DOI:
10.1039/D4FO04832F
(Paper)
Food Funct., 2025, Advance Article
The impact of a walnut-rich breakfast on cognitive performance and brain activity throughout the day in healthy young adults: a crossover intervention trial†
Received
2nd October 2024
, Accepted 31st January 2025
First published on 3rd February 2025
Abstract
A healthy diet is essential for optimal brain health and many bioactives from food are linked to cognitive benefits. To examine whether walnuts led to cognitive improvements throughout the day, 32 healthy young adults, aged 18–30, were tested in a double-blind, crossover pilot study, to compare the effects of a breakfast containing 50 g walnuts with a calorie-matched control containing no nuts. Cognition, mood, blood, and EEG measures were recorded at baseline and 2, 4, and 6 hours postprandially. Mood ratings for negative affect appeared worse following walnuts compared to control, possibly due to a general dislike of the intervention. However, walnuts elicited faster reaction times throughout the day on executive function tasks. Memory recall performance at 2 hours was worse for walnuts compared to control, but by 6 hours this finding was reversed with walnuts outperforming the control. EEG PSD analysis revealed differences in frontoparietal activity in the walnut condition compared to the control condition during memory recall and during executive function performance. These results may reflect between-treatment differences in cognitive effort or attentional processes while performing the tasks. Blood analysis revealed lower circulating non-esterified fatty acids and small increases in glucose availability following consumption of walnuts compared to control, suggesting enhanced glucose availability as one possible mechanism of action. Further investigations of other potential mechanisms are needed. Overall, these findings provide evidence for reaction time benefits throughout the day following a walnut-rich breakfast, while memory findings were mixed with benefits only observed later in the day. However, more research is needed into how diets containing walnuts might regulate cognitive improvements in humans postprandially and over the longer term.
Introduction
Diet represents one of the most important lifestyle factors that can strongly influence cognitive functioning. Thus, a well-balanced diet, rich in nutrients, can improve cognitive function throughout life.1 One class of foods that has been linked with beneficial cognitive wellbeing is the nut family, with several epidemiological studies highlighting a positive relationship between regular nut consumption and cognitive function. For example, epidemiology studies such as the Doetinchem Cohort Study found that levels of nut consumption positively predicted memory, processing speed, cognitive flexibility, and global cognitive function in middle-aged adults,2 while data from the Nurses’ Health Study showed that older women consuming 5 or more servings of nuts per week had better cognitive function than non-consumers, along with an indication that walnuts positively contributed to this effect.3 Pre-clinical studies have also demonstrated the cognitive benefits of walnut supplementation. Studies in rodents have found that walnuts facilitated improved working memory performance in the Morris Water Maze task4 and improved Elevated-Plus and Radial Arm maze learning and memory performance.5
Despite the promising epidemiology and pre-clinical work, relatively few human clinical trials have investigated the cognitive effects of walnuts. Cognitive benefits have been observed on learning, memory, and global cognition in older adults,6,7 and to inferential reasoning and academic stress in young adults,8,9 following daily doses of whole walnuts ranging from 30–60 g per day, for durations ranging from 8 weeks to 6 months. However, not all studies have supported these benefits to cognition10,11 suggesting these effects may be age- or dose-dependent, or specific to certain cognitive domains. Nevertheless, neurochemical and physiological changes have also been observed following regular walnut consumption, such as increased levels of brain derived neurotrophic factor (BDNF),12 reduced blood pressure (suggestive of improved vascular function),13 a lowering of LDL cholesterol,14 and improved gut microbiota diversity,9 suggesting several possible mechanisms for improved cognitive function. Walnuts have also been noted for their benefits to postprandial satiety, through regulation of the postprandial glucose response, providing an additional mechanism that may benefit cognition.15
Cognitive benefits of walnuts may be due to their specific nutrient content as they are known to be high in omega-3 fatty acids (n3-FA), particularly α-linolenic acid (ALA);16 protein/peptides;17,18 and flavonoids, a class of polyphenols.19 There is a growing body of evidence that flavonoid-rich interventions have a positive effect on cognitive function.20–23 Similarly, evidence from supplementation studies across the lifespan have demonstrated beneficial effects of consumption of n3-FA24–26 and of nut peptides17,27 on cognitive performance and mood. There is also some evidence that the n3-FA, protein/peptide, and flavonoid content of walnuts may work together in a synergistic way;28 it is known that proteins/peptides regulate the absorption of fats,29 and the presence of fats increase absorption of vitamin E, carotenoids, and flavonoids.30 To date, however, clinical research specifically investigating cognitive benefits of walnut interventions remains limited and has focused on daily supplementation over a sustained period, with little consideration of acute benefits in the immediate postprandial period. Furthermore, no research has considered acute neurological or physiological outcomes of walnut intervention in concert with cognitive, and mood outcomes. A recent systematic review,31 examining the effects of walnuts on cognitive function, highlighted the need for further gold standard randomized controlled trials (RCTs) to be carried out to fully understand the benefits of walnut consumption. The review also highlighted the importance of concurrent measurement of biological and physiological parameters to allow determination of underlying mechanisms.
Other nuts and flavonoid-rich interventions such as blueberries have demonstrated acute cognitive and mood benefits.22,32–34 Possible mechanisms of action include vascular, glucoregulatory, and neurochemical effects,22 which are also potential physiological actions of walnuts. Therefore, the main objective of the current study was to determine the cognitive and mood effects of acute consumption of a walnut-rich breakfast in healthy young adults throughout the day and, secondly, to investigate potential neurological and physiological mechanisms that may explain any cognitive benefits.
Methods
Ethics statement
This study was conducted according to the guidelines laid down in the Declaration of Helsinki and all procedures involving human subjects were approved by the University of Reading Research Ethics Committee; UREC 19/37. Written informed consent was obtained from all subjects. The trial was registered at clinicaltrials.gov (identifier: NCT04075448). CONSORT reporting guidelines were followed.32
Design
A cross-over study design was used to examine the acute effects of walnut consumption on cognitive performance and mood in healthy young adults. Participants were counterbalanced to consume the walnuts on either the first or second test visit, with the control intervention consumed on the other, and with a one-week separation between the 2 test visits. The study was conducted double-blind and the true nature of the study was initially concealed from participants who were told the study was investigating different breakfast cereals; a full debrief was provided at the end of the study. Cognitive and EEG measures were taken at baseline prior to consumption of the intervention, then again at 2-, 4- and 6-hours following consumption (spanning the expected postprandial timings for the appearance of circulating walnut metabolites33). Blood samples were taken at baseline and at either 2-, 4- or 6-hours post-consumption of the intervention (counterbalanced across the sample but remaining consistent across visits for each participant). An overview of the complete trial design is shown in Fig. 1.
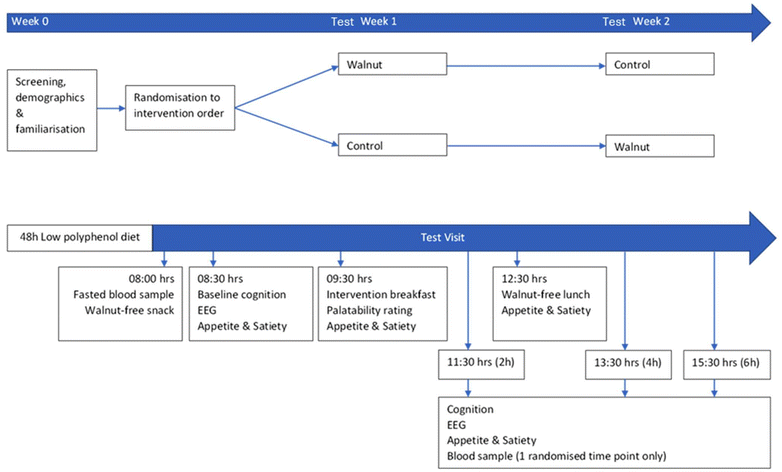 |
| Fig. 1 A crossover study design with acute supplementation of a walnut or control breakfast and cognitive testing at baseline and throughout a 6 h postprandial period, with concurrent EEG recording. | |
To reduce the impact of practice effects, all participants attended a separate screening and familiarisation visit one week prior to beginning the trial, where they practiced the cognitive tests. Prior to each test visit, participants followed diet and lifestyle restrictions, which involved avoidance of nuts, seeds, oily fish, and flavonoid-rich fruits and vegetables for 48 hours and avoiding vigorous exercise and any alcohol or caffeine for 24 hours (dietary compliance was monitored via food diary). They attended all test visits in a fasted state, and each received a standardised snack (croissant) and lunch (cheese sandwich and crisps) in addition to the intervention breakfast. During all breaks in testing, participants rested within the vicinity of the laboratory.
Participants
Participants were healthy, young adult students aged 18 to 30 years. Eligibility exclusion criteria included BMI ≥30, smoking, the use of any medication other than oral contraceptives, food allergies, and following any form of restrictive diet such as being vegan or vegetarian.
Interventions
Both the walnut and control interventions were administered in the form of a breakfast (see Table 1 for the composition of the walnut and control interventions). The walnut intervention consisted of 50 g of crushed walnuts mixed with muesli and vanilla yoghurt. The control also consisted of muesli and vanilla yoghurt but with the addition of melted butter to match the total fat content of the walnuts. Overall, the interventions were well-matched in terms of macro-nutrient content, total weight, and total number of calories. The walnut intervention contained approximately 4 g of omega-3 fatty acids and 1 g of polyphenols, whereas these were negligible in the control intervention. The walnut intervention also contained an additional 5 g protein (attributable to walnut protein) not found in the control.
Table 1 Composition of walnut and control breakfast interventions
|
Walnut composition |
Control composition |
Ingredients |
Walnuts |
50 g |
— |
Muesli |
25 g |
35 g |
Vanilla yoghurt |
150 g |
150 g |
Butter |
— |
40 g |
Macro-nutrient content |
Carbohydrate |
43 g |
44 g |
(incl. fibre) |
5 g |
4 g |
Protein |
17 g |
12 g |
Fat |
35 g |
36 g |
Calories |
549 kcal |
548 kcal |
Total weight |
225 g |
225 g |
Actives |
Estimated n-3s34 |
4 g |
— |
Estimated polyphenols35 |
1 g |
— |
Primary outcomes: cognition & mood
Primary cognitive outcomes included measures of memory, executive function, and mood, presented in the following order:
Auditory verbal learning task (AVLT). This episodic memory task was a multi-trial word list learning task with immediate and delayed free recall, and delayed recognition components.36–38 Dependent variables were number of correctly recalled or identified words (out of 15) at each time point.
Modified attention network task (MANT). This executive function task was a modified version of the Attention Network Task,39 used to investigate multiple facets of attention.40,42,43 The dependent variables were average reaction time (ms) and accuracy (% correct expressed as a decimal) under varying congruency and load conditions.
Switching task (TST). In this previously published executive function task,40 participants observed a stimulus digit and discerned whether the stimulus was odd/even, or higher/lower than 5 depending on its location, switching every 4 trials. The dependent variables were average reaction time (ms) and accuracy (% correct expressed as a decimal).
PANAS-now. In this mood questionnaire,41 participants rated 20 mood adjectives (10 positive and 10 negative) on a scale of 1–5. Dependent variables were composite positive affect and negative affect scores (each out of 50). An additional rating of mental fatigue used a 9-point scale. This dependent variable (scored out of 9) was used as an indicator of cognitive fatigue.42 These subjective mood variables were recorded at the end of each cognitive test battery.
Secondary outcomes: satiety & appetite, EEG, blood sampling
Secondary outcomes included satiety and appetite ratings, EEG measures and blood sampling as follows:
Satiety and appetite. These subjective measures were assessed using visual analogue scales43 at baseline, following consumption of the intervention breakfast and lunch, at each of the post-intervention timepoints, and at the end of each study visit. For appetite measures of fatty, salty, savoury, or sugary cravings, lower scores indicated higher craving. Palatability ratings were also recorded immediately after consuming the intervention breakfast in order to check for any perceived differences in the matched interventions. For palatability ratings of smell and taste, higher scores indicated worse ratings.
EEG. Electrical brain activity was measured using EEG. All participants were tested in our dedicated lab within the Reading University Centre for Integrative Neuroscience and Neurodynamics (CINN) using the Brain Products ActiCap EEG system with 32 channel active electrode caps. Electrode positioning on the cap was in accordance with the international 10–20 system. To ensure an adequate EEG signal, impedances were adjusted to be <10 kΩ. EEG data was collected at each cognitive testing time point, for the full duration of each of the cognitive tasks (AVLT, MANT and TST). Previously planned analysis of event-related potentials (ERPs) was put aside due to poor clarity/resolution of N200 and P300 peaks (likely due to visual complexities within the task designs). EEG data were instead analysed to determine any between-intervention difference in power spectral density (PSD; μV2 Hz−1) for alpha, theta, gamma and delta wave bands, thought to be associated with internal thought processes,44 memory encoding,45 neuronal synchronisation and visual processing,50,51 and inhibition of sensory information as required for optimal internal concentration,46 respectively. The focus of the EEG analysis was on frontal and parietal brain region, as frontoparietal networks are known to be recruited when performing tasks requiring executive functions such as selective attention and cognitive inhibition (MANT), cognitive flexibility including task switching (TST), and working memory including the encoding and retrieval of episodic memories (AVLT).47
Blood sampling. Serum blood samples were collected to measure biomarkers of inflammation, metabolism, and brain function. Blood was drawn from volunteers at baseline and at one post-intervention timepoint, either 2, 4 or 6 hours according to randomisation. This limitation in the blood sampling schedule was due to ethical constraints on the number of samples permitted during a single visit. Biomarkers of brain function and inflammation (BDNF, IL-6 and CRP), as well as metabolic markers (glucose and NEFA), were analysed at baseline and 2, 4 and 6 hours as available, to assess between-intervention differences throughout the day. BDNF and IL-6 were determined using ELISA. CRP, glucose, and NEFA were determined using a RANDOX Daytona Plus clinical chemistry analyser.
Sample size estimation
A power calculation, based on average effect size data from a review of acute cognitive effects following flavonoid supplementation (Cohen's d = 0.55),22 determined that a sample size of 30 would achieve 90% statistical power. To allow for a 10% attrition rate, the aim was to recruit 33 volunteers.
EEG analyses
Raw EEG data: Brain Vision Analyser software was used to convert the raw EEG signal into PSD data for statistical analysis. The raw EEG signal underwent pre-processing using high and low-pass filters (0.3 Hz and 70 Hz respectively) with a notch of 50, to remove interfering frequencies. Ocular correction ICA was used to detect and correct for eye movements. For the PSD analysis, the signal recorded during each cognitive task was segmented using 2 seconds segments with a sampling rate of 2048 Hz. Mean PSD activity for alpha (7.5–12.5 Hz), gamma (30–200 Hz), delta (0.5–3.5 Hz) and theta (3.5–7.5 Hz) wave bands was determined using Fast Fourier Transform.
Statistical analyses
All data were analysed using IBM SPSS statistics version 27. A similar statistical approach was taken for all cognitive, mood, EEG, appetite and satiety, and all biological outcomes. For reaction time data, only correct responses were included in mean values. For all data, z-score analysis was used to identify outliers; datapoints with z score >3.29 were removed prior to statistical analysis.48 Prior to running linear mixed models (LMM), paired t-tests confirmed that there were no significant baseline differences in cognitive performance between each of the treatment conditions (see ESI Table S1†). Subsequently, maximum likelihood LMM with an unstructured covariance matrix was used to analyse data for all endpoints, with baseline performance included as a repeated covariate. Treatment (intervention: walnut or control) was included as a fixed factor. Time was also included as a repeated factor when modelling successive test sessions (in the case of outcomes from serum analysis, time was an independent factor as each participant only provided blood samples at a single postprandial time point counterbalanced across participants). Palatability outcomes were not measured over time, therefore analysis of these ratings only included comparison between the two treatment conditions. Other factors were added to the statistical model where relevant. For example, analysis of AVLT rate of acquisition included recall trial number; the delayed AVLT recognition measure included word type; congruency and cognitive load were included for the MANT task; and trial type was included for TST. Post-hoc pairwise comparisons were used to investigate any significant effects of Treatment. A Bonferroni correction was applied to all multiple comparisons. Only significant main effects and interactions along with significant pairwise comparisons (p < 0.05) are reported.
In addition to LMM, Pearson r correlations between cognitive endpoints and biochemical parameters were determined for each intervention type (Walnut or Control). Change from baseline (CFB) at each post-intervention timepoint was calculated for each cognitive measure and biochemical parameter. The CFB cognitive data was correlated with the corresponding biochemical data according to the post-intervention blood sampling timepoint for each volunteer.
Results
31 participants successfully completed the full study, mean age 20 years (SD 1.57), 7 male. An additional participant withdrew before completing the final test session. The trial ran from November 2019 to October 2020 (with a break from March 2020 to August 2020 due to Covid restrictions) and ended when the recruitment target was met. No harms relating to the control or walnut interventions were reported. The consort diagram is shown in Fig. 2. All raw data can be found in the ESI.†
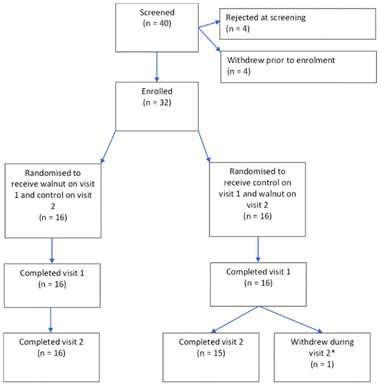 |
| Fig. 2 Consort diagram showing the numbers of participants screened, enrolled, and completed. *Participant fainted during blood sampling. | |
Cognitive behaviour & EEG results
All cognitive data are summarised in ESI Table S2.† EEG data are summarised in ESI Table S4.†
AVLT (cognitive)
For the immediate recall rate of acquisition analysis, a significant treatment by time interaction was observed (F(2, 442) = 7.79, p < 0.001), with pairwise comparisons revealing fewer words recalled following the walnut intervention (M = 9.98 words) compared to the control (M = 10.49 words) at 2 h (p = 0.003). However, this finding was reversed at 6 hours when performance was better following the walnut intervention (M = 10.75 words) compared to the control (M = 10.32 words) (p = 0.015). Additional within-treatment changes in performance were also observed over time as shown in Fig. 3A. Notably, recall performance improved between 2 hours and 4 hours (p = 0.007), and between 2 hours and 6 hours (p < 0.001) following the walnut intervention, whereas performance decreased between 4 hours and 6 hours following the control (p = 0.027). We also saw possible evidence of walnuts positively influencing the rate of acquisition, combined with a protective effect against cognitive fatigue at the 6 h time point, shown in Fig. 3B. Pairwise comparisons conducted for individual recall points (R1–R5) revealed that performance was poorer at 2 hours for the walnut condition relative to the control for R3 and R4 (p = 0.041, p = 0.009, respectively), but at 6 hours walnut outperformed control at R2 (p = 0.038). Significant increases in R2 & R3 were observed between 2 hours & 6 hours following the walnut intervention (p = 0.032, p = 0.046), but a significant decrease in R2 was observed between 4 hours and 6 hours following the control (p = 0.034). No other main effects or treatment interactions were observed to be significant for this AVLT measure, or for any other AVLT measures including immediate word span, learning, total acquisition, final acquisition, proactive and retroactive interference, delayed recall, and word recognition (p > 0.05).
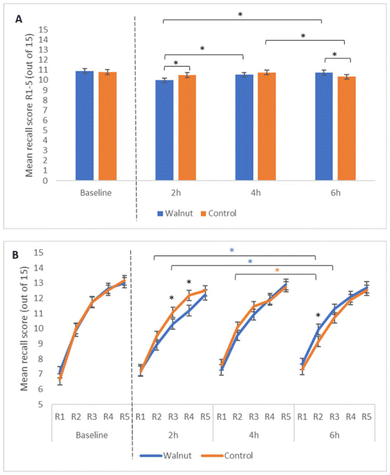 |
| Fig. 3 Treatment effects on AVLT performance. Panel A shows the treatment by time interaction observed for the AVLT immediate recall rate of acquisition analysis, showing mean number of words correctly recalled across 5 recall attempts using the same word list (R1 to R5). Panel B shows performance at each recall point individually. Baseline performance is included as a covariate in the model and is shown in the figure for information only. Error bars represent mean standard error. *Indicates a significant difference between treatments or time points (p < 0.05). | |
AVLT (EEG)
During the encoding and immediate recall phases of the AVLT task, PSD analysis revealed significant main effects of treatment for alpha (F(1, 511) = 7.49, p = 0.006), delta (F(1, 491) = 7.70, p = 0.006) and theta wave bands (F(1, 600) = 5.50, p = 0.019) in the frontal brain region, with greater activity following the walnut compared to the control intervention for all three wave bands (alpha: walnut M = 7.54 μV, control M = 6.25 μV; delta: walnut M = 222.31 μV, control M = 174.02 μV; theta: walnut M = 14.34 μV, control M = 12.17 μV). The same pattern was observed during the delayed recall phase of the task, with main effects of treatment again observed for alpha (F(1, 565) = 8.75, p = 0.003), delta (F(1, 589) = 4.54, p = 0.033) and theta (F(1, 574) = 9.33, p = 0.002) wave bands; greater activity was observed following walnut compared with the control intervention for alpha (walnut M = 8.83 μV, control M = 7.13 μV), delta (walnut M = 229.12 μV, control M = 169.62 μV), and theta wave bands (walnut M = 14.57 μV, control M = 11.56 μV). These findings for theta activity, in conjunction with the similar treatment-related differences in alpha and delta activity, may reflect between-treatment differences in attentional processing during encoding and recall. For delayed recognition, there was a main effect of treatment for gamma band (F(1, 556) = 5.73, p = 0.017), this time with greater activity following the control (M = 0.23 μV) compared to the walnut (M = 0.18 μV) intervention, reflecting possible between-treatment differences in visual stimulus evaluation while performing the task.
EEG effects observed in the parietal region of the brain typically showed a reverse pattern to those seen in the frontal region and were limited to alpha and theta wave bands. During encoding and immediate recall, parietal alpha band mean PSD activity showed a main effect of treatment (F(1, 676) = 6.12, p = 0.01), with activity lower in the walnut condition compared to the control (walnut M = 5.64 μV, control M = 5.97 μV). Similarly for delayed recognition, there were significant main effects of treatment in the parietal region for alpha band (F(1, 711) = 10.66, p = 0.001) and theta band (F(1, 664) = 4.33, p = 0.04) with greater mean PSD activity following the control compared to the walnut intervention (alpha: control M = 4.45 μV, walnut M = 4.17 μV; theta: control M = 5.05 μV, walnut M = 4.82 μV). This reversal may reflect reallocation of resources from parietal to frontal regions during attentional aspects of encoding and recognition (such as paying attention to new stimuli). However, for delayed recall we observed significantly increased alpha band activity (F(1, 738) = 5.33, p = 0.02) in the parietal region following the walnut condition compared to the control condition (alpha band: walnut M = 6.25 μV, control M = 5.83 μV), possibly reflecting the lower external attention demands required for this task and mimicking our findings in the frontal region.
MANT (cognitive)
A main effect of treatment was observed for reaction time during MANT performance (F(1, 456) = 20.55, p < 0.001), such that significantly faster performance was seen following the walnut compared with the control intervention (M = 455.45 ms vs. 463.63 ms, respectively), as shown in Fig. 4A.
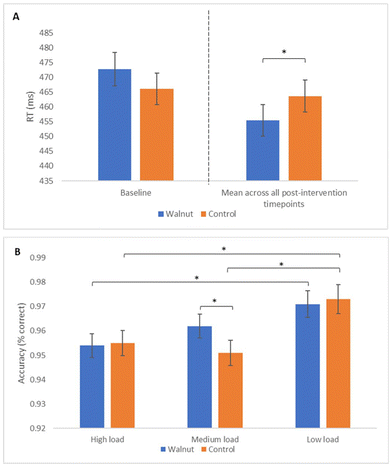 |
| Fig. 4 Treatment effects on MANT performance. Panel A shows a treatment effect on MANT reaction time, while panel B shows a treatment by load interaction for MANT accuracy. Baseline performance is included as a covariate in the model and is shown in panel A for information only. Error bars represent mean standard error. *Indicates a significant difference between treatments (p < 0.05). | |
In addition to the RT effect, there was a significant treatment by load interaction for MANT accuracy (F(1, 411) = 4.75, p = 0.030), with pairwise comparisons revealing better performance following the walnut compared with the control intervention (M = 0.96 vs. 0.95) for medium load trials (p = 0.005). Additional within-treatment differences in performance were also observed over varying load conditions as shown in Fig. 4B.
MANT (EEG)
In the frontal brain region, PSD analysis revealed a significant treatment effect for the alpha wave band (F(1, 352) = 5.21, p = 0.023), with greater mean PSD activity following the walnut compared with the control intervention (M = 9.60 μV vs. M = 8.20 μV), matching the behavioural effects and likely associated with attention/inhibition requirements of the task. No significant findings were observed parietally.
TST (cognitive)
Similar to MANT, there was a main effect of treatment for reaction time during the TST (F(1, 348) = 16.28, p < 0.001) with faster RTs following the walnut intervention compared to the control intervention (M = 764.24 ms vs. 786.31 ms, respectively). However, there were no significant findings for TST accuracy.
TST (EEG)
There were no significant PSD findings for the TST task frontally. However in the parietal region there was a significant main effect of treatment for delta wave band (F(1, 779) = 7.15, p = 0.01), with greater mean PSD activity following the walnut compared to the control breakfast (M = 37.96 μV vs. M = 33.42 μV), possibly associated with task switching costs.
Mood, appetite, & palatability results
Mood data are summarised in ESI Table S3.† Appetite and palatability data are summarised in ESI Table S6.† A significant main effect of treatment for PANAS negative affect score (F(1, 88) = 11.95, p = 0.001) revealed greater negative affect following the walnut (M = 12.42) compared to the control intervention (M = 11.51), but no treatment × time interaction was observed. No significant main effects of treatment or treatment-related interactions were observed for the additional mood measures of positive affect score or fatigue rating (p > 0.05). The unexpected negative affect ratings following the walnut intervention compared to the control may, however, be related to the relative appetite and palatability ratings following each of the interventions. A significant main effect of treatment for ‘Fatty Craving’ (F(1, 187) = 5.11, p = 0.025) revealed that craving for fatty food was significantly greater following the walnut (M = 62.7) compared to the control intervention (M = 65.7). Additionally, the walnut intervention (M = 55.1) was rated as having a worse smell than the control (M = 43.9) intervention (F(1, 32) = 9.48, p = 0.004). No other subjective ratings of appetite or intervention palatability were found to show between-treatment differences (p > 0.05).
Biomarkers of inflammation, metabolism, and brain function
Biological data are summarised in ESI Table S5.† Whilst there were no significant main effects of treatment or treatment interactions for BDNF, IL-6 or CRP (p > 0.05), a significant main effect of treatment was observed for serum concentrations of glucose (F(1, 29) = 5.74, p = 0.023), with higher levels seen following the walnut (M = 5.11) compared to the control intervention (M = 4.87). In addition, a main effect of treatment was also observed for serum concentrations of NEFA (F(1, 27) = 6.24, p = 0.019), with lower concentrations following the walnut (M = 0.24) compared to the control intervention (M = 0.29). No treatment by time interactions were observed.
Correlations between biomarkers and cognitive performance
Correlation matrices are presented in ESI Tables S7 and S8.† The correlations between cognitive performance and biomarkers were examined for each intervention after first calculating change from baseline scores. For the walnut intervention, there were no significant correlations between behavioural and biomarker outcomes. However, for the control intervention, there were two correlations of interest. Firstly, a significant negative correlation between delayed recall and NEFA concentration (r = −0.444, p = 0.030) revealed that post-control increases in NEFA concentration were related to poorer performance on the delayed recall task. Secondly, a significant negative correlation between MANT RT and BDNF (r = −0.533, p = 0.007) revealed that faster reaction times on the MANT were related to increased levels of circulating BDNF. Combined, the biomarker data and correlations with cognitive outcomes offer some potential mechanisms of action.
Discussion
Data from this walnut intervention study has started to address some of the recommendations made in a recent systematic review/meta-analysis,31 by using standardized methodology to investigate the impact of a fixed, easily achievable dose of walnuts on specific cognitive domains, at fixed time points, with concurrent investigation of changes to electrical brain activity and blood parameters. In our study, improvements to executive function were observed following a single 50 g dose of walnuts, equivalent to ∼1.5 ounces, when compared to a calorie-matched control intervention, with faster reaction times observed across the day on cognitive tasks measuring inhibition and task-switching aspects of executive function. However, memory effects were mixed, with worse memory performance observed at 2 h compared with the control (perhaps resulting from mood or motivational issues following consumption of a large walnut breakfast that participants disliked). Memory recall benefits of walnut only appeared at 6 hours, possibly suggestive of a protective effect against cognitive fatigue (although the subjective ratings of mental fatigue recorded at each time point did not reflect this), or that memory benefits depend on the slower absorption of omega 3s and proteins, or the delayed appearance of specific flavonoid metabolites. These delayed benefits to memory were specific to the rate of learning, suggesting that varying mechanisms of action may underlie benefits across different cognitive processes.
In all cases, the magnitude of treatment-related differences in cognitive scores was small but nevertheless relevant. For example, clinical reaction time performance reductions as small as 18 ms can be indicative of concussion in young athletes,49 and small differences in average AVLT memory scores of around 0.2 words have been shown to discriminate brain abnormality from a healthy brain.50 However, these strictly controlled laboratory tasks are not directly comparable to real world behaviours, so further research is needed to see how changes of this magnitude in the laboratory may impact everyday life. Given that AVLT effects were observed specifically for the rate of acquisition analysis, future studies should investigate these learning effects in more detail, perhaps using a word pair learning paradigm or similar learning-based task.
Overall, the acute cognitive benefits observed here are important, demonstrating that walnuts may provide a healthy way of boosting cognition throughout the day, in addition to eliciting longer term cognitive benefits following daily supplementation as shown in previous research.6–9 Interestingly, the current findings are similar to those observed in previous flavonoid research22 where acute benefits to memory and/or executive function have been observed following consumption of citrus juices, berries, cocoa, and ginkgo biloba, therefore it seems plausible that the flavonoid/polyphenol content of the walnuts may be contributing to observed benefits. In previous research, flavonoid benefits have also been linked with high cognitive demand.51 For the MANT and TST tasks investigated here, cognitive demand did not appear to play a role in TST accuracy or reaction time performance, but for MANT, walnuts elicited a significant benefit to accuracy in the medium load condition, therefore it may be that walnuts provide a processing advantage when the brain is under only specific cognitive load conditions, and that future cognitive tasks may need to be carefully tailored to identify these.
The observed cognitive benefits to learning, memory, and executive function were accompanied by changes in frontal and parietal brain activity across alpha, delta, theta, and gamma bands. The frontoparietal network has previously been associated with episodic memory, attention, and executive functions including inhibition and task switching.53,57,58 Theta band, in particular, is thought to be associated with memory encoding45 while delta activity has been associated with task switching,52 alpha activity has been associated with attentional inhibition,53 and gamma has been associated with visual processing.54 The current findings concur with similar postprandial EEG research following nutritional interventions62,63 and confirm that the cognitive tasks used here involve the recruitment of frontoparietal networks, demonstrating that cognitive performance on these tasks was benefitted by acute walnut supplementation.
Whilst the cognitive effects of walnuts observed here seem robust, evidence for the effects of the current walnut intervention on mood were unexpectedly negative. On balance, there was evidence to suggest greater negative affect reported following the walnut intervention. Previous research has observed acute mood benefits following similar flavonoid-rich,33,64 or protein-rich55 interventions, so it is unclear why beneficial mood effects were not observed here. Smell, taste, and palatability of the walnut intervention were rated worse than the control (with differences achieving statistical significance for smell), so it is possible that this may have negatively influenced mood, particularly in the immediate period following consumption. Although there was no significant treatment by time interaction to fully support this theory, meal palatability has previously been shown to impact postprandial mood.56 In addition, self-reported cravings for fatty foods were greater following the walnut intervention compared to the control. However, this may simply be due to the high butter content of the control breakfast which likely reduced fatty cravings disproportionately in this condition, potentially making cravings in the walnut condition appear greater.
Serum analysis found no evidence to suggest that circulating levels of BDNF, IL-6, or CRP were responsible for the observed acute cognitive benefits of walnuts. It is possible that this analysis was underpowered as blood samples could only be collected at a single post-intervention time point for each participant. However, these findings are consistent with a recent review of supplementation studies which did not find any significant benefit of walnut supplementation on CRP or IL-6,31 suggesting that different inflammatory markers may be impacted by walnut, or that effects may be transient, or that alternative mechanisms may be responsible for the acute beneficial effects of walnuts. Despite this lack of effect of the walnut intervention on serum concentrations of inflammatory biomarkers, higher glucose levels were observed in this study following acute walnut consumption. The observed treatment-related differences in glucose levels were small compared to those considered medically relevant to metabolic health, such as in the monitoring of diabetes. However, even small increases in availability may have an impact on the delivery and uptake of glucose to the brain during periods of high cognitive demand,57,58 thereby benefitting postprandial cognitive performance.59 It is also theoretically possible that following walnut consumption participants found the cognitive battery less cognitively demanding, although this was not reflected in participants ratings of mental fatigue following completion of the battery. Increased postprandial glucose levels following the walnut intervention could similarly suggest a smoother and more elongated glucose curve without sharp peaks and troughs, which are characteristic of poor glucose control. Indeed walnuts have previously been shown to impact postprandial glucoregulation.15 However, whilst evidence also suggests that nuts may increase insulin sensitivity,60 a recent review did not find consistent evidence for beneficial effects of walnut consumption on glucose control,61 although the authors noted the need for higher quality randomised controlled trials. As such, it is recommended that future studies test this theory by implementing a greater number of postprandial blood sampling timepoints to establish the glucose response to walnuts over time and determine how these glucose changes relate to the observed timings of postprandial cognitive benefits.
In addition to metabolic observations, a concurrent lowering of NEFA levels following walnut consumption was observed, suggestive of a possible postprandial omega 3 effect,62 due to the lower saturated fat content and higher PUFA content (including omega-3s) of the walnut intervention compared with the control intervention. Regulating saturated fatty acid intake may have further implications for cognitive performance as cardiovascular health is strongly associated with cerebrovascular function.63 Indeed previous research has observed benefits to blood pressure in the immediate postprandial period following omega 3, although cognitive benefits failed to reach significance in this pilot investigation.64 NEFA levels reported in the current study include both saturated and unsaturated fatty acids, and therefore further studies are recommended to confirm whether acute walnut consumption can specifically reduce circulating levels of saturated fatty acids. Emerging research also suggests that long-term consumption of foods rich in polyphenols, beneficial fats, and fibre can improve the composition and diversity of the gut microbiome.65 Indeed such an effect has been observed following walnut consumption.9,66 These benefits to the gut are likely to result in improved digestion and absorption with resulting increases in the availability of beneficial metabolites such as SCFA66 that provide an additional mechanism for the enhancement of cognitive function following nutritional intervention through enhanced gut–brain communication. SCFAs are also thought to play a role in the modulation of satiety.67 While these gut-based effects are unlikely to emerge following only a single dose of walnuts, they are likely to be relevant during long-term supplementation and therefore warrant future investigation.
In addition to the individual analysis of each biomarker, correlations were performed to investigate possible relationships between the biomarkers and cognitive performance. These did not reveal any consistent support for the mechanisms proposed here. Associations were found between increased serum concentrations of NEFA and worsening of delayed recall performance, and between improved performance on the MANT and increased serum concentrations of BDNF, but only within the control condition. A possible explanation for a lack of correlation within the walnut condition might be the slightly higher variance inherent in the walnut data. Irrespective, the observed correlational relationships should be interpreted with caution as only behavioural data corresponding to the post-intervention blood sampling timepoint for each volunteer could be included in the analysis. These small sub-samples for each timepoint likely resulted in lower statistical power to determine any correlation between cognitive performance and serum biomarkers within each intervention condition. Nevertheless, it appears promising that NEFA concentrations following the walnut intervention were not associated with detriments to delayed recall, whereas this was the case for the control intervention.
Conclusions
This was the first acute walnut supplementation trial to be conducted in healthy young adults. There are several strengths to the study design, including the double-blind, controlled, within-subjects methodology in addition to the mechanistic approach. The findings are promising, with evidence for a possible protective effect on memory from the typical decline in rate of learning often observed with fatigue, in addition to faster reaction times when completing executive function tasks. These cognitive benefits occurred concurrently with changes in PSD brain activity, potentially indicating more efficient allocation of neural resources following consumption of walnuts. The biochemical mechanisms responsible for these effects remain to be fully elucidated but may relate to improved glucose availability and reduced circulating free fatty acids. Whilst the use of whole foods prevents the ability to form any strong conclusions as to the active ingredients responsible for the observed effects, it is likely that walnuts may confer their benefits due to their protein/peptide, flavonoid and/or omega-3 content, with a growing body of evidence demonstrating the cognitive benefits that supplementation with these bioactives can have.17,20,22,24,25,68 This area of research warrants further investigation, to establish mechanisms of action, and to confirm benefits of longer-term supplementation in this population. Furthermore, by ensuring the continued use of rigorous methodological protocols for the investigation of walnut-induced changes in cognition and physiology/biochemistry, a stronger evidence base is likely to emerge for the benefits of walnuts, as recently recommended.31 A caveat to the findings is that a majority (78%) of participants were female, so reported effects may not generalise to a male population. Appropriate investigation of sex differences should be included in future research. Finally, it remains to be seen whether greater benefit would be evident in at risk populations such as older individuals, as well as clinical populations such as those with cognitive impairment or metabolic syndrome.
Author contributions
C. M. W., B. S.-H., and A. R. W. designed the study. L. B., G. F. D., and A. R. W. conducted the study. L. B., G. F. D., and M. J. analysed the data. G. F. D. and D. F. analysed the blood samples. L. B., and G. F. D. performed all statistical analyses. L. B., G. F. D., and A. R. W. drafted the paper. C. M. W. had primary responsibility for the final content. All authors contributed to the writing of the paper, and all have approved the final version of the manuscript.
Data availability
The current data collected from human participants are not available publicly for confidentiality reasons, but they are available from the corresponding authors upon reasonable request.
Conflicts of interest
There are no conflicts to declare.
Acknowledgements
The authors thank all participants who contributed to the study. The study was funded by the California Walnut Commission, USA. The funder made no contribution during the design or implementation of the study, nor in the interpretation of findings or the decision to publish.
References
- M. G. Miller, N. Thangthaeng, S. M. Poulose and B. Shukitt-Hale, Role of fruits, nuts, and vegetables in maintaining cognitive health, Exp. Gerontol., 2017, 94, 24–28 CrossRef CAS PubMed.
- A. C. Nooyens, H. B. Bueno-de-Mesquita, M. P. van Boxtel, B. M. van Gelder, H. Verhagen and W. M. Verschuren, Fruit and vegetable intake and cognitive decline in middle-aged men and women: the Doetinchem Cohort Study, Br. J. Nutr., 2011, 106, 752–761 CrossRef CAS PubMed.
- J. O'Brien, O. Okereke, E. Devore, B. Rosner, M. Breteler and F. Grodstein, Long-term intake of nuts in relation to cognitive function in older women, J. Nutr., Health Aging, 2014, 18, 496–502 CrossRef PubMed.
- L. M. Willis, B. Shukitt-Hale, V. Cheng and J. A. Joseph, Dose-dependent effects of walnuts on motor and cognitive function in aged rats, Br. J. Nutr., 2008, 101, 1140–1144 CrossRef PubMed.
- S. Haider, Z. Batool, S. Tabassum, T. Perveen, S. Saleem, F. Naqvi, H. Javed and D. J. Haleem, Effects of walnuts (Juglans regia) on learning and memory functions, Plant Foods Hum. Nutr., 2011, 66, 335–340 CrossRef CAS PubMed.
- E. H. Martínez-Lapiscina, P. Clavero, E. Toledo, R. Estruch, J. Salas-Salvadó, B. San Julián, A. Sanchez-Tainta, E. Ros, C. Valls-Pedret and M. Á. Martinez-Gonzalez, Mediterranean diet improves cognition: the PREDIMED-NAVARRA randomised trial, J. Neurol., Neurosurg. Psychiatry, 2013, 84, 1318–1325 CrossRef PubMed.
- C. Valls-Pedret, A. Sala-Vila, M. Serra-Mir, D. Corella, R. De la Torre, M. Á. Martínez-González, E. H. Martínez-Lapiscina, M. Fitó, A. Pérez-Heras and J. Salas-Salvadó, Mediterranean diet and age-related cognitive decline: a randomized clinical trial, JAMA Intern. Med., 2015, 175, 1094–1103 CrossRef.
- P. Pribis, R. N. Bailey, A. A. Russell, M. A. Kilsby, M. Hernandez, W. J. Craig, T. Grajales, D. J. Shavlik and J. Sabate, Effects of walnut consumption on cognitive performance in young adults, Br. J. Nutr., 2012, 107, 1393–1401 CrossRef CAS PubMed.
- M. F. Herselman, S. Bailey, P. Deo, X.-F. Zhou, K. M. Gunn and L. Bobrovskaya, The effects of walnuts and academic stress on mental health, general well-being and the gut microbiota in a sample of university students: A randomised clinical trial, Nutrients, 2022, 14, 4776 CrossRef CAS PubMed.
- A. Pinar-Martí, F. Gignac, S. Fernández-Barrés, D. Romaguera, A. Sala-Vila, I. Lázaro, O. T. Ranzani, C. Persavento, A. Delgado and A. Carol, Effect of walnut consumption on neuropsychological development in healthy adolescents: a multi-school randomised controlled trial, eClinicalMedicine, 2023, 59, 101954 CrossRef PubMed.
- A. Sala-Vila, C. Valls-Pedret, S. Rajaram, N. Coll-Padrós, M. Cofán, M. Serra-Mir, A. M. Pérez-Heras, I. Roth, T. M. Freitas-Simoes and M. Doménech, Effect of a 2-year diet intervention with walnuts on cognitive decline. The Walnuts And Healthy Aging (WAHA) study: a randomized controlled trial, Am. J. Clin. Nutr., 2020, 111, 590–600 CrossRef PubMed.
- A. Sánchez-Villegas, C. Galbete, M. Á. Martinez-González, J. A. Martinez, C. Razquin, J. Salas-Salvadó, R. Estruch, P. Buil-Cosiales and A. Martí, The effect of the Mediterranean diet on plasma brain-derived neurotrophic factor (BDNF) levels: the PREDIMED-NAVARRA randomized trial, Nutr. Neurosci., 2011, 14, 195–201 CrossRef PubMed.
- M. Domènech, M. Serra-Mir, I. Roth, T. Freitas-Simoes, C. Valls-Pedret, M. Cofán, A. López, A. Sala-Vila, C. Calvo and S. Rajaram, Effect of a walnut diet on office and 24-hour ambulatory blood pressure in elderly individuals: findings from the WAHA randomized trial, Hypertension, 2019, 73, 1049–1057 CrossRef PubMed.
- S. Rajaram, M. Cofán, A. Sala-Vila, E. Haddad, M. Serra-Mir, E. Bitok, I. Roth, T. M. Freitas-Simoes, A. Kaur and C. Valls-Pedret, Effects of walnut consumption for 2 years on lipoprotein subclasses among healthy elders: findings from the WAHA randomized controlled trial, Circulation, 2021, 144, 1083–1085 CrossRef PubMed.
- M. Zibella and M. Parillo, Effects of nuts on postprandial glycemia, satiety and hunger sensations in healthy individuals, Mediterr. J. Nutr. Metab., 2017, 10, 243–249 Search PubMed.
- L. Maguire, S. O'sullivan, K. Galvin, T. O'connor and N. O'brien, Fatty acid profile, tocopherol, squalene and phytosterol content of walnuts, almonds, peanuts, hazelnuts and the macadamia nut, Int. J. Food Sci. Nutr., 2004, 55, 171–178 CrossRef CAS PubMed.
- A.-L. Yeh, C.-L. Chao, W.-F. Huang, H.-C. Lin and C.-J. Wang, Walnut (Juglans regia L.) oligopeptide effects on enhancing memory, cognition and improving sleep quality in teenagers and elderly people in a randomized double-blind controlled trial, Nat. Prod. Commun., 2022, 17, 1934578X221089065 CrossRef CAS.
- J. Wang, J. Liu, A. John, Y. Jiang, H. Zhu, B. Yang and L. Wen, Structure identification of walnut peptides and evaluation of cellular antioxidant activity, Food Chem., 2022, 388, 132943 CrossRef CAS PubMed.
- J. Yang, R. H. Liu and L. Halim, Antioxidant and antiproliferative activities of common edible nut seeds, LWT–Food Sci. Technol., 2009, 42, 1–8 CrossRef CAS.
- D. J. Lamport, L. Dye, J. D. Wightman and C. L. Lawton, The effects of flavonoid and other polyphenol consumption on cognitive performance: a systematic research review of human experimental and epidemiological studies, Nutr. Aging, 2012, 1, 5–25 Search PubMed.
- N. Cheng, L. Bell, D. J. Lamport and C. M. Williams, Dietary flavonoids and human cognition: a meta–analysis, Mol. Nutr. Food Res., 2022, 2100976 CrossRef CAS PubMed.
- L. Bell, D. J. Lamport, L. T. Butler and C. M. Williams, A review of the cognitive effects observed in humans following acute supplementation with flavonoids, and their associated mechanisms of action, Nutrients, 2015, 7, 10290–10306 CrossRef CAS PubMed.
- T.-S. Yeh, C. Yuan, A. Ascherio, B. A. Rosner, W. C. Willett and D. Blacker, Long-term dietary flavonoid intake and subjective cognitive decline in US men and women, Neurology, 2021, 97, e1041–e1056 CAS.
- I. Bauer, M. Hughes, R. Rowsell, R. Cockerell, A. Pipingas, S. Crewther and D. Crewther, Omega-3 supplementation improves cognition and modifies brain activation in young adults, Hum. Psychopharmacol., 2014, 29, 133–144 CrossRef CAS PubMed.
- W. Stonehouse, C. A. Conlon, J. Podd, S. R. Hill, A. M. Minihane, C. Haskell and D. Kennedy, DHA supplementation improved both memory and reaction time in healthy young adults: a randomized controlled trial, Am. J. Clin. Nutr., 2013, 97, 1134–1143 CrossRef CAS PubMed.
- E. Derbyshire, Brain health across the lifespan: a systematic review on the role of omega-3 fatty acid supplements, Nutrients, 2018, 10, 1094 CrossRef PubMed.
- W. Wu, B. Niu, L. Peng, Q. Chen, H. Chen, H. Chen, W. Xia, L. Jin, J. Simal-Gandara and H. Gao, Recent advances on the effect of nut consumption on cognitive improvement, Food Front., 2023, 4, 1737–1746 CrossRef.
- N. Pahlavani, D. Rostami, F. Ebrahimi and F. Azizi-Soleiman, Nuts effects in chronic disease and relationship between walnuts and satiety: review on the available evidence, Obes. Med., 2020, 17, 100173 CrossRef.
- S. Nagaoka, A. Takeuchi and A. Banno, Plant-derived peptides improving lipid and glucose metabolism, Peptides, 2021, 142, 170577 CrossRef CAS.
- M. Morris, D. Evans, C. Tangney, J. Bienias and R. Wilson, Associations of vegetable and fruit consumption with age-related cognitive change, Neurology, 2006, 67, 1370–1376 CrossRef CAS PubMed.
- D. Cahoon, S. P. Shertukde, E. E. Avendano, J. Tanprasertsuk, T. M. Scott, E. J. Johnson, M. Chung and N. Nirmala, Walnut intake, cognitive outcomes and risk factors: a systematic review and meta-analysis, Ann. Med., 2021, 53, 971–997 Search PubMed.
- K. Dwan, T. Li, D. G. Altman and D. Elbourne, CONSORT 2010 statement: extension to randomised crossover trials, Br. Med. J., 2019, 366, 14378 Search PubMed.
- E. H. Haddad, N. Gaban-Chong, K. Oda and J. Sabaté, Effect of a walnut meal on postprandial oxidative stress and antioxidants in healthy individuals, Nutr. J., 2014, 13, 1–9 CrossRef.
- R. A. McCance and E. M. Widdowson, McCance and Widdowson's the Composition of Foods, Royal Society of Chemistry, 2014 Search PubMed.
- J. A. Vinson and Y. Cai, Nuts, especially walnuts, have both antioxidant quantity and efficacy and exhibit significant potential health benefits, Food Funct., 2012, 3, 134–140 RSC.
- M. D. Lezak, D. B. Howieson and D. W. Loring, Neuropsychological assessment, Oxford University Press, New York, 2004 Search PubMed.
- A. R. Whyte, N. Cheng, E. Fromentin and C. M. Williams, A Randomized, Double-Blinded, Placebo-Controlled Study to Compare the Safety and Efficacy of Low Dose Enhanced Wild Blueberry Powder and Wild Blueberry Extract (ThinkBlue) in Maintenance of Episodic and Working Memory in Older Adults, Nutrients, 2018, 10, 660 CrossRef PubMed.
- A. R. Whyte, G. Schafer and C. M. Williams, Cognitive effects following acute wild blueberry supplementation in 7- to 10-year-old children, Eur. J. Nutr., 2016, 55, 2151–2162 CrossRef CAS PubMed.
- J. Fan, B. D. McCandliss, T. Sommer, A. Raz and M. I. Posner, Testing the efficiency and independence of attentional networks, J. Cognit. Neurosci., 2002, 14, 340–347 CrossRef PubMed.
- M. G. Miller, D. A. Hamilton, J. A. Joseph and B. Shukitt-Hale, Dietary blueberry improves cognition among older adults in a randomized, double-blind, placebo-controlled trial, Eur. J. Nutr., 2018, 57, 1169–1180 CrossRef CAS PubMed.
- D. Watson, L. A. Clark and A. Tellegen, Development and validation of brief measures of positive and negative affect: The PANAS scales., J. Abnorm. Psychol. Soc. Psychol., 1988, 54, 1063–1070 CAS.
- R. Holtzer, M. Shuman, J. R. Mahoney, R. Lipton and J. Verghese, Cognitive fatigue defined in the context of attention networks, Aging Neuropsychol. Cognit., 2010, 18, 108–128 CrossRef PubMed.
- A. Flint, A. Raben, J. Blundell and A. Astrup, Reproducibility, power and validity of visual analogue scales in assessment of appetite sensations in single test meal studies, Int. J. Obes., 2000, 24, 38 CrossRef CAS PubMed.
- M. Benedek, R. J. Schickel, E. Jauk, A. Fink and A. C. Neubauer, Alpha power increases in right parietal cortex reflects focused internal attention, Neuropsychologia, 2014, 56, 393–400 CrossRef PubMed.
- E. Nyhus and T. Curran, Functional role of gamma and theta oscillations in episodic memory, Neurosci. Biobehav. Rev., 2010, 34, 1023–1035 CrossRef PubMed.
- T. Harmony, The functional significance of delta oscillations in cognitive processing, Front. Integr. Neurosci., 2013, 7, 83 Search PubMed.
- M. Scolari, K. N. Seidl-Rathkopf and S. Kastner, Functions of the human frontoparietal attention network: Evidence from neuroimaging, Curr. Opin. Behav. Sci., 2015, 1, 32–39 CrossRef PubMed.
- B. G. Tabachnick and L. S. Fidell, Using Multivariate Statistics, Pearson, Boston, MA, 2013 Search PubMed.
- J. B. Caccese, J. T. Eckner, L. Franco-MacKendrick, J. B. Hazzard, M. Ni, S. P. Broglio, T. McAllister, M. McCrea, P. F. Pasquina and T. A. Buckley, Clinical reaction time after concussion: change from baseline versus normative-based cutoff scores, J. Athl. Train., 2020 DOI:10.4085/JAT0457-20.
- E. Z. Zimmer, L. Lowenstein, M. Bronshtein, D. Goldsher and J. Aharon-Peretz, Clinical significance of isolated mega cisterna magna, Arch. Gynecol. Obstet., 2007, 276, 487–490 CrossRef PubMed.
- A. R. Whyte, G. Schafer and C. M. Williams, The effect of cognitive demand on performance of an executive function task following wild blueberry supplementation in 7 to 10 years old children, Food Funct., 2017, 8, 4129–4138 RSC.
- M. E. López, S. Pusil, E. Pereda, F. Maestú and F. Barceló, Dynamic low frequency EEG phase synchronization patterns during proactive control of task switching, NeuroImage, 2019, 186, 70–82 CrossRef PubMed.
- J. J. Foxe and A. C. Snyder, The role of alpha-band brain oscillations as a sensory suppression mechanism during selective attention, Front. Psychol., 2011, 2, 154 Search PubMed.
- M. M. Müller, T. Gruber and A. Keil, Modulation of induced gamma band activity in the human EEG by attention and visual information processing, Int. J. Psychophysiol., 2000, 38, 283–299 CrossRef.
- Y.-C. Zeng, S.-M. Li, G.-L. Xiong, H.-M. Su and J.-C. Wan, Influences of protein to energy ratios in breakfast on mood, alertness and attention in the healthy undergraduate students, Health, 2011, 3, 383 CrossRef CAS.
- T. Pribic, L. Hernandez, A. Nieto, C. Malagelada, A. Accarino and F. Azpiroz, Effects of meal palatability on postprandial sensations, Neurogastroenterol. Motil., 2018, 30, e13248 CrossRef PubMed.
- D. L. Korol and P. E. Gold, Glucose, memory, and aging, Am. J. Clin. Nutr., 1998, 67, 764S–771S CrossRef CAS PubMed.
- A. B. Scholey, S. Harper and D. O. Kennedy, Cognitive demand and blood glucose, Physiol. Behav., 2001, 73, 585–592 CrossRef CAS PubMed.
- A. Nilsson, K. Radeborg and I. Björck, Effects on cognitive performance of modulating the postprandial blood glucose profile at breakfast, Eur. J. Clin. Nutr., 2012, 66, 1039–1043 CrossRef CAS PubMed.
- A. M. Tindall, E. A. Johnston, P. M. Kris-Etherton and K. S. Petersen, The effect of nuts on markers of glycemic control: a systematic review and meta-analysis of randomized controlled trials, Am. J. Clin. Nutr., 2019, 109, 297–314 CrossRef PubMed.
- E. P. Neale, V. Guan, L. C. Tapsell and Y. C. Probst, Effect of walnut consumption on markers of blood glucose control: a systematic review and meta-analysis, Br. J. Nutr., 2020, 124, 641–653 CrossRef CAS PubMed.
- S. H. Schirmer, C. M. Werner, S. B. Binder, M. E. Faas, F. Custodis, M. Böhm and U. Laufs, Effects of omega-3 fatty acids on postprandial triglycerides and monocyte activation, Atherosclerosis, 2012, 225, 166–172 CrossRef CAS PubMed.
- A. Rees, G. F. Dodd and J. P. E. Spencer, The Effects of Flavonoids on Cardiovascular Health: A Review of Human Intervention Trials and Implications for Cerebrovascular Function, Nutrients, 2018, 10, 1852 CrossRef PubMed.
- A. Pipingas, J. M. Reddan, S. Gauci, L. M. Young, G. Kennedy, R. Rowsell, R. King, S. Spiteri, A. M. Minihane and A. Scholey, Post-Prandial Cognitive and Blood Pressure Effects of a DHA-Rich Omega-3 Powder in Middle-Aged Males: A Pilot Study, Nutrients, 2023, 15, 2198 CrossRef CAS PubMed.
- J. Muralidharan, S. Galiè, P. Hernández-Alonso, M. Bulló and J. Salas-Salvadó, Plant-based fat, dietary patterns rich in vegetable fat and gut microbiota modulation, Front. Nutr., 2019, 6, 157 CrossRef PubMed.
- C. Bamberger, A. Rossmeier, K. Lechner, L. Wu, E. Waldmann, S. Fischer, R. G. Stark, J. Altenhofer, K. Henze and K. G. Parhofer, A walnut-enriched diet affects gut microbiome in healthy caucasian subjects: a randomized, controlled trial, Nutrients, 2018, 10, 244 CrossRef PubMed.
- H. Yang and Z. Duan, The local defender and functional mediator: gut microbiome, Digestion, 2018, 97, 137–145 CAS.
- M. J. Patan, D. O. Kennedy, C. Husberg, S. O. Hustvedt, P. C. Calder, J. Khan, J. Forster and P. A. Jackson, Supplementation with oil rich in eicosapentaenoic acid, but not in docosahexaenoic acid, improves global cognitive function in healthy, young adults: results from randomized controlled trials, Am. J. Clin. Nutr., 2021, 114, 914–924 CrossRef CAS PubMed.
|
This journal is © The Royal Society of Chemistry 2025 |
Click here to see how this site uses Cookies. View our privacy policy here.