Disparities in potential nitrate exposures within Iowa public water systems†
Received
7th November 2024
, Accepted 19th February 2025
First published on 25th February 2025
Abstract
Nitrates (measured as nitrate-nitrogen) in drinking water exceeding the maximum contaminant level (MCL) of 10 mg L−1 can cause significant health risks, such as methemoglobinemia. Even long-term exposure to concentrations below the MCL can also increase the risks of cancer. Iowa, a major agricultural producer, has grappled with decades-long nitrate pollution in its water systems due to intensive farming practices and animal feeding operations. To help in developing interventions and policies to protect public health, this study delves into long-term nitrate levels in 871 Iowa public water systems (PWSs) between 2012 and 2022 and examines sociodemographic disparities in potential nitrate exposure in drinking water. Average nitrate concentration in Iowa's PWSs increased between 2012 and 2016, reaching an average peak of 3 mg L−1 in 2016. 2.5% of 871 PWSs are classified as ‘high-risk’, with nitrate concentrations consistently exceeding 5 mg L−1 over the study period, primarily in eastern and western Iowa, where animal feeding operations are concentrated. The absence of nitrate removal processes at these PWSs contributes to the sustained elevated levels. On average, 7.4% of the state's population served by PWSs has been exposed to nitrate levels consistently exceeding 5 mg L−1 in the past decade. Disparities exist among various sociodemographic groups, with statistically significant higher exposure rates (10.1%, 9.6%, 9.2%, and 8.7%) observed for people whose incomes are below the federal poverty threshold ($26
496/year), older adults (65 years and above), people of colour, and children (5 years and younger). These disparities are particularly concerning as these populations often lack the resources to address the consequences of water contamination. Our study highlights inequities in Iowa's PWSs concerning potential nitrate exposures and underscores a need for nitrate remediation in specific areas. Addressing these disparities is crucial to safeguarding the health of vulnerable populations and promoting environmental justice in water management.
Water impact
The study investigates the long-term trends in nitrate concentrations in Iowa's public water systems over the period of 2012 to 2022, with a focus on identifying sociodemographic disparities in potential exposure to nitrates in drinking water. The findings highlight an urgent need for targeted public health interventions and policies to address nitrate contamination in vulnerable communities. By providing a localized analysis of water quality, this study advances the understanding of environmental justice issues, building upon and refining previous research in the field.
|
Introduction
Nitrate (NO3−) is a compound commonly found in fertilizers, animal manures, and wastewater sewage.1 Nitrate originating from agricultural activities, industrial discharges, and wastewater treatment processes can contaminate drinking water sources such as groundwater and surface water bodies. In the United States, the Safe Drinking Water Act (SDWA) establishes a maximum contamination level (MCL) for nitrate at 10 mg L−1 NO3-N in drinking water. This regulation is implemented due to the adverse health effects of elevated nitrate exposure,1 including methemoglobinemia in infants, commonly known as “blue baby syndrome”. This condition reduces the blood's oxygen-carrying capacity, leading to cyanosis and potential life-threatening complications.2,3 Extended exposure to nitrate levels below the MCL in drinking water can still have adverse effects on health, potentially increasing the risks of preterm birth,4 birth defects,4–6 and various types of cancer.2,7–17
High levels of nitrate have been found in surface and groundwater drinking water sources across the United States,18,19 notably in regions characterized by intensive farming and animal production. As the primary producer of corn and swine in the U.S., Iowa faces challenges in managing nutrient levels in the environment.20,21 Average nitrate concentrations in Iowa's drinking water increased between 2003 and 2017, with a concentration averaged across 236 towns reaching as high as 5 mg L−1 NO3–N.19 Nearly half of the water samples taken from streams in heavily farmed areas in eastern Iowa had nitrate levels surpassing the MCL.1 However, only 4% of the PWSs across the state have some form of nitrate treatment, including anion exchange, reverse osmosis, and blending (see ESI†). These facilities require substantial capital investment and have high operational cost,22,23 making them inaccessible for many small and low-resourced communities across the state. Although the current MCL for nitrate, set at 10 mg L−1 as nitrogen (N), aligns with the maximum contaminant level goal set by the EPA, emerging research and ongoing assessments have raised questions about whether this regulatory limit adequately protects all population groups, particularly sensitive groups such as postmenopausal women, infants, and pregnant individuals.24 Recent studies have suggested potential health effects at concentrations below the current MCL, underscoring the need for a closer examination of low-level exposures.12,15,17,25,26 The ongoing Integrated Risk Information System (IRIS) assessment by the EPA provides a critical framework for assessing nitrate exposure from drinking water separately from food, particularly among vulnerable populations. This assessment calls out the need to revisit health impacts of nitrate in water using updated scientific methodologies.24
Vulnerable populations can be exposed to the risks from high nitrate concentrations in public water supplies, turning what should be a basic human right—clean drinking water—into a hidden health hazard.27 Communities of color, families with low income, and residents of certain geographic regions disproportionately bear the brunt of inadequate water services28–35 including exposure to contaminants, insufficient infrastructure for clean water access, and financial barriers to securing adequate water services. In the San Joaquin Valley of California, community water systems serving larger percentages of Latinx populations had drinking water with higher nitrate levels, particularly in smaller water systems,36 and people of colour were associated with a 31% increase in the likelihood of elevated nitrate levels.37 These issues often stem from systemic socio-economic inequalities and historical neglect.34,38–41 A nationwide study revealed that 5.6 million Americans are served by a water system that had an average nitrate concentration of at least 5 mg L−1 NO3–N.42 In particular, water systems serving communities in the top quartile for Hispanic population exceeded 5 mg L−1 nearly three times as often as those serving the lowest quartile.42 In the Midwest, particularly in the agricultural regions of Iowa, Minnesota, and Wisconsin, areas where drinking water shows elevated levels of nitrate frequently align with communities whose median household incomes fall below the state's median.43
Although many studies have investigated nitrate exposure disparities across sociodemographic groups, these investigations often lack the spatial granularity required, such as at the utility level, to inform effective decision-making relevant to affected communities. This knowledge gap is characterized by a lack of comprehensive analysis of long-term nitrate levels in finished water from individual drinking water treatment plants, as well as inadequate information on the sociodemographic characteristics of the population served by each treatment plant. In this paper, we addressed this gap by aggregating and analyzing data for active Iowa public water systems (PWSs). Specifically, we analyzed long-term trends of average nitrate levels in finished water from PWSs in Iowa, identified ‘hotspots’ or high-risk facilities, and explored sociodemographic disparities related to potential nitrate exposures (Fig. 1). Our study reveals decade-old nitrate issues in Iowa's drinking water, highlights inequities concerning potential nitrate exposures, and underscores the need for nitrate remediation in specific areas. This research is especially relevant because Iowa's PWSs serve a multitude of small communities that face challenges related to funding, maintenance, and protecting public health and the environment.38,41 Findings from this paper can help local water managers and regulatory agencies prioritize efforts, while emphasizing considerations of equity and justice in decision-making processes.
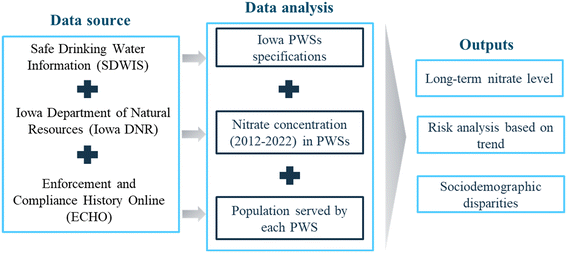 |
| Fig. 1 Flowchart summarizing the approach used in this study. | |
Methods
Data description and preliminary processing
A PWS is defined as a public utility that provides potable water for consumption and domestic usage to residents.44 Such a system must either have a minimum of 15 service connections or cater to an average daily population of at least 25 individuals for at least 60 days per year. We obtained data on Iowa's PWSs from three sources: the U.S. Environmental Protection Agency's (EPA's) Safe Drinking Water Information System (SDWIS), Iowa Department of Natural Resources (IDNR), and EPA's Enforcement and Compliance History Online (ECHO).
SDWIS provides PWS characteristics including system name, activity status, system type (community, non-community, etc.), number of people served, source water type (groundwater or surface water), and region served by the system (county), start and end dates of the tests and results (i.e., air quality, toxicity, lead, and copper). PWSs are grouped using EPA's classification of system size: very small (serving ≤500 people), small (serving 501–3300 people), medium (serving 3301–10
000 people), large (serving 10
001–100
000 people), and very large (serving >100
000 people).44 We compiled PWS characteristics records for a total of 1076 active PWSs in Iowa.
Through collaboration with IDNR, we also acquired the reported annual nitrate concentration (NO3–N mg L−1) data for finished water from each PWS in Iowa, spanning the period from 2012 to 2022. These data were collected by the PWSs as mandated components of compliance monitoring and reported to state regulatory authorities (e.g., IDNR) in adherence to the requirements outlined in the SDWA. We refined the dataset by selecting active community water systems (a subclass of PWS, characterized by its year-round service to residential areas44) that reported nitrate data, resulting in a final set of 871 PWSs and a total of 21
198 nitrate records. Not every PWS has a complete 10-year record of reported nitrate data. Detailed selection criteria are described in the (ESI†). We also obtained information about nitrate treatment technologies used at PWSs from IDNR. This data contains records for 31 PWSs including information such as name and identification code of the system, its classification as a community-based system, the source of the water supply, the population it serves, and the specific treatment methods employed.
Lastly, we sourced sociodemographic information linked to each PWS from the EPA's ECHO database. This dataset includes the percentage of the population (as of 2016–2020 American Community Survey (ACS) 5-Year Summary) within a 5-mile radius of each PWS with specific characteristics, namely individuals with low income (less than or equal to twice the poverty level), people of colour, children aged 5 years and younger, and individuals aged 65 years and older. We associated this information with each PWS in Iowa and assumed that the sociodemographic profile within a 5-mile radius of the PWS represents the overall population served by that PWS This decision was driven by both data availability and practical considerations because the EPA's ECHO database provides sociodemographic information for radius of 1, 3, and 5 miles around each PWS. Our assumption may impose limitations because factors such as urban–rural variations, demographic clusters, and community dynamics can influence the diversity within the entire service area of the PWS. We validated the assumption by, comparing the percentage distribution of each sociodemographic category within a 5-mile radius of all PWSs in each county with the corresponding percentage within the entire county in the year 2016–2020. The comparison suggested slight overestimation for sociodemographic characteristics within the county (Fig. S1†). However, we believe that our approach can still provide a good approximation of the sociodemographic characteristics served by each PWS due to the historical pattern in which water treatment plants were often constructed near marginalized communities.38,45
We cross-checked data from the three sources and implemented data cleaning and quality assurance protocols (see ESI†). As a result, we generated a comprehensive dataset encompassing 871 PWSs in Iowa, or 80.9% of all PWSs. The 871 PWSs are representative of the entire 1076 PWSs in Iowa (Fig. S2†). This dataset includes the following key information: system name, system size, geographic location (e.g., latitude, longitude), county, population served, source water type, annual average nitrate concentrations from 2012 to 2022, existing nitrate treatment technology, and percentage of population with specific sociodemographic classification. Of the 871 PWSs included in our dataset, only 28 PWSs utilize surface water as their primary source. Therefore, we do not anticipate significant seasonality in nitrate levels due to hydrologic variability (i.e., precipitation). In addition to analysing results at the individual PWS level, we also analysed results at the geographic division established by the Iowa Department of Public Health, known as the Regional Medical Coordination Centres (RMCCs) for COVID-19 monitoring (Fig. S3†). Challenges with the dataset refinement and processing are described in ESI† - dataset refinement process.
Nitrate exposure assessment for Iowa PWSs
Taking annual mean nitrate concentration for each of the 871 PWSs between 2012 and 2022, we classified nitrate concentrations C into four categories in accordance with scholarly literature:4,25,26,46–48C ≤ 1 mg L−1, 1 mg L−1 < C ≤ 3 mg L−1, 3 mg L−1 < C ≤ 5 mg L−1, and C > 5 mg L−1. The regulatory limit for nitrate in public drinking water supplies (10 mg L−1) aims to safeguard against infant methemoglobinemia. We adopted 5 mg L−1 as a threshold for elevated concentration because there is strong epidemiological evidence linking drinking water nitrate ingestion to adverse health outcomes at 5 mg L−1.12,15,17,25,26 Additionally, nitrate concentrations as low as 2–3 mg L−1 can exacerbate lead corrosion in plumbing systems containing lead-tin solder, potentially leading to the release of lead-bearing particulates into the water supply.49 Therefore, it is also important to evaluate the implications of nitrate concentrations below 5 mg L−1.
We analysed the trends of annual average nitrate concentration for each PWS using linear regression. A positive slope of the regression line for PWS indicates an upward trend. We also characterized each PWS into high, medium, or low risk based on whether the annual nitrate concentrations consistently exceeded the 5 mg L−1 threshold between 2012 and 2022. High-risk systems were characterized by average nitrate concentration consistently exceeding 5 mg L−1. In contrast, systems with an average concentration consistently below 5 mg L−1 over the decade were classified as low risk. Medium-risk systems were defined as those without exhibiting a consistent trend above or below the 5 mg L−1 threshold.50 In analysing the potential disparity among socio-demographic groups, we adopted the use of quantile method in ArcGIS. Using the quantile method for colour shading on these maps provides a more effective way to communicate geographic patterns and trends in the data by enhancing pattern detection.51,52 The quantile method focuses on relative ranking rather than absolute values. This is particularly valuable when comparing variables with wide-ranging scales, such as income levels or age demographics alongside nitrate levels. Focusing on relative standing rather than exact numbers (i.e., quantiles place data points into categories that represent their position relative to the rest of the data instead of using fixed value ranges) allows clearer comparisons across demographic groups without letting extreme values distort the visual interpretation.53 Finally, the quantile method enhances differentiation within closely grouped data because average nitrate concentration data can often be skewed, with most counties clustering at lower levels and only a few at higher levels.54,55 By grouping counties with similar rankings, the quantile approach accentuates colour variation, making it easier to spot geographic “hotspots” without overemphasizing small differences. These advantages make quantile-based shading an ideal choice for effectively conveying both demographic and environmental patterns on these maps.
Results and discussion
Overview of Iowa's PWSs
Iowa's primary water source is groundwater, with 74% of PWSs providing treated groundwater to homes. In contrast, 140 (16%) PWSs supply water from surface sources, while the remaining 10% were not identified. Iowa's PWSs are predominantly categorized as very small (53.9%), small (33.5%), and medium (8.1%) (Fig. 2a). Most counties feature a range of system sizes, with very small and small systems prevailing (Fig. 2b). Only 4% of Iowa's PWSs have nitrate treatment facilities; small systems face unique challenges related to funding, maintenance, public health, environmental protection, and compliance with water quality standards.40
 |
| Fig. 2 Classification of Iowa public water systems by size: (a) classification for all 1076 PWSs considered. (b) Classification at the county level. Size of pie indicates total number of PWSs within the county.% of small systems encompasses “very small”, “small”, and “medium” as defined by EPA.44 | |
Average nitrate concentrations in Iowa's PWSs
Approximately 73% of the PWSs have a 10-year average nitrate concentration less than 1 mg L−1 (Fig. 3a), the minimal risk level outlined in environmental health guidelines.56 This observation translates to approximately 1.3 million Iowans receiving treated drinking water with low nitrate concentration (Fig. 3b), despite the absence of nitrate removal processes in their systems. This may be attributed to sourcing water from deeper aquifers that are less affected by nitrate contamination.57–59
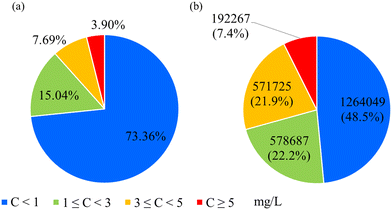 |
| Fig. 3 Average nitrate concentrations and population exposed: (a) average nitrate concentration for 871 PWSs. (b) Fraction of population exposed to different nitrate concentrations across Iowa. | |
The second most common range for nitrate concentrations is between 1 and 3 mg L−1, covering about 15% of Iowa's PWSs and serving approximately 578
687 people. These concentrations, though not immediately hazardous, warrant monitoring to prevent any potential escalation that could affect public health. Around 11.6% of the public water systems, serving 763
992 people, have average concentrations above 3 mg L−1. Consistent exposure to these nitrate levels, particularly for vulnerable populations, may pose significant health risks. A study in Iowa found that long-term exposure (over five years) to average nitrate concentrations above 2.98 mg L−1 in public water systems was associated with increased incidences of ovarian, bladder, and breast cancer in postmenopausal women.12,13,15 This subset of the data underscores the need for targeted water quality interventions and continuous monitoring to ensure public safety.
We performed statistical analysis for the nitrate levels for all facilities and determined that applying an average value is an appropriate approach for comparison (see ESI† – statistical analysis of nitrate concentration data). Average nitrate concentrations in Iowa's PWSs increased between 2012 and 2016, followed by a decline until 2020, and a resurgence through 2022 (Fig. 4). The decline and resurgence trends are significant at p < 0.05. A drought in 2012 that led to the accumulation of substantial nitrogen (N) in soils was followed by record spring rainfall in 2013. The transition from a dry to a wet year created conditions facilitating nitrogen transport from agricultural areas to water bodies, which resulted in a more than 400% increase in the amount of nitrate detected in Iowa streams from 2012 to 2013.21 This surge made 2013 one of the worst years in recent decades for nitrate pollution, with the absolute amount of nitrate in streams statewide trending upward until 2016,60–62 This also led to elevated nitrate levels in treated drinking water as observed in Fig. 4, despite the existence of nitrate removal measures at the treatment plants.62
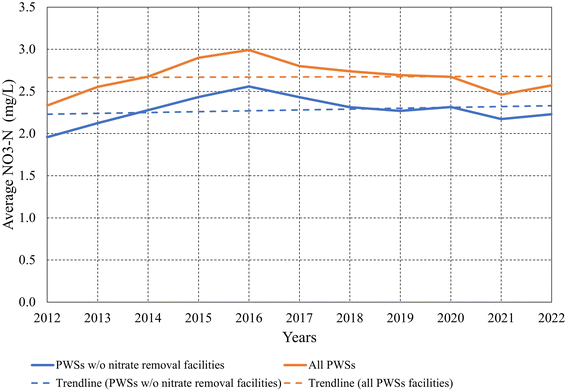 |
| Fig. 4 Average nitrate concentration across Iowa PWSs between 2012 and 2022. Thick blue line is averaged for PWSs without nitrate removal facilities. Thick orange line is averaged for all PWSs. Dashed lines are trendlines for both cases. | |
Average nitrate concentrations in PWSs without nitrate treatment are lower than in all PWSs (Fig. 4). While this may appear counterintuitive, it is not surprising. PWSs with nitrate removal facilities have higher nitrate concentrations in their raw water sources. Even after treatment, nitrate levels are only reduced to just below the MCL to comply with regulations. This treated water often still has higher nitrate concentrations than the raw water in many PWSs without nitrate removal facilities. The effectiveness of the existing treatment systems varies, with some possibly being outdated or not sufficiently robust to handle the increasing nitrate levels.63–65 Furthermore, the time it takes for facilities to adapt or upgrade their treatment processes can result in ongoing exposure to higher nitrates.66 These nuances are important in understanding the data and the complex interplay of environmental factors, infrastructure capabilities, and the efficacy of current water treatment methods.
Hotspots
PWSs located in regions 3, 4, 5, and 6, corresponding to the Eastern and Western parts of Iowa, tend to have higher nitrate concentrations (Fig. 5). Eastern Iowa has 16 hotspots, or PWSs with an average nitrate concentration exceeding 5 mg L−1, whereas Western Iowa has 18. Despite having fewer hotspots, the average nitrate concentration in Eastern Iowa (6.74 mg L−1 with a standard deviation of 2.29) is higher than in Western Iowa (6.36 mg L−1 with a standard deviation of 1.65), of which both are statistically significant at p < 0.05. Previous studies have shown that nitrate contamination in groundwater is particularly severe in these regions: Eastern Iowa (Floyd, Bremer, Tama, Jasper counties) with contamination in 11% of the wells, Western Iowa (Sioux, Cherokee, Ida, Cass counties) with contamination in 38.2% of the wells, and South-Central Iowa (Polk, Clarke, Wayne counties) with contamination in 28.1% of the wells.67,68 High nitrate concentrations in certain regions may be linked to the limited adoption of advanced treatment technologies69 as well as local agricultural activities and geological conditions affecting water quality.60,69 In our preliminary observation, the spatial pattern of these hotspots somewhat aligns with the distribution of confined animal operations in Iowa.70 However, it is outside the scope of this study to analyze the relationship between the spatial patterns of nitrate hotspots and the distribution of confined animal operations.
 |
| Fig. 5 Nitrate concentration averaged for 2012–2022 for each PWS in Iowa. Outline color indicates the type of nitrate removal process. Size of circle indicates system size. Underlying background color indicates Iowa Department of Public Health Regional Medical Coordination Centres. | |
Temporal trends in Iowa PWSs
There are 19 high-risk PWSs—or facilities with average nitrate concentrations consistently above 5 mg L−1—and 750 low-risk PWSs (Fig. 6 and S4†). Average nitrate concentrations increased over the study period for 512 PWSs, with high-risk facilities showing levels ranging from 4 to 9 mg L−1. Conversely, 311 PWSs exhibited a decreasing trend in nitrate levels, while 11 PWSs maintained consistently low nitrate concentrations, averaging around 0.05 mg L−1 over the study period. In Eastern Iowa (regions 5 and 6), ten PWSs were classified as high-risk, while in Western Iowa (regions 3 and 4), nine PWSs were high-risk. Seven of the nine high-risk PWSs in Western Iowa showed an upward trend in nitrate levels, whereas in Eastern Iowa, five out of ten high-risk PWSs displayed a similar upward trend. Overall, there are 12 PWSs classified as high-risk with an upward trend, and 7 high-risk PWSs that show a downward trend. There are 470 PWSs classified as low-risk but with an upward trend. The high-risk PWSs with an upward trend are particularly concerning due to their consistently high nitrate concentrations and lack of signs of improvement. Amongst these systems, ten are identified as small systems and only two are large systems. Only one of the 12 PWSs had implemented a nitrate treatment method. This observation highlights the vulnerability of small systems and the reliance of these systems on source water quality rather than active mitigation strategies.
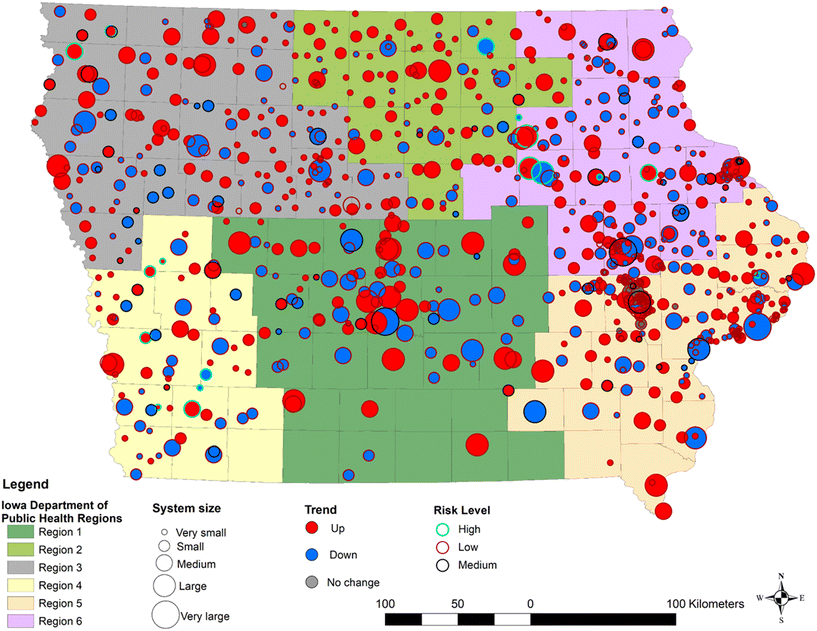 |
| Fig. 6 Trend of average nitrate concentration between 2012 and 2022 for each PWS in Iowa. Outline color indicates risk level. Size of circle indicates system size. Underlying background color indicates Iowa Department of Public Health regions. | |
Disparities in potential nitrate exposure
There are noticeable disparities among different sociodemographic groups regarding potential exposure to average nitrate concentrations exceeding 5 mg L−1 in their drinking water across all active PWSs with no inclusion of private wells. On average, 7.4% of Iowa's population has been exposed to nitrate concentrations exceeding 5 mg L−1 in the past decade. Certain groups face higher exposure rates: 9.2% of people of colour, 10.1% of low-income individuals, 9.6% of adults aged 65 and older, and 8.7% of children aged 5 and under. The unequal variances t-test suggests that results are significant at p < 0.05. We further analysed disparities by dividing the data into two distinct periods: 2012–2016 and 2017–2022, given that annual average nitrate concentrations peaked in 2016 (Fig. 4). Despite a decline in average nitrate concentrations after 2016, significant disparities among sociodemographic groups remain statistically significant (Table S1†).
We also analysed disparities at the county level by aggregating the results of individual PWS to the counties where they are located (Fig. 7). County names and population density are detailed in Fig. S5.† The x-axis represents nitrate concentration averaged at the county level for all PWSs with concentrations ranging from 0.1 mg L−1 to 5.1 mg L−1 which is consistent throughout Fig. 7; the y-axis are different for each category: people of colour - 0 to 47%, low-income individuals - 2% to 49%, adults aged 65 and older - 2% to 27%, and children aged 5 and younger - 1% to 8%. Counties such as Black Hawk, Wapello, and Crawford, where percentages of people of colour are relatively high, all have average nitrate concentrations exceeding 5 mg L−1 (Fig. 7a). For low-income individuals, we observed that counties such as Black Hawk, Crawford, Audubon, Cass, and Jasper exhibit both a high percentage of low-income individuals and high average nitrate concentrations in drinking water (Fig. 7b). For adults aged 65 and older, we identified disparities in counties such as Butler, Monona, Audubon, and Cass (Fig. 7c). For children aged 5 and younger, counties such as Black Hawk, Crawford, Butler, Cass, and Boone exhibit concerning high nitrate concentrations, putting young children at risk (Fig. 7d). Black Hawk and Crawford counties appear in all categories except for adults aged 65 and older, highlighting discernible disparities in nitrate exposure among different sociodemographic groups in these regions. Black Hawk County has nine PWSs, seven of which are categorized as small systems. In Crawford County, all public water systems are small. Despite the high nitrate concentrations in their drinking water, none of the PWSs in these two counties have implemented nitrate removal processes. The average nitrate concentrations in the finished water supplied to homes were as high as 7.2 mg L−1 in Black Hawk County and 8.1 mg L−1 in Crawford County. Nitrogen fertilizer application in Black Hawk County contributes to the high nitrate in its groundwater wells, which are the main source of drinking water for the county.71 As the fifth most populous county in Iowa, Black Hawk faces the challenge of balancing economic growth with the imperative of ensuring safe and clean drinking water for its residents, particularly for the most vulnerable sociodemographic groups. According to the U.S. Centers for Disease Control and Prevention, Crawford County has the highest social vulnerability index score in the region.72 It also ranks among the twelve Iowa counties most at risk for drought.73 These factors exacerbate concerns for Crawford County communities, which are already struggling with limited resources to address nitrate contamination in their drinking water while grappling with ongoing water scarcity issues.57–60
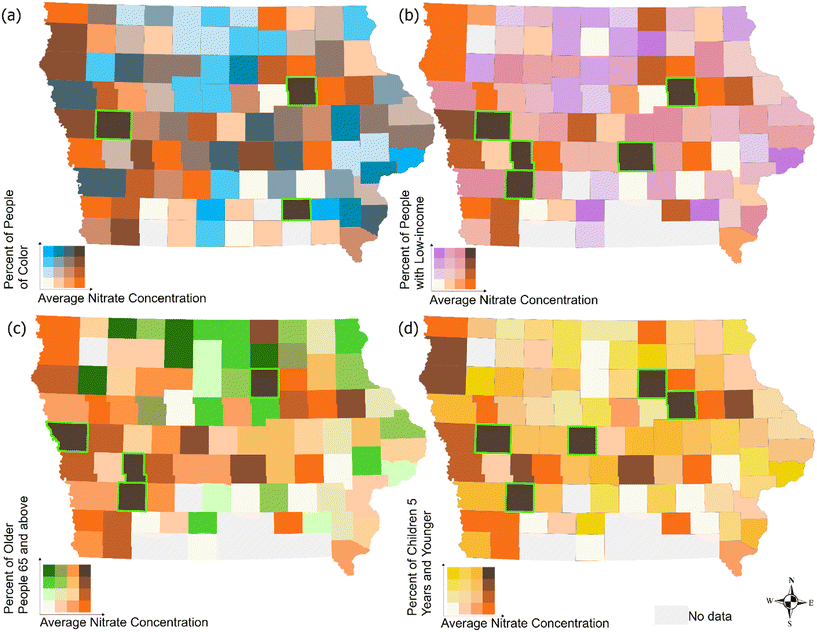 |
| Fig. 7 Disparity in potential nitrate exposure among different sociodemographic groups (a) people of colour (b) low-income communities (c) older people 65 and above and (d) children 5 years and younger. The hatchet areas depict areas that do not have or report any information in EPA ECHO. Counties named in the text are highlighted with green outlines. | |
Adequately treating nitrate in drinking water requires substantial investment. The current EPA MCL of 10 mg L−1 was set to balance financial feasibility with public health protection.74 Recent epidemiological studies have shown that ingesting nitrate at lower than MCL levels for an extended period still results in severe adverse health outcomes,12,13,15 leading to significant economic costs. One study estimates a potential economic impact of $1.3 to $6.5 billion in lost productivity across the United States, using a high nitrate threshold of 4.5 mg L−1.5 Another study demonstrated that reducing nitrate concentration from 10 mg L−1 to 3.87 mg L−1 could yield about $302 million dollars economic health benefits in Denmark.75 These findings highlight that the economic benefits of lowering nitrate levels can outweigh the associated costs, providing a strong case for revising current nitrate standards. While our study does not challenge the importance of the current MCL, we emphasize the need to investigate the adverse health risks associated with nitrate levels below the MCL, particularly their disproportionate impact on marginalized communities with limited resources.
All in all, our results underscore the necessity for public health policies and environmental regulations that specifically address the needs of the most vulnerable populations.
Conclusion
Iowa is dominated by small and very small PWSs, according to EPA categorization. Addressing nitrate contamination in the state's drinking water has long been a challenge, particularly for small and under-resourced communities, which make up the majority in Iowa. Our study tracks the long-term trends of nitrate concentrations across 871 active PWSs in Iowa and identifies critical ‘hotspots’ requiring immediate intervention. Notably, Eastern and Western Iowa (Regions 3, 4, 5, and 6) are areas of high concern, with average nitrate levels consistently exceeding 5 mg L−1 throughout the study period for many PWSs in these two regions. The absence of advanced nitrate removal technologies, such as anion exchange and reverse osmosis, exacerbates the problem, contributing to persistently high nitrate levels in these communities' drinking water.
Furthermore, our analysis reveals that low-income individuals, older adults, children, and people of colour are disproportionately affected by high nitrate levels, with these groups experiencing higher rates of exposure compared to the state average of 7.4%. We have identified ‘hotspot’ counties where PWSs exhibit elevated nitrate concentrations, frequently in socially vulnerable communities. These findings underscore the need for targeted interventions to lower nitrate levels in water supplies, especially in high-risk areas, to safeguard the health of Iowa's most vulnerable populations. The implications of these findings extend beyond immediate health concerns, emphasizing a need for infrastructure investment in water treatment facilities, especially in the high-risk areas we've identified. This issue intersects with public health and social equity, as the situation at hand disproportionately affects disadvantaged communities. On a policy level, there may be a need to revise regulatory standards and enforcement to ensure safer water for Iowans and the nation as a whole since epidemiological studies show that even lower levels could potentially harm human health. Moreover, our findings advocate for initiating more robust public health initiatives, which could include enhanced water quality monitoring to ensure the risks are managed proactively. Public awareness campaigns and community support programs are also essential in mitigating the risks associated with nitrate exposure until more permanent solutions take hold. By addressing these issues comprehensively, there is an opportunity to improve health outcomes and advance environmental justice, ensuring that all residents have access to safe drinking water.
Data availability
The datasets generated and analyzed during this study are available from the corresponding author upon request.
Author contributions
E. P. M. was responsible for data curation, formal analysis, and investigation; he developed the methodology, contributed software and resources, created visualizations, and wrote the original draft. L. L. conceptualized the project, secured the necessary funding, and oversaw project administration, providing essential resources and supervision; she also contributed to validating the work and reviewing and editing the paper. C. R. contributed to acquiring funding, managing the project, providing necessary resources, assisting in the validation process, and reviewing and editing the paper.
Conflicts of interest
There are no conflicts to declare.
Acknowledgements
The authors would like to acknowledge the Environmental Protection Agency for funding support (Grant number R840461: accelerating technical and community readiness for water reuse in small systems), and the Iowa Department of Natural Resources for providing data. Additionally, we would like to acknowledge ISU undergraduate students—Matthew Decker, Sarah Kothlow, Suryansh Mishra, and Jacob Dunn—for their contributions to our study.
References
-
Water Quality in the Eastern Iowa Basins Iowa and Minnesota, 1996–98, [cited 2024 Jul 14], available from: http://water.usgs.gov/nawqa/ Search PubMed.
- A. J. De Roos, M. H. Ward, C. F. Lynch and K. P. Cantor, Nitrate in public water supplies and the risk of colon and rectum cancers, Epidemiology, 2003, 14(6), 640–649 CrossRef PubMed.
- T. Chambers, J. Douwes, A. Mannetje, A. Woodward, M. Baker and N. Wilson,
et al., Nitrate in drinking water and cancer risk: the biological mechanism, epidemiological evidence and future research, Aust. N. Zeal. J. Public Health., 2022, 46, 105–108 CrossRef PubMed.
- L. Lin, S. St Clair, G. D. Gamble, C. A. Crowther, L. Dixon and F. H. Bloomfield,
et al., Nitrate contamination in drinking water and adverse reproductive and birth outcomes: a systematic review and meta-analysis, Sci. Rep., 2023, 13(1), 563 CrossRef CAS PubMed.
- A. Temkin, S. Evans, T. Manidis, C. Campbell and O. V. Naidenko, Exposure-based assessment and economic valuation of adverse birth outcomes and cancer risk due to nitrate in United States drinking water, Environ. Res., 2019, 176, 108442 CrossRef CAS PubMed.
- J. D. Brender, P. J. Weyer, P. A. Romitti, B. P. Mohanty, M. U. Shinde and A. M. Vuong,
et al., Prenatal nitrate intake from drinking water and selected birth defects in offspring of participants in the national birth defects prevention study, Environ. Health Perspect., 2013, 121(9), 1083–1089 CrossRef PubMed.
- N. Espejo-Herrera, E. Gràcia-Lavedan, E. Boldo, N. Aragonés, B. Pérez-Gómez and M. Pollán,
et al., Colorectal cancer risk and nitrate exposure through drinking water and diet, Int. J. Cancer, 2016, 139(2), 334–346 CrossRef CAS PubMed.
- A. J. De Roos, M. H. Ward, C. F. Lynch and K. P. Cantor, Nitrate in public water supplies and the risk of colon and rectum cancers, Epidemiology, 2003, 14(6), 640–649 CrossRef PubMed.
- B. A. Kilfoy, Y. Zhang, Y. Park, T. R. Holford, A. Schatzkin and A. Hollenbeck,
et al., Dietary nitrate and nitrite and the risk of thyroid cancer in the NIH-AARP diet and health study, Int. J. Cancer, 2011, 129(1), 160–172 CrossRef CAS.
- M. H. Ward, B. A. Kilfoy, P. J. Weyer, K. E. Anderson, A. R. Folsom and J. R. Cerhan, Nitrate intake and the risk of thyroid cancer and thyroid disease, Epidemiology, 2010, 21(3), 389–395 CrossRef PubMed.
- H. F. Chiu, S. S. Tsai and C. Y. Yang, Nitrate in drinking water and risk of death from bladder cancer: An ecological case-control study in Taiwan, J. Toxicol. Environ. Health, Part A, 2007, 70(12), 1000–1004 CrossRef CAS PubMed.
- M. Inoue-Choi, R. R. Jones, K. E. Anderson, K. P. Cantor, J. R. Cerhan and S. Krasner,
et al., Nitrate and nitrite ingestion and risk of ovarian cancer among postmenopausal women in Iowa, Int. J. Cancer, 2015, 137(1), 173–182 CrossRef CAS PubMed.
- R. R. Jones, P. J. Weyer, C. T. Dellavalle, M. Inoue-Choi, K. E. Anderson and K. P. Cantor,
et al., Nitrate from drinking water and diet and bladder cancer among postmenopausal women in Iowa, Environ. Health Perspect., 2016, 124(11), 1751–1758 CrossRef CAS PubMed.
- A. J. L. Quist, M. Inoue-Choi, P. J. Weyer, K. E. Anderson, K. P. Cantor and S. Krasner,
et al., Ingested nitrate and nitrite, disinfection by-products, and pancreatic cancer risk in postmenopausal women, Int. J. Cancer, 2018, 142(2), 251–261 CrossRef CAS PubMed.
- M. Inoue-Choi, M. H. Ward, J. R. Cerhan, P. J. Weyer, K. E. Anderson and K. Robien, Interaction of nitrate and folate on the risk of breast cancer among postmenopausal women, Nutr. Cancer, 2012, 64(5), 685–694 CrossRef CAS.
- G. Gulis, M. Czompolyova and J. R. Cerhan, An ecologic study of nitrate in municipal drinking water and
cancer incidence in Trnava District, Slovakia, Environ. Res., 2002, 88(3), 182–187 CrossRef CAS PubMed.
- R. R. Jones, P. J. Weyer, C. T. Dellavalle, K. Robien, K. P. Cantor and S. Krasner,
et al., Ingested Nitrate, Disinfection By-products, and Kidney Cancer Risk in Older Women, Epidemiology, 2017, 28(5), 703–711 CrossRef PubMed.
- B. T. Nolan, B. C. Ruddy, K. J. Hitt and D. R. Helsel, Risk of nitrate in groundwaters of the United States - A national perspective, Environ. Sci. Technol., 1997, 31(8), 2229–2236 CrossRef CAS , available from: https://pubs.usgs.gov/publication/70019648.
-
A. W. Schechinger, EWG Investigation: Across Farm Country, Nitrate Pollution of Drinking Water for More Than 20 Million Americans Is Getting Worse [Internet], 2020 [cited 2024 Jul 11], available from: https://www.ewg.org/interactive-maps/2020-nitrate-pollution-of-drinking-water-for-more-than-20-million-americans-is-getting-worse/ia/ Search PubMed.
- D. L. Karlen, C. A. Cambardella and R. S. Kanwar, Challenges of Managing Liquid Swine Manure, Appl. Eng. Agric., 2004, 20(5), 693–699 Search PubMed.
-
Iowa Department of Agriculture and Land Stewardship (IDALS), Iowa Department of Natural Resources (IDNR) and Iowa State University College of Agriculture and Life Sciences (ISU CALS), Iowa Nutrient Reduction Strategy, A science and technology-based framework to assess and reduce nutrients to Iowa waters and the Gulf of Mexico, 2016 Search PubMed.
- J. J. Schoeman and A. Steyn, Nitrate removal with reverse osmosis in a rural area in South Africa, Desalination, 2003, 15, 15–26 CrossRef , available from: https://researchspace.csir.co.za/dspace/handle/10204/1649.
- M. Shrimali and K. P. Singh, New methods of nitrate removal from water, Environ. Pollut., 2001, 112(3), 351–359 CrossRef CAS PubMed , available from: https://pubmed.ncbi.nlm.nih.gov/11291441/.
-
K. Christensen, A. Lee, R. Mezencev, A. Persad, M. Powers and R. Shaffer, et al., U.S. EPA. Protocol for the Nitrate and Nitrite IRIS Assessment (Oral) (Preliminary Assessment Materials) | IRIS | US EPA [Internet], 2023, available from: https://iris.epa.gov/Document/&deid=338654.
- P. D. Mathewson, S. Evans, T. Byrnes, A. Joos and O. V. Naidenko, Health and economic impact of nitrate pollution in drinking water: a Wisconsin case study, Environ. Monit. Assess., 2020, 192(11), 724 CrossRef CAS PubMed.
- P. J. Weyer, J. R. Cerhan, B. C. Kross, G. R. Hallberg, J. Kantamneni and G. Breuer,
et al., Municipal Drinking Water Nitrate Level and Cancer Risk in Older Women: The Iowa Women's Health Study, Epidemiology, 2001, 12, 327–338 CrossRef CAS PubMed.
- M. M. Maroneze, L. Q. Zepka, J. G. Vieira, M. I. Queiroz and E. Jacob-Lopes, A tecnologia de remoção de fósforo: Gerenciamento do elemento em resíduos industriais, Rev. Ambient. Água, 2014, 9(3), 445–458 Search PubMed.
-
C. Montag, Water/Color [Internet], The Thurgood Marshall Institute at LDF, 2019, available from: https://www.naacpldf.org/wp-content/uploads/Water_Report_Executive-Summary_5_21_19_FINAL-V2.pdf Search PubMed.
-
C. R. Campbell Larrison, Frustrated Jackson Locals Are Nearing Two Weeks With No Water, The Daily Beast [Internet], 2021, available from: https://www.thedailybeast.com/residents-of-jackson-are-nearing-two-weeks-with-no-running-water Search PubMed.
-
K. P. Fedinick, S. Taylor and M. Roberts, Watered Down Justice, Natural Resources Defense Council, 2019, available from: https://www.nrdc.org/sites/default/files/watered-down-justice-report.pdf Search PubMed.
- Flint and Newark, New Drinking Water Report: Communities of Color More Likely to Suffer Drinking Water Violations For Years [Internet], 2019, available from: https://www.nrdc.org/press-releases/new-drinking-water-report-communities-color-more-likely-suffer-drinking-water.
-
N. Mirumachi, A. Duda, J. Gregulska and J. Smętek, The Human Right to Drinking Water: Impact of large-scale agriculture and industry, 2021 Search PubMed.
- A. I. Patel, L. A. Schmidt and P. R. Lee, AJPH Editorials: Water access in the United States: Health disparities abound & solutions are urgently needed, Am. J. Public Health, 2017, 107, 1354–1356 CrossRef PubMed.
-
S. Dtac, SAMHSA Disaster Technical Assistance Center Supplemental Research Bulletin Greater Impact: How Disasters Affect People of Low Socioeconomic Status, 2017 Search PubMed.
-
P. Orum, R. Moore, M. Roberts and J. Sanchez, A Demographic Analysis Of Chemical Disaster Vulnerability Zones, 2014, May [cited 2024 Jul 15]; Available from: https://comingcleaninc.org/assets/media/images/Reports/Who%27s%20in%20Danger%20Report%20FINAL.pdf Search PubMed.
- C. Balazs, R. Morello-Frosch, A. Hubbard and I. Ray, Social Disparities in Nitrate-Contaminated Drinking Water in California's San Joaquin Valley, Environ. Health Perspect., 2011, 119(9), 1272–1278 CrossRef CAS PubMed.
- C. Pace, C. Balazs, K. Bangia, N. Depsky, A. Renteria and R. Morello-Frosch,
et al., Inequities in Drinking Water Quality Among Domestic Well Communities and Community Water Systems, California, 2011–2019, Am. J. Public Health, 2022, 112(1), 88–97 CrossRef PubMed , available from: https://ajph.aphapublications.org/doi/10.2105/AJPH.2021.306561.
-
I. Khalsa, The impact of water infrastructure inequality on marginalized communities, Honors Theses, University of Tennessee at Chattanooga, 2022, available from: https://scholar.utc.edu/honors-theses/379 Search PubMed.
- J. Vanderslice, Drinking water infrastructure and environmental disparities: Evidence and methodological considerations, Am. J. Public Health, 2011, 101(SUPPL. 1), S109–S114 CrossRef PubMed.
- K. McFarlane and L. M. Harris, Small systems, big challenges: Review of small drinking water system governance, Environ. Rev., 2018, 26, 378–395 CrossRef.
-
EPA U, DWSRF Disadvantaged Community Definitions: A Reference for States, 2022 Oct [cited 2024 Jul 15], available from: https://www.google.com/url?sa=t&source=web&rct=j&opi=89978449&url=https://www.epa.gov/system/files/documents/2022-10/DWSRF%2520DAC%2520Definitions%2520Report_October%25202022%2520Updates_FINAL_508.pdf&ved=2ahUKEwiPlY2O9u6KAxW3NzQIHfw2CwsQFnoECBwQAQ&usg=AOvVaw1o8-ij86PWRC47YcIT0lY2.
- L. A. Schaider, L. Swetschinski, C. Campbell and R. A. Rudel, Environmental justice and drinking water quality: Are there socioeconomic disparities in nitrate levels in U.S. drinking water?, Environ. Health, 2019, 18(1), 3 Search PubMed.
-
Ewg, EWG Investigation: Across Farm Country, Nitrate Pollution of Drinking Water for More Than 20 Million Americans Is Getting Worse Search PubMed.
-
G. Chuck, State of Iowa Public Drinking Water Program 2016 Annual Compliance Report, 2017 Search PubMed.
- J. M. Liddie, L. A. Schaider and E. M. Sunderland, Sociodemographic Factors Are Associated with the Abundance of PFAS Sources and Detection in U.S. Community Water Systems, Environ. Sci. Technol., 2023, 57(21), 7902–7912, DOI:10.1021/acs.est.2c07255.
- A. J. De Roos, M. H. Ward, C. F. Lynch and K. P. Cantor, Nitrate in public water supplies and the risk of colon and rectum cancers, Epidemiology, 2003, 14(6), 640–649 Search PubMed.
- M. E. Hildesheim, K. P. Cantor, C. F. Lynch, M. Dosemeci, J. Lubin and M. Alavanja,
et al., Drinking Water Source and Chlorination Byproducts II. Risk of Colon and Rectal Cancers, Epidemiology, 1998, 9, 29–35 CrossRef CAS PubMed.
- R. R. Jones, P. J. Weyer, C. T. Dellavalle, M. Inoue-Choi, K. E. Anderson and K. P. Cantor,
et al., Nitrate from drinking water and diet and bladder cancer among postmenopausal women in Iowa, Environ. Health Perspect., 2016, 124(11), 1751–1758 Search PubMed.
- K. G. Lopez, J. Xiao, C. Crockett, C. Lytle, H. Grubbs and M. Edwards, Seasonal Fluctuations in Nitrate Levels Can Trigger Lead Solder Corrosion Problems in Drinking Water, Environ. Sci. Technol. Lett., 2023, 10(1), 21–26, DOI:10.1021/acs.estlett.2c00581.
- J. D. Nilles, D. Lim, M. P. Boyer, B. D. Wilson, R. A. Betar and H. A. Showalter,
et al., The occurrence of bone and joint cancers and their association with rural living and radon exposure in Iowa, Environ. Geochem. Health, 2023, 45(3), 925–940 CrossRef CAS PubMed.
- C. A. Brewer and L. Pickle, Evaluation of Methods for Classifying Epidemiological Data on Choropleth Maps in Series, Ann. Assoc. Am. Geogr., 2002, 92, 662–681 CrossRef , available from: https://api.semanticscholar.org/CorpusID:8423819.
- J. M. Olson, Class Interval Systems on Maps of Observed Correlated Distributions, Cartographica, 1972, 9, 122–131 CrossRef , available from: https://api.semanticscholar.org/CorpusID:119740250.
- R. G. Cromley, D. M. Hanink and J. Lin, Developing Choropleth Maps of Parameter Results for Quantile Regression, Cartographica, 2013, 48, 177–188 CrossRef , available from: https://api.semanticscholar.org/CorpusID:41247744.
- K. McGreevy, S. R. Lipsitz, J. A. Linder, E. B. Rimm and D. G. Hoel, Using median regression to obtain adjusted estimates of central tendency for skewed laboratory and epidemiologic data, Clin. Chem., 2009, 55(1), 165–169 CrossRef CAS PubMed , available from: https://api.semanticscholar.org/CorpusID:12864934.
-
Z. Lin, J. Liu and N. Lin, Accurate quantile estimation for skewed data streams, 2017 IEEE 28th Annual International Symposium on Personal, Indoor, and Mobile Radio Communications (PIMRC) [Internet], 2017, pp. 1–7, available from: https://api.semanticscholar.org/CorpusID:3414563 Search PubMed.
- B. T. Nolan and J. D. Stoner, Nutrients in groundwaters of the conterminous United States, 1992–1995, Environ. Sci. Technol., 2000, 34(7), 1156–1165 CrossRef CAS.
-
N. Troudi, F. Hamzaoui-Azaza, O. Tzoraki and M. Zammouri, Spatial Distribution of Nitrate Level in a Deep Aquifer Located in an Agricultural Region (North Tunisia), in New Prospects in Environmental Geosciences and Hydrogeosciences, ed. H. Chenchouni, H. I. Chaminé, M. F. Khan, B. J. Merkel, Z. Zhang and P. Li, et al., Springer International Publishing, Cham, 2022, pp. 551–555 Search PubMed.
- M. R. Burkart and J. D. Stoner, Nitrate in aquifers beneath agricultural systems, Water Sci. Technol., 2007, 56(1), 59–69, DOI:10.2166/wst.2007.436.
-
N. M. Dubrovsky and P. A. Hamilton, Nutrients in the Nation's Streams and Groundwater: National Findings and Implications The Quality of Our Nation's Waters Occurrence and Distribution of Nutrients in Streams and Groundwater, 2010 Search PubMed.
-
Iowa Capital Dispatch, Iowa primed for potentially ‘epic’ increase in stream pollution, Iowa Capital Dispatch [Internet], 2024, available from: https://iowacapitaldispatch.com/2024/02/29/iowa-primed-for-potentially-epic-increase-in-stream-pollution/.
- C. S. Jones, C. A. Davis, C. W. Drake, K. E. Schilling, S. H. P. Debionne and D. W. Gilles,
et al., Iowa Statewide Stream Nitrate Load Calculated Using In Situ Sensor Network, J. Am. Water Resour. Assoc., 2018, 54(2), 471–486 CrossRef CAS , available from: https://onlinelibrary.wiley.com/doi/full/10.1111/1752-1688.12618.
- P. C. Van Metre, J. W. Frey, M. Musgrove, N. Nakagaki, S. Qi and B. J. Mahler,
et al., High Nitrate Concentrations in Some Midwest United States Streams in 2013 after the 2012 Drought, J. Environ. Qual., 2016, 45(5), 1696–1704 Search PubMed , available from: https://pubmed.ncbi.nlm.nih.gov/27695770/.
- V. B. Jensen, J. L. Darby, C. J. Seidel and C. Gorman, Nitrate in Potable Water Supplies: Alternative Management Strategies, Crit. Rev. Environ. Sci. Technol., 2014, 44, 2203–2286 CrossRef CAS , available from: https://api.semanticscholar.org/CorpusID:98288669.
- S. E. Vero, N. B. Basu, K. Van Meter, K. G. Richards, P.-E. Mellander and M. G. Healy,
et al. Review: the environmental status and implications of the nitrate time lag in Europe and North America, Hydrogeol. J., 2018, 26, 7–22 CrossRef CAS.
- E. Abascal, L. Gómez-Coma, I. Ortiz and A. Ortiz, Global diagnosis of nitrate pollution in groundwater and review of removal technologies, Sci. Total Environ., 2022, 810, 152233 CrossRef CAS PubMed , available from: https://pubmed.ncbi.nlm.nih.gov/34896495/.
-
I. Bogardi, R. D. Kuzelka and W. G. Ennenga, Nitrate Contamination: Exposure, Consequence, and Control, 1992, available from: https://api.semanticscholar.org/CorpusID:127478097 Search PubMed.
- B. T. Nolan, R. W. Healy, P. E. Taber, K. Perkins, K. J. Hitt and D. M. Wolock, Factors influencing ground-water recharge in the eastern United States, J. Hydrol., 2007, 332(1–2), 187–205 Search PubMed.
- B. C. Kross, G. R. Hallberg, D. R. Bruner, K. Cherryholmes and J. K. Johnson, The nitrate contamination of private well water in Iowa, Am. J. Public Health, 1993, 83(2), 270 Search PubMed , available from: https://ajph.aphapublications.org/doi/epdf/10.2105/AJPH.83.2.270.
- M. H. Ward, T. M. deKok, P. Levallois, J. Brender, G. Gulis and B. T. Nolan,
et al., Workgroup report: Drinking-water nitrate and health - Recent findings and research needs, Environ. Health Perspect., 2005, 113(11), 1607–1614 CrossRef CAS PubMed.
-
C. Kohrt, Animal Feeding Operations Download | Iowa Geospatial Data Clearinghouse [Internet], 2022 [cited 2024 Jul 15], available from: https://geodata.iowa.gov/documents/abfbd972640d4e87b6c48dc669775767/about Search PubMed.
-
R. L. Lande, The Cedar Falls Groundwater Investigation, Iowa Department of Natural Resources, 2012 Search PubMed.
- B. E. Flanagan, E. W. Gregory, E. J. Hallisey and J. L. Heitgerd,
et al., A Social Vulnerability Index for Disaster Management, J. Homel. Secur. Emerg. Manag., 2011, 8(1), 3 Search PubMed , available from: https://www.atsdr.cdc.gov/place-health/media/pdfs/2024/07/Flanagan_2011_SVIforDisasterManagement-508.pdf.
-
Iowa Environmental Council, Drought & Iowa's Drinking Water, 2024 Search PubMed.
-
USEPA, The Benefits of Reducing Nitrate Contamination in Private Domestic Wells Under CAFO Regulatory Options [Internet], Washington, DC, 2002, available from: https://www3.epa.gov/npdes/pubs/cafo_benefit_nitrate.pdf Search PubMed.
- B. H. Jacobsen, B. Hansen and J. Schullehner, Health-economic valuation of lowering nitrate standards in drinking water related to colorectal cancer in Denmark, Sci. Total Environ., 2024, 906, 167368 CrossRef CAS PubMed.
|
This journal is © The Royal Society of Chemistry 2025 |
Click here to see how this site uses Cookies. View our privacy policy here.