DOI:
10.1039/D4AN01375A
(Paper)
Analyst, 2025,
150, 989-997
Identification and characterization of microplastics released during the actual use of disposable cups using laser direct infrared imaging†
Received
23rd October 2024
, Accepted 13th January 2025
First published on 14th January 2025
Abstract
Disposable plastic cups are commonly used as beverage containers. This study investigated the characteristics of microplastics (MPs) released from plastic cups under everyday conditions and assessed the emission of MPs from disposable cups, including polypropylene (PP) and polystyrene (PS). We systematically examined the impact of material, temperature, and liquid type on the release of MPs using laser direct infrared (LDIR) spectroscopy. The findings indicated that all cups released irregularly shaped MPs smaller than 50 μm. When filled with water at 95 °C for 15 min, the average MP concentrations in the PP and PS cups were 1340 and 980 particles per L, respectively. The disposable plastic cups exhibited higher average MP concentrations at elevated temperatures. Similar profiles were observed for both types of cups, with the MP release capacity at 95 °C being 1.5 times higher than that at 50 °C. Low temperatures facilitated the self-release of MPs from disposable cups. Furthermore, reusable cups released fewer MPs than single-use cups. The study estimated that regular use of a cup three times a day could lead to the unintentional ingestion of 294–402 MPs per day. These results provide a basis for evaluating possible health hazards associated with human exposure to MPs.
Introduction
Microplastics (MPs), a new recognized type of contaminant in food and supply chains, have garnered widespread global attention.1 Comprehensive evidence indicates that MPs can be ingested intentionally or unintentionally via respiration, skin contact, or dietary routes. Traces of MPs have been detected in water2 and various foods, including seafood,3 fruits and vegetables.4,5 Furthermore, MPs have been identified in human fluids and6 feces7 and even in lungs,8 gallstones,9 kidneys,10 and stomach,11 indicating potential human exposure through dietary intake. Studies have demonstrated that MPs exhibit cytotoxicity towards various human cell lines, including liver and kidney cells,12,13 gastrointestinal cells,14 spermatogenic epithelial cells,15 myocardial cells,16 lung cells,17 and nerve cells.18 Recent research has further confirmed that the accumulation of MPs in multiple mouse organs poses potential health risks, including liver damage,19 pulmonary fibrosis,20 gastrointestinal toxicity,21 oxidative stress,22 and inflammatory injury.23
As common containers for cold drinks or tea, disposable plastic cups are primarily categorized into polypropylene (PP), polyethylene (PE), polystyrene (PS), and other materials. The demand from the takeaway catering industry has significantly contributed to their widespread use. Numerous studies have concentrated on the quantity of MP released from PE paper cups under diverse service conditions, such as temperature, exposure time, and liquid composition.24–27 High temperature, vigorous shaking, and prolonged exposure can induce MP release owing to the fragmentation of the PE film. This is a serious concern, as a significant amount of MP can be directly ingested by humans. PP and PS are also extensively utilized in catering and takeout domains. However, only two studies have reported the MPs released from PP and PS cups, neither of which performed component identification or analyzed particle size. Zhang et al.28 found that the MP quantity released ranged from 1000 to 5000 particles per L when soaked at 95 °C for 20 min, while Yetim et al.29 identified a similar level. These findings provide valuable insights into MP release from plastic cups; however, there remains a paucity of studies on MP release from PP and PS cups. To date, the amount of MPs released from PP and PS cups during the use or reuse of cold beverages, among other conditions, remains unclear. Additionally, the characteristics (size, type, etc.) of MPs released from PP and PS cups have not been thoroughly investigated. Understanding the MP characteristics ingested by humans through plastic cups is crucial for assessing potential health risks.
Several techniques have been devised for MP detection. Pyrolysis-gas chromatography/mass spectrometry (Pyr-GC/MS),34 can provide detailed information on the chemical composition, but does not yield data on particle count or size. The intricate preparation and time-consuming further constrain its applications. Raman and Fourier transform infrared spectroscopy (FTIR) have also been extensively employed in MP analysis.30 Raman can offer distinctive fingerprints with high sensitivity; yet, it is less efficient in detecting complex samples and failing to comprehensively capture the MP contamination. FTIR utilizes the IR absorption of polymers to determine the chemical composition, particularly combined with an in-built polymer spectral library but is limited by its sluggish detection speed and low spatial resolution. Notably, the methods mentioned above typically rely on a stereomicroscope for quantitative analysis, with a false positive rate of 37% for <50 μm MPs. To surmount these challenges, innovative technologies such as laser direct infrared (LDIR) imaging have been developed.31 LDIR system employs a fast-tunable quantum cascade laser as the light source, linked to a high-speed scanning imaging system, that is capable of simultaneously scanning multiple particles, providing information on chemical composition and spatial distribution. It can generate reflection spectra within merely 1 s, presenting the advantages of high speed and resolution, far surpassing Pyr-GC/MS or Raman. Compared with FTIR, LDIR can not only identify polymer types but also determine the number, size, and morphology of particles, significantly enhancing the detection throughput. Additionally, the automated LDIR system mitigates the demand for cumbersome sample preparations, rendering it an effective alternative to FTIR. Hence, in contrast to traditional techniques, LDIR exhibits immense potential for MP detection.
This study evaluated the MP release characteristics of disposable plastic cups used daily (Fig. 1). We used six batches of commercial disposable plastic cups, including PP and PS cups, to characterize the size, number, and composition of MPs and other particulate fragments released during simulated use. We first accurately assessed potential MP contamination in this experiment using LDIR imaging. Then, we systematically studied, temperature, usage frequency, and liquid composition (e.g., pure water or tea) on MP release from disposable plastic cups. Our preliminary research results provide reference data for further assessment of the risk of children and adults ingesting pollutants in their diet.
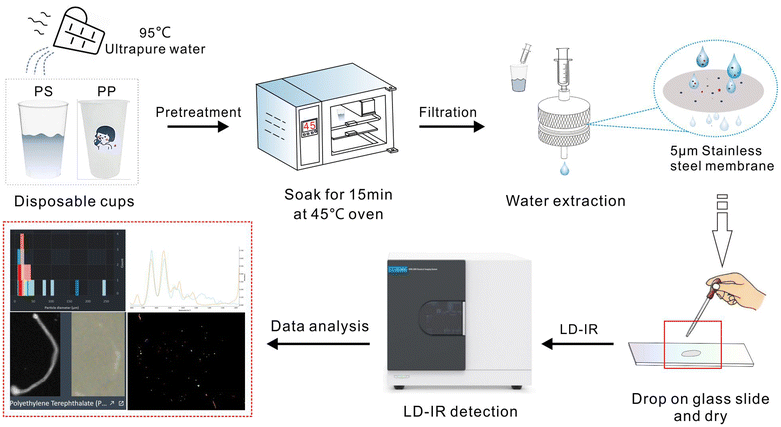 |
| Fig. 1 LDIR-based MP sample preparation, detection, and classification. | |
Materials and methods
Chemicals and materials
Six sets of transparent disposable plastic cups (PP and PS) were purchased from supermarkets. The selected cups had a volume of 200 mL; the tea was purchased online; ethanol was purchased from Sigma Aldrich; water was generated by a Milli-Q system; stainless-steel filters (SSF, pore size 5 μm), and glass syringes were purchased from De filter company. PS-MPs (50 μm) were provided by Yiyuan Biotechnology Co, Ltd.
MPs release experiment
To investigate the effect of temperature on MP release, the experimental procedure was repeated using water at 50 and 95 °C. Ice packs were placed in a water bath to cool the water to 4 °C, simulating cold beverages; to explore the effect of usage frequency on MP release, the experiment was repeated thrice at 95 °C. A certain number of tea leaves were added to the cups, followed by adding 95 °C water and conducting the aforementioned experiments.
Before use, the plastic cups were rinsed with deionized water and then filled with 50 mL at 95 °C. Cups were sealed with aluminum foil to prevent contamination with MPs. Plastic cups were placed on a horizontal rotary shaker and shaken at 100 rpm for 5 min, followed by incubation at 45 °C for 15 min.
MPs collection
An SSF was used to filter MPs from the samples. Before filtration, the SSF was washed thrice with filtered ethanol. Subsequently, the SSF membrane was held using a pair of tweezers, and the particles gathered on the SSF membrane were eluted with ethanol. The sample solution was then collected in a glass vial. The collected sample solution was dispensed onto a low-E glass slide, and placed on an LDIR instrument for detection.
LDIR imaging
Agilent 8700 LDIR imaging was suitable for sample analysis on Mirr-IR slides. Disperse particles in ethanol were dropped onto a Mirr-IR slide. First, the instrument obtains a clear image using a large-field camera (optical resolution of approximately 24 μm). Next, the system boasts single-wavelength precision (spectral resolution of 8 cm−1) for measuring, counting, locating, and analyzing particles in the image. Subsequently, the IR spectrum of each particle was measured in reflection mode. Finally, the obtained IR spectra were automatically compared with the reference library using Clarity software (version 1.5.58) to identify the chemical composition. Particles were considered acceptable when the IR spectral match with the standard exceeded 70%. The reference spectrum library includes polymers and other materials such as chitin, coal, and natural polyamides (PA). In addition, a selected visible light image of the target particle can be obtained under the objective, with a visible light resolution of 1 μm.
Quality assurance and quality control
Potential contamination.
Plastic cups at the centre of each pile were used in the study. During sample preparation and experimentation, we wore pure cotton laboratory coats and single-use nitrile gloves, with the cuffs of the laboratory coat secured inside the gloves. All glassware was rinsed thrice with filtered water and ethanol, and the MP in ethanol and Mirr IR low radiation glass was negligible (Fig. S1†). Each experiment was repeated thrice, and the data was taken as the average of the three repetitions.
Selective capabilities of LDIR imaging.
The polymer type identification via LDIR imaging was validated using PS reference materials (Fig. S2†).
Recovery assessment.
By adding PS standard samples of known concentration and size to blank samples, the LDIR detection method was adopted for analysis. The recovery test results revealed that for microplastics within a specific size range, the recovery rate reached 85%, indicating the relatively good accuracy of this method (Table S1†). Furthermore, due to the pre-filtered Milli-Q water and the strengthened procedural blank, the significance of quality control measures in MP experiments was accentuated.
Statistical analysis.
One-way ANOVA was used to analyze the statistical differences in the MP released under different conditions, with statistical significance set at p < 0.05 (F value > F critical value). The relevant analysis was completed using Excel ANOVA 2016. Sankey and Chord diagrams were used to compare and analyze the relationships between different variables, and the correlation analysis was completed using Origin 2021.
Determination of daily intake quantities.
The daily consumption of MPs released from single-use plastic cups was determined by using the estimated dietary intake (EDI) data obtained from previous research studies:28 | 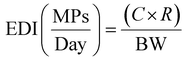 | (1) |
where, C: median value of the concentrations of MPs in food matrix (w g−1), R: rate of intake (g day−1), BW: average body weight (kg).
According to the equation provided, the daily MP exposure from single-use cups was determined as follows:
| 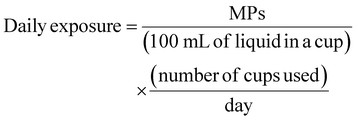 | (2) |
Results
Identification of MPs during the experimental process
To minimize the pollution of disposable plastic cup samples in each step, multiple filtration procedures were conducted on water, followed by LDIR detection for the presence of MPs (Fig. 2a; Fig. S3†). Unfiltered water contains polyamide (PA), polyvinyl chloride (PVC), vinyl chloride (VC), and non-MP, natural PA, chitin, etc. (Fig. 2b and c), indicating the necessity of pre-filtered water for quality control and reflecting that LDIR imaging can effectively distinguish non-plastic polymers. A significant reduction in MP abundance was observed after each round of filtration (Fig. 2d). After six rounds of filtration, only five particles, mainly composed of PA, PVC, and VC (Fig. 2e), remain in the water sample. This process effectively removed particulate matter with a diameter exceeding 30 μm (Fig. 2f).
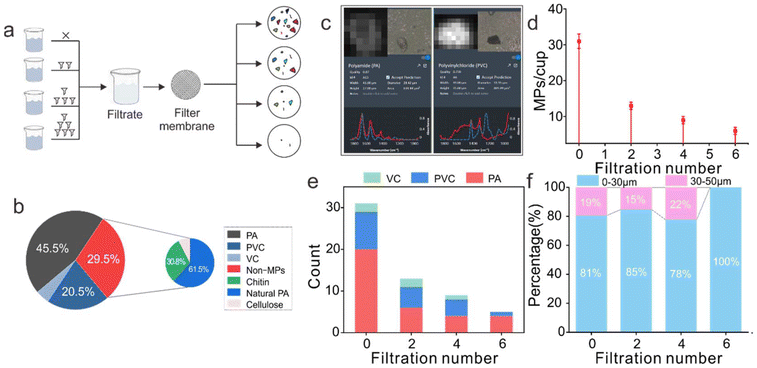 |
| Fig. 2 (a) Schematic diagram of the filtration process; (b) MP types in unfiltered water samples; (c–f) Representative IR imaging (c), quantity (d), polymer type (e), and size distribution (f) of MPs in water samples after varying filtration durations. | |
MPs released from plastic cups
Capacity of the released MPs.
Six batches of disposable plastic cups, including PP and PS cups, were meticulously examined at 95 °C to investigate the release of MPs in water. As shown in Fig. 3a, the MP released in each test cup was significantly higher than that in the control sample (p < 0.05), which clearly showed that disposable plastic cups had a high risk of MP release under specific conditions. It is worth noting that the release of MPs from PS and PP cups fell within the ranges of 920–2220 and 480–1440 particles per L, respectively, reflecting the difference in MP release quantity between different materials or brands of cups.
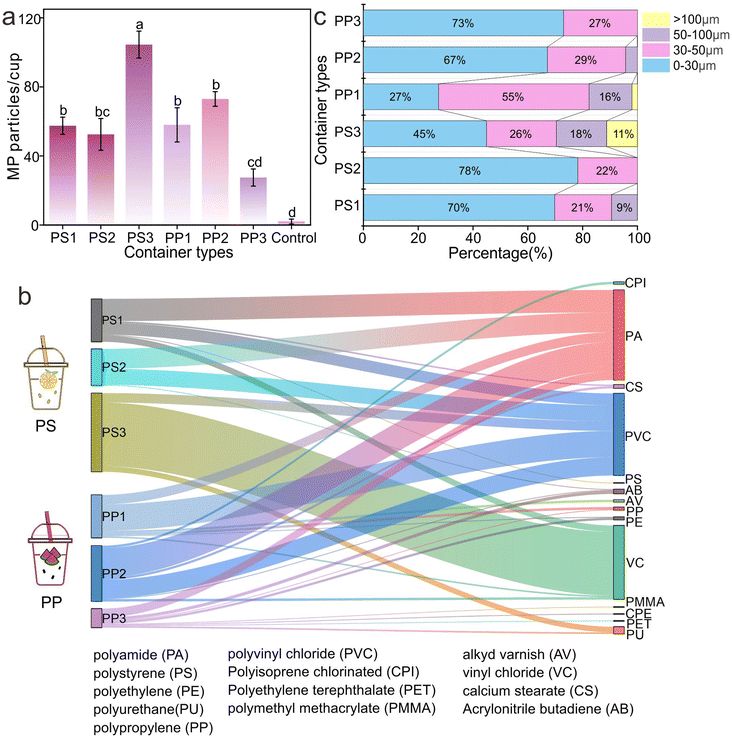 |
| Fig. 3 Release capacities (a), components (b), and size distribution (c) of MPs released from plastic cups containing 95 °C water. Bars and error bars represent the mean concentration and standard deviation, respectively. Different letters indicate significant differences (p < 0.05). | |
Specifically, the average release of the PS cup is higher than that of the PP cup,32 which is closely related to the physical and chemical properties of the two materials.33 PS typically exhibits high brittleness and low crystallinity, which may readily facilitate the MP release under elevated temperatures.29 Nevertheless, the observation does not imply that the PP cup is a safe alternative; though it demonstrates a comparatively low release level under the experimental condition. Compared with the reported MP abundance in drinking water (10−3 to 103 particles per L), a worrying situation is that the MPs released from plastic cups used in daily life may significantly increase human exposure to MPs.34 This means that even if people only drink water with disposable plastic cups, they may unconsciously ingest a large number of MPs.
Identification of the released MPs.
Visible in the membrane were particles of diverse shapes and sizes, including granular, sheet-like, and filamentous forms (Fig. S4†). Simultaneously, LDIR automatically obtained the IR spectra of the particles and matched them against the “microplastics library” to determine the chemical composition of the particles released in this study. The IR spectra of some particles resembled those of the corresponding polymer materials in disposable cups, exhibiting similar characteristic peaks (Fig. S5†). The IR spectra of the particles released from the PP and PS cups within the 1200–1800 cm−1 region exhibited typical adsorption bands, namely those for PP and PS, thereby explicitly confirming the possibility of MP particles leaching from disposable cups into the beverages.
The types of MPs released from plastic cups produced by different manufacturers are different (Fig. 3b). The MPs released from the PS cup were mainly composed of PA, PVC, and VC, and also contained a small number of PS, calcium stearate (CS), polymethylmethacrylate (PMMA), and acrylonitrile butadiene (AB). This ingredient diversity indicated the complexity of raw materials and the possible introduction of a variety of impurities in the production process of plastic cups.35 For example, PA can be derived from the need to enhance the strength of plastics; PVC and VC may be related to the basic composition or additives of plastics.36 A small number of PS, CS, PMMA, and AB may be additives added in the production process to improve the specific properties of plastic cups.37,38 MPs released from the PP plastic cup are mainly composed of PA and PVC and also contain a small amount of PP, AB, alkyd varnish (AV), chlorinated polyisoprene (CPI), etc. The difference in MP composition, on the one hand, reflects the difference in the characteristics of PP and PS plastic materials, on the other hand, it also reflects the different treatment of additives and other aspects.
Based on the above results, it can be clearly seen that in the process of processing, production and packaging of plastic cups, in addition to the particles from the plastic material of the cup itself, the presence of other particles also shows additional MP exposure risk.33 This means that we should not only focus on the main materials of plastic cups but also pay enough attention to various impurities and additives that may be introduced in the production process. These extra MP particles may come from impure raw materials, the wear and tear of production equipment, the residue of processing aids, or the pollution of packaging materials.
Features of the released MPs.
The size distribution of the MPs released from disposable cups is shown in Fig. 3c. For both types of cups, the size distribution of the released MP particles was within the range of 0–30 μm (PS: 69.86%, 78.7%, and 44.9%; PP: 27.7%, 67.6%, and 61.8%).
Ranjan et al. employed fluorescence microscopy to characterize the size range of MPs released from PE paper cups, which ranged from 26 to 765 μm.26 In this study, MPs with sizes <30 μm predominated, whereas MPs with sizes >50 μm were relatively scarce. It is noteworthy that previous studies have shown that the gastrointestinal tract can absorb MPs with particle size <10 μm and MPs with particle size >130 μm can even enter the circulatory system.39 It can be seen that the MPs released from disposable cups, in this study, are almost all within the particle size range that can be absorbed by the human body, which undoubtedly poses a considerable threat to human health. This means that when using disposable plastic cups, the MPs released are likely to be ingested by the human body and accumulated in the body, which may lead to a series of health problems.
Effects of the use conditions on MP release
Temperature.
This study focused on the effect of temperature, a key factor, on MP release. Different from the common studies focusing on MP release at high temperatures, this time we comprehensively and deeply carried out MP release at low temperatures (4 °C) and relatively high temperatures (50 °C). As shown in Fig. 4a, the average number of MPS released at 4 °C was about 60 particles per cup, which was not significantly different from that at 95 °C (p > 0.05). The number of MPs released at 50 °C was significantly lower than that at 95 °C (p < 0.05).25 Compared with the temperature of 50 °C, the low temperature of 4 °C may have a significant impact on the stability of plastics.
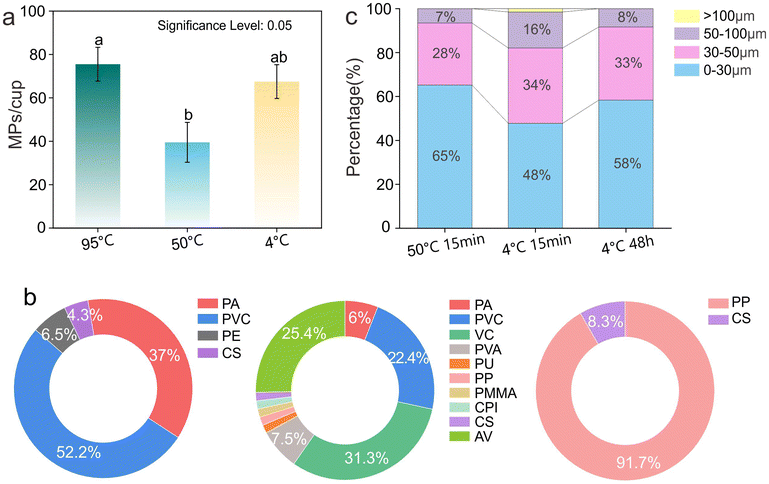 |
| Fig. 4 Quantity (a), chemical composition (b), and size distribution (c) of MPs released from PP plastic cups with different temperatures. | |
A similar situation was observed in the two test cups. The release of MP at 95 °C was two to four times that at 50 °C, which further confirmed the key role of temperature in the release of MPs (Fig. S6†). It can be seen that temperature is an important variable that cannot be ignored when evaluating the risk of MP release from plastic cups. In low or high-temperature environments, especially when it is close to or reaches the performance change threshold of plastics, the risk of MP release increases significantly.
Subsequently, the chemical composition of MPs released at 4 °C and 50 °C was analyzed. As shown in Fig. 4b, the primary types of MPs released from the PP plastic cups were PA, PVC, and PE, with small MPs (<30 μm) being the dominant fraction at 50 °C. Similar trends were observed for PS plastic cups (Fig. S7†). Low temperature at 4 °C accelerated the release of a variety of MPs including PA, PVC, VC, AV, PVA, PP, and CPI (Fig. S8†), of which the first four types were the main components, and the particle size was mainly concentrated in <30 μm (Fig. 4c). Prolonged low-temperature exposure (4 °C, 48 h) resulted in the release of small PP particles (0–30 μm) consistent with the plastic cup material. This finding aligns with the study by Chai et al., which demonstrated that the MPs in ice can undergo obvious oxidative degradation in a short time (36 h).40
To this end, AFM technology was employed to characterize the micromorphology of the plastic cup before and after 48 h treatment at 4 °C (Fig. S9†). The inner wall of the untreated cup was relatively smooth and flat. Small protrusions appear after treatment, which may indicate that low temperature impacts the surface characteristics of the plastic cup, thereby influencing MP release.28 Collectively, these results indicate that specific types of MPs are released from plastic cups under different temperature conditions. In conclusion, temperature not only affects the quantity of MPs released but also plays a critical role in determining their chemical composition and particle size distribution, providing an important basis for understanding the mechanisms governing MP release.
Usage frequency.
Fig. 5 summarizes the characteristics of MPs released after one or thrice uses. At the third reuse, the number of MPs released was approximately 43 particles per cup, which was about half of that at the first use (Fig. 5a), with a statistically significant difference (P < 0.05). This trend was consistent with the release characteristics of MPs identified in a 21-day study using PP feeding bottles reported in the literature,41 further validating the general pattern of MP release from plastic products during multiple uses.
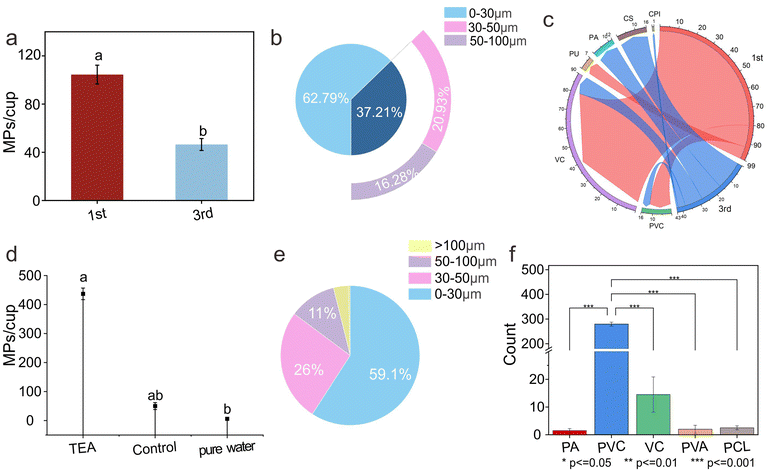 |
| Fig. 5 Quantity, size distribution, and chemical composition of MPs released from disposable cups under different usage frequencies (a–c) or liquids (d–f). | |
Subsequent in-depth analysis of the chemical composition and size distribution of MPs revealed additional critical information. It was found that MPs released after repeated use were predominantly small particles (0–30 μm) (Fig. 5b). The substantial release of such small particles may pose more far-reaching potential hazards. Smaller particle sizes mean that MPs can more easily penetrate biological tissues, potentially leading to more complex and severe health issues.42 In addition to the common PA and PVC, repeated use resulted in high levels of VC release (Fig. 5c). As a harmful substance, the massive release of VC undoubtedly poses a continuous threat to human health. Furthermore, the release of endocrine disruptors and stabilizers (CS) during use cannot be overlooked.37 Endocrine disruptors may interfere with the function of the normal endocrine system, leading to hormonal imbalances and subsequent physiological and metabolic disorders. The release of stabilizers may also introduce potential risks due to their unknown biological toxicity.
This research demonstrates that the release of MPs from disposable cups is a continuous process. This process does not simply follow a linear trend with increased use frequency but involves complex changes in chemical composition and particle size distribution. Although the quantity of the MPs released decreases with repeated use, the chemical composition of the released MPs becomes more complex, with an increased proportion of small particles and harmful substances, thereby posing a continuous and evolving threat to human health.
Composition of the liquid.
Disposable plastic cups are commonly used in daily life to hold a variety of beverages. To investigate the correlation between the characteristics of released MPs and the liquid contained, a popular tea beverage (pH 5.5) was selected to study the release of MPs. The results showed that although there was no statistically significant difference in MP release between the tea beverage and the control group, the quantity increased,43 reaching an average of 437 particles per L (Fig. 5d). This phenomenon indicated that under specific environmental conditions, the composition of the liquid can influence the material properties of disposable cups. This finding is consistent with previous research reports indicating that PS plastic cups, due to their chemical and mechanical stability, generally resist interactions with liquid components. However, the results also suggest that the potential impact of liquid composition cannot be ignored.
According to the analysis of the size and chemical composition of MPs (Fig. 5e and f), the PS plastic cup primarily released MPs in the form of PA and PVC after adding the tea beverage, with particle sizes ranging from 0–30 μm. Notably, smaller MPs have a larger specific surface area, which means they can have more extensive contact with the external environment, making it easier for them to enter organisms and pose a potential health threat to humans.12–14 In conclusion, while the liquid composition did not cause significant changes in the MP release quantity, it did exert a certain influence on the release characteristics. When evaluating the safety of plastic cups, it is essential to consider not only the material characteristics of the cups but also the potential effects of the liquid composition. This comprehensive understanding is crucial for reducing the potential risks of plastic products to human health.
What number of MPs are used for single-use cups?.
When consuming 100 mL of hot water from a disposable cup, it is estimated that 98–134 particles are ingested daily. The data highlights that in seemingly routine drinking behavior, people may unknowingly be exposed to a significant number of MPs. It is worth noting that the intake amount is derived from specific experimental conditions and calculation methods; actual exposure may vary due to factors such as cup material, temperature, and liquid type. Further analysis indicates that if disposable cups are used three times a day, the daily intake increases to 294–402 particles. This elevated intake serves as a warning that frequent use of disposable cups may lead to a substantial increase in MP exposure levels. This not only poses potential health risks to individuals but also reflects the cumulative environmental and health risks associated with the widespread use of disposable cups on a macro scale. The results obtained in this study (Table S3†) align with previous studies on MP abundance at the micron scale. On one hand, its consistency verifies the reliability of this method. On the other hand, it underscores the fact that despite differences in research perspectives and methodologies, common issues regarding MP release and exposure in disposable plastic cups persist.
However, it is essential to acknowledge the limitations of this study. Firstly, the experimental scenarios for disposable cup usage were relatively limited, failing to fully capture the diverse real-life usage situations. Secondly, while LDIR technology was employed to identify MPs within the 20–500 μm range, the analysis of MPs smaller than 20 μm remains insufficient. A more comprehensive analysis, potentially incorporating techniques such as SEM, is required for a thorough understanding. In summary, this study has preliminarily revealed the release of MP during the use of disposable cups, but there are still many issues that need further in-depth research, to provide a more comprehensive and scientific basis for reducing the environmental and health risks caused by disposable plastic products.
Conclusions
This study used LDIR to automatically, qualitatively, and quantitatively discern the release of MPs from disposable plastic cups during daily usage. MPs were detected in the water collected from various plastic cups. The release of MPs from PS and PP cups was 920–2220 and 480–1440 particles per L, respectively. The size distribution of the released MP particles was centered within the range of 0–30 μm, with fewer particles exceeding 50 μm in size. Both high (95 °C) and low temperatures (4 °C) expedited the release of MPs from disposable plastic cups. The results revealed that the average MP concentration released from disposable plastic cups used for the third time was approximately half that of the first use, and tea beverages had a higher propensity to promote MP release than pure water. In this study, we discovered that MP fragments discharged from cups are a matter of concern as a direct source of human contact.
Abbreviations
PA | Polyamide |
PS | Polystyrene |
PE | Polyethylene |
PU | Polyurethane |
PP | Polypropylene |
PVC | Polyvinyl chloride |
CPI | Polyisoprene chlorinated |
PET | Polyethylene terephthalate |
PMMA | Polyethylene methacrylate |
PVA | Polyvinyl alcohol |
AV | Alkyd varnish |
VC | Vinyl chloride |
CS | Calcium stearate |
AB | Acrylonitrile butadiene |
Author contributions
Conceptualization, Shanshan Du; funding acquisition, Shanshan Du; investigation, Ziyan Liu, Lei Wu and Fangbiao Tao; methodology, Shanshan Du; software, Fangbiao Tao; visualization, Shanshan Du; writing – original draft, Shanshan Du; writing – review & editing, Shanshan Du.
Data availability
The data supporting this article have been included as part of the ESI.†
Conflicts of interest
There are no conflicts to declare.
Acknowledgements
This research was funded by the Natural Science Research Project of Anhui Educational Committee, grant number 2023AH050605. Thanks for the technical support from the Center for Preventive Medicine Research and the Center for Scientific Research of Anhui Medical University.
References
- C. G. Eze, C. E. Nwankwo, S. Dey, S. Sundaramurthy and E. S. Okeke, Environ. Chem. Lett., 2024, 22, 1889–1927 CrossRef CAS.
- W. Xia, Q. Rao, J. Liu, J. Chen and P. Xie, J. Hazard. Mater., 2024, 480, 135998 CrossRef CAS PubMed.
- L. Nalbone, F. Giarratana, M. Genovese and A. Panebianco, Mar. Pollut. Bull., 2024, 206, 116739 CrossRef CAS PubMed.
- Y. Bai, Y. Chen, Y. Song, R. Bai, W. He, M. Zhao, J. Zhang, W. Zhang, Y. Zhang and S. Dong, J. Hazard. Mater., 2024, 477, 135310 CrossRef CAS PubMed.
- A. Sherman, L. E. Hämmerle, E. Ben Mordechay, B. Chefetz, T. Hüffer and T. Hofmann, Front. Environ. Sci., 2024, 12, 1384506 CrossRef.
- Y. Yang, F. Zhang, Z. Jiang, Z. Du, S. Liu, M. Zhang, Y. Jin, Y. Qin, X. Yang and C. Wang, Part. Fibre Toxicol., 2024, 21, 34 CrossRef CAS PubMed.
- C. Hartmann, I. Lomako, C. Schachner, E. El Said, J. Abert, V. Satrapa, A.-M. Kaiser, H. Walch and S. Köppel, Sci. Total Environ., 2024, 951, 175825 CrossRef CAS PubMed.
- A. Ö. Alpaydin, E. S. Uçan, M. Köktürk, M. Atamanalp, Ç. Kalyoncu, S. Yiğit, A. Uçar, G. Ö. Şimşek, K. C. Tertemiz and V. Karaçam, Environ. Pollut., 2024, 363, 125054 CrossRef.
- D. Zhang, C. Wu, Y. Liu, W. Li, S. Li, L. Peng, L. Kang, S. Ullah, Z. Gong and Z. Li, J. Hazard. Mater., 2024, 467, 133631 CrossRef CAS PubMed.
- S. Massardo, D. Verzola, S. Alberti, C. Caboni, M. Santostefano, E. E. Verrina, A. Angeletti, F. Lugani, G. M. Ghiggeri and M. Bruschi, Environ. Int., 2024, 184, 108444 CrossRef CAS PubMed.
- S. Özsoy, S. Gündogdu, S. Sezigen, E. Tasalp, D. A. Ikiz and A. E. Kideys, Forensic Sci. Int., 2024, 364, 112246 CrossRef.
- P. Li, D. Miyamoto, T. Adachi, T. Hara, A. Soyama, H. Matsushima, H. Imamura, K. Kanetaka, W. Gu and S. Eguchi, Ecotoxicol. Environ. Saf., 2024, 288, 117330 CrossRef CAS PubMed.
- R. Hong, Y. Shi, Z. Fan, Y. Gao, H. Chen and C. Pan, Toxicology, 2024, 509, 153996 CrossRef CAS PubMed.
- W. Shaoyong, L. Sun, Y. Gan, H. Jin, W. Wang, L. Yin, Y. Wang and M. Jin, ACS Nano, 2024, 18, 28849–28865 CrossRef CAS PubMed.
- Q. Fang, C. Wang and Y. Xiong, Chem. – Biol. Interact., 2024, 396, 111043 CrossRef CAS.
- P. Lin, X. Tong, F. Xue, C. Qianru, T. Xinyu, L. Zhe, B. Zhikun and L. Shu, Toxicology, 2022, 480, 153338 CrossRef CAS PubMed.
- M. S. Jeon, J. W. Kim, Y. B. Han, M. H. Jeong, H. R. Kim, H. S. Kim, Y. J. Park and K. H. Chung, Environ. Toxicol., 2023, 38, 359–367 CrossRef CAS PubMed.
- S. Liu, Y. He, J. Yin, Q. Zhu, C. Liao and G. Jiang, J. Hazard. Mater., 2024, 134054 CrossRef CAS PubMed.
- M. M. Tiao, J. M. Sheen, I. C. Lin, M. Khwepeya and H. R. Yu, Int. J. Mol. Sci., 2023, 24, 13457 CrossRef CAS PubMed.
- J. Lei, Q. Ma, X. Ding, Y. Pang, Q. Liu, J. Wu, H. Zhang and T. Zhang, Environ. Chem. Lett., 2024, 1–29 CAS.
- X. Wang, K. Deng, P. Zhang, Q. Chen, J. T. Magnuson, W. Qiu and Y. Zhou, Sci. Total Environ., 2024, 170962 CrossRef CAS PubMed.
- J. Fu, L. Zhang, K. Xiang, Y. Zhang, G. Wang and L. Chen, Biomater. Sci., 2023, 11, 4298–4307 RSC.
- Z. Liu, Q. Zhuan, L. Zhang, L. Meng, X. Fu and Y. Hou, J. Hazard. Mater., 2022, 424, 127629 CrossRef CAS PubMed.
- T. Zhang, J. L. Hu, Y. Duan, S. Chen, D. Li, B. Dong, M. Z. Mo, J. Wang, J. G. Zheng and H. N. Zhong, Food Control, 2023, 153, 109901 CrossRef CAS.
- G. Zhou, Q. Wu, P. Tang, C. Chen, X. Cheng, X. F. Wei, J. Ma and B. Liu, J. Hazard. Mater., 2023, 441, 129982 CrossRef CAS.
- V. P. Ranjan, A. Joseph and S. Goel, J. Hazard. Mater., 2021, 404, 124118 CrossRef CAS PubMed.
- J. Lee, J. Kong and S. Jeong, Microchem. J., 2024, 196, 109629 CrossRef CAS.
- H. Chen, L. Xu, K. Yu, F. Wei and M. Zhang, Sci. Total Environ., 2023, 854, 158606 CrossRef CAS PubMed.
- S. Akbulut, P. K. Akman, F. Tornuk and H. Yetim, Foods, 2024, 13, 1564 CrossRef CAS PubMed.
- A. Käppler, D. Fischer, S. Oberbeckmann, G. Schernewski, M. Labrenz, K.-J. Eichhorn and B. Voit, Anal. Bioanal. Chem., 2016, 408, 8377–8391 CrossRef PubMed.
- P. J. I. Chandran and S. Veerasingam, Talanta, 2024, 127284 Search PubMed.
- F. Du, H. Cai, Q. Zhang, Q. Chen and H. Shi, Hazard. Mater., 2020, 399, 122969 CrossRef CAS PubMed.
- Y. Y. Hee, K. Weston and S. Suratman, Food Packag. Shelf Life, 2022, 32, 100826 CrossRef CAS.
- L. Yang, S. Kang, X. Luo and Z. Wang, Environ. Pollut., 2024, 123857 CrossRef CAS PubMed.
- V. Shruti and G. Kutralam-Muniasamy, Trends Anal. Chem., 2024, 170, 117421 CrossRef CAS.
- F. E. Borrelli, J. Vinyl Addit., 2007, 13, 138–142 CrossRef CAS.
- L. D. V. E. León, V. A. Escócio, L. L. Y. Visconte, A. M. F Sousa, A. L. N. Silva and E. B. A. V. Pacheco, eXPRESS Polym. Lett., 2025, 19, 76–93 CrossRef.
- B. Kumar, S. Kumar and J. D. Barma, Phys. Scr., 2024, 100, 015972 CrossRef.
- X. Zhang, Z. Jin, M. Shen, Z. Chang, G. Yu, L. Wang and X. Xia, Fish Shellfish Immunol., 2022, 125, 276–284 CrossRef CAS PubMed.
- C. Tian, J. Lv, W. Zhang, H. Wang, J. Chao, L. Chai and Z. Lin, Angew. Chem., 2022, 134, e202206947 CrossRef.
- D. Li, Y. Shi, L. Yang, L. Xiao, D. K. Kehoe, Y. K. Gun'ko, J. J. Boland and J. J. Wang, Nat. Food, 2020, 1, 746–754 CrossRef CAS PubMed.
- M. K. Momeni, H. Taghipour, M. Ghayebzadeh, M. Mohammadi and R. Keikhaee, Environ. Pollut., 2025, 365, 125389 CrossRef CAS PubMed.
- Z. Lin, T. Jin, T. Zou, L. Xu, B. Xi, D. Xu, J. He, L. Xiong, C. Tang and J. Peng, Environ. Pollut., 2022, 304, 119159 CrossRef CAS PubMed.
Footnote |
† Electronic supplementary information (ESI) available: Optical and LDIR images of ethanol, Mirr-IR low-E glass slides after cleaning; TEM, IR imaging images, IR spectrum matching and optical images of PS standard particles; size distribution and IR imaging images of MPs with different filtration times; different plastic cups in the color-highlighted images of particles; the matching identification of the IR spectra of the particles filtered from water in disposable cups with the spectra in the spectral library; the influence of temperature on the release of MP in PP plastic cups; size distribution and composition of MPs released by PS plastic cups at 50 °C for 15 min; IR spectra of MPs released at different water temperatures; AFM image of untreated PP plastic cup surface after being kept at 4 °C for 15 min; recovery test on PS particles; estimation of daily exposure to MPs; summary and comparison of MPs release from disposable cups. See DOI: https://doi.org/10.1039/d4an01375a |
|
This journal is © The Royal Society of Chemistry 2025 |
Click here to see how this site uses Cookies. View our privacy policy here.