DOI:
10.1039/D3TB02752J
(Review Article)
J. Mater. Chem. B, 2024,
12, 2236-2252
Plant-derived exosomes: a green approach for cancer drug delivery
Received
18th November 2023
, Accepted 1st February 2024
First published on 14th February 2024
Abstract
Plant-derived exosomes (PDEs) are natural extracellular vesicles (EVs). In the current decade, they have been highlighted for cancer therapeutic development. Cancer is a global health crisis and it requires an effective, affordable, and less side effect-based treatment. Emerging research based on PDEs suggests that they have immense potential to be considered as a therapeutic option. Research evidences indicate that PDEs’ internal molecular cargos show impressive cancer prevention activity with less toxicity. PDEs-based drug delivery systems overcome several limitations of traditional drug delivery tools. Extraction of PDEs from plant sources employ diverse methodologies, encompassing ultracentrifugation, immunoaffinity, size-based isolation, and precipitation, each with distinct advantages and limitations. The core constituents of PDEs comprise of lipids, proteins, DNA, and RNA. Worldwide, a few clinical trials on plant-derived exosomes are underway, and regulatory affairs for their use as therapeutic agents are still not understood with clarity. This review aims to comprehensively analyze the current state of research on plant-derived exosomes as a promising avenue for drug delivery, highlighting anticancer activity, challenges, and future orientation in effective cancer therapeutic development.
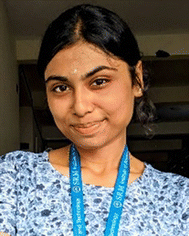
Shrishti Madhan
| Shrishti Madhan is a third year undergraduate student of SRM Institute of Science and Technology, Kattankulathur, Chengalpattu District-603 203, Tamil Nadu, India, pursuing her B. Tech in Biotechnology with specialization in Genetic Engineering. Under the guidance of Dr Arikketh Devi, she is currently working on the therapeutic impact of phytochemicals in cancer. Shrishti's main area of interest includes cancer therapeutics with a focus on exosomes. |
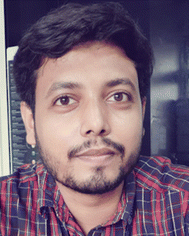
Rajib Dhar
| Rajib Dhar is a PhD Research Scholar at SRM Institute of Science and Technology, Kattankulathur, Chengalpattu District-603 203, Tamil Nadu, India. Under the guidance of Dr. Arikketh Devi, his research focus on the development of exosomes-based therapeutic approach against cancer. Rajib is actively involved in publishing articles on exosomes biology and his main interest includes exosomes and cancer. |
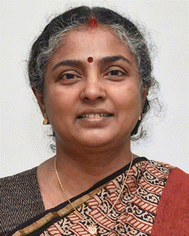
Dr. Arikketh Devi
| Dr. Arikketh Devi is an Associate Professor at SRM Institute of Science and Technology, Kattankulathur, Chengalpattu District-603 203, Tamil Nadu, India. Her team is actively involved in identifying therapeutic targets for Oral and Cervical cancers. Cancer Biology, Stem Cell Biology, and Lipid Biology are the areas of research interest currently pursued in the lab. |
1. Introduction
Cancer is the second death-causing health crisis globally each year. Its intricate biology, heterogeneity, and adaptability have rendered it one of the most formidable diseases confronting modern medicine.1,2 According to the World Cancer Report 2023, an estimated 1
958
310 new cancer cases were diagnosed worldwide in 2023, with approximately 609
820 cancer-related deaths projected in the United States of America.3 Despite significant progress, its incidence continues to rise and it remains a leading cause of morbidity and mortality worldwide.4 These staggering statistics underscore the urgent need for ongoing research and innovation in the field of oncology. This has ultimately propelled researchers to investigate extracellular vesicles. Within these lipid bilayer-encased membranous entities lie a spectrum of minute structures, comprising apoptotic bodies, microvesicles, and exosomes (subpopulation of EVs).5 Exosomes are intricately linked to cancer development and progression, with their involvement spanning a multitude of critical events.6 Tumor-derived exosomes (TEXs)7 regulate several events such as cancer cellular proliferation, immune cell reprogramming,8 angiogenesis,9 and extracellular matrix remodeling. Furthermore, they play pivotal roles in metastasis by modulating the epithelial–mesenchymal transition (EMT)10via miRNA regulation, orchestrating organ-specific metastatic11 processes through surface integrin interactions, and conferring resistance to drugs and therapeutic interventions.12,13 Additionally, they contribute to the development of cancer stem cells,14 thus intensifying their significance within the tumor microenvironment. Exosomes are found in several sources such as blood plasma, serum, saliva, CSF, etc.,15,16 and they also exhibit cancer-healing properties.17 In general, the cancer therapeutic potential of exosomes (Fig. 1) is based on their sources, such as stem cells exosomes, plant exosomes, chimeric exosomes, modified exosomes and tumor-derived exosomes (though due to their oncogenic cargos, this source still has been questioned for its role in therapeutics).
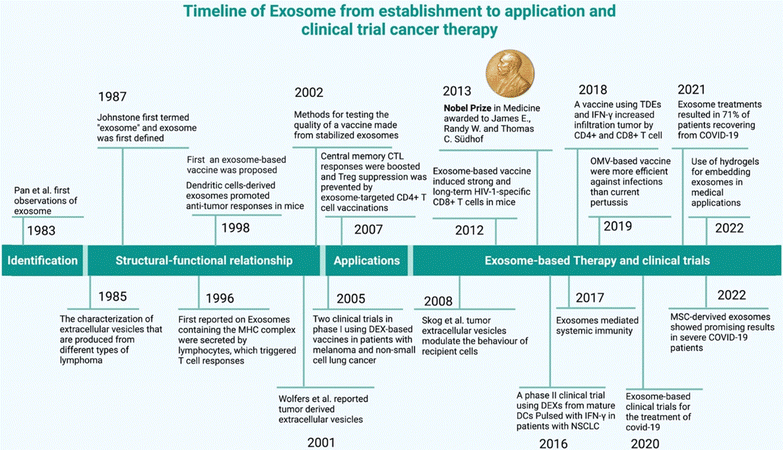 |
| Fig. 1 Exosome-based cancer therapy (reproduced with permission under Creative Commons CC BY 4.0 license from ref. 18. Copyright 2022 The Authors). | |
Exosomes are chosen as a drug delivery tool due to many reasons (Fig. 2). Exosomes have become a promising drug transporter due to their unique features, such as biocompatibility, low immunogenicity, low toxicity (plant exosomes toxicity is lower than that of stem cell-derived exosomes and chemically or genetically modified or hybrid exosomes), stability, biological membrane cross capability, and are target-specific. Plant-derived exosomes (PDEs) are key players in this changing landscape.19 In PDEs research, the most challenging step is PDEs isolation from a wide range of fruits, vegetables and other plant parts, involves strategic methods to control exosomes heterogeneity and to identify methods to detect effective bioactive components.20,21 Though the environmental effects of PDEs are still under review, the potential therapeutic applications of plant-derived exosomes in areas like cancer therapy, immunomodulation, and regenerative medicine are indeed exciting. These natural nanocarriers offer a novel approach to drug delivery which may mitigate some of the limitations associated with traditional drug delivery systems.22
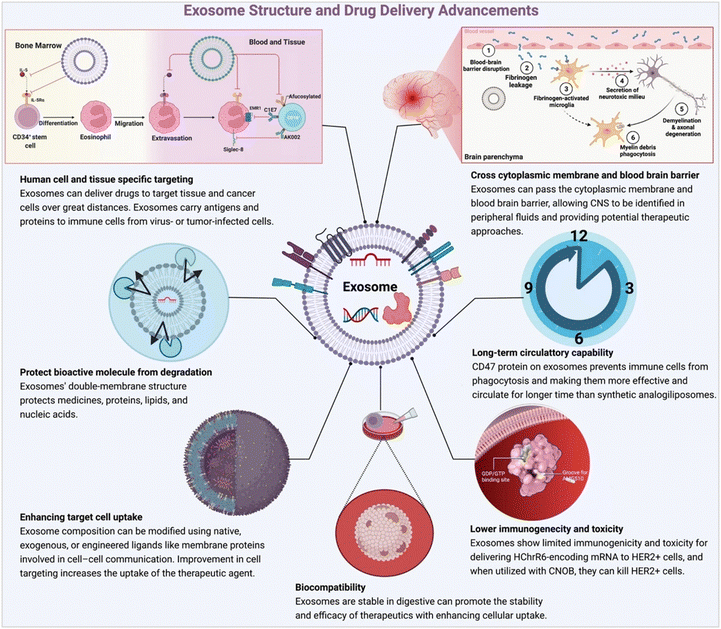 |
| Fig. 2 Advantage of exosome-based drug delivery (reproduced with permission under Creative Commons CC BY 4.0 license from ref. 18. Copyright 2022 The Authors). | |
2. Biogenesis
Exosomes are endosomal-derived membrane-bound EVs that are responsible for carrying a complex cargo of proteins,23 lipids,24 and nucleic acids.25,26 The inward budding of the multivesicular body membrane, which eventually results in the formation of an exosome, starts with its invagination to form an intraluminal vesicle (ILV).27 The budding is known to happen in a reverse orientation manner.28 The cytoskeletal network is responsible for the transportation of the multivesicular bodies (MVBs) to the plasma membrane before they fuse and get secreted as exosomes.29 Other MVBs fuse with lysosomes- directly or indirectly, to follow a degradation pathway.30 Exosomes biogenesis (Fig. 3) occurs either through endosomal sorting complex required for transport (ESCRT)-dependent or ESCRT-independent pathways. The former involves an intricate protein machinery encompassing four unique proteins that collectively work in MVB fusion with the plasma membrane, vesicle budding, and cargo sorting and are called ESCRT – endosomal sorting complex required for transport (0 through III).31–34 Certain ubiquitinated proteins bind to the endosome membrane via the ubiquitin-binding domains provided by ESCRT-0. This moves forward combining with ESCRT-I and ESRT-II to combine with ESCRT-III, a protein complex that promotes budding. Once the bud cleaves to form the ILV, the ESCRT-III establishes separation with the energy supplied by another sorting protein Vps4.35,36 Although the involvement of the ESCRT complex in exosome release is highly debated, the discovery of the exosomal protein Alix cemented the involvement of ESCRT.37 The alternative pathway depends on raft-based microdomains highly enriched in sphingomyelinase. They are responsible for the production of ceramide through the removal of a phosphocholine moiety.38,39 Ceramides have a cone-shaped structure40 which aids in the spontaneous induction of negative curvature in the endosomal membrane, thereby promoting budding. They are also involved in lateral phase separation.41 Apart from lipid involvement, proteins such as tetraspanin enriched microdomains (TEMs)42 are also involved and they assist in compartmentalization and signaling. Furthermore, along with tetraspanin, CD8143 helps in bioactive cargo and receptor sorting. The choice of pathway thus depends on the origin and type of cell. In cancer, exosome biogenesis depends on ESCRT-independent pathways and the tumor microenvironment pH.
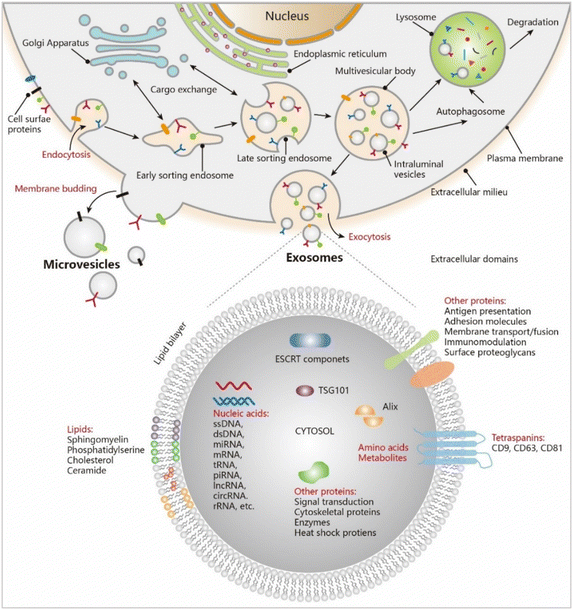 |
| Fig. 3 Exosome biogenesis. (Reproduced with permission from ref. 44 Copyright @ 2021 American Chemical Society.) | |
3. Plant exosomes sources
Exosomes are nanoscale extracellular vesicles that have emerged from diverse cellular origins, including mammals, plants, and bacteria.45 Remarkably, MVBs, the precursors of exosomes, were identified in plant cells as early as 1967, preceding their discovery in mammalian cells.46 In the botanical realm, PDEs assume pivotal roles including intercellular communication,47,48 fundamental immune responses, and adaptive strategies during environmental stresses.49,50 Blueberries and oranges are known to produce two distinct classes of exosomes characterized by spherical or oval shapes; however, the precise cellular or disease models they engage with, remains undefined.51 In contrast, carrots yield exosomes of diverse sizes, ranging from a modest 80 to an impressive 1500 nanometres, bearing distinctive cup-shaped or spherical morphologies. These find their niche in promoting intestinal homeostasis, accessible through oral administration.52 Coconuts, an emblem of tropical abundance, contribute spherical EVs derived from both milk and water; however, their intended utilization or associated models remain shrouded in ambiguity.53 Watermelons, sunflower seeds,54 pears, soybeans, and tomatoes exhibit the production of two types of spherical or oval EVs, yet the specifics of their applications await further elucidation. Arabidopsis leaves have spherical EVs spanning a diminutive 50 to a substantial 300 nanometres,55 while ginger bestows spherical or cup-shaped EVs with dimensions ranging from 100 to a significant 1000 nanometres.56 Ginger's multifaceted exosomes contribute significantly to inhibiting colon cancer cell proliferation and angiogenesis.57 They also serve as promising candidates in the realm of liver diseases, and has been advocated for oral administration.58 Grape-derived exosomes have also been successfully isolated and studied regarding their therapeutic abilities.59 Grapefruits, renowned for their zesty flavors, exhibit cup-shaped or spherical EVs, varying from 50 to 800 nanometres in size, the PDEs showing effectiveness against liver cancer.60,61 Citrus fruit-derived PDEs stimulate the activation of the Bad and Bax genes while simultaneously suppressing the influence of Survivin and Bcl-xL in tumor cells. The resultant outcome is a harmonious symphony of TRAIL-induced cell death and the abatement of tumorous growth.62 Lemon-derived exosomes inhibit cancer cell proliferation. Even Asparagus exhibits a modicum of anti-tumor capabilities.63 This rich tapestry of plant-derived exosomes presents an enticing vista for the transformation of medical treatments. From the precision of drug delivery to the staunch guardianship against the relentless tide of chronic diseases, these hold promise in reshaping the very landscape of medicine itself.64
4. Molecular signature
Plant-derived extracellular vesicles (P-EVs) manifest as reservoirs of an exceptionally diverse and multifaceted biomolecular ensemble, originating from diverse botanical sources.65 The molecular signature of an exosome is highly dependent on its source and the condition under which it is secreted.66 Within this intricate cargo, an array of proteins govern pivotal biological processes, encompassing responses to environmental and pathogenic stresses, intricate metabolic signaling cascades, the orchestration of cell-wall dynamics, the establishment of cytoskeletal frameworks, the facilitation of cellular transport, and the intricate machinery underlying secretory pathways.67,68 Annexins are proteins that have discerned plant exosomes harvested from diverse sources such as citrus juices, apoplastic fluids, sunflower seeds, and arabidopsis leaves.55 Their existence signifies a nexus with plant responses to the rigors of both biotic and abiotic stresses. Heat shock proteins (HSPs) which are involved in similar roles have also been identified.69 A spectrum of HSPs (including HSP60, 70, 80, and 90) have been documented within exosomes derived from citrus fruits, sunflowers, olives, grapefruits, grapes, and tomatoes.70 Exosomes from botanical sources such as citrus, grape, broccoli, and arabidopsis have revealed the presence of aquaporins.71 These membrane proteins contribute substantially to the stability of plasma membranes and the facilitation of water permeability. Pollen-derived P-EVs have been found to contain pectin methyl esterase, a pivotal participant in the expansive phenomenon of cell-wall growth and modification.72 The presence of actins hints at a spectrum of dynamic cellular processes possibly being orchestrated within the exosomes, encompassing cell division, cellular expansion, organelle mobility, and vesicular trafficking. Various members of the patellin family (patellin 1, 2, and 3) have surfaced in the repertoire of proteins retrieved from arabidopsis and citrus.73 They seem instrumental in membrane-trafficking events and in facilitating the transfer of hydrophobic molecules. Syntaxins have also been identified in exosomes from arabidopsis and citrus.74 The family of clathrin heavy chain proteins, including clathrin 1 and 2, has been identified in exosomes from arabidopsis, citrus, and sunflower. The lipidic profile of P-EVs deviates distinctively from that of EVs procured from animal cells. Notably, phosphatidic acid (PA),75 phosphatidylethanolamine (PE), and phosphatidylcholine (PC) have emerged as conspicuous lipid constituents within P-EVs.76,77 Sphingolipids were also identified in PDEs.78,79 These lipids exert a profound influence over membrane curvature, vesicle formation, membrane-mediated cellular signalling, and interactions with animal cells, with intriguing potential for modulating intestinal microbiota.80 In addition to their protein and lipidic constituents, P-EVs are generously laden with an assorted compendium of metabolites.81 Among these conventional metabolites, naringenin has been documented for its antineoplastic properties.82 P-EVs obtained from strawberries exhibit a notable abundance of ascorbic acid.83 Apple-derived EVs contain a repertoire of flavonoids and furanocoumarins recognized for their fungicidal and insecticidal attributes.84 Ginger and turmeric PDEs are potential antioxidant agents. Despite challenges in miRNA identification due to incomplete plant-specific sequences, P-EVs show potential for modulating gene expression in specific pathological conditions.85 However, PDEs requires more exploration of molecular-level proofing of their inner cargos for better therapeutic development.86
5. Plant exosomes isolation
Exosomes isolation has been carried out by several methods and each one has its own advantages and disadvantages (Table 1). Nanoplatforms based PDEs offer a wide array of advantages, including biocompatibility, stability, biodistribution, extended half-life, and efficient cellular internalization. PDE characterization has been described in Fig. 4.
Table 1 Exosomes isolation methods
Isolation methods |
Principle |
Advantages |
Disadvantages |
Ref. |
Ultracentrifugation |
This technique harnesses the force of high-speed centrifugation to differentially segregate particles based on their density, size, and morphological attributes. Starting with the crucial step of initial sample purification, it effectively removes larger cellular undesirable debris from the sample. Subsequent refinement, often facilitated by the employment of gradients incorporating sucrose or iodixanol, significantly increases the purity of isolated exosomes. |
Simplicity, affordability, moderate time consumption, minimal sample preparation. |
Costly to purchase and maintain equipment, requires extensive training and a dedicated team, contamination with similar-sized particles, generation of exosomal aggregates. |
87–89
|
|
Size-based isolation |
Size-based isolation techniques, exemplified by ultrafiltration, pivots on the principle of segregating exosomes based on their size or molecular weight. Size-exclusion chromatography (SEC) emerges as a compelling method of choice due to heightened exosomes purity. Flow field-flow fractionation manifests as a cutting-edge method in which particles undergo discrimination based on their hydrodynamic diameter. It is chosen to maintain the structural integrity and bioactivity of the exosomes. |
High purity, intact exosomes, efficient, relatively fast, avoids aggregation problems. |
Scalability and equipment accessibility |
89–94
|
|
Immunoaffinity |
Immunoaffinity isolation leverages antigen–antibody interactions within exosomes membranes for high specificity. It involves coating magnetic beads with antibodies targeting specific exosomes proteins and incubating them with the samples. However, its potential hasn't been extensively explored for plant-derived exosomes due to our limited surface composition knowledge. |
High specificity, improved purity, suitable for small sample size, can target specific proteins within exosomes. |
Reduced exosomes yield, potential damage to exosomes, costly due to antibody usage, may not capture all exosomes subpopulations. |
95–97
|
|
Precipitation |
This method employs an aqueous PEG solution to envelop exosomes, fostering the creation of exosomes conglomerates subsequently amenable to precipitation via low-speed centrifugation at 1500 g. It can co-precipitate non-exosomes impurities, such as vesicles and aggregates, posing purity challenges. By adjusting the PEG6000 concentration, different-sized nanoparticles can be obtained. Furthermore, commercial kits, like ExoQuick™, based on precipitation methods are also available for easier use. This approach overcomes the expense of ultracentrifugation but requires careful optimization to avoid impurities. |
High yield, fast, cost-effective, adjustable particle size based isolation. |
Co-precipitates non-exosomes impurities, lower yield compared to ultracentrifugation, potential scaling issues, further -omics research is hindered. |
98,99
|
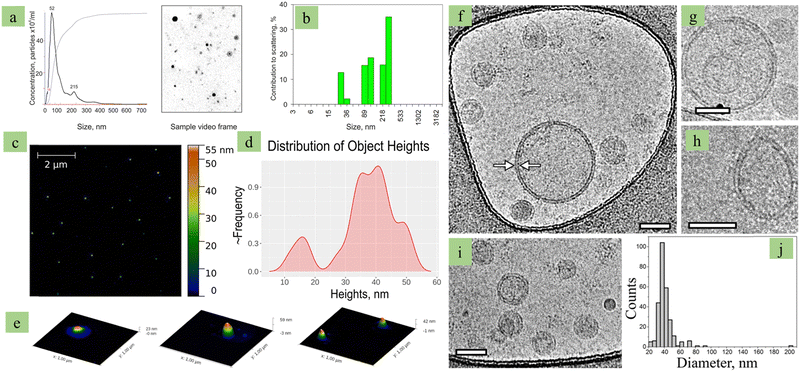 |
| Fig. 4 Plant exosome characterization. (a) Grape-derived exosomes NTA (nanoparticle tracking assay) based size and concentration analysis, (b) grape-derived exosomes DLS based size analysis, (c) AFM image of grape-derived exosomes, (d) height discussion of grape-derived exosomes, (e) 3D image of grape-derived exosomes, (f) Cry-TEM image of grape-derived exosomes (f–i), and (j) size analysis by histogram. (Reproduced with permission under Creative Commons CC BY 4.0 license from ref. 100 Copyright 2021 The Authors.) | |
The ultimate goal of refining isolation techniques is to engineer Plant Exosome-like Nanovesicles (PELNVs) based nanostructures that can yield optimal clinical outcomes for treating various ailments. Currently, two types of nanoplatforms are employed for therapeutic and drug delivery purposes: one employs PELNVs in their pristine form, while the other utilizes nano vectors derived from PELNVs. However, it is worth noting that the existing isolation methods for obtaining pure PELNVs are time-intensive and necessitate refinement to facilitate the commercial and therapeutic application of PELNVs. Therefore, there is an imperative need for the standardization of both qualitative and quantitative analyses to foster improved comparative interpretations of research outcomes. The fundamental concepts of plant exosomes isolation and characterization are summarized in Fig. 5.
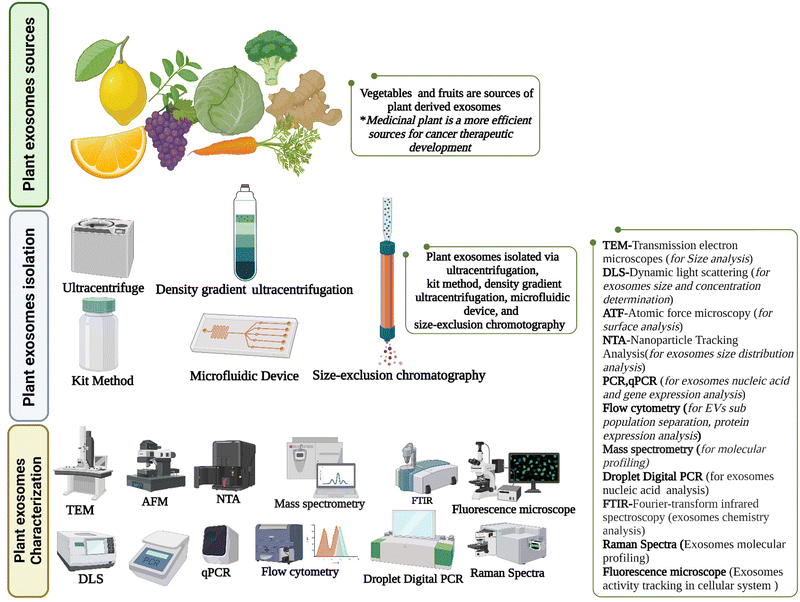 |
| Fig. 5 Plant exosomes isolation and characterization (created with https://biorender.com). | |
6. Exosomes and cancer
Cancer is a multifaceted group of diseases characterized by uncontrolled cellular proliferation, genetic instability, and potential to metastasize, posing a formidable challenge to both diagnosis and treatment.101 Scientific investigations found that in the tumor microenvironment (TME), tumor cells release TEXs to regulate cancer development and progression102–104 (Fig. 6). Also, immune cells derived exosomes are associated with complex cellular activation processes.105 Cancer-associated fibroblasts (CAFs) are major components of solid cancers and exosomes derived from CAFs affect cellular metabolism, epithelial–mesenchymal transition (EMT), drug resistance, and metastasis through the transfer of miRNA-21, miRNA-378, and miRNA-143.106 In colon cancer, fibroblast-derived exosomes develop into more aggressive cancer and chemotherapy resistance.107 Breast cancer metastasis is regulated via CAF-derived exosomes (they carry high expression of TGF-β and noncoding RNA).108,109 In addition, cancer initiating cells, cancer stem cells (CSCs) are a subgroup of tumour cells that possess the capacity for both self-renewal and differentiation.110 Exosomes have a role in both preserving CSCs homeostasis and controlling the transition between non-CSCs and CSCs. They mediate this through the transfer of specific RNAs such as lncRNA FMR1-AS1 – which activates the TLR7-NFκB signaling pathway, and miR-19b-3p.108 TEXs also have the capability to influence CSCs by targeting specific signaling pathways crucial for their functions. The exciting evidence is that p120-catenin-containing tumor exosomes show anticancer activity.111 In the TME, exosomes alter immune cell's function112 and in cancer, TEXs reprogram immune cells and promote cancer development. TEXs suppress the T (CD8+) cell's activity via exosomal FasL.113 Hypoxic TEX miRNA-103 promotes M2 polarization in colon cancer114 and exosomes promote epithelial–mesenchymal transition (EMT) characteristics.115,116 In colon cancer, exosomes miRNA-106 regulates the EMT event.117 After EMT phage tumor cells come into the circulation system and TEXs integrin promote organ-specific metastasis.10 TEXs are also associated with drug and therapeutic resistance.118,119 Finally, exosomes biology explains cancer in a different way.
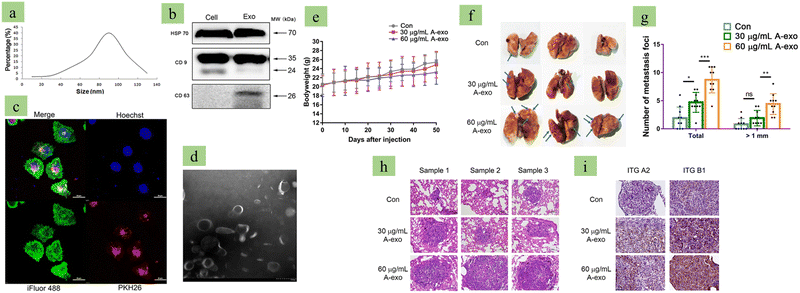 |
| Fig. 6 Tumor-derived exosomes promote metastasis. (a) Lung cancer (A549) cell-derived exosome size distribution analysis via NTA, (b) exosome marker analysis via western blots, (c) confocal microscopy image of A549-derived exosome-treated A549 cells. (d) Transmission Electron Microscopy image of exosomes, Images (e) to (i) are evidence of A549-derived exosomes promoting metastasis. (e) A549-derive exosomes reduce body weight in a mouse model, (f) A549-derived exosomes develop metastasis nodules in the lungs, (g) statistical analysis of metastatic foci, (h) H&E staining indicating that high concentration A549-derived exosomes promote metastasis, and (i) A549-derived exosomes promote non-small cell lung cancer based on a dose dependent manner. (Reproduced with permission under Creative Commons CC BY 4.0 license from ref. 120 Copyright 2020 The Authors.) | |
7. Plant exosomes-based cancer drug delivery
Cancerous neoplasms represent a formidable threat to human health, prompting the exploration of medicinal flora as valuable complements to contemporary medical approaches. Recent advancements in microRNA (miRNA) research have unveiled the intricate connections between miRNAs and the processes of tumor proliferation and apoptosis.121 Moreover, the expanding field of extracellular lipid vesicles has brought to light the ability of plant-derived miRNAs to transcend species boundaries and exert regulatory influences within the human organism.122,123 Contemporary chemotherapeutic treatments struggle with the intertwined challenges of inadequate target specificity and heightened toxicity. This challenge was addressed via an innovative approach, namely epithelial cell-based capsule development and incorporation with a therapeutic agent (it showed more effectiveness in the in-vivo model).124 PDEs can solve liposomes-based drug delivery limitations125 and their anti-cancer activity (Fig. 7) is promising.
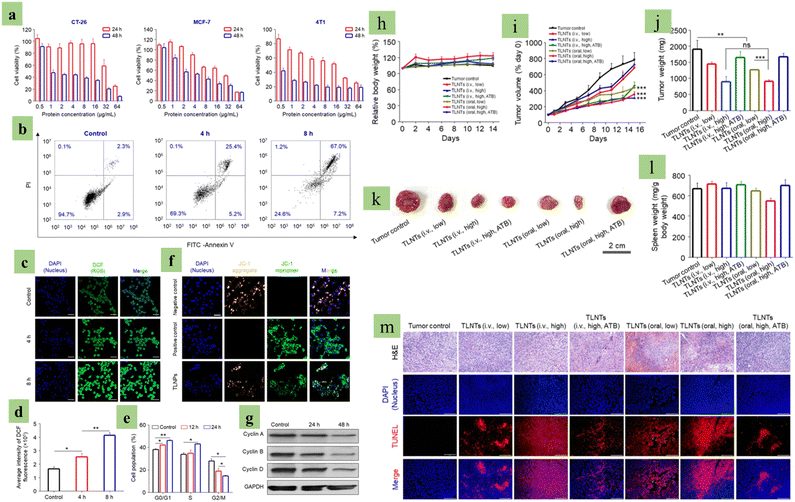 |
| Fig. 7 Anti-cancer effect of plant-derived exosomes (PDEs). Images (a) to (g) are the PDEs’ anti-cancer effects in an in vitro model. (a) Cytotoxic analysis of tea leaf-derived exosomes, (b) apoptosis analysis of tea leaf-derived exosome-treated cells, (c) confocal microscopic image of tea leaf-derived exosomes and cancer cells co-cultured after incubation, (d) ROS fluorescence intensity analysis, (e) mitochondrial membrane potential changes of cancer cells, (f) cell cycle analysis of leaf-derived exosomes and cancer cell co-cultured cells, and (g) cell cycle-associated protein expression analysis via western blot. Images (h) to (m) are in vivo evidence of PDEs’ anti-cancer effect against breast cancer, (h) body weight and (i) tumor growth alteration during the experiment period, (j) tumor weight, (k) image, (j) respective tumor image, (l) spleen weight variation in different experimental mice groups, and (m) KEGG pathway analysis for cell apoptosis-associated genes. (Reproduced with permission under Creative Commons CC BY 4.0 license from ref. 126 Copyright 2020 The Authors). | |
In addition, the re-engineered nano vectors derived from plant exosomes have exhibited better safety and precision in drug delivery. Scientists have successfully re-engineered nano vectors from ginger-derived exosomes to deliver Doxorubicin127 to colon tumor cells.128 These exhibit exceptional internalization efficiency within Colon-26 tumor model cells,129 resulting in growth inhibition. Ginger-derived exosomes show anti-cancer activity by reducing oxidative stress and pro-inflammatory cytokine production. Moreover, these nano vectors display an opportunistic Doxorubicin release triggered by the acidic pH of the tumor's extracellular microenvironment, thus signifying a reduction in Doxorubicin's adverse effects. Furthermore, they have been deemed harmless, biocompatible, and amenable to cost-effective large-scale production. Comparative assessments with artificially synthesized liposomes reveal their superiority in terms of biocompatibility, safety, apoptosis induction, electric cell-substrate impedance, and pH-dependent drug release profiles. Grapefruit-derived exosomes-based Folic Acid and Paclitaxel transport is observed in colon cancer.130–132 Modified grape-derived exosomes (surface coating via inflammatory chemokine receptor) increase effectiveness against colon and breast cancer. This type of surface modification develops strong specificity of drug delivery tools.133 PDEs are a promising chemotherapeutic drug delivery tool and their own anti-cancer property makes them more effective.134,135 Their therapeutic potential activity is impressive but this domain needs deeper investigation, specifically PDE miRNA.136 PDE-based drug delivery is described in Fig. 8.
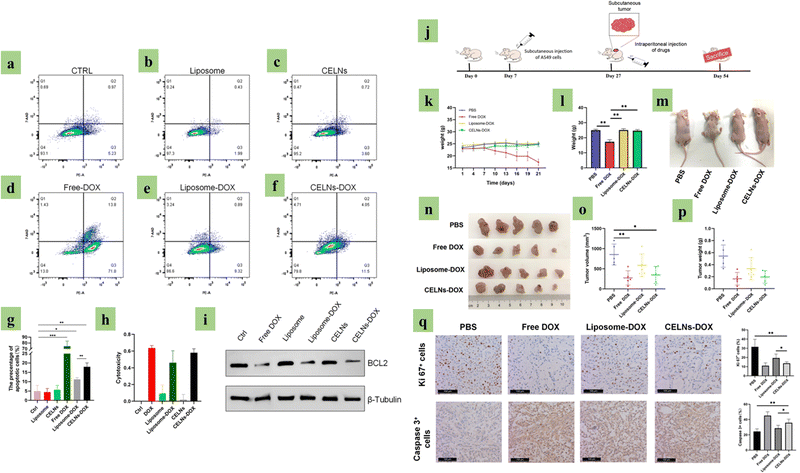 |
| Fig. 8 Plant exosome-based (PDE) drug delivery. Image (a) to (i) is the PDE effect in an in vitro model. (a) to (f) show PDE-treated A549 cells apoptosis analysis, (g) histogram analysis for (a) to (f), (h) PDE-treated A549 cell cytotoxicity analysis, (i) apoptosis markers (BCL2 and β-Tubulin) in treated A549 cells via western blot, (j) in vivo experimental protocol, (k) reducing body weight after drug injection, (l) 4 group body weight douching comparison, (m) image of the 4 mice groups’ body size alteration, (n) tumor size of the 4 groups of mice, (o) and (p) size and weight analysis of the 4 experimental mice groups, and (q) qKi 67 and Caspase 3 marker analysis via immunohistochemistry, on samples that are collected from 4 tumor groups. (Reproduced with permission under Creative Commons CC BY 4.0 license from ref. 137 Copyright 2020 The Authors.) | |
Plant-derived exosomes have emerged as promising candidates for drug delivery with extracts from edible plants such as ginger, lemon, and grapefruit demonstrating remarkable potential.138,139 These innovative carriers address the limitations associated with drugs like Methotrexate (MTX), Doxorubicin (DOX), Paclitaxel (PTX), Curcumin, and others, including issues like poor water solubility, rapid clearance by the body, and inadequate biocompatibility.140 Exosomes exhibit a remarkable ability to encapsulate therapeutic agents such as cisplatin and nanoparticles like gold nanoparticles.141,142 Elahe Mahdipour isolated exosomes from beet extracts, revealing their antioxidant and scavenging effects.143 Similarly, Fikrettin Şahin explored the role of wheat exosomes in wound healing, demonstrating their efficacy in cell survival and migration, providing a novel avenue for skin wound repair.144 Inflammatory bowel disease (IBD), often linked with colitis-associated cancer (CAC), involves inflammatory cells producing tumor-promoting cytokines.145 Plant-derived exosomes have shown promise for drug delivery targeting the intestine.146 Their established oral tolerance, stemming from interactions with the intestinal immune system and regular dietary consumption, makes them particularly appealing.147 They also exhibit high resistance to gastric proteolytic enzymes, the pancreas, and bile extracts, supporting their viability for oral administration, especially when targeting tumors within the gastrointestinal tract. Noteworthy examples include Folate-modified, Doxorubicin-loaded ginger-derived exosomes-mimetic nanovesicles, showcasing excellent tissue compatibility and anti-tumor effects in colorectal cancer.128 Additionally, grapefruit-derived nano-carriers have demonstrated effective delivery of therapeutic drugs and enhanced homing ability to inflammatory tumor tissues.148 These findings underscore the potential of plant derived EVs as a novel and promising avenue for drug delivery.149–151
8. Clinical trials
It is widely acknowledged that exosomes represent a promising therapeutic potential for a range of medical conditions. Prior to embarking on clinical trials, extensive preclinical investigations have consistently underscored the benefits of exosomes in a spectrum of diseases, in diverse domains such as regenerative medicine and oncology. In recent years, clinical trials have delved into the exploration of exosomes. These trials have predominantly employed two categories of exosomes: those derived from human cellular sources and others from plants. Plant exosomes-related clinical trials are listed in Table 2. In the future, more PDE-based (if medicinally significant plants get priority, it will hopefully become a great initiative) clinical trials are needed for a better cancer therapeutic solution.
Table 2 Clinical trial of plant-derived exosomes
Trail ID |
Status |
Source of exosomes |
Cancer |
Clinical significance |
Funding |
Link: https://clinicaltrials.gov |
NCT01294072 |
Unknown status |
Plant exosomes |
Colon cancer |
Plant exosomes to deliver Curcumin |
University of Louisville |
|
NCT01668849 |
Completed |
Grape exosomes |
Head and neck cancer |
Preliminary clinical trial investigating the ability of plant exosomes to abrogate oral mucositis induced by combined chemotherapy and radiation in head and neck cancer patients |
University of Louisville |
9. Animal-derived exosomes vs. Plant-derived exosomes
Clinical applications of plant-derived exosomes are more effective compared to animal-derived exosomes in several aspects (Table 3). Significantly, PDE-based therapeutics show more promising outcomes in cancer treatment. In future research, plant phytochemical participation in PDEs therapeutic activity must be explored.
Table 3 Animal-derived exosomes vs. Plant-derived exosomes
Facts |
Animal-derived exosomes |
Plant-derived exosomes |
Sources |
Stem cells, tumor cells, immune cells |
Vegetables and fruits |
Cost |
Production cost is high |
Production cost is low |
Toxicity |
This source of exosomes shows mixed outcomes (it may heal cancer or promote cancer) |
This source of exosomes has low toxicity compared to Animal-derived exosomes |
Ethical complications |
Present |
Not applicable |
Applications in translational research |
Can be used as a drug delivery tool, biomarkers investigation, cancer therapeutics |
Can be used as a cancer therapeutic agent |
10. Future perspectives
Historically, the landscape of cancer treatment has primarily revolved around surgical procedures,152 chemotherapy,153 targeted therapy,154 and radiotherapy.155 Unfortunately, these therapeutic approaches often come with a plethora of side effects, the development of acquired resistance, frequent metastasis, and disease recurrence, posing significant challenges to successful treatment. In recent times, a paradigm shift has occurred with the emergence of cancer immunotherapy.156 This innovative approach offers a promising alternative to the traditional treatment modalities mentioned earlier. Cancer immunotherapy has shown remarkable clinical outcomes, garnering significant attention at the forefront of next-generation cancer treatments. This therapeutic approach has several drawbacks,157,158 and its limitations can be overcome when it is combined with the intrinsic cancer vaccination (ICV) method.159 The exosomes-based therapeutic approach shows more efficiency and specificity.160–162 In cancer therapeutic development, exosomes are a promising platform. They may support future cancer vaccine163 and cancer precision medicine164 development. Exosomes biology requires an effective solution for exosomes heterogeneity165 which is a big limitation in exosomes-based cancer therapy development. The single exosomes profiling166 (Fig. 9) phenomenon is an effective method to decode this complication. PDEs are a green approach for cancer therapeutic development.167–169 Their biocompatibility, anti-inflammatory, and anti-oxidant properties make them an exciting source of cancer therapy.149,170,171 The fusion of PDEs and advanced technology will address cancer-related solutions in a new way.172,173 Surface-engineered and genetically modified PDEs may be a game changer in the future.
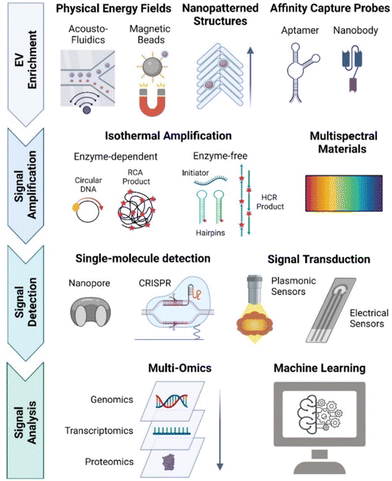 |
| Fig. 9 Single exosome profiling. (Reproduced with permission from ref. 174 Copyright @ 2022 American Chemical Society.) | |
11. Conclusion
Plant-derived exosomes represent a promising and sustainable platform for delivering bioactive molecules, and have opened up exciting avenues of research with profound implications for various aspects of science and medicine. However, it is crucial to acknowledge that the field of plant-derived exosomes is still in its infancy, and many questions remain unanswered. The safety profile of PDEs remains a subject of persistent scrutiny within the scientific discourse. These inquiries are primarily centred around two fundamental concerns: the consistency and quality stability of plant-derived raw materials, as well as the intricate composition of PDEs extracts. The doubts surrounding the safety of PDEs often hinge on the presence of impurities within the extracts, which have the potential to instigate unintended viral dissemination within the body. Addressing the multifaceted challenges and concerns associated with PDEs necessitates a concerted effort spanning research, technology, and quality control. To address the complexity of PDEs extract molecular profiling, these methods enable a comprehensive characterization of the constituents within PDEs, facilitating a deeper understanding of their composition and potential impact on safety. Developing innovative approaches such as freeze-drying or encapsulation in protective matrices can help maintain the integrity of exosomes during storage and delivery. The route of injection and method of delivery has not been studied in detail and therefore further exploration on this topic is required to understand the effectiveness of PDEs on cancer therapy. Comprehensive safety assessments have been conducted focusing on potential adverse effects of PDEs, entailing both in vitro and in vivo studies, including toxicity assessments and long-term safety evaluations, which will mitigate concerns regarding safety. It is prudent to emphasize that a comprehensive investigation in this domain remains somewhat limited, necessitating further in-depth inquiry to elucidate the nuanced dynamics and effects of PDEs. This has ultimately paved the way to the development of novel methodologies that scrutinize exosomes at the single particle level, thereby affording an unprecedented opportunity to delve into their biogenesis intricacies, establish correlations among distinctive markers to bolster specificity and establish a direct linkage between exosomes cargo and its origin or ultimate destination. In summary, extant research may underscore both the positive and negative impacts of PDEs on human biology and provide insight on PDEs potential for future effective and affordable cancer therapy.
Conflicts of interest
There are no conflicts to declare.
References
- K. Minton, Pan-cancer atlas of intratumor heterogeneity, Nat. Rev. Genet., 2023, 24, 487 CrossRef CAS PubMed.
- R. A. Cairns, I. S. Harris and T. W. Mak, Regulation of Cancer Cell Metabolism, Nat. Rev. Cancer, 2011, 11, 85–95 CrossRef CAS PubMed.
- R. L. Siegel, K. D. Miller, N. S. Wagle and A. Jemal, Cancer statistics, 2023, Ca-Cancer J. Clin., 2023, 73(1), 17–48 CrossRef PubMed.
- J. Zhao, L. Xu and J. Sun, Global trends in incidence, death, burden and risk factors of early-onset cancer from 1990 to 2019, BMJ Oncol., 2023, 2, e000049, DOI:10.1136/bmjonc-2023-000049.
- L. Lippens and C. Pinheiro,
et al., Extracellular vesicle analysis, Nat. Rev. Methods Primers, 2023, 3, 56 CrossRef.
- R. Dhar, S. Mallik and A. Devi, Exosomal microRNAs (exoMIRs): micromolecules with macro impact in oral cancer, 3 Biotech., 2022, 12(7), 155 CrossRef PubMed.
- S. Kumar, R. Dhar, L. B. S. S. Kumar, G. G. Shivji, R. Jayaraj and A. Devi, Theranostic signature of tumor-derived exosomes in cancer, Med. Oncol., 2023, 40(11), 321 CrossRef PubMed.
- M. R. Mohammadi, S. M. Rodriguez and J. C. Luong,
et al., Exosome loaded immunomodulatory biomaterials alleviate local immune response in immunocompetent diabetic mice post islet xenotransplantation, Commun. Biol., 2021, 4, 685 CrossRef CAS PubMed.
- S. Miaomiao, W. Xiaoqian and S. Yuwei,
et al., Cancer-associated fibroblast-derived exosome microRNA-21 promotes angiogenesis in multiple myeloma, Sci. Rep., 2023, 13, 9671 CrossRef PubMed.
- R. Dhar, A. Devi, S. Gorai, S. K. Jha, A. Alexiou and M. Papadakis, Exosome and epithelial-mesenchymal transition: A complex secret of cancer progression, J. Cell. Mol. Med., 2023, 27(11), 1603–1607 CrossRef CAS PubMed.
- R. Dhar, S. Mukherjee, N. Mukerjee, D. Mukherjee, A. Devi, G. M. Ashraf, R. F. Alserihi, H. H. Tayeb, A. M. Hashem, A. Alexiou and N. Thorate, Interrelation between extracellular vesicles miRNAs with chronic lung diseases, J. Cell. Physiol., 2022, 237(11), 4021–4036 CrossRef CAS PubMed.
- L. Qian, X. Yang and S. Li,
et al., Reduced O-GlcNAcylation of SNAP-23 promotes cisplatin resistance by inducing exosome secretion in ovarian cancer, Cell Death Discov., 2021, 7, 112 CrossRef CAS PubMed.
- J. C. Santos, N. Lima and L. O. Sarian,
et al., Exosome-mediated breast cancer chemoresistance via miR-155 transfer, Sci. Rep., 2018, 8, 829 CrossRef PubMed.
- S. Hoque,
et al., Cancer stem cells (CSCs): key player of radiotherapy resistance and its clinical significance, Biomarkers, 2023, 28(2), 139–151 Search PubMed.
- H. Chen, L. Wang, X. Zeng, H. Schwarz, H. S. Nanda, X. Peng and Y. Zhou, Exosomes, a New Star for Targeted Delivery, Front. Cell Dev. Biol., 2021, 9, 751079 Search PubMed.
- A. Krishnan, B. Bhattacharya, D. Mandal, R. Dhar and S. Muthu, Salivary exosomes: A theranostics secret of oral cancer - Correspondence, Int. J. Surg., 2022, 108, 106990 Search PubMed.
- L. Zitvogel,
et al., Eradication of established murine tumors using a novel cell-free vaccine: dendritic cell-derived exosomes, Nat. Med., 1998, 4, 594–600 CrossRef CAS PubMed.
- B. M. Hussen, G. S. H. Faraj, M. F. Rasul, H. J. Hidayat, A. Salihi, A. Baniahmad, M. Taheri and S. Ghafouri-Frad, Strategies to overcome the main challenges of the use of exosomes as drug carrier for cancer therapy, Cancer Cell Int., 2022, 22(1), 323 CrossRef PubMed.
- R. Dhar, N. Mukerjee and D. Mukherjee,
et al., Plant-derived exosomes: a new dimension in cancer therapy, Phytother. Res., 2023, 10, 1002 Search PubMed.
- P. Akuma, O. D. Okagu and C. C. Udenigwe, Naturally Occurring Exosome Vesicles as Potential Delivery Vehicle for Bioactive Compounds, Front. Sustainable. Food Syst., 2019, 3, 23 CrossRef.
- D. Ratnadewi, C. H. Widjaja, A. Barlian, R. M. Amsar, I. D. Ana, A. C. Hidajah, H. B. Notobroto and T. D. K. Wungu, Isolation of Native Plant-Derived Exosome-like Nanoparticles and Their Uptake by Human Cells, Hayati., 2023, 30, 182–192 CrossRef.
- I. K. Herrmann, M. J. A. Wood and G. Fuhrmann, Extracellular vesicles as a next-generation drug delivery platform, Nat. Nanotechnol., 2021, 16, 748–759 CrossRef CAS PubMed.
- R. J. Simpson, J. W. Lim, R. L. Moritz and S. Mathivanan, Exosomes: proteomic insights and diagnostic potential, Expert Rev. Proteomics, 2009, 6(3), 267–283 CrossRef CAS PubMed.
- J. A. Lee, J. An, J. Taniguchi, G. Kashiwazaki, G. N. Pandian, N. Parveen, T. M. Kang, H. Sugiyama, D. De and K. K. Kim, Targeted epigenetic modulation using a DNA-based histone deacetylase inhibitor enhances cardio myogenesis in mouse embryonic stem cells, J. Cell. Physiol., 2021, 236(5), 3946–3962 CrossRef CAS PubMed.
- C. Jancic, A. Savina and C. Wasmeier,
et al., Rab27a regulates phagosomal pH and NADPH oxidase recruitment to dendritic cell phagosomes, Nat. Cell Biol., 2007, 9, 367–378 CrossRef CAS PubMed.
- B. Bhattacharya, R. Dhar and S. Mukherjee,
et al., Exosome DNA: An untold story of cancer, Clin. Transl. Disc., 2023, 3, e218 CrossRef.
- V. R. Minciacchi, M. R. Freeman and D. Di Vizio, Extracellular vesicles in cancer: exosomes, microvesicles and the emerging role of large oncosomes, Semin. Cell Dev. Biol., 2015, 40, 41–51 CrossRef CAS PubMed.
- C. Théry, L. Zitvogel and S. Amigorena, Exosomes: composition, biogenesis and function, Nat. Rev. Immunol., 2002, 2, 569–579 CrossRef PubMed.
- M. Colombo, G. Raposo and C. Théry., Annu. Rev. Cell Dev. Biol., 2014, 30(1), 255–289 CrossRef CAS PubMed.
- R. Kalluri and V. S. LeBleu, The biology, function, and biomedical applications of exosomes, Science, 2020, 367(6478), eaau6977 CrossRef CAS PubMed.
- L. Lemus and V. Goder, Membrane trafficking: ESCRTs act here, there, and everywhere, Curr. Biol., 2022, 32(6), R292–R294 CrossRef CAS PubMed.
- P. I. Hanson, S. Shim and S. A. Merrill, Cell biology of the ESCRT machinery, Curr. Opin. Cell Biol., 2009, 21(4), 568–574 CrossRef CAS PubMed.
- S. Ghosh, R. Dhar, G. Gurudas Shivji, D. Dey, A. Devi, S. K. Jha, M. D. Adhikari and S. Gorai, Clinical Impact of Exosomes in Colorectal Cancer Metastasis, ACS Appl. Bio Mater., 2023, 6(7), 2576–2590 CrossRef CAS PubMed.
- G. G. Shivji, R. Dhar and A. Devi, Role of exosomes and its emerging therapeutic applications in the pathophysiology of non-infectious diseases, Biomarkers, 2022, 27(6), 534–548 Search PubMed.
- W. M. Henne, N. J. Buchkovich and S. D. Emr, The ESCRT pathway, Dev. Cell, 2011, 21(1), 77–91 CrossRef CAS PubMed.
- S. Gurung, D. Perocheau, L. Touramanidou and J. Baruteau, The exosome journey: from biogenesis to uptake and intracellular signalling, Cell Commun. Signaling, 2021, 19(1), 47 CrossRef CAS PubMed.
- C. Villarroya-Beltri, F. Baixauli, C. Gutiérrez-Vázquez, F. Sánchez-Madrid and M. Mittelbrunn, Sorting it out: regulation of exosome loading, Semin. Cancer Biol., 2014, 28, 3–13 CrossRef CAS PubMed.
- M. V. Airola and Y. A. Hannun, Sphingolipid metabolism and neutral sphingomyelinases, Handb. Exp. Pharmacol., 2013, 215, 57–76 CAS.
- B. M. Castro, M. Prieto and L. C. Silva, Ceramide: a simple sphingolipid with unique biophysical properties, Prog. Lipid Res., 2014, 54, 53–67 CrossRef CAS PubMed.
- C. Mencarelli and P. Martinez–Martinez, Ceramide function in the brain: when a slight tilt is enough, Cell. Mol. Life Sci., 2013, 70, 181–203 CrossRef CAS PubMed.
- M. DiPasquale, T. G. Deering, D. Desai, A. K. Sharma, S. Amin, T. E. Fox, M. Kester, J. Katsaras, D. Marquardt and F. A. Heberle, Influence of ceramide on lipid domain stability studied with small-angle neutron scattering: The role of acyl chain length and unsaturation, Chem. Phys. Lipids, 2022, 245, 105205 CrossRef CAS PubMed.
- P.-H. Daniel, G.-V. Cristina, J. Inmaculada, L.-M. Soraya, U. Angeles, S.-M. Francisco, V. Jesús and Y.-M. María, The Intracellular Interactome of Tetraspanin-enriched Microdomains Reveals Their Function as Sorting Machineries toward Exosomes, J. Biol. Chem., 2013, 288(17), 11649–11661 CrossRef PubMed.
- J. G. van den Boorn, J. Dassler, C. Coch, M. Schlee and G. Hartmann, Exosomes as nucleic acid nanocarriers, Adv. Drug Delivery Rev., 2013, 65(3), 331–335 CrossRef CAS PubMed.
- H. Yan, Y. Li, S. Cheng and Y. Zeng, Advances in Analytical Technologies for Extracellular Vesicles, Anal. Chem., 2021, 93(11), 4739–4774 CrossRef CAS PubMed.
- J. Jankovičová, P. Sečová, K. Michalková and J. Antalíková, Tetraspanins, More than Markers of Extracellular Vesicles in Reproduction, Int. J. Mol. Sci., 2020, 21(20), 7568, DOI:10.3390/ijms21207568.
- E. Woith and M. F. Melzig, Extracellular vesicles from fresh and dried plants—simultaneous purification and visualization using gel electrophoresis, Int. J. Mol. Sci., 2019, 20, 357 CrossRef PubMed.
- Q. An, A. J. van Bel and R. Hückelhoven, Do plant cells secrete exosomes derived from multivesicular bodies?, Plant Signal Behav., 2007, 2, 4–7 CrossRef PubMed.
- J. Wang, Y. Ding, J. Wang, S. Hillmer, Y. Miao, S. W. Lo, X. Wang, D. G. Robinson and L. Jiang, EXPO, an exocyst-positive organelle distinct from multivesicular endosomes and autophagosomes, mediates cytosol to cell wall exocytosis in Arabidopsis and tobacco cells, Plant Cell, 2010, 22, 4009–4030 CrossRef CAS PubMed.
- D. G. Robinson, Y. Ding and L. Jiang, Unconventional protein secretion in plants: a critical assessment, Protoplasma, 2016, 253, 31–43 CrossRef CAS PubMed.
- L. L. Hansen and M. E. Nielsen, Plant exosomes: using an unconventional exit to prevent pathogen entry?, J. Exp. Bot., 2018, 69, 59–68 CrossRef PubMed.
- J. Xiao, S. Feng, X. Wang, K. Long, Y. Luo, Y. Wang, J. Ma, Q. Tang, L. Jin and X. Li, Identification of exosome-like nanoparticle-derived microRNAs from 11 edible fruits and vegetables, PeerJ, 2018, 6, e5186 CrossRef PubMed.
- J. Mu, X. Zhuang, Q. Wang, H. Jiang, Z. B. Deng, B. Wang, L. Zhang, S. Kakar, Y. Jun and D. Miller, Interspecies communication between plant and mouse gut host cells through edible plant derived exosome-like nanoparticles, Mol. Nutr. Food Res., 2014, 58, 1561–1573 CrossRef CAS PubMed.
- Z. Zhao, S. Yu, M. Li, X. Gui and P. Li, Isolation of exosome-like nanoparticles and analysis of microRNAs derived from coconut water based on small RNA high-throughput sequencing, J. Agric. Food Chem., 2018, 66, 2749–2757 CrossRef CAS PubMed.
- M. Regente, G. Corti-Monzón, A. M. Maldonado, M. Pinedo, J. Jorrín and L. de la Canal, Vesicular fractions of sunflower apoplastic fluids are associated with potential exosome marker proteins, FEBS Lett., 2009, 583, 3363–3366 CrossRef CAS PubMed.
- B. D. Rutter and R. W. Innes, Extracellular vesicles isolated from the leaf apoplast carry stress-response proteins, Plant Physiol., 2017, 173, 728–741 CrossRef CAS PubMed.
- M. Zhang, X. Wang, M. K. Han, J. F. Collins and D. Merlin, Oral administration of ginger-derived nanolipids loaded with siRNA as a novel approach for efficient siRNA drug delivery to treat ulcerative colitis, Nanomedicine, 2017, 12, 1927–1943 CrossRef CAS PubMed.
- A. C. Brown, C. Shah, J. Liu, J. T. Pham, J. G. Zhang and M. R. Jadus, Ginger's (Zingiber officinale Roscoe) inhibition of rat colonic adenocarcinoma cells proliferation and angiogenesis in vitro, Phytother. Res., 2009, 23, 640–645 CrossRef PubMed.
- X. Zhuang, Z.-B. Deng, J. Mu, L. Zhang, J. Yan, D. Miller, W. Feng, C. J. McClain and H.-G. Zhang, Ginger-derived nanoparticles protect against alcohol-induced liver damage, J. Extracell. Vesicles, 2015, 4, 28713 CrossRef PubMed.
- S. Ju, J. Mu, T. Dokland, X. Zhuang, Q. Wang, H. Jiang, X. Xiang, Z.-B. Deng, B. Wang and L. Zhang, Grape exosome-like nanoparticles induce intestinal stem cells and protect mice from DSS-induced colitis, Mol. Ther., 2013, 21, 1345–1357 CrossRef CAS PubMed.
- Q. Wang, Y. Ren, J. Mu, N. K. Egilmez, X. Zhuang, Z. Deng, L. Zhang, J. Yan, D. Miller and H.-G. Zhang, Grapefruit-derived nanovectors use an activated leukocyte trafficking pathway to deliver therapeutic agents to inflammatory tumor sites, Can. Res., 2015, 75, 2520–2529 CrossRef CAS PubMed.
- X. Zhuang, Y. Teng, A. Samykutty, J. Mu, Z. Deng, L. Zhang, P. Cao, Y. Rong, J. Yan and D. Miller, Grapefruit-derived nanovectors delivering therapeutic miR17 through an intranasal route inhibit brain tumor progression, Mol. Ther., 2016, 24, 96–105 CrossRef CAS PubMed.
- S. Raimondo, F. Naselli, S. Fontana, F. Monteleone, A. lo Dico, L. Saieva, G. Zito, A. Flugy, M. Manno and M. A. di Bella,
et al., Citrus Limon-Derived Nanovesicles Inhibit Cancer Cell Proliferation and Suppress CML Xenograft Growth by Inducing TRAIL-Mediated Cell Death, Oncotarget, 2015, 6, 19514–19527 CrossRef PubMed.
- L. Zhang, F. He, L. Gao, M. Cong, J. Sun, J. Xu, Y. Wang, Y. Hu, S. Asghar and L. Hu,
et al., Engineering Exosome-like Nanovesicles Derived from Asparagus Cochinchinensis Can Inhibit the Proliferation of Hepatocellular Carcinoma Cells with Better Safety Profile, Int. J. Nanomed., 2021, 16, 1575–1586 CrossRef PubMed.
- M. Nemati, B. Singh and R. A. Mir,
et al., Plant-derived extracellular vesicles: a novel nanomedicine approach with advantages and challenges, Cell Commun. Signaling, 2022, 20, 69 CrossRef CAS PubMed.
- D. Subha, K. Harshnii, K. G. Madhikiruba, M. Nandhini and K. S. Tamilselvi, Plant derived exosome- like Nanovesicles: an updated overview, Plant Nano Biol., 2023, 3, 100022 CrossRef.
- Q. Zhou, K. Ma, H. Hu, X. Xing, X. Huang and H. Gao, Extracellular vesicles: their functions in plant–pathogen interactions, Mol. Plant Pathol., 2021, 23, 760–771 CrossRef PubMed.
- L. Garaeva, R. Kamyshinsky, Y. Kil, E. Varfolomeeva, N. Verlov, E. Komarova, Y. Garmay, S. Landa, V. Burdakov and A. Myasnikov, Delivery of functional exogenous proteins by plant-derived vesicles to human cells in vitro, Sci. Rep., 2021, 11, 1–12 CrossRef PubMed.
- E. Woith, G. Fuhrmann and M. F. Melzig, Extracellular vesicles—connecting kingdoms, Int. J. Mol. Sci., 2019, 20, 5695 CrossRef CAS PubMed.
- G. Pocsfalvi, L. Turiák, A. Ambrosone, P. Del Gaudio, G. Puska, I. Fiume, T. Silvestre and K. Vékey, Protein biocargo of citrus fruit-derived vesicles reveals heterogeneous transport and extracellular vesicle populations, J. Plant Physiol., 2018, 229, 111–121 CrossRef CAS PubMed.
- A. Tukmechi, J. Rezaee, V. Nejati and N. Sheikhzadeh, Effect of acute and chronic toxicity of paraquat on immune system and growth performance in rainbow trout Oncorhynchus mykiss, Aquac Res., 2014, 45, 1737–1743 CAS.
- N. Prado, A. J. de Dios, J. Casado-Vela, S. Mas, M. Villalba, R. Rodríguez and E. Batanero, Nanovesicles are secreted during pollen germination and pollen tube growth: a possible role in fertilization, Mol. Plant, 2014, 7, 573–577 CrossRef CAS PubMed.
- E. Woith, G. Guerriero, J.-F. Hausman, J. Renaut, C. C. Leclercq, C. Weise, S. Legay, A. Weng and M. F. Melzig, Plant extracellular vesicles and nanovesicles: focus on secondary metabolites, proteins and lipids with perspectives on their potential and sources, Int. J. Mol. Sci., 2021, 22, 3719 CrossRef CAS PubMed.
- M. Regente, M. Pinedo, H. San Clemente, T. Balliau, E. Jamet and L. De La Canal, Plant extracellular vesicles are incorporated by a fungal pathogen and inhibit its growth, J. Exp. Bot., 2017, 68, 5485–5495 CrossRef CAS PubMed.
- O. Urzì, S. Raimondo and R. Alessandro, Extracellular vesicles from plants: current knowledge and open questions, Int. J. Mol. Sci., 2021, 22, 5366 CrossRef PubMed.
- N. Abdyazdani, A. Nourazarian, H. N. Charoudeh, M. Kazemi, N. Feizy, M. Akbarzade, A. Mehdizadeh, J. Rezaie and R. Rahbarghazi, The role of morphine on rat neural stem cells viability, neuro-angiogenesis and neuro-steroidgenesis properties, Neurosci. Lett., 2017, 636, 205–212 CrossRef CAS PubMed.
- M. Alfieri, A. Leone and A. Ambrosone, Plant-derived nano and microvesicles for human health and therapeutic potential in nanomedicine, Pharmaceutics, 2021, 13, 498 CrossRef CAS PubMed.
- M. Raja, Do small headgroups of phosphatidylethanolamine and phosphatidic acid lead to a similar folding pattern of the K+ channel?, J. Membr. Biol., 2011, 242, 137–143 CrossRef CAS PubMed.
- L. V. Michaelson, J. A. Napier, D. Molino and J.-D. Faure, Plant sphingolipids: their importance in cellular organization and adaption, Biochim. Biophys. Acta, Mol. Cell Biol. Lipids, 2016, 1861, 1329–1335 CrossRef CAS PubMed.
- N.-J. Liu, L.-P. Hou, J.-J. Bao, L.-J. Wang and X.-Y. Chen, Sphingolipid metabolism, transport, and functions in plants: recent progress and future perspectives, Plant Commun., 2021, 2, 100214 CrossRef CAS PubMed; L. V. Michaelson, J. A. Napier, D. Molino and J.-D. Faure, Plant sphingolipids: their importance in cellular organization and adaption, Biochim. Biophys. Acta, Mol. Cell Biol. Lipids, 2016, 1861, 1329–100235 CrossRef PubMed.
- J. Rizzo, A. Taheraly and G. Janbon, Structure, composition and biological properties of fungal extracellular vesicles, microLife, 2021, 2, uqab009 CrossRef PubMed.
- J. Kim, S. Li, S. Zhang and J. Wang, Plant-derived exosome-like nanoparticles and their therapeutic activities, Asian J. Pharm. Sci., 2021, 17, 53–69 CrossRef PubMed.
- L. Yepes-Molina, M. I. Pérez-Jiménez, M. Martínez-Esparza, J. A. Teruel, A. J. Ruiz-Alcaraz, P. García-Peñarrubia and M. Carvajal, Membrane vesicles for nanoencapsulated sulforaphane increased their anti-inflammatory role on an in vitro human macrophage model, Int. J. Mol. Sci., 1940, 2022, 23 Search PubMed.
- F. Perut, L. Roncuzzi, S. Avnet, A. Massa, N. Zini, S. Sabbadini, F. Giampieri, B. Mezzetti and N. Baldini, Strawberry-derived exosome-like nanoparticles prevent oxidative stress in human mesenchymal stromal cells, Biomolecules, 2021, 11, 87 CrossRef CAS PubMed.
- D. Fujita, T. Arai, H. Komori, Y. Shirasaki, T. Wakayama, T. Nakanishi and I. Tamai, Apple-derived nanoparticles modulate expression of organic-anion-transporting polypeptide (OATP) 2B1 in Caco-2 cells, Mol. Pharm., 2018, 15, 5772–5780 CrossRef CAS PubMed.
- Q. Cai, L. Qiao, M. Wang, B. He, F.-M. Lin, J. Palmquist, S.-D. Huang and H. Jin, Plants send small RNAs in extracellular vesicles to fungal pathogen to silence virulence genes, Science, 2018, 360, 1126–1129 CrossRef CAS PubMed.
- Y. Cui, J. Gao, Y. He and L. Jiang, Plant extracellular vesicles, Protoplasma, 2020, 257, 3–12 CrossRef CAS PubMed.
-
R. P. Alexander, N.-T. Chiou and K. M. Ansel, 2016, Improved exosome isolation by sucrose gradient fractionation of ultracentrifuged crude exosome pellets. Protocol Exchange Search PubMed.
- A. Liga, A. D. B. Vliegenthart, W. Oosthuyzen, J. W. Dear and M. Kersaudy-Kerhoas, Exosome isolation: A microfluidicroad-map, Lab on a Chip, 2015, 15(11), 2388–2394 RSC.
- A. Cheruvanky, H. Zhou, T. Pisitkun, J. B. Kopp, M. A. Knepper, P. S. T. Yuen and R. A. Star, Rapid isolation of urinary exo-somal biomarkers using a nanomembrane ultrafiltration concen-trator, Am. J. Physiol.: Renal Physiol., 2007, 292(5), 1657–1661 CrossRef PubMed.
- D. Kang, S. Oh, S. M. Ahn, B. H. Lee and M. H. Moon, Pro-teomic analysis of exosomes from human neural stem cells by flow field-flow fractionation and nanoflow liquid chromatography-tandem mass spectrometry, J. Proteome Res., 2008, 7(8), 3475–3480 CrossRef CAS PubMed.
- E. Zeringer, T. Barta, M. Li and A. V. Vlassov, Strategies forisolation of exosomes, Cold Spring Harbor Protocols, 2015, 2015(4), 319–323 CrossRef PubMed.
- X. X. Yang, C. Sun, L. Wang and X. L. Guo, Newinsightinto isolation, identification techniques and medical applicationsof exosomes, J. Controlled Release, 2019, 308, 119–129 CrossRef CAS PubMed.
- J. S. Yang, J. C. Lee, S. K. Byeon, K. H. Rha and M. H. Moon, Size dependent lipidomic analysis of urinary exosomesfrom patients with prostate cancer by flow field-flow fraction-ation and nanoflow liquid chromatography-tandem mass spec-trometry, Anal. Chem., 2017, 89(4), 2488–2496 CrossRef CAS PubMed.
- S. Sitar, A. Kejžar, D. Pahovnik, K. Kogej, M. Tušek-Žnidarič, M. Lenassi and E. Žagar, Size characterization and quan-tification of exosomes by asymmetrical-flow field-flow fraction-ation, Anal. Chem., 2015, 87(18), 9225–9233 CrossRef CAS PubMed.
- S. Ju, J. Mu, T. Dokland, X. Zhuang, Q. Wang, H. Jiang, X. Xiang, Z. B. Deng, B. Wang, L. Zhang, M. Roth, R. Welti, J. Mobley, Y. Jun, D. Miller and H. G. Zhang, Grape exosome-like nanoparticles induce intestinal stem cells and protect mice induced colitis, Mol. Ther., 2013, 21(7), 1345–1357 CrossRef CAS PubMed.
- Y. Cheng, Q. Zeng, Q. Han and W. Xia, Effect of pH, temperature and freezing-thawing on quantity changes and cellular uptake of exosomes, Proteins Cell, 2019, 10, 295–299 CrossRef CAS PubMed.
- N. Zarovni, A. Corrado, P. Guazzi, D. Zocco, E. Lari, G. Radano, J. Muhhina, C. Fondelli, J. Gavrilova and A. Chiesi, Inte-grated isolation and quantitative
analysis of exosomeshuttled pro-teins and nucleic acids using immunocapture approaches, Methods, 2015, 87, 46–58 CrossRef CAS PubMed.
- S. P. Kalarikkal, D. Prasad, R. Kasiappan, S. R. Chaudhari and G. M. Sun-daram, A cost-effective polyethylene glycol-basedmethod for the isolation of functional edible nanoparticles from ginger rhizomes, Sci. Rep., 2020, 10, 1 CrossRef PubMed.
- N. Koliha, Y. Wiencek, U. Heider, C. Jüngst, N. Kladt, S. Krauthäuser, I. C. D. Johnston, A. Bosio, A. Schauss and S. Wild, A novel multiplex bead-based platform highlights the diversity of extracellular vesicles, J. Extracell. Vesicles, 2016, 5, 581 Search PubMed.
- L. Garaeva, R. Kamyshinsky, Y. Kil, E. Varfolomeeva, N. Verlov, E. Komarova, Y. Garmay, S. Landa, V. Burdakov, A. Myasnikov, I. A. Vinnikov, B. Margulis, I. Guzhova, A. Kagansky, A. L. Konevega and T. Shtam, Delivery of functional exogenous proteins by plant-derived vesicles to human cells in vitro, Sci. Rep., 2021, 11(1), 6489 CrossRef CAS PubMed.
- A. Upadhyay, Cancer: An unknown territory; rethinking before going ahead, Genes Dis., 2020, 8(5), 655–661 CrossRef PubMed.
- C. Roma-Rodrigues, R. Mendes, P. V. Baptista and A. R. Fernandes, Targeting tumor microenvironment for cancer therapy, Int. J. Mol. Sci., 2019, 20, 840 CrossRef CAS PubMed.
- I. Li and B. Y. Nabet, Exosomes in the tumor microenvironment as mediators of cancer therapy resistance, Mol. Cancer, 2019, 18, 32 CrossRef PubMed.
- T. L. Whiteside, Tumor-derived exosomes and their role in cancer progression, Adv. Clin. Chem., 2016, 74, 103–141 CAS.
- E. N. Nolte-‘t Hoen, S. I. Buschow, S. M. Anderton, W. Stoorvogel and M. H. Wauben, Activated T cells recruit exosomes secreted by dendritic cells via LFA-1. Blood, J. Am. Soc. Hematol., 2009, 113(9), 1977–1981 Search PubMed.
- X. Yang, Y. Li, L. Zou and Z. Zhu, Role of exosomes in crosstalk between cancer-associated fibroblasts and cancer cells, Front. Oncol., 2019, 9, 356 CrossRef PubMed.
- T. X. Huang, X. Y. Guan and L. Fu, Therapeutic targeting of the crosstalk between cancer-associated fibroblasts and cancer stem cells, Am. J. Cancer Res., 2019, 9, 1889–1904 CAS.
- W. Li,
et al., TGFbeta1 in fibroblasts-derived exosomes promotes epithelial-mesenchymal transition of ovarian cancer cells, Oncotarget, 2017, 8, 96035–96047 CrossRef PubMed.
- Y. Hao, D. Baker and P. Ten Dijke, TGF-beta-mediated epithelial-mesenchymal transition and cancer metastasis, Int. J. Mol. Sci., 2019, 20, 2767 CrossRef CAS PubMed.
- Z. Sun, L. Wang, L. Dong and X. Wang, Emerging role of exosome signalling in maintaining cancer stem cell dynamic equilibrium, J. Cell. Mol. Med., 2018, 22, 3719–3728 CrossRef PubMed.
- Z. Cheng,
et al., Exosome-transmitted p120-catenin suppresses hepatocellular carcinoma progression via STAT3 pathways, Mol. Carcinog., 2019, 58, 1389–1399 CrossRef CAS PubMed.
- Q. H. Xie, J. Q. Zheng, J. Y. Ding, Y. F. Wu, L. Liu, Z. L. Yu and G. Chen, Exosome-Mediated Immunosuppression in Tumor Microenvironments, Cells, 2022, 11(12), 1946 CrossRef CAS PubMed.
- L. Muller,
et al., Human tumor-derived exosomes (TEX) regulate Treg functions via cell surface signaling rather than uptake mechanisms, Oncoimmunology, 2017, 6, e1261243 CrossRef PubMed.
- Y. L. Hsu,
et al., Hypoxic lung-cancer-derived extracellular vesicle microRNA-103a increases the oncogenic effects of macrophages by targeting PTEN, Mol. Ther., 2018, 26, 568–581 CrossRef CAS PubMed.
- N. Syn, L. Wang, G. Sethi, J. P. Thiery and B. C. Goh, Exosome-mediated metastasis: from epithelial-mesenchymal transition to escape from immunosurveillance, Trends Pharm. Sci., 2016, 37, 606–617 CrossRef CAS PubMed.
- X. Liu,
et al., Roles of signaling pathways in the epithelial-mesenchymal transition in cancer, Asian Pac. J. Cancer Prev., 2015, 16, 6201–6206 CrossRef PubMed.
- Z. Qu,
et al., Exosomes derived from HCC cells with different invasion characteristics mediated EMT through TGF-beta/Smad signaling pathway, OncoTargets Ther., 2019, 12, 6897–6905, DOI:10.2147/OTT.S209413.
- K. E. Gilligan and R. M. Dwyer, Engineering exosomes for cancer therapy, Int. J. Mol. Sci., 2017, 18, 1122 CrossRef PubMed.
- A. Srivastava,
et al., Exosomes as theranostics for lung cancer, Adv. Cancer Res., 2018, 139, 1–33 CrossRef CAS PubMed.
- W. Huang, Y. Yan, Y. Liu, M. Lin, J. Ma, W. Zhang, J. Dai, J. Li, Q. Guo, H. Chen, B. Makabel, H. Liu, C. Su, H. Bi and J. Zhang, Exosomes with low miR-34c-3p expression promote invasion and migration of non-small cell lung cancer by upregulating integrin α2β1, Signal Transduction Targeted Ther., 2020, 5(1), 39 CrossRef CAS PubMed.
- F. Ingenito, G. Roscigno, A. Affinito, S. Nuzzo, I. Scognamiglio, C. Quintavalle and G. Condorelli, The role of exo-miRNAs in cancer: a focus on therapeutic and diagnostic applications, Int. J. Mol. Sci., 2019, 20(19), 4687 CrossRef CAS PubMed.
- Y. C. Liu, W. L. Chen, W. H. Kung and H. D. Huang, Plant miRNAs found in human circulating system provide evidences of cross kingdom RNAi, BMC Genomics, 2017, 18, 1–6 CrossRef PubMed.
- M. Zhang, E. Viennois, M. Prasad, Y. Zhang, L. Wang, Z. Zhang, M. K. Han, B. Xiao, C. Xu, S. Srinivasan and D. Merlin, Edible ginger-derived nanoparticles: A novel therapeutic approach for the prevention and treatment of inflammatory bowel disease and colitis-associated cancer, Biomaterials, 2016, 101, 321–340 CrossRef CAS PubMed.
- L. H. Peng, Y. H. Zhang, L. J. Han, C. Z. Zhang, J. H. Wu, X. R. Wang, J. Q. Gao and Z. W. Mao, Cell membrane capsules for encapsulation of chemotherapeutic and cancer cell targeting in vivo, ACS Appl. Mater. Interfaces, 2015, 7(33), 18628–18637 CrossRef CAS PubMed.
- L. Sercombe, T. Veerati, F. Moheimani, S. Y. Wu, A. K. Sood and S. Hua, Advances and challenges of liposome assisted drug delivery, Front. Pharmacol., 2015, 6, 286 Search PubMed.
- Q. Chen, M. Zu, H. Gong, Y. Ma, J. Sun, S. Ran, X. Shi, J. Zhang and B. Xiao, Tea leaf-derived exosome-like nanotherapeutics retard breast tumor growth by pro-apoptosis and microbiota modulation, J. Nanobiotechnol., 2023, 21(1), 6 CrossRef CAS PubMed.
- S. Sritharan and N. Sivalingam, A comprehensive review on time-tested anticancer drug doxorubicin, Life Sci., 2021, 278, 119527 CrossRef CAS PubMed.
- M. Zhang, B. Xiao, H. Wang, M. K. Han, Z. Zhang, E. Viennois, C. Xu and D. Merlin, Edible ginger-derived nano-lipids loaded with doxorubicin as a novel drug-delivery approach for colon cancer therapy, Mol. Ther., 2016, 24(10), 1783–1796 CrossRef CAS PubMed.
- A. Bonetto, J. E. Rupert, R. Barreto and T. A. Zimmers, The Colon-26 Carcinoma Tumor-bearing Mouse as a Model for the Study of Cancer Cachexia, J. Vis. Exp., 2016, 117, 54893 Search PubMed.
- J. Gallego-Jara, G. Lozano-Terol, R. A. Sola-Martínez, M. Cánovas-Díaz and T. de Diego Puente, A Compressive Review about Taxol®: History and Future Challenges, Molecules, 2020, 25(24), 5986 CrossRef CAS PubMed.
- H. Kawamoto, T. Yuasa, Y. Kubota, M. Seita, H. Sasamoto, J. M. Shahid, T. Hayashi, H. Nakahara, R. Hassan, M. Iwamuro, E. Kondo, S. Nakaji, N. Tanaka and N. Kobayashi, Characteristics of CD133(+) human colon cancer SW620 cells, Cell Transplant., 2010, 19(6), 857–864 Search PubMed.
- Q. Wang, X. Zhuang, J. Mu, Z. B. Deng, H. Jiang, L. Zhang, X. Xiang, B. Wang, J. Yan, D. Miller and H. G. Zhang, Delivery of therapeutic agents by nanoparticles made of grapefruit-derived lipids, Nat. Commun., 2013, 4(1), 1867 CrossRef PubMed.
- H. Nojima, T. Konishi, C. M. Freeman, R. M. Schuster, L. Japtok, B. Kleuser, M. J. Edwards, E. Gulbins and A. B. Lentsch, Chemokine Receptors, CXCR1 and CXCR2, Differentially Regulate Exosome Release in Hepatocytes, PLoS One, 2016, 11(8), e0161443 CrossRef PubMed.
- M. I. Amaro, J. Rocha, H. Vila-Real, M. Eduardo-Figueira, H. Mota-Filipe, B. Sepodes and M. H. Ribeiro, Anti-inflammatory activity of naringin and the biosynthesised naringenin by naringinase immobilized in microstructured materials in a model of DSS-induced colitis in mice, Food Res. Int., 2009, 42(8), 1010–1017 CrossRef.
- W. Dou, J. Zhang, A. Sun, E. Zhang, L. Ding, S. Mukherjee, X. Wei, G. Chou, Z. T. Wang and S. Mani, Protective effect of naringenin against experimental colitis via suppression of Toll-like receptor 4/NF-κB signalling, Br. J. Nutr., 2013, 110(4), 599–608 CrossRef CAS PubMed.
- W. J. Zhu, Y. Liu, Y. N. Cao, L. X. Peng, Z. Y. Yan and G. Zhao, Insights into Health-Promoting Effects of Plant MicroRNAs: A Review, J. Agric. Food Chem., 2021, 69(48), 14372–14386 CrossRef CAS PubMed.
- X. Lu, Q. Han, J. Chen, T. Wu, Y. Cheng, F. Li and W. Xia, Celery (Apium graveolens L.) Exosome-like Nanovesicles as a New-Generation Chemotherapy Drug Delivery Platform against Tumor Proliferation, J. Agric. Food Chem., 2023, 71(22), 8413–8424 CrossRef CAS PubMed.
- Y. Zhao, H. Tan, J. Zhang, B. Pan, N. Wang, T. Chen, Y. Shi and Z. Wang, Plant-Derived Vesicles: A New Era for Anti-Cancer Drug Delivery and Cancer Treatment, Int. J. Nanomed., 2023, 18, 6847–6868 CrossRef CAS PubMed.
- S. Lu, C. Zhang, J. Wang, L. Zhao and G. Li, Research progress in nano-drug delivery systems based on the characteristics of the liver cancer microenvironment, Biomed. Pharmacother., 2024, 170, 116059 CrossRef CAS PubMed.
- N. S. Orefice, R. Di Raimo, D. Mizzoni, M. Logozzi and S. Fais, Purposing plant-derived exosomes-like nanovesicles for drug delivery: patents and literature review, Expert Opin. Ther. Pat., 2023, 33(2), 89–100 CrossRef CAS PubMed.
- D. J. A. Crommelin, P. van Hoogevest and G. Storm, The role of liposomes in clinical nanomedicine development. What now? Now what?, J. Controlled Release, 2020, 318, 256–263 CrossRef CAS PubMed.
- Y. Malam, M. Loizidou and A. M. Seifalian, Liposomes and nanoparticles: nanosized vehicles for drug delivery in cancer, Trends Pharmacol. Sci., 2009, 30(11), 592–599 CrossRef CAS PubMed.
- E. Mahdipour, Beta vulgaris juice contains biologically active exosome-like nanoparticles, Tissue Cell, 2022, 76, 101800 CrossRef CAS PubMed.
- F. Şahin, P. Koçak, M. Y. Güneş, İ. Özkan, E. Yıldırım and E. Y. Kala, In Vitro Wound Healing Activity of Wheat-Derived Nanovesicles, Appl. Biochem. Biotechnol., 2019, 188(2), 381–394 CrossRef PubMed.
- Y. Cai, L. Zhang, Y. Zhang and R. Lu, Plant-Derived Exosomes as a Drug-Delivery Approach for the Treatment of Inflammatory Bowel Disease and Colitis-Associated Cancer, Pharmaceutics, 2022, 14(4), 822 CrossRef CAS PubMed.
- X. Wei, X. Li, Y. Zhang, J. Wang and S. Shen, Advances in the Therapeutic Applications of Plant-Derived Exosomes in the Treatment of Inflammatory Diseases, Biomedicines, 2023, 11(6), 1554 CrossRef CAS PubMed.
- O. M. Elsharkasy, J. Z. Nordin, D. W. Hagey, O. G. de Jong, R. M. Schiffelers, S. E. Andaloussi and P. Vader, Extracellular vesicles as drug delivery systems: Why and how?, Adv. Drug Deliv. Rev., 2020, 159, 332–343 CrossRef CAS PubMed.
- D. Subha, K. Harshnii, K. G. Madhikiruba, M. Nandhini and K. S. Tamilselvi, Plant derived exosome-like Nanovesicles: an updated overview, Plant Nano Biol., 2023, 3, 100022 CrossRef.
- X. Zhang, M. Cao, N. Diao, X. Cai, X. Chen, Y. Xiao, C. Guo and D. Chen, Plant exosome nanovesicles (PENs): green delivery platforms, Materials Horizons. Polymer, 2023, 10, 3879–3894 RSC.
- M. Barzin, A. M. Bagheri, M. Ohadi, A. M. Abhaji, S. Salarpour and G. Dehghannoudeh, Application of plant-derived exosome-like nanoparticles in drug delivery, Pharm. Dev. Technol., 2023, 28(5), 383–402 CrossRef CAS PubMed.
- N. P. Ly, H. S. Han, M. Kim, J. H. Park and K. Y. Choi, Plant-derived nanovesicles: Current understanding and applications for cancer therapy, Bioact. Mater., 2022, 22, 365–383 Search PubMed.
- D. J. Benjamin, The efficacy of surgical treatment of cancer - 20 years later, Med. Hypotheses, 2014, 82(4), 412–420 CrossRef PubMed.
- U. Anand, A. Dey, A. K. S. Chandel, R. Sanyal, A. Mishra, D. K. Pandey, V. De Falco, A. Upadhyay, R. Kandimalla, A. Chaudhary, J. K. Dhanjal, S. Dewanjee, J. Vallamkondu and J. M. Pérez de la Lastra, Cancer chemotherapy and beyond: Current status, drug candidates, associated risks and progress in targeted therapeutics, Genes Dis., 2022, 10(4), 1367–1401 CrossRef PubMed.
- Z. Zhou and M. Li, Targeted therapies for cancer, BMC Med., 2022, 20(1), 90 CrossRef PubMed.
- R. Baskar, K. A. Lee, R. Yeo and K. W. Yeoh, Cancer and radiation therapy: current advances and future directions, Int. J. Med. Sci., 2012, 9, 3 Search PubMed.
- K. Esfahani, L. Roudaia, N. A. Buhlaiga, S. V. Del Rincon, N. Papneja and W. H. Miller, A review of cancer immunotherapy: from the past, to the present, to the future, Curr. Oncol., 2020, 27(s2), 87–97 CrossRef PubMed.
- D. M. Pardoll, The blockade of immune checkpoints in cancer immunotherapy, Nat. Rev. Cancer, 2012, 12(4), 252–264 CrossRef CAS PubMed.
- J. Rieth and S. Subramanian, Mechanisms of Intrinsic Tumor Resistance to Immunotherapy, Int. J. Mol. Sci., 2018, 19(5), 1340 CrossRef PubMed.
- Y. Yang, G. H. Nam, G. B. Kim, Y. K. Kim and I. S. Kim, Intrinsic cancer vaccination, Adv. Drug Deliv. Rev., 2019, 151–152, 2–22 CrossRef CAS PubMed.
- J. Maia, S. Caja, M. C. Strano Moraes, N. Couto and B. Costa-Silva, Exosome-Based Cell-Cell Communication in the Tumor Microenvironment, Front. Cell Dev. Biol., 2018, 6, 18 CrossRef PubMed.
- G. H. Nam, Y. Choi, G. B. Kim, S. Kim, S. A. Kim and I. S. Kim, Emerging Prospects of Exosomes for Cancer Treatment: From Conventional Therapy to Immunotherapy, Adv. Mater., 2020, 32, 2002440 CrossRef CAS PubMed.
- S. Samanta, S. Rajasingh, N. Drosos, Z. Zhou, B. Dawn and J. Rajasingh, Exosomes: new molecular targets of diseases, Acta Pharmacol. Sin., 2018, 39(4), 501–513 CrossRef CAS PubMed.
- R. Dhar, B. Bhattacharya, D. Mandal, A. Devi and N. D. Thorat, Exosome-based cancer vaccine: A cutting-edge approach - Correspondence, Int. J. Surg., 2022, 108, 106993 CrossRef PubMed.
- R. Dhar, S. Gorai and A. Devi,
et al., Exosome: A megastar of future cancerpersonalized and precision medicine, Clin. Transl. Disc., 2023, 3, e208 CrossRef.
- R. Dhar, A. Devi, S. Patil and M. R. Tovani-Palone, Exosomes in cancer therapy: Advances and current challenges, Electron. J. Gen. Med., 2023, 20(5), em524 CrossRef PubMed.
- R. Dhar, S. Gorai, A. Devi, R. Muthusamy, A. Alexiou and M. Papadakis, Decoding of exosome heterogeneity for cancer theranostics, Clin. Transl. Med., 2023, 13(6), e1288 CrossRef CAS PubMed.
- Y. Wang, Y. Wei, H. Liao, H. Fu, X. Yang, Q. Xiang and S. Zhang, Plant Exosome-like Nanoparticles as Biological Shuttles for Transdermal Drug Delivery, Bioengineering, 2023, 10(1), 104 CrossRef CAS PubMed.
- L. Xiao-Jie, X. Hui-Ying, K. Zun-Ping, C. Jin-Lian and J. Li-Juan, CRISPR-Cas9: a new and promising player in gene therapy, J. Med. Genet., 2015, 52(5), 289–296 CrossRef PubMed.
- H. A. Dad, T. W. Gu, A. Q. Zhu, L. Q. Huang and L. H. Peng, Plant Exosome-like Nanovesicles: Emerging Therapeutics and Drug Delivery Nanoplatforms, Mol. Ther., 2021, 29(1), 13–31 CrossRef CAS PubMed.
- J. Mondal, S. Pillarisetti, V. Junnuthula, M. Saha, S. R. Hwang, I. K. Park and Y. K. Lee, Hybrid exosomes, exosome-like nanovesicles and engineered exosomes for therapeutic applications, J. Controlled Release, 2023, 353, 1127–1149 CrossRef CAS PubMed.
- A. Sarasati, M. H. Syahruddin, A. Nuryanti, I. D. Ana, A. Barlian, C. H. Wijaya, D. Ratnadewi, T. D. K. Wungu and H. Takemori, Plant-Derived Exosome-like Nanoparticles for Biomedical Applications and Regenerative Therapy, Biomedicines, 2023, 11(4), 1053 CrossRef CAS PubMed.
- H. A. Dad, T. W. Gu, A. Q. Zhu, L. Q. Huang and L. H. Peng, Plant Exosome-like Nanovesicles: Emerging Therapeutics and Drug Delivery Nanoplatforms, Mol. Ther., 2021, 29(1), 13–31 CrossRef CAS PubMed.
- J. Gopinathan and I. Noh, Recent trends in bioinks for 3D printing, Biomater. Res., 2018, 22, 1–15 CrossRef PubMed.
- R. T. Morales and J. Ko, Future of Digital Assays to Resolve Clinical Heterogeneity of Single Extracellular Vesicles, ACS Nano, 2022, 16(8), 11619–11645 CrossRef CAS PubMed.
Footnote |
† Equally contributing authors. |
|
This journal is © The Royal Society of Chemistry 2024 |
Click here to see how this site uses Cookies. View our privacy policy here.