge Article)
Chem. Sci., 2024,
15, 16169-16175
A switch strategy for the synthesis of C4-ethylamine indole and C7-aminoindoline via controllable carbon elimination†
Received
31st July 2024
, Accepted 5th September 2024
First published on 6th September 2024
Abstract
Controllable β-carbon elimination to extrude norbornene remains a long-standing challenge in palladium and norbornene chemistry. Herein, this manuscript describes a switchable synthesis of biologically active C4-ethylaminoindole and C7-aminoindoline scaffolds by controlling β-carbon elimination, utilizing aziridine as a C–H ethylamination reagent through a C–N bond cleavage reaction. Furthermore, the protecting groups of the product can be easily removed, offering an unusual method for the synthesis of dopamine receptor agonists.
Introduction
Indole and indoline are the most common heterocycles widely found in nature, possessing unique biological activities.1 Among them, ethylamino indole is involved in the entire metabolic process of the human body.2 For example, tryptophan is one of the essential amino acids for humans, involved in protein synthesis.3 Melatonin is a key hormone regulating the body's biological clock, and serotonin is an important neurotransmitter closely related to feelings of happiness and well-being.4 Specifically, C4-ethylamino indole derivatives have also been proven to be dopamine receptor agonists. One such derivative, ropinirole, is a medication used to treat Parkinson's disease (PD) and restless legs syndrome (RLS). It is one of the most commonly prescribed medications in the United States.5 On the other hand, C7-aminoindoline is a type of microtubule protein inhibitor and is considered an important antitumor drug (Fig. 1a).6 Based on this, we envision that the one-step synthesis of C4-ethylamine indole and C7-aminoindoline using aziridine through C–N bond cleavage ring-opening reactions and C–H alkylation reactions is of significant importance.
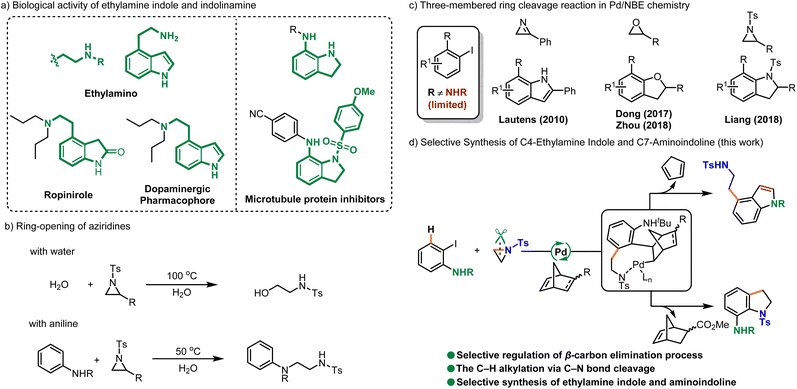 |
| Fig. 1 Selective synthesis of C4-ethylamine indole and C7-aminoindoline. | |
Palladium/norbornene (Pd/NBE) chemistry, namely Catellani-type reactions,7 provides a strategy for the multi-functionalization of arenes. This reaction integrates the features of C–H functionalization and cross-coupling.8 In 2000, the cyclization reaction catalyzed by Pd/NBE cooperatively was first discovered by Lautens.9 The cyclization reaction has since been widely used in materials chemistry, natural product synthesis, and pharmaceutical synthesis.8i,10 In 2009, Lautens discovered that using norbornadiene (NBD) instead of norbornene (NBE) in non-Catellani-type cascade cyclization reactions triggers a retro-Diels–Alder reaction, providing a method for synthesizing isoquinolinones and indoles.11 In recent years, Liang,10d Kwong,12 Cheng,13 and our group10d,14 developed a series of tandem cyclization reactions combining Catellani-type reactions with retro-Diels–Alder reactions. Interestingly, Cheng discovered that oxanorbornadiene exhibits better retro-Diels–Alder reaction activity than NBD in 2018.13a In recent years, the ring-opening C–H alkylation reaction of strained tricyclic heterocycles was achieved by Lautens,15 Dong,16 Zhou,17 and Liang10d,14,18 under Pd/NBE catalysis (Fig. 1c). However, C–H alkylation between o-iodoanilines and aziridines under Pd/NBE cooperative catalysis is difficult due to the susceptibility of aziridine to nucleophilic attack leading to ring-opening reactions (Fig. 1b).19
β-carbon elimination to extrude norbornene has consistently been a focal point of interest in Pd/NBE research.8g,20 In 2018, the Dong group utilized the steric hindrance effect of C1 norbornene to effectively promote β-carbon elimination and achieve single C–H functionalization of aryl iodides without an ortho-substituent.20c In 2019, Dong made a breakthrough in the halogenated olefin version by using norbornene amides.21 The Dong group further utilized this strategy to accelerate the extrusion of norbornene, inhibiting the formation of nitrene cyclization products and achieving the introduction of secondary amines in 2024.22 Interestingly, the Jiao group used hybrid cycloolefin ligands to achieve norbornene-like β-carbon elimination.23 However, controllable β-carbon elimination to extrude norbornene remains a long-standing challenge. Herein, this manuscript described a switchable synthesis of biologically active C4-ethylaminoindole and C7-aminoindoline scaffolds by controlling the β-carbon elimination, utilizing aziridine as a C–H ethylamination reagent through a C–N bond cleavage reaction.
Table 1 Optimization of reaction conditions for C4-ethylamine indolea
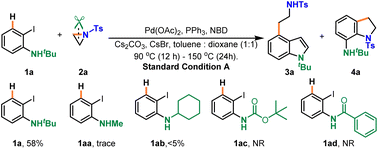
|
Entry |
Deviation from the standard conditions |
Yield (3a) |
Yield (4a) |
Standard conditions A: substrate 1a (0.2 mmol), 2a (0.5 mmol, 2.5 equiv.), Pd(OAc)2 (10 mmol%), PPh3(25 mmol%), CsBr (1.0 equiv.), Cs2CO3 (1.0 equiv.), NBD (0.6 mmol, 3.0 equiv.), toluene : dioxane (1 : 1, 2 mL) 90 °C 12 h, and then 150 °C, 24 h.
|
1 |
— |
58 |
Trace |
2 |
Toluene or dioxane as solvent |
31/40 |
8/trace |
3 |
Without CsBr |
48 |
Trace |
4 |
NBE instead of NBD |
0 |
16 |
5 |
K2CO3 instead of Cs2CO3 |
30 |
12 |
6 |
2.0 equiv. of Cs2CO3 |
43 |
Trace |
7 |
140 °C and 24 h |
32 |
18 |
Results and discussion
Initially, we used sterically hindered N-tert-butyl-o-iodoaniline as the substrate, aziridine as the ring-opening C–H alkylating reagent, and norbornadiene (NBD) instead of norbornene (NBE) to attempt to achieve the synthesis of C4-ethylaminoindole. After carefully studying various reaction parameters, Pd(OAc)2 and triphenylphosphine were chosen as the Pd/ligand combination, cesium carbonate (Cs2CO3) was used as the base, and a mixture of toluene and dioxane served as the solvent. Under an argon atmosphere, the reaction mixture was stirred initially at 90 °C for 12 hours, followed by an increase in temperature to 150 °C and further stirring for 24 hours. This procedure resulted in a 58% isolated yield of the C4-ethylamino indole 3a, with no formation of the cyclized product 4a resulting from β-carbon elimination. It is worth mentioning that we found that heating to 150 °C in the later stage promoted the retro-Diels–Alder reaction to release cyclopentadiene. Next, deviation experiments from the standard conditions were explored. We first investigated the use of a single solvent instead of a mixed solvent. When toluene was used as the solvent, the yield of C4-ethylamino indole 3a decreased to 31%, and 8% of C7-aminoindoline 4a was detected. When dioxane was used as the solvent, the yield of 3a decreased to 40%. Notably, omitting CsBr as an additive also resulted in a yield of 48%. Subsequently, when we attempted to replace NBD with NBE, no target product 3a was detected, while the yield of indoline 4a increased to 16%. Potassium carbonate instead of cesium carbonate as the base reduced the yield of the indole 3a to 30%, with 12% indoline 4a formed. Increasing the amount of cesium carbonate from 1.0 equivalent to 2.0 equivalents decreased the yield of the target product to 43%. Additionally, directly stirring at 140 °C for 24 hours resulted in a yield of 32% for 3a, and formation of 18% of 4a was detected. Finally, we investigated various protecting groups on the nitrogen of o-iodoaniline, such as methyl, cyclohexyl, t-butoxycarbonyl (Boc), and benzoyl, but none yielded the target product (Table 1).
After achieving optimal conditions for generating C4-ethylamino indole, we aimed to direct the catalytic cycle towards β-carbon elimination to produce C7-aminoindoline 4a. After various conditional screenings, we found that when using p-chlorotriphenylphosphine as a ligand and 5-norbornene-2-carboxylate (N1) as a co-catalyst, indoline product 4a was obtained with a yield of 56%. After obtaining the optimal reaction conditions, simple control experiments were conducted. When triphenylphosphine was used instead of P(p-Cl-C6H4)3 as the ligand, the yield decreased to 40%. Similarly, replacing N1 with NBE led to a reduced yield of 42%. However, when NBD is used instead of N1, the yield decreases to 19%, which may be due to the inhibition of β-carbon elimination. Lastly, omitting KI as an additive still yielded the target product with a 39% yield (Table 2).
Table 2 Optimization of reaction conditions for C7-aminoindolinea

|
Entry |
Deviation from the standard conditions |
Yield (4a) |
Yield (3a) |
Standard conditions B: substrate 1a (0.2 mmol), 2a (0.5 mmol, 2.5 equiv.), Pd(OAc)2 (10 mmol%), P(p-Cl-C6H4)3 (20 mmol%), KI (0.5 equiv.), K2CO3 (0.6 mmol, 3.0 equiv.), N1 (0.2 mmol, 1.0 equiv.), toluene (2 mL), 100 °C, 24 h.
|
1 |
— |
56 |
— |
2 |
PPh3 instead of P(p-Cl-C6H4)3 |
40 |
— |
3 |
NBE instead of N1 |
42 |
— |
4 |
NBD instead of N1 |
19 |
Trace |
5 |
Without KI |
39 |
— |
After obtaining the optimal reaction conditions, we first studied the functional group tolerance of C4-ethylamine indole (Table 3). Both electron-donating groups (–Me, –OMe, tBu, and –Ph) and electron-withdrawing groups (–F and –Cl) were suitable for this method. Notably, 2-methylaziridine underwent a selective ring-opening reaction to form the corresponding C4-ethylaminoindole product 3k. More importantly, C4-ethylamino-aza-indole 3n was successfully synthesized via this strategy.
Table 3 Investigation of substrate scopea
Standard conditions A: substrate 1 (0.2 mmol), 2 (0.5 mmol, 2.5 equiv.), Pd(OAc)2 (10 mmol%), PPh3(25 mmol%), CsBr (1.0 equiv.), Cs2CO3 (1.0 equiv), NBD (0.6 mmol, 3.0 equiv.), toluene : dioxane (1 : 1, 2 mL) 90 °C 12 h, and then 150 °C, 24 h. Standard conditions B: substrate 1 (0.2 mmol), 2 (0.5 mmol, 2.5 equiv.), Pd(OAc)2 (10 mmol%), P(p-Cl-C6H4)3 (20 mmol%), KI (0.5 equiv.), K2CO3 (0.6 mmol, 3.0 equiv.), N1 (0.2 mmol, 1.0 equiv.), toluene (2 mL), 100 °C, 24 h.
|
|
Subsequently, we investigated the substrate scope of C7-aminoindoline. First, we studied the tolerance of functional groups on the indoline ring of the product. Both halogens (–F, –Cl, and –Br) and strong electron-withdrawing groups (–NO2 and –CO2Me) were suitable for the method, and the target products were obtained in 53–93% yield. It is noteworthy that the method can also achieve the biologically active C7-amino-aza-indoline scaffold (4g) with good yield. Specifically, 2-methylaziridine underwent a selective ring-opening reaction to produce the 2-methylindoline product 4h. Additionally, aziridines with different benzenesulfonyl protecting groups on the nitrogen atom can be used to synthesize the corresponding C7-aminoindoline derivatives with antitumor activity.
Finally, we found that the tert-butyl group on the nitrogen atom of indole can be easily removed in hydrochloric acid, while the p-toluenesulfonyl group can be removed under basic conditions. Since pharmaceutically active indole or indoline molecules often have exposed N–H bonds, this further enhances the application value of this synthetic method (Fig. 2). Additionally, the resulting C4-ethylaminoindole can be further converted into dopamine receptor agonists using established methods.24
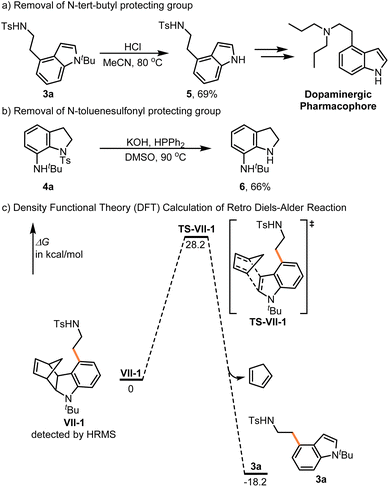 |
| Fig. 2 Removal of protecting groups and density functional theory (DFT) calculation. | |
Based on the above experimental results and our previous mechanistic studies,10d,14a we proposed a possible catalytic cycle (Fig. 3). First, o-iodoaniline 1a undergoes oxidative addition with the Pd(0) complex to form intermediate I. Subsequently, it undergoes migratory insertion with norbornadiene (NBD) or norbornene (N1), followed by C–H bond activation and cyclization in the presence of carbonate, resulting in the formation of the aryl-norbornene-palladacycle ANP intermediate II. Among them, the common byproduct II′, which is detected in low-yielding cases as shown in Table 3, is generated from the reductive elimination reaction of intermediate II. Then, the intermediate II undergoes a ring-opening oxidative addition process with aziridine to generate the Pd(IV) intermediate III, and the C–H alkylation intermediate IV is obtained through reductive elimination. It is worth mentioning that intermediate IV can follow two distinct pathways, leading to the selective formation of C4-ethylaminoindole (Pathway A) and C7-aminoindoline (Pathway B), respectively. In Pathway A, intermediate IV is attacked by an anion, resulting in the cleavage of the N–Pd bond to form intermediate V-1. Its σ bond rotates and undergoes deprotonation to coordinate with the nitrogen atom of aniline, forming intermediate VI-1. Finally, the five-membered ring intermediate VII-1 is obtained through reductive elimination, and it further forms C4-ethylaminoindole via a retro-Diels–Alder reaction. Density Functional Theory (DFT) calculations revealed that the retro-Diels–Alder reaction releases 18.2 kcal mol−1, indicating that the process is irreversible (Fig. 2c). In Pathway B, intermediate IV selectively undergoes a β-carbon elimination to extrude norbornene, followed by reductive elimination to yield C7-aminoindoline.
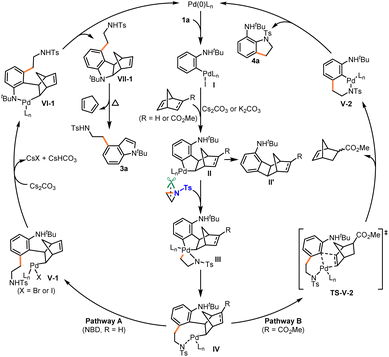 |
| Fig. 3 Proposed reaction mechanism. | |
Conclusions
In summary, we developed the first palladium-catalyzed regioselective synthesis of C4-ethylaminoindoles by utilizing the C–N bond ring-opening cleavage reaction of aziridine for the ortho-C–H ethylamination of iodobenzene. Subsequently, by controlling β-carbon elimination to extrude norbornene, we further achieved the synthesis of the C7-aminoindoles. In addition, the reaction also effectively inhibited the nucleophilic addition of the amine group of o-iodoaniline to aziridine, making a smooth Pd/NBE catalytic cycle possible. Moreover, the tert-butyl or p-toluenesulfonyl protecting groups on C4-ethylaminoindole and C7-aminoindole were easily removed, providing a novel synthetic route to dopamine receptor agonists.
Data availability
All data associated with this study are available in the article and ESI.†
Author contributions
Conceptualization, B.-S. Z. and Y.-M. L.; methodology, B.-S. Z. and B.-J. D.; investigation, B.-J. D., Y.-X. Z., T.-J. G., and Y.-M. W.; writing – original draft, B.-S. Z. and B.-J. D.; writing – review & editing, B.-S. Z., B.-J. D., X.-Y. G. and Y.-M.; L.; funding acquisition, B.-S. Z., X.-C. W., Z.-J. Q. and Y.-M. L.; resources, B.-S. Z., X.-C. W. and Z.-J. Q.; supervision, B.-S. Z., X.-C. W. and Z.-J. Q.
Conflicts of interest
There are no conflicts to declare.
Acknowledgements
Financial support was received from the National Natural Science Foundation of China: 22101232 (B.-S. Z.), 22067018 (X.-C. W.), 22061038 (Z.-J. Q.) and 22171114 (Y.-M. L.) and the Long Yuan Youth Innovative Project of Gansu Province (2019–39).
Notes and references
-
(a) R. D. Taylor, M. MacCoss and A. D. G. Lawson, Rings in Drugs, J. Med. Chem., 2014, 57, 5845–5859 CrossRef CAS PubMed;
(b) C. R. Jamison, J. J. Badillo, J. M. Lipshultz, R. J. Comito and D. W. C. MacMillan, Catalyst-controlled oligomerization for the collective synthesis of polypyrroloindoline natural products, Nat. Chem., 2017, 9, 1165–1169 CrossRef CAS PubMed;
(c) H. Wei, B. Li, N. Wang, Y. Ma, J. Yu, X. Wang, J. Su and D. Liu, Development and Application of Indolines in Pharmaceuticals, ChemistryOpen, 2023, 12, e202200235 CrossRef CAS PubMed.
-
(a) F. Tylš, T. Páleníček and J. Horáček, Psilocybin – Summary of knowledge and new perspectives, Eur. Neuropsychopharmacol., 2014, 24, 342–356 CrossRef PubMed;
(b) R. Tittarelli, G. Mannocchi, F. Pantano and S. F. Romolo, Recreational Use, Analysis and Toxicity of Tryptamines, Curr. Neuropharmacol., 2015, 13, 26–46 CrossRef CAS PubMed.
- A. Slominski, I. Semak, A. Pisarchik, T. Sweatman, A. Szczesniewski and J. Wortsman, Conversion of L-tryptophan to serotonin and melatonin in human melanoma cells, FEBS Lett., 2002, 511, 102–106 CrossRef CAS PubMed.
-
(a) L. F. Mohammad-Zadeh, L. Moses and S. M. Gwaltney-Brant, Serotonin: a review, J. Vet. Pharmacol. Ther., 2008, 31, 187–199 CrossRef CAS PubMed;
(b) F. Auld, E. L. Maschauer, I. Morrison, D. J. Skene and R. L. Riha, Evidence for the efficacy of melatonin in the treatment of primary adult sleep disorders, Sleep Med. Rev., 2017, 34, 10–22 CrossRef PubMed.
-
(a)
J. M. Cassady and J. A. Clemens, Use of 4-(2-di-n-propylaminoethyl)indole or a salt thereof as a presynaptic dopamine autoreceptor stimulant, US pat., 4378368A, 1981;
(b) H. Wikstroem, J. H. Lii and N. L. Allinger, The dopaminergic moiety of the ergots. A controversial topic studied with molecular mechanics, J. Med. Chem., 1987, 30, 1928–1934 CrossRef CAS PubMed;
(c) D. J. Tompson and D. Vearer, Steady-state pharmacokinetic properties of a 24-hour prolonged-release formulation of ropinirole: Results of two randomized studies in patients with Parkinson's disease, Clin. Ther., 2007, 29, 2654–2666 CrossRef CAS PubMed.
-
(a) S. Mehndiratta, Y.-F. Chiang, M.-J. Lai, H.-Y. Lee, M.-C. Chen, C.-C. Kuo, C.-Y. Chang, J.-Y. Chang and J.-P. Liou, Concise syntheses of 7-anilino-indoline-N-benzenesulfonamides as antimitotic and vascular disrupting agents, Bioorg. Med. Chem., 2014, 22, 4917–4923 CrossRef CAS PubMed;
(b) S.-Y. Wang, X. Liu, L.-W. Meng, M.-M. Li, Y.-R. Li, G.-X. Yu, J. Song, H.-Y. Zhang, P. Chen, S.-Y. Zhang and T. Hu, Discovery of indoline derivatives as anticancer agents via inhibition of tubulin polymerization, Bioorg. Med. Chem. Lett., 2021, 43, 128095 CrossRef CAS PubMed.
-
(a) M. Catellani, F. Frignani and A. Rangoni, A Complex Catalytic Cycle Leading to a Regioselective Synthesis of o,o'-Disubstituted Vinylarenes, Angew. Chem., Int. Ed. Engl., 1997, 36, 119–122 CrossRef CAS;
(b) D. Dupommier and T. Besset, New trends for transition metal-catalyzed ortho/ipso difunctionalizations of arenes, Chem, 2024 DOI:10.1016/j.chempr.2024.07.003.
-
(a) J. Ye and M. Lautens, Palladium-catalysed norbornene-mediated C–H functionalization of arenes, Nat. Chem., 2015, 7, 863–870 CrossRef CAS PubMed;
(b) N. Della Ca’, M. Fontana, E. Motti and M. Catellani, Pd/Norbornene: A Winning Combination for Selective Aromatic Functionalization via C–H Bond Activation, Acc. Chem. Res., 2016, 49, 1389–1400 CrossRef PubMed;
(c) H.-G. Cheng, S. Chen, R. Chen and Q. Zhou, Palladium(II)-Initiated
Catellani-Type Reactions, Angew. Chem., Int. Ed., 2019, 58, 5832–5844 CrossRef CAS PubMed;
(d) J. Wang and G. Dong, Palladium/Norbornene Cooperative Catalysis, Chem. Rev., 2019, 119, 7478–7528 CrossRef CAS PubMed;
(e) R. Li and G. Dong, Structurally Modified Norbornenes: A Key Factor to Modulate Reaction Selectivity in the Palladium/Norbornene Cooperative Catalysis, J. Am. Chem. Soc., 2020, 142, 17859–17875 CrossRef CAS PubMed;
(f) L.-Y. Liu, J. X. Qiao, K.-S. Yeung, W. R. Ewing and J.-Q. Yu, meta-Selective C–H Arylation of Fluoroarenes and Simple Arenes, Angew. Chem., Int. Ed., 2020, 59, 13831–13835 CrossRef CAS PubMed;
(g) H. Shi, Y. Lu, J. Weng, K. L. Bay, X. Chen, K. Tanaka, P. Verma, K. N. Houk and J.-Q. Yu, Differentiation and functionalization of remote C–H bonds in adjacent positions, Nat. Chem., 2020, 12, 399–404 CrossRef CAS PubMed;
(h) L. Guillemard, N. Kaplaneris, L. Ackermann and M. J. Johansson, Late-stage C–H functionalization offers new opportunities in drug discovery, Nat. Rev. Chem, 2021, 5, 522–545 CrossRef CAS PubMed;
(i) H.-G. Cheng, S. Jia and Q. Zhou, Benzo-Fused-Ring Toolbox Based on Palladium/Norbornene Cooperative Catalysis: Methodology Development and Applications in Natural Product Synthesis, Acc. Chem. Res., 2023, 56, 573–591 CrossRef CAS PubMed.
-
(a) M. Lautens and S. Piguel, A New Route to Fused Aromatic Compounds by Using a Palladium-Catalyzed Alkylation– Alkenylation Sequence, Angew. Chem., Int. Ed., 2000, 39, 1045–1046 CrossRef CAS;
(b) B. Mariampillai, J. Alliot, M. Li and M. Lautens, A Convergent Synthesis of Polysubstituted Aromatic Nitriles via Palladium-Catalyzed C–H Functionalization, J. Am. Chem. Soc., 2007, 129, 15372–15379 CrossRef CAS PubMed;
(c) A. Rudolph, N. Rackelmann and M. Lautens, Stereochemical and Mechanistic Investigations of a Palladium-Catalyzed Annulation of Secondary Alkyl Iodides, Angew. Chem., Int. Ed., 2007, 46, 1485–1488 CrossRef CAS PubMed;
(d) D. A. Candito and M. Lautens, Palladium-Catalyzed Domino Direct Arylation/N-Arylation: Convenient Synthesis of Phenanthridines, Angew. Chem., Int. Ed., 2009, 48, 6713–6716 CrossRef CAS PubMed;
(e) H. Weinstabl, M. Suhartono, Z. Qureshi and M. Lautens, Total Synthesis of (+)-Linoxepin by Utilizing the Catellani Reaction, Angew. Chem., Int. Ed., 2013, 52, 5305–5308 CrossRef CAS PubMed;
(f) A. Whyte, M. E. Olson and M. Lautens, Palladium-Catalyzed, Norbornene-Mediated, ortho-Amination ipso-Amidation: Sequential C–N Bond Formation, Org. Lett., 2018, 20, 345–348 CrossRef CAS PubMed.
-
(a) P. Zhao, Y. Guo and X. Luan, Total Synthesis of Dalesconol A by Pd(0)/Norbornene-Catalyzed Three-Fold Domino Reaction and Pd(II)-Catalyzed Trihydroxylation, J. Am. Chem. Soc., 2021, 143, 21270–21274 CrossRef CAS PubMed;
(b) M. Bai, S. Jia, J. Zhang, H.-G. Cheng, H. Cong, S. Liu, Z. Huang, Y. Huang, X. Chen and Q. Zhou, A Modular Approach for Diversity-Oriented Synthesis of 1,3-trans-Disubstituted Tetrahydroisoquinolines: Seven-Step Asymmetric Synthesis of Michellamines B and C, Angew. Chem., Int. Ed., 2022, 61, e202205245 CrossRef CAS PubMed;
(c) B.-S. Zhang, Y.-X. Yang, J. C. A. Oliveira, Z.-Q. Zhang, S. Warratz, Y.-M. Wang, S.-X. Li, X.-C. Wang, X.-Y. Gou, Y.-M. Liang, Z.-J. Quan and L. Ackermann, Combined C-H amination and intermolecular alkyne insertion for a three-component cyclization, Cell Rep. Phys. Sci., 2023, 101647 CrossRef CAS;
(d) B.-S. Zhang, Y. Li, Z. Zhang, Y. An, Y.-H. Wen, X.-Y. Gou, S.-Q. Quan, X.-G. Wang and Y.-M. Liang, Synthesis of C4-Aminated Indoles via a Catellani and Retro-Diels–Alder Strategy, J. Am. Chem. Soc., 2019, 141, 9731–9738 CrossRef CAS PubMed.
- P. Thansandote, D. G. Hulcoop, M. Langer and M. Lautens, Palladium-Catalyzed Annulation of Haloanilines and Halobenzamides Using Norbornadiene as an Acetylene Synthon: A Route to Functionalized Indolines, Isoquinolinones, and Indoles, J. Org. Chem., 2009, 74, 1673–1678 CrossRef CAS PubMed.
- W. C. Fu, Z. Wang, W. T. K. Chan, Z. Lin and F. Y. Kwong, Regioselective Synthesis of Polycyclic and Heptagon-embedded Aromatic Compounds through a Versatile π-Extension of Aryl Halides, Angew. Chem., Int. Ed., 2017, 56, 7166–7170 CrossRef CAS PubMed.
-
(a) W. Lv, S. Wen, J. Yu and G. Cheng, Palladium-Catalyzed Ortho-Silylation of Aryl Iodides with Concomitant Arylsilylation of Oxanorbornadiene: Accessing Functionalized (Z)-β-Substituted Vinylsilanes and Their Analogues, Org. Lett., 2018, 20, 4984–4987 CrossRef CAS PubMed;
(b) W. Lv, J. Yu, B. Ge, S. Wen and G. Cheng, Palladium-Catalyzed Catellani-Type Bis-silylation and Bis-germanylation of Aryl Iodides and Norbornenes, J. Org. Chem., 2018, 83, 12683–12693 CrossRef CAS PubMed;
(c) Q. Tian, J. Ge, Y. Liu, X. Wu, Z. Li and G. Cheng, Solvent-Controlled Enantiodivergent Construction of P(V)-Stereogenic Molecules via Palladium-Catalyzed Annulation of Prochiral N-Aryl Phosphonamides with Aromatic Iodides, Angew. Chem., Int. Ed., 2024, e202409366 Search PubMed;
(d) Y. Zhang, Y. Chen, Q. Tian, B. Wang and G. Cheng, Palladium-Catalyzed Multicomponent Assembly of (Z)-Alkenylborons via Carbopalladation/Boronation/Retro-Diels–Alder Cascade Reaction, J. Org. Chem., 2023, 88, 11793–11800 CrossRef CAS PubMed.
-
(a) B.-S. Zhang, Z.-Q. Zhang, T.-J. Guo, J. C. A. Oliveira, S. Warratz, B.-J. Deng, Y.-M. Wang, J.-S. Zhou, X.-Y. Gou, X.-C. Wang, Z.-J. Quan and L. Ackermann, Direct Synthesis of C4-Acyl Indoles via C–H Acylation, Org. Lett., 2024, 26, 4998–5003 CrossRef CAS PubMed;
(b) Y. An, B.-S. Zhang, Y.-N. Ding, Z. Zhang, X.-Y. Gou, X.-S. Li, X. Wang, Y. Li and Y.-M. Liang, Palladium-catalyzed C–H glycosylation and retro Diels–Alder tandem reaction via structurally modified norbornadienes (smNBDs), Chem. Sci., 2021, 12, 13144–13150 RSC.
-
(a) D. A. Candito and M. Lautens, Exploiting the Chemistry of Strained Rings: Synthesis of Indoles via Domino Reaction of Aryl Iodides with 2H-Azirines, Org. Lett., 2010, 12, 3312–3315 CrossRef CAS PubMed;
(b) P. Thansandote, M. Raemy, A. Rudolph and M. Lautens, Synthesis of Benzannulated N-Heterocycles by a Palladium-Catalyzed C–C/C–N Coupling of Bromoalkylamines, Org. Lett., 2007, 9, 5255–5258 CrossRef CAS PubMed.
-
(a) R. Li, F. Liu and G. Dong, Palladium-catalyzed asymmetric annulation between aryl iodides and racemic epoxides using a chiral norbornene cocatalyst, Org. Chem. Front., 2018, 5, 3108–3112 RSC;
(b) R. Li and G. Dong, Direct Annulation between Aryl Iodides and Epoxides through Palladium/Norbornene Cooperative Catalysis, Angew. Chem., Int. Ed., 2018, 57, 1697–1701 CrossRef CAS PubMed;
(c) Z. Wang, Y. Kuninobu and M. Kanai, Palladium-Catalyzed Oxirane-Opening Reaction with Arenes via C–H Bond Activation, J. Am. Chem. Soc., 2015, 137, 6140–6143 CrossRef CAS PubMed;
(d) G. Cheng, T.-J. Li and J.-Q. Yu, Practical Pd(II)-Catalyzed C–H Alkylation with Epoxides: One-Step Syntheses of 3,4-Dihydroisocoumarins, J. Am. Chem. Soc., 2015, 137, 10950–10953 CrossRef CAS PubMed.
-
(a) C. Wu, H.-G. Cheng, R. Chen, H. Chen, Z.-S. Liu, J. Zhang, Y. Zhang, Y. Zhu, Z. Geng and Q. Zhou, Convergent syntheses of 2,3-dihydrobenzofurans via a Catellani strategy, Org. Chem. Front., 2018, 5, 2533–2536 RSC;
(b) G. Qian, M. Bai, S. Gao, H. Chen, S. Zhou, H.-G. Cheng, W. Yan and Q. Zhou, Modular One-Step Three-Component Synthesis of Tetrahydroisoquinolines
Using a Catellani Strategy, Angew. Chem., Int. Ed., 2018, 57, 10980–10984 CrossRef CAS PubMed;
(c) H.-G. Cheng, C. Wu, H. Chen, R. Chen, G. Qian, Z. Geng, Q. Wei, Y. Xia, J. Zhang, Y. Zhang and Q. Zhou, Epoxides as Alkylating Reagents for the Catellani Reaction, Angew. Chem., Int. Ed., 2018, 57, 3444–3448 CrossRef CAS PubMed.
- C. Liu, Y. Liang, N. Zheng, B.-S. Zhang, Y. Feng, S. Bi and Y.-M. Liang, Synthesis of indolines via a palladium/norbornene-catalyzed reaction of aziridines with aryl iodides, Chem. Commun., 2018, 54, 3407–3410 RSC.
-
(a) Z. Wang, Y.-T. Cui, Z.-B. Xu and J. Qu, Hot Water-Promoted Ring-Opening of Epoxides and Aziridines by Water and Other Nucleopliles, J. Org. Chem., 2008, 73, 2270–2274 CrossRef CAS PubMed;
(b) M. Zhu and B. Moasser, Aqueous ring opening of N-tosylaziridine with aniline derivatives, Tetrahedron Lett., 2012, 53, 2288–2291 CrossRef CAS;
(c) S. Sabir, G. Kumar, V. P. Verma and J. L. Jat, Aziridine Ring Opening: An Overview of Sustainable Methods, ChemistrySelect, 2018, 3, 3702–3711 CrossRef CAS.
-
(a) M. Catellani and E. Motti, Selective aryl coupling via palladacycles: a new route to m-alkylbiphenyls or m-terphenyls, New J. Chem., 1998, 22, 759–761 RSC;
(b) Z. Dong, G. Lu, J. Wang, P. Liu and G. Dong, Modular ipso/ortho Difunctionalization of Aryl Bromides via Palladium/Norbornene Cooperative Catalysis, J. Am. Chem. Soc., 2018, 140, 8551–8562 CrossRef CAS PubMed;
(c) J. Wang, R. Li, Z. Dong, P. Liu and G. Dong, Complementary site-selectivity in arene functionalization enabled by overcoming the ortho constraint in palladium/norbornene catalysis, Nat. Chem., 2018, 10, 866–872 CrossRef CAS PubMed;
(d) H. Shi, A. N. Herron, Y. Shao, Q. Shao and J.-Q. Yu, Enantioselective remote meta-C–H arylation and alkylation via a chiral transient mediator, Nature, 2018, 558, 581–585 CrossRef CAS PubMed;
(e) K.-J. Xiao, L. Chu, G. Chen and J.-Q. Yu, Kinetic Resolution of Benzylamines via Palladium(II)-Catalyzed C–H Cross-Coupling, J. Am. Chem. Soc., 2016, 138, 7796–7800 CrossRef CAS PubMed.
- J. Wang, Z. Dong, C. Yang and G. Dong, Modular and regioselective synthesis of all-carbon tetrasubstituted olefins enabled by an alkenyl Catellani reaction, Nat. Chem., 2019, 11, 1106–1112 CrossRef CAS PubMed.
- X. Liu, Q. Zhu and G. Dong, Beyond Tertiary Amines: Introducing Secondary Amines by Palladium/Norbornene-Catalyzed Ortho Amination, Angew. Chem., Int. Ed., 2024, 63, e202404042 CrossRef CAS PubMed.
-
(a) Y.-X. Zheng and L. Jiao, Hybrid cycloolefin ligands for palladium-olefin cooperative catalysis, Nat. Synth., 2022, 1, 180–187 CrossRef;
(b) F.-Y. Wang, Y.-X. Li and L. Jiao, Functionalized Cycloolefin Ligand as a Solution to Ortho-Constraint in the Catellani-Type Reaction, J. Am. Chem. Soc., 2023, 145, 4871–4881 CrossRef CAS PubMed.
-
(a) T. Ankner and G. Hilmersson, Instantaneous Deprotection of Tosylamides and Esters with SmI2/Amine/Water, Org. Lett., 2009, 11, 503–506 CrossRef CAS PubMed;
(b) X. Jiang, C. Zheng, L. Lei, K. Lin and C. Yu, Synthesis of 2-Oxindoles from Substituted Indoles by Hypervalent-Iodine Oxidation, Eur. J. Org Chem., 2018, 2018, 1437–1442 CrossRef CAS.
|
This journal is © The Royal Society of Chemistry 2024 |
Click here to see how this site uses Cookies. View our privacy policy here.