DOI:
10.1039/D4FO05556J
(Paper)
Food Funct., 2025, Advance Article
Human milk peptide MAMP-1 alleviates necrotizing enterocolitis via inhibition of the TLR4-mediated PI3K-AKT-NF-κB signaling pathway†
Received
12th November 2024
, Accepted 16th January 2025
First published on 7th February 2025
Abstract
Background: Necrotizing enterocolitis (NEC) is a disease with prevalent and serious intestinal inflammation that poses a significant threat to the lives of newborns. Human milk has been shown to prevent and treat the occurrence of NEC; however, the underlying mechanisms remain unclear. MAMP-1 is a significantly overexpressed endogenous peptide derived from β-casein extracted from the human milk of premature mothers, which is resistant to gastrointestinal conditions and exhibits favorable physicochemical properties. This study aims to investigate the mechanism by which the human milk-derived peptide MAMP-1 mitigates NEC in mice, offering new insights for clinical treatment. Methods: The C57BL/6 mice were categorized into three distinct groups randomly on the 7th day after birth, with 40 mice in each group. The NEC model was established using “artificial feeding + hypoxia + cold”, and the MAMP-1 group received daily MAMP-1 gavage during NEC modeling. Ileum tissues and feces were collected. Pathological damage in the intestines was evaluated by H&E staining. ZO-1 expression was analyzed through immunofluorescence staining and western blot. Apoptosis in the intestine was assessed using western blot and TUNEL staining. The effects of signaling pathways were confirmed through western blot and RNA sequencing. The expression of inflammatory factors was assessed using RT-PCR. 16S high-throughput sequencing was used to determine the diversity and abundance of the gut microbiota. Results: MAMP-1 reduced the mortality rate of NEC mice, alleviated ileum injury, increased the ZO-1 expression of the intestinal barrier, reduced the apoptotic protein expression, lowered the TUNEL positive area, increased anti-apoptotic protein expression, and reduced the levels of TLR4, P-PI3K, P-AKT, and NF-κB, leading to a reduction in the release of inflammatory cytokines. Furthermore, MAMP-1 decreased the abundance of harmful bacteria and increased the abundance of beneficial bacteria at both the phylum and genus levels. Conclusion: MAMP-1 might inhibit the TLR4-PI3K-AKT-NF-κB signaling pathways, further reducing inflammation factor release, and might decrease intestinal cell apoptosis. Results indicated that MAMP-1 might alleviate intestinal damage in NEC mice. Meanwhile, MAMP-1 might positively modulate the composition of the microbiota of NEC mice and further achieve the preventive and therapeutic effect of MAMP-1 on NEC.
Introduction
Necrotizing enterocolitis (NEC) is a disease with severe intestinal inflammation that poses a significant threat to the lives of newborns, with a mortality rate of up to 50% among patients requiring surgery.1 Multiple risk factors, including prematurity, enteral feeding in newborns, immature immune system development, and alterations of the gut microbiota, can eventuate the onset of NEC.2,3 Currently, the primary treatment approaches for NEC include antibiotic therapy, symptomatic support, and surgical treatment.4,5 However, these treatment methods are associated with a poor prognosis for NEC, resulting in malnutrition and long-term neurodevelopmental disorders in newborns.6
Intestinal injury in NEC is related to inflammation and apoptosis. TLR4 (Toll-like receptor 4) is activated by lipopolysaccharides from Gram-negative bacteria, increasing the release of inflammatory cytokines that make intestinal wall thinner and lead to increased intestinal permeability and disrupt intestinal tight junction, thereby accelerating intestinal cell apoptosis.7 Apoptotic intestinal cells in NEC may exacerbate the disruption of intestinal barrier function, allowing more microorganisms to enter the submucosal area and trigger more severe inflammatory responses.8 The imbalance between intestinal injuries and repair further forms a vicious cycle of inflammation and apoptosis and finally causes intestinal mucosal necrosis.
Related studies have shown that breastfeeding could effectively reduce the incidence of neonatal NEC and the risk of mortality associated with diseases such as sepsis and pneumonia.9,10 Compared to formula feeding, breastfeeding is more effective in protecting newborns from NEC, promoting intestinal immunity, improving intestinal lesions, repairing intestinal barriers, decreasing intestinal cell apoptosis, and reducing the occurrence of intestinal inflammation.11 Human milk contains numerous bioactive components (including human milk oligosaccharides, lactoferrin, β-casein, and immunoglobulin) that not only nourish babies but also protect them from various diseases.12 β-Casein exhibits a high expression of free peptides in the breast milk of premature mothers, which is very important for the development of intestinal health in premature infants.13 Therefore, exploring endogenous peptides derived from β-casein that exhibit protective effects on the intestine could provide new insights into the prevention and cure of NEC.
In this study, we found a significantly overexpressed endogenous peptide derived from β-casein isolated from human milk of premature mothers, which was named MAMP-1. MAMP-1 was analyzed using the ExPASy ProtParam online (https://web.expasy.org/protparam/), which revealed that the molecular weight of MAMP-1 is 1471.2 Da, with a half-life of 100.0 h, a lipid solubility index of 125.0, and a hydrophilicity index of 0.44 (Fig. 1A). Using mass spectrometry, the content of this peptide in human milk from premature mothers was found to be 2.74 times higher than that in the milk of normal mothers (Fig. 1B). Analysis using Spiral Wheel software (https://rzlab.ucr.edu/Scripts/wheel/wheel.cgi) indicated that MAMP-1 exhibits hydrophilicity (Fig. 1C). Analysis through UniProt (https://www.uniprot.org/) and the SWISS-MODEL model server (https://swissmodel.expasy.org/interactive) shows that MAMP-1 is derived from β-casein and consists of 14 amino acids, located at positions 213–226 (VTQPLAPVHNPISV) (Fig. 1D). Given the physicochemical properties and tolerance to the gastrointestinal environment of MAMP-1, we inferred that MAMP-1 may have a protective effect on the intestine, potentially aiding in the prevention and cure of NEC. To investigate the role of MAMP-1 in preventing and treating NEC in mice, we conducted relevant experiments.
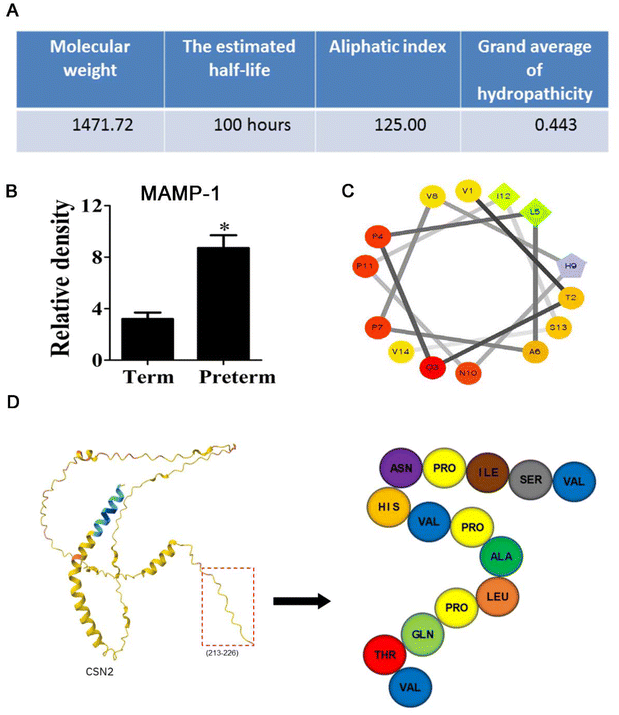 |
| Fig. 1 Basic characteristics of MAMP-1. (A–C) Physicochemical properties and structure of MAMP-1. (D) Prediction construction of MAMP-1. | |
Materials and methods
MAMP-1 synthesis
The process begins with the preparation of Wang resin, which is soaked in dichloromethane (DCM) and then washed and dried with N,N-dimethylformamide (DMF). The C-terminal first amino acid, valine (Val), is coupled to the resin using 1-hydroxy-benzotriazole (HOBt), N,N-diisopropylcarbodiimide (DIC), and 4-dimethylaminopyridine (DMAP) at 30 °C. After capping with acetic anhydride, the resin is washed and dried. The Fmoc protecting group is removed using 20% piperidine in DMF, followed by washing and drying. The ninhydrin test is used to confirm successful deprotection. Subsequent amino acids are coupled in a similar manner, with each coupling step followed by a ninhydrin test to ensure complete reaction. After all amino acids are coupled, the Fmoc group of the terminal glycine (Gly) is removed, and the resin is dried with methanol. The peptide is cleaved from the resin using a 95% trifluoroacetic acid-based cleavage solution, followed by precipitation with cold ether. The crude peptide is purified by high-performance liquid chromatography (HPLC) and then lyophilized to yield a peptide of a certain purity (GRKKRRQRRRPPQQVTQPLAPVHNPISV).
Mice for NEC modeling and drug intervention
All animal procedures were conducted in accordance with the Guidelines for Care and Use of Laboratory Animals of Guangdong Medical University and were approved by the Animal Ethics Committee of Guangdong Medical University. The ethical permission number is GDY2302208. As described in the previous methods,14 7 days after the birth of the C57BL/6 mice, they were separated from the mother mice, and artificial feeding was started. A mixture of baby milk powder (Abbott Similac) and puppy milk powder (PetAg Esbilac) in a ratio of 2
:
1 (3 g of baby milk powder and 1.5 g of puppy milk powder were dissolved in 10 mL of distilled water) was orally fed four times per day, and then the mice were subjected to hypoxia and hypothermia (95%N2, 5%O2 for 10 min and 4 °C for 10 min twice a day) for 4 days to induce NEC. Meanwhile, MAMP-1 was administered through gavage at 100 μg kg−1 for NEC modeling (Fig. 2A). Therefore, mice were divided into three groups (the Control group, the NEC group, and the MAMP-1 group). Untreated mice of same age that lived with their mothers were in the Control group. After 4 days, mice were dissected and intestinal tissues were obtained for further experiments.
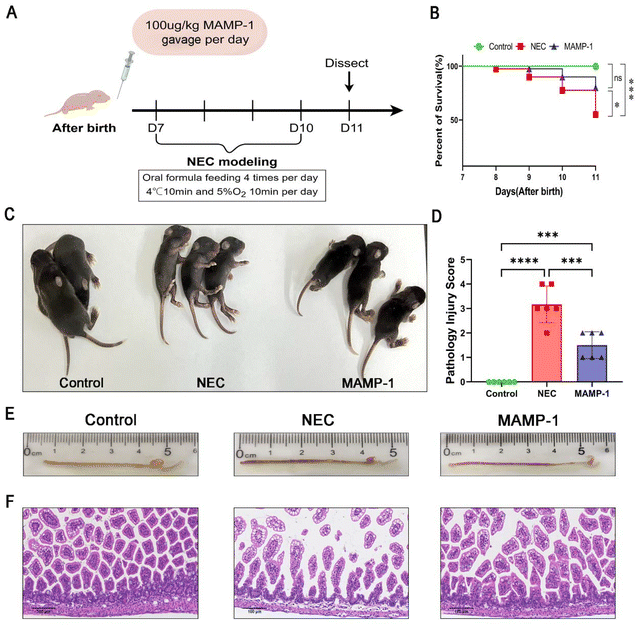 |
| Fig. 2 General situation of three groups of mice and the effect of MAMP-1 on the gross and pathological changes of the ileum in NEC-induced mice. (A) NEC modeling and MAMP-1 administration process. (B) The percent of survival in each group. n = 40 in each group. (C) The general situation of three groups of mice. Comparison of physique, activity, and defecation among the three groups of mice. (D) Comparison of pathological scores among three groups of mice. (E) The macroscopic situation of the intestinal tract in three groups of mice. In the Control group, the appearance of the intestine was transparent. In the NEC group, severe intestinal swelling is accompanied by bleeding. In the MAMP-1 group, mild swelling of the intestine occurs. (F) Characteristics of intestinal pathological staining in three groups of mice. Complete intestinal structure in the Control group. In the NEC group, thinning of the intestinal wall, separation of the lamina propria and mucosal layer, edema and shedding of ileum epithelial cells. In the MAMP-1 group, slight separation of intestinal wall lamina propria and mucosal layer. n = 6 in each group. Data are expressed as mean ± SD, magnification × 200. *: P < 0.05, ***: P < 0.005, and ****: P < 0.001. | |
Tissue collection and injury evaluation
We evaluated the intestines grossly and a 1–2 cm segment of intestinal tissue was extracted from the distal ileum. This tissue was then immersed in a 4% PFA solution for 72 hours. Following this process, the tissues underwent dehydration and were subsequently embedded in paraffin. The samples were sectioned into 4 μm slices. Then the sections were stained with Hematoxylin and Eosin, which facilitated the assessment of pathological injury scores by two independent, blinded pathologists with an established pathological scoring criterion (6 mice per group). Tissues with histologic scores ≥ 2 were considered as exhibiting NEC.15
Immunofluorescence
The intestinal paraffin sections of the three groups (4 mice per group) were deparaffinized with xylene × 2 for 10 min, anhydrous ethanol × 2 for 5 min, 90% ethanol for 5 min, and 80% ethanol for 5 min and then subjected to treatment with EDTA antigen repair buffer (pH = 8.0) for 10 min in a microwave oven. Following a wash with phosphate-buffered saline (PBS), endogenous peroxidases were eliminated using a 3% hydrogen peroxide solution. The slices were blocked with 5% bovine serum albumin for 30 minutes to inhibit nonspecific binding. The slices were subsequently incubated with primary anti-ZO-1 (1
:
50, Invitrogen, USA) at 4 °C overnight. The slices were washed with PBS and then incubated with a Goat anti-Rabbit IgG Highly Cross-Adsorbed Secondary Antibody (1
:
200, Alexa Fluor 488, Invitrogen, USA) at room temperature for a duration of 30 minutes. The entire process needs to be kept away from light. Finally, DAPI (Beyotime, China) was used to cover the slices. Images were observed under a fluorescence microscope after sealing.
Western blot
Freshly extracted intestinal tissues (4 mice per group) were proportionally treated with RIPA lysis buffer (Beyotime, China), with a protease inhibitor and a phosphatase inhibitor. Then tissues were ground using a tissue homogenizer to obtain homogenates and kept on ice for 30 min. The mixture was centrifuged for 15 minutes and the supernatant was collected. The protein concentrations were ascertained using a Pierce BCA Protein Assay Kit (Beyotime, China). The supernatant was then amalgamated with sodium dodecyl sulfate sample buffer (EpiZyme, China) in a 4
:
1 ratio and denatured at 100 °C for 10 minutes. Mixtures were separated using 7.5% and 12.5% polyacrylamide gels (EpiZyme, China), transferred to 0.45 μm/0.22 μm PVDF membranes (Millipore, USA), and detected using primary anti-β-actin (1
:
10
000, Abclonal, China), anti-TLR4 (1
:
1000, Santa, US), anti-NF-κB, anti-Bax, anti-Bcl2, anti-Cleaved-Caspase3, anti-PPI3K, anti-PAKT (1
:
1000, CST, US), and anti-ZO-1 (1
:
500, Invitrogen, USA) at 4 °C overnight. Membranes were incubated with the corresponding secondary antibody (1
:
4000, Abclonal, China) at room temperature for 60 min. Signals were detected using chemiluminescence (ECL, Mce, USA). The Image J analysis system was employed to quantify the relative intensity of target bands.
mRNA expression analysis
RNA was extracted from the terminal ileum (6 mice per group) using a SteadyPure Universal RNA Extraction kit (AG, China). The RNA quality was detected by a nanodrop spectrophotometer. The quantified RNA was reversed into cDNA using Evo M-MLVRT Mix Kit (AG, China). And the cDNAs were used for RT-PCR assay by SYBR Green Premix Pro Taq HS qPCR Kit (AG, China). The relative expression of IL-1β, IL-6, and TNF-α was normalized to β-actin. Data were calculated using the ΔΔCt method. The sequence of primers of RT-PCR is shown in Table S1.†
mRNA sequencing analysis
mRNA was extracted following the above step and RNA sequencing was performed. The libraries were sequenced on an Illumina Novaseq 6000 platform and 150 bp paired-end reads were generated. Raw reads for the three groups (6 mice per group) of fastq format were firstly processed using fastp and the low-quality reads were removed to obtain the clean reads. Then each sample was retained for subsequent analyses. The clean reads were mapped to the reference genome using HISAT2. FPKM of each gene was calculated and the read counts of each gene were obtained by HTSeq-count. Gene Set Enrichment Analysis (GSEA) was performed using GSEA software. The analysis used a predefined gene set, and the genes were ranked according to the degree of differential expression in the two types of samples. Then it is tested whether the predefined gene set was enriched at the top or bottom of the ranking list. The raw sequence data have been submitted to the NCBI short Read Archive (SRA) with accession number (BioProjectID: PRJNA1199205, https://www.ncbi.nlm.nih.gov/bioproject/PRJNA1199205).
Fecal sample collection and microbiota analysis
Feces were collected from the rectum after dissecting. The CTAB method was used to extract total DNA from microbial samples of feces among the three groups (6 mice per group) and the quality of extracted DNA was detected by agarose gel electrophoresis, while the DNA was quantified using a ultraviolet spectrophotometer. PCR amplification was carried out with the V3–V4 amplification fragment. The PCR product was purified by AMPure XT beads (Beckman Coulter Genomics, Danvers, MA, USA), quantified by Qubit (Invitrogen, USA) and confirmed by 2% agarose gel electrophoresis. The purified PCR products were evaluated using an Agilent 2100 Bioanalyzer (Agilent, USA) and an Illumina Library Quantification Kit (Kapa Biosciences, Woburn, MA, USA) and sequenced using a NovaSeq6000 sequencer. The resulting raw sequence files were submitted to the NCBI Sequence Read Archive (SRA) database (BioProjectID: PRJNA1199133, https://submit.ncbi.nlm.nih.gov/subs/sra/SUB14934388). Data were subsequently subjected to bioinformatics analysis. The primer sequence was removed and the base sequence was balanced from RawData, each pair of paired-end reads was concatenated into a longer tag based on the overlap region, and a window-based quality scan was performed on the sequencing reads. The default scanning window is 100 bp. When the average quality value within the window is below 20, the portion of the read from the beginning of the window to the end of 3′ was cut off, sequences with a length less than 100 bp after truncation were removed, sequences with a content of N (uncertain fuzzy base) of more than 5% after truncation were removed, and chimeric sequences were removed. ASV (feature) sequences and ASV (feature) abundance tables were obtained. α-diversity analysis and β-diversity analysis were performed based on the obtained ASV (feature) sequence and ASV (feature) abundance tables and according to the ASV (feature) abundance table, the abundance of each species in each sample is statistically analyzed. Based on the statistical information of the obtained species abundance, the differences at the phylum and genus levels were analyzed between each comparison group.
Results
MAMP-1 improved the general condition and reduced the mortality rate of mice with NEC
We initially investigated whether the oral administration of MAMP-1 could improve the general situation of NEC mice. We established a model of 4-day mice with NEC with simultaneous administration of MAMP-1. The survival rate statistics for each group (Fig. 2B) clearly showed differences between these three groups: 100% (40/40) in the Control group, 55.0% (22/40) in the NEC group, and 80% (32/40) in the MAMP-1 group. In our experiment, compared with the Control group, mice in the NEC group were thinner, less active, insensitive to tail stimulation, and exhibited urinary retention and difficulty in defecation (Fig. 2C). Although mice in the MAMP-1 group exhibited urinary retention, they showed better mobility and were more sensitive to tail stimulation than those in the NEC group (Fig. 2C). Overall, these findings indicated that MAMP-1 could improve the general condition of NEC mice. We next explored the effect of MAMP-1 on the intestine of NEC mice.
Effect of MAMP-1 on the gross and histopathological morphology of the intestine of NEC mice
MAMP-1 has been shown to improve the general condition and reduce the mortality rate of NEC mice. After modeling, the terminal ilea were harvested. We observed that no apparent lesions were present in the Control group (Fig. 2E). In contrast, intestines in the NEC group exhibited decreased elasticity, accompanied by swelling, bleeding, and continuous bead-like vacuoles (Fig. 2E). Only slight swelling and pneumatosis were observed in the MAMP-1 group (Fig. 2E). Subsequent observations under an optical microscope using H&E staining showed intact villi and no oedema or shedding of ileum epithelial cells of a complete intestine in the Control group (Fig. 2F). The intestinal structure of NEC mice was flimsy with separation between the lamina propria and the mucosal layer and exhibited edema of the intestinal villi along with shedding of epithelial cells (Fig. 2F). However, the intestinal wall in the MAMP-1 group was thicker than that in mice in the NEC group, and both epithelial cell edema and shedding were reduced (Fig. 2F). The histopathological scores indicated statistical differences among the three groups, with the MAMP-1 group showing significantly lower scores than the NEC group (Fig. 2D). These findings indicate that MAMP-1 could reduce the degree of intestinal edema and shedding of ileum epithelial cells, thereby improving intestinal injury. To further investigate the effect of MAMP-1 on the intestine in NEC mice, we next explored the mechanisms underlying the protective effects of MAMP-1 on intestinal injury.
MAMP-1 promoted tight junction proteins in the intestines of NEC mice and improved intestinal barrier function
Researchers have found that one of the most important features of NEC is intestinal barrier injury. We accordingly investigated the effect of MAMP-1 on intestinal tight junction proteins in NEC mice. The expression of ZO-1 which is included in tight junction (TJ) proteins was observed in each group using immunofluorescence staining (IF) under an optical microscope. Compared with other groups, the NEC group showed significantly lower expression levels of ZO-1 (Fig. 3A and B), and western blot analysis suggested that the ZO-1 expression was reduced in the NEC group (Fig. 3C and D). In contrast, ZO-1 expression was increased in the MAMP-1 group (Fig. 3C and D). These results revealed that MAMP-1 could repair the damage to intestinal barrier function caused by NEC in mice. TJ proteins were known to regulate intestinal mucosal apoptosis, therefore, we further explored the effect of MAMP-1 on apoptosis in NEC mice.
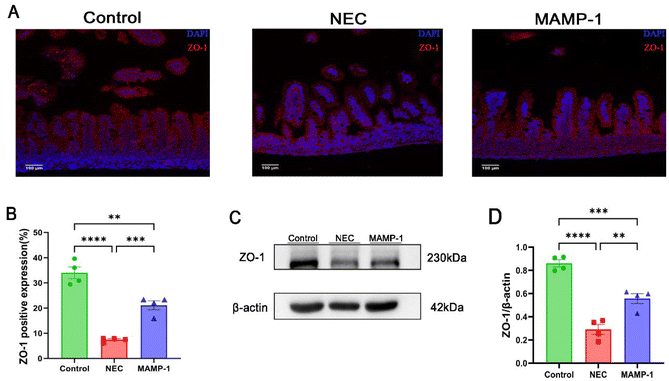 |
| Fig. 3 Effect of MAMP-1 on the tight junction protein (ZO-1) in the intestine of NEC-induced mice. Red fluorescence indicates a ZO-1 positive expression area in the intestine, while blue fluorescence indicates nuclear staining. (A) ZO-1 was assessed in the ileum tissue of the three groups by immunofluorescence staining. (B) The expressions of ZO-1 by immunofluorescence staining in each group. (C and D) Protein expression of ZO-1 was proved by western blot analysis among the three groups. n = 4 in each group. Data are expressed as mean ± SD, magnification × 200. *: P < 0.05, **: P < 0.01, ***: P < 0.005, and ****: P < 0.001. | |
MAMP-1 inhibited ileum mucosal cell apoptosis in NEC mice
The intestinal injury associated with NEC is closely related to cellular apoptosis. To investigate the effect of MAMP-1 on intestinal epithelial cell apoptosis in mice with NEC, we employed TUNEL staining and western blot analysis to detect apoptosis in the intestinal cells. The results of TUNEL staining (488 nm fluorescence) under a fluorescence microscope showed that the positive area expression in NEC mice was significantly increased compared with other groups (Fig. 4A and B). Western blot analysis showed that in the NEC group, the expression levels of Bax and Cleaved-Caspase3 were increased (Fig. 4C and D), while the expression of Bcl2 was decreased (Fig. 4C and E). In contrast, the expression levels of Bax and Cleaved Caspase 3 were decreased (Fig. 4C and E), while Bcl2 expression increased in the MAMP-1 group (Fig. 4C and E). These results indicate that the apoptosis of intestinal tissue in the NEC group was significantly greater than in mice in the MAMP-1 group. In summary, we conclude that MAMP-1 could inhibit ileum mucosal cell apoptosis, thereby repairing the injured gut barrier in NEC mice. However, these results are preliminary and have not been thoroughly studied. We next employed RNA sequencing and additional experiments to explore the mechanism of MAMP-1 in NEC mice.
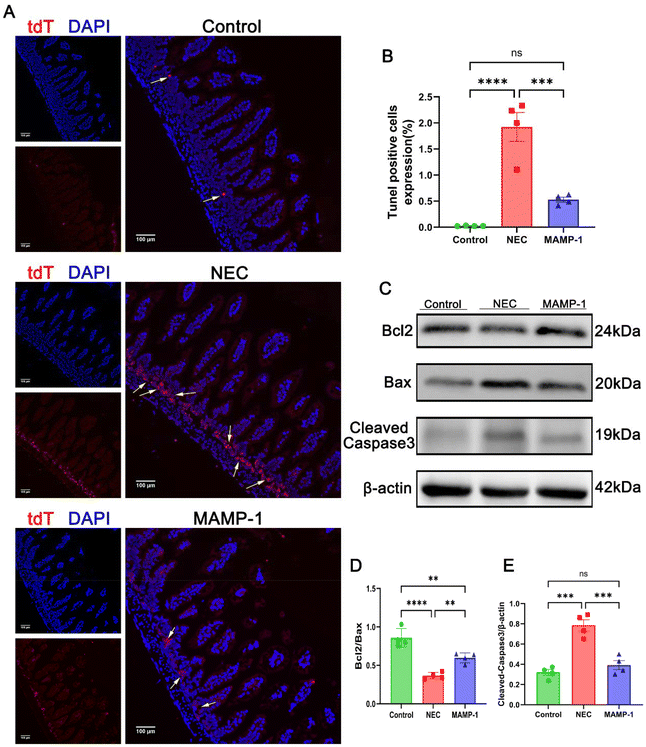 |
| Fig. 4 Effect of MAMP-1 on apoptosis in the intestine in NEC-induced mice. Red dot fluorescence represents TUNEL positive expression area, and blue fluorescence represents nuclear staining. (A) Images of TUNEL (Flour488) staining among the three groups. (B) The expressions of TUNEL staining in each group. (C–E) Protein expressions of Bax, Bcl2 and Cleaved-Caspase 3 were assessed by western blot analysis and were analyzed in intensity. n = 4 in each group. Data are expressed as mean ± SD, magnification × 200. *: P < 0.05, **: P < 0.01, and ****: P < 0.001. | |
MAMP-1 inhibited the activation of the TLR4/NF-κB pathway in NEC mice and reduced the release of inflammatory cytokines
Previous studies have demonstrated the significant role of toll-like receptor 4 (TLR4) in immunity. The intestine is stimulated by pathogens, during which TLR4 is highly expressed and activates intestinal immunity. The nuclear factor kappa B (NF-κB) signaling pathway is activated, and NF-κB acts as a transcription factor that releases inflammatory factors, coordinating the development of inflammation.16 To determine the effect of MAMP-1 on the TLR4/NF-κB signaling pathway in NEC mice, the protein expressions of TLR4 and NF-κB were assessed by western blot analysis, while mRNA expression of inflammatory factors (IL-6, IL-1β, TNF-α) was detected by RT-PCR. Western blot analysis showed that the expression of TLR4 and NF-κB increased in the NEC group (Fig. 5E, F and H). However, TLR4 and NF-κB expression levels were significantly decreased in the MAMP-1 group (Fig. 5E, F and H). RT-PCR results indicated that compared to the Control group, NEC caused a dramatic increase in mRNA expression levels of IL-6, IL-1β, and TNF–α (Fig. 5J). Conversely, MAMP-1 treatment significantly reversed this tendency (Fig. 5J). These results suggested that MAMP-1 could lower TLR4 and NF-κB expression, inhibiting the TLR4/NF-κB signaling pathway, subsequently leading to a reduction in the release of inflammatory factors and ultimately improving the intestine damage in NEC mice.
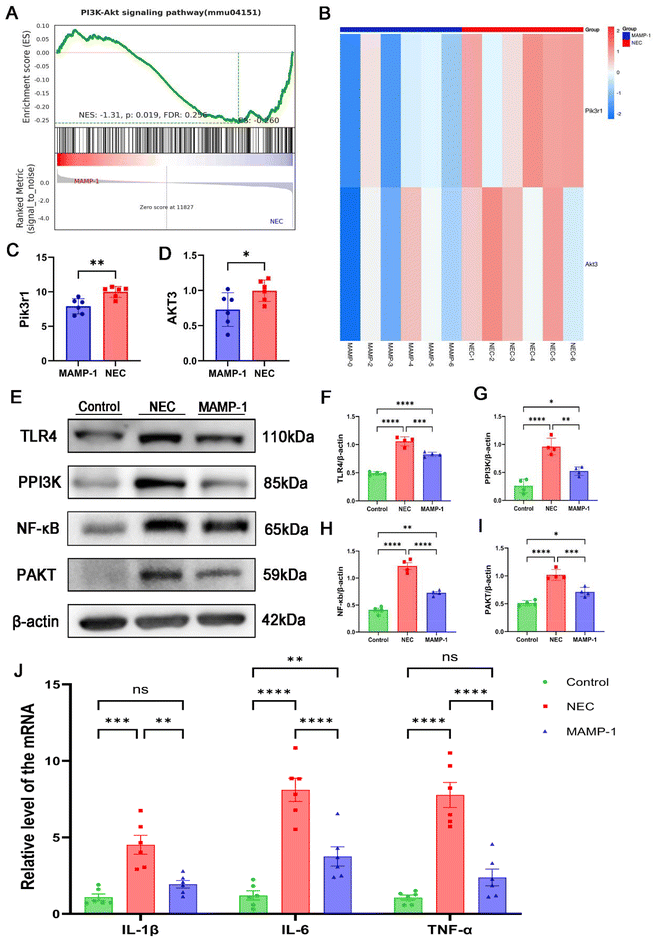 |
| Fig. 5 Effects of MAMP-1 on the TLR4/NF-κB signaling pathway and the TLR4/PI3K/AKT/NF-κB signaling pathway and on the inflammatory cytokines in NEC-induced mice. (A) PI3K/AKT signaling pathway through GSEA between the NEC group and the MAMP-1 group. n = 6 in each group. (B) Heatmap of expression of Pik3r1 and Akt3 between the NEC group and the MAMP-1 group. (C and D) Bar chart analysis of expression of Pik3r1 and Akt3. (E–I) Protein expressions of TLR4, P-PI3K, P-AKT, and NF-κB in the three groups were assessed by western blot analysis and were analyzed for intensity. n = 4 in each group. (J) The mRNA expression of IL-1β, IL-6, and TNF-α. n = 6 in each group. Data are expressed as mean ± SD *: P < 0.05, **: P < 0.01, ***: P < 0.005, and ****: P < 0.001. | |
MAMP-1 inhibited TLR4-mediated PI3K/AKT signaling pathway activation in NEC mice to reduce NF-κB expression
We verified the TLR4/NF-κB classical pathways of the effect of MAMP-1 in NEC mice. Gene GSEA of the RNA sequencing showed that, compared to the NEC group, genes in the PI3K/AKT pathway were downregulated in the MAMP-1 group (Fig. 5A). Notably, Pik3r1 and Akt3 were significantly downregulated in the MAMP-1 group (Fig. 5B and C). Previous research has demonstrated that the PI3K/AKT signaling pathway plays a significant role in the inflammatory response. Researchers have found a link between TLR4 and PI3K/AKT activation; moreover, the PI3K/AKT signaling pathway exhibits a significant correlation with NF-κB.17 Therefore, to determine whether PI3K/AKT signaling pathway activation was inhibited by MAMP-1 treatment, we conducted an enrichment analysis of GSEA in the PI3K/AKT pathway and assessed the expression of TLR4, P-PI3K, P-AKT, and NF-κB by western blot analysis. The results showed that the expression levels of TLR4, P-PI3K, P-AKT, and NF-κB were increased in the NEC group (Fig. 5F–H). However, in the MAMP-1 group, the expression levels of TLR4, P-PI3K, P-AKT, and NF-κB were significantly lower than in the NEC group (Fig. 5F–H). These results indicated that MAMP-1 reduced NF-κB expression by inhibiting TLR4-mediated PI3K/AKT signaling pathway activation in NEC mice. Overall, we elucidated the mechanism by which MAMP-1 acts on NEC mice, specifically the TLR4-mediated activation of the PI3K/AKT signaling pathway. Additionally, we gathered fecal samples from the mice to analyze the gut microbial community, as the disruption of the gut microbiota is also a risk factor for NEC.
MAMP-1 participates in the colonization of the gut microbiota in NEC mice
We used 16s rRNA sequencing to investigate the effect of MAMP-1 on the gut microbiota in NEC mice. The α-diversity including Shannon, Chao1, and Simpson indices did not exhibit any significant differences between the NEC and MAMP-1 groups (Fig. 6A–C), indicating that MAMP-1 did not affect the overall community diversity and bacterial richness in NEC mice. PCoA analysis revealed an overlap between the NEC and MAMP-1 groups. However, both groups differed from the Control group in ASV clusters (Fig. 6D), indicating that MAMP-1 may affect the abundance of certain microbial communities within the gut microbiota in NEC mice. The bar chart of relative abundance at the phylum level showed that the main compositions of the gut microbiota in neonatal mice were Proteobacteria, Firmicutes, and Bacteroidetes (Fig. 6E). Similarly, compared to the Control and MAMP-1 group, the relative abundance of Proteobacteria increased, whereas the relative abundances of both Firmicutes and Bacteroidetes decreased (Fig. 6F). Furthermore, at the genus level, the relative abundance of Citrobacter and Acinetobacter increased, while the relative abundance of Ligilactobacillus, Streptococcus, and Rodentibacter decreased in the NEC group (Fig. 6G and H). After MAMP-1 intervention, the relative abundance of Citrobacter decreased, while the relative abundance of Ligilactobacillus, Streptococcus, and Rodentibacter also decreased (Fig. 6G and H). These results indicate that MAMP-1 may be involved in the colonization of the gut microbiota in mice of NEC, reducing the abundance of pathogenic bacteria while increasing that of probiotics to maintain intestinal homeostasis.
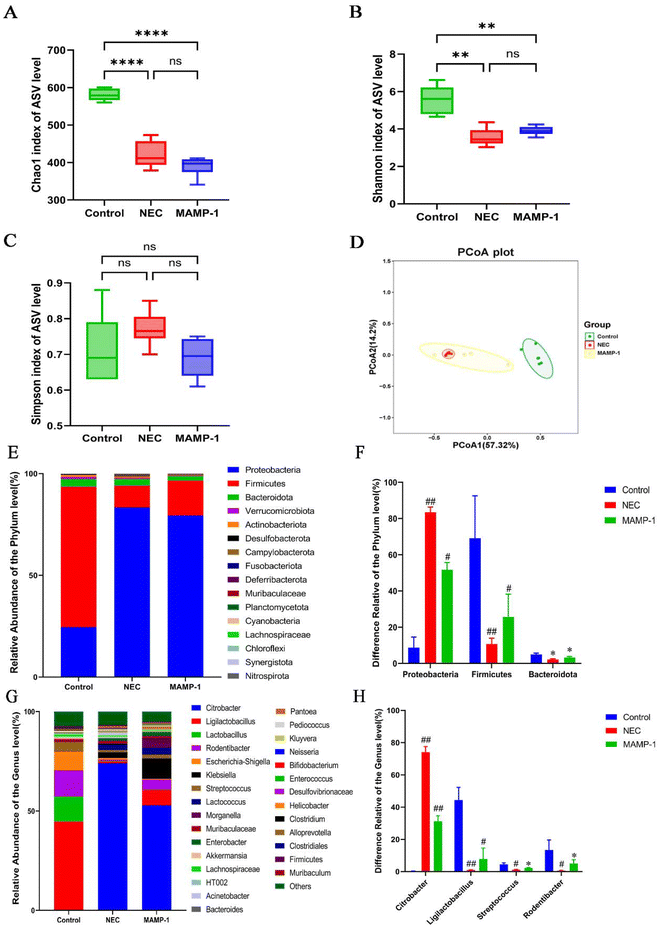 |
| Fig. 6 Effect of MAMP-1 on the diversity, composition and differential expression of the gut microbiotas at the phylum and genus levels in NEC-induced mice. (A–C) The α-diversity of the gut microbiota was determined using Chao, Shannon and Simpson indices. (D) Unweighted UniFrac-based principal coordinate analysis (PCoA) based on the ASV levels. (E) Bar chart of relative abundance at the phylum level in the three groups of gut microbiotas. (F) Difference relative at the Phylum level in the three groups of gut microbiotas. (G) Bar chart of relative abundance at the genus level in the three groups of gut microbiotas. (H) Difference relative at the genus level in the three groups of gut microbiotas. n = 6. Data are expressed as mean ± SD. *: P < 0.05. #: P < 0.01. and ##: P < 0.001. Data are expressed as mean ± SD. ***: P < 0.005 and ****: P < 0.001. | |
Discussion
Necrotizing enterocolitis (NEC) is one of the main causes of death in newborns in clinical practice, with complex course, severe condition, and poor prognosis. Studies have confirmed that feeding human milk could effectively reduce the incidence of NEC, and some neonates with NEC through feeding with human milk could alleviate the severity of NEC.18,19 However, due to the complex composition of human milk, newborns cannot sufficiently and efficiently obtain active ingredients in human milk. Therefore, it is particularly important to find components in human milk that fulfill a role in preventing and treating NEC.
Researchers have identified β-casein as an active ingredient in human milk that participates in immune responses in newborns, regulates the gastrointestinal microbiota environment, and has a protective effect on the intestines.20 Our group had identified a series of bioactive peptides sourced from human milk. These peptides were associated with various functions, including the regulation of energy metabolism, the promotion of fat function, and the modulation of immune balance.21,22 Different amino acid sequences had different activities;23 therefore, we concentrated on a sequence that comprised unique, milk-derived peptides, specifically MAMP-1. In this study, MAMP-1 is a significantly overexpressed endogenous peptide extracted from the human milk of premature mothers, with good physicochemical properties that can tolerate the gastrointestinal environment. This article identifies that MAMP-1 has significant effects in alleviating intestinal inflammation, inhibiting intestinal cell apoptosis, repairing gut barrier damage, and participating in the modulation of the gut microbiota in NEC mice.
The intestine is the biggest immune organ in humans and other animals, which can isolate harmful factors brought by the environment to the body with effect. The intestinal barrier is regulated by tight junction proteins (TJs). ZO-1 is a key protein of TJs that can effectively regulate intestinal permeability.24 Intestinal barrier damage is one of the pathogenic factors of NEC, increasing intestinal permeability and leading to the invasion of external pathogens into the intestine and the release of inflammatory factors, which further increases intestinal permeability and exacerbates intestinal inflammation.25 Prior research has indicated that the expression of inflammatory factors is reduced in NEC newborns who are fed human milk, enhancing the ability of Paneth cells and Goblet cells to reshape the gut barrier.26 This study found that compared with the NEC group, mice in the MAMP-1 group showed significantly increased ZO-1 expression, indicating that MAMP-1 may repair the gut barrier by increasing ZO-1 expression to achieve its preventive and therapeutic effects on NEC.
As NEC progresses, there is widespread necrosis and apoptosis of intestinal epithelial cells. Apoptosis is the outcome of non-periodic cell death, causing more severe intestinal damage in NEC newborns.27 Bcl2 and Bax are key factors released by mitochondria to regulate cell apoptosis, and they antagonize each other.28 Caspase-3 is cleaved within mitochondria, assisting in the process of cell apoptosis.29 The principle of TUNEL staining is that when cells undergo apoptosis, some DNA endonucleases are activated. The 3′-OH exposed following DNA cleavage can be enzymatically catalyzed by Terminal Deoxynucleotide Transferase (TdT) to incorporate fluorescein-labeled dUTP, which can detect cell apoptosis through fluorescence microscopy.30 Related studies have shown that Bax expression increases, while Bcl2 expression decreases. TUNEL staining shows an increase in positive area expression.7,31 An active peptide derived from breast milk effectively improves NEC apoptosis by weakening the PERK-eIF2α-QRICH1 pathway.32 This study found that in comparison with the NEC group, the proportion of apoptosis of intestinal cells in the MAMP-1 group was decreased, Bax and Cleaved Caspase 3 protein expression was reduced, Bcl2 expression was increased, and TUNEL positive area expression was reduced. The findings suggest that MAMP-1 may mitigate intestinal damage by inhibiting apoptosis in intestinal cells, thereby preventing and treating NEC in mice.
Due to incomplete development of the intestines in newborns, TLR4 receptors in intestinal epithelial cells are the main target of inflammatory response.33 TLR4/NF-κB is a classic signaling pathway that activates the body's immune response. Increased intestinal permeability in NEC newborns leads to external pathogens, most of which are Gram-negative bacteria. The released LPS from Gram-negative bacteria binds to TLR4 and increases TLR4 expression, inducing nuclear translocation of NF-κB in intestinal cells and transcriptional release of amplified inflammatory factors.34,35 Breast milk polysaccharides (HMOs) have been found to inhibit the TLR4/NF-κB pathway activated, accelerate crypt cell renewal, and protect mice intestinal epithelial cells.36 In this study, after intervention with MAMP-1, the expression of TLR4 in NEC mice decreased, resulting in a decrease in NF-κB expression and a reduction in the release of proinflammatory factors. Other studies found that TLR4 activation increases expression, leading to downstream PI3K/AKT pathway activation, NF-κB nuclear translocation releasing inflammatory factors, and activation of inflammatory response.37,38 Xiangyun Yan et al. found that the peptide [Tat (48–60) YVEEL] extracted from human milk whey can inhibit the PI3K/AKT pathway activated and prevent the occurrence of NEC by suppressing the expression of TLR4 protein.39 In this study, compared with the NEC group, RNA sequencing showed that Pik3r1 and Akt3 declined in the MAMP-1 group. Pik3r1 (p85α) is a regulatory subunit of phosphoinositol 3-kinase (PI3K), and Akt3 is a member of the AKT protein kinase family; these participate in immune response and are involved in the TLR4 pathway.17,40 WB showed that the expression of TLR4 decreased, PI3K and AKT protein phosphorylation was inhibited in the MAMP-1 group, and PI3K/AKT pathway activation was inhibited, leading to a reduction in the NF-κB expression. These results indicate that MAMP-1 may alleviate gut inflammation in NEC mice by inhibiting the TLR4/NF-κB pathway and reducing proinflammatory cytokine expression. MAMP-1 may also inhibit PI3K/AKT activation by lowering TLR4 expression, resulting in reduced NF-κB expression.
The gut microbiota constitutes a crucial component external to the intestinal barrier, activating the intestinal immune response and regulating intestinal metabolism. Due to the initial stage of intestinal development in newborns, the gut microbiota is of paramount importance.41 Clinical studies have found that premature infants have physiological immaturity throughout the body, leading to disrupted colonization of the gut microbiota, disruption of intestinal homeostasis, and pathogen invasion ultimately resulting in NEC.42,43 Newborns with NEC will experience changes in their gut microbiota before onset, characterized by an elevated relative abundance of Proteobacteria and a diminished relative abundance of Firmicutes and Bacteroidetes. At the phylum level, Proteobacteria, Firmicutes, and Bacteroidetes play key roles in NEC. As the dominant Gram-negative bacterial community in the gut microbiota of premature infants, Proteobacteria stimulates the gut to activate pro-inflammatory immune responses through TLR4 signaling before NEC occurs;44 Firmicutes and Bacteroidetes are related to the maturation and support of intestinal barrier function and they can promote the production of anti-inflammatory cytokine IL-10, thereby improving intestinal inflammation.44 In NEC mice, analysis of the gut microbiota demonstrated a heightened relative abundance of Proteobacteria at the phylum level, a decrease in the relative abundance of Firmicutes and Bacteroidetes, and a 25% increase in the relative abundance of Klebsiella at the genus level.34 Further research on the microbiota has revealed that Citrobacter is a specific pathogen used to simulate human pathogenic Escherichia coli infection and inflammatory bowel disease;45 Lactobacillus is crucial for maintaining intestinal health and overall well-being and it can promote the balance of the gut microbiota and enhance the formation of tight junctions between intestinal epithelial cells;46 also, Streptococcus may interact with other bacteria through their metabolites that maintain intestinal health.47 Our study found that compared with the Control group, the gut microbiota of NEC group mice changed. At the phylum level, there was a notable increase in the abundance of Proteobacteria, while Firmicutes and Bacteroidetes exhibited a decrease. At the genus level, an increase was observed in the abundance of Citrobacter, whereas Lactobacillus, Rodentibacter, and Streptococcus demonstrated a decline. On the contrary, in the MAMP-1 group, the abundance of Proteobacteria and Citrobacter was reduced; while the abundance of Firmicutes, Bacteroidetes, Lactobacillus, Rodentibacter, and Streptococcus was increased.
Human milk feeding has been shown to provide superior regulation of modulating the composition of the microbiota of NEC mice. Kannikar Vongbhavit et al. found that breastfeeding can shape the gut microbiota of premature infants, regulate gut microbiota dysbiosis, and reduce the risk of NEC.48 In summary, MAMP-1 may be involved in the regulation of the gut microbiota in NEC mice, maintaining intestinal homeostasis, reducing pathogen invasion, and further preventing and treating NEC.
In conclusion, MAMP-1 might inhibit the TLR4-PI3K-AKT-NF-κB signaling pathways, further reducing inflammation factor release, and it might decrease intestinal cell apoptosis. Results indicated that MAMP-1 might alleviate intestinal damage in NEC mice. Meanwhile, MAMP-1 might positively modulate the composition of the microbiota of NEC mice and further achieve the preventive and therapeutic effect of MAMP-1 on NEC. The mechanism diagram, drawn with FIGDRAW, is depicted in Fig. 7. However, it just showed the effect of MAMP-1 in vivo, and further clarification of the detailed mechanism of MAMP-1 needs to be explored in cell experiments.
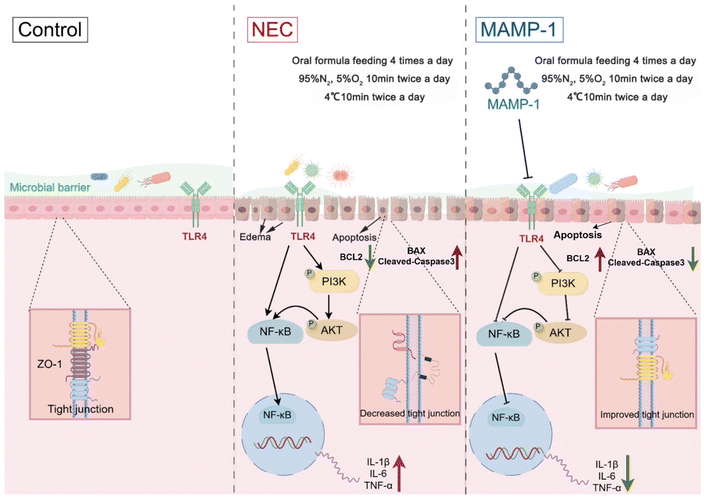 |
| Fig. 7 The mechanism of action of MAMP-1 in preventing and treating NEC. | |
Data availability
Data are available on request from the authors.
Conflicts of interest
There are no conflicts to declare.
Acknowledgements
This work was supported by the Guangdong Basic and Applied Basic Research Foundation (2022A1515140063) and the Dongguan Science and Technology of Social Development Program (20231800939962).
References
- C. Flahive, A. Schlegel and E. A. Mezoff, Necrotizing Enterocolitis: Updates on Morbidity and Mortality Outcomes, J. Pediatr., 2020, 220, 7–9 CrossRef PubMed.
- J. Duchon, M. E. Barbian and P. W. Denning, Necrotizing Enterocolitis, Clin. Perinatol., 2021, 48(2), 229–250 CrossRef PubMed.
- M. E. S. Sampah and D. J. Hackam, Dysregulated Mucosal Immunity and Associated Pathogeneses in Preterm Neonates, Front. Immunol., 2020, 11, 899 CrossRef CAS PubMed.
- J. Ou, C. M. Courtney, A. E. Steinberger, M. E. Tecos and B. W. Warner, Nutrition in Necrotizing Enterocolitis and Following Intestinal Resection, Nutrients, 2020, 12(2), 520 CrossRef CAS PubMed.
- E. Pace, T. D. Yanowitz, P. Waltz and M. J. Morowitz, Antibiotic therapy and necrotizing enterocolitis, Semin. Pediatr. Surg., 2023, 32(3), 151308 CrossRef PubMed.
- Antimicrobial Resistance Collaborators, Global burden of bacterial antimicrobial resistance in 2019: a systematic analysis, Lancet, 2022, 399(10325), 629–655 CrossRef PubMed.
- X. Wang, Y. Mi, X. Xiong and Z. Bao, The Protective Effect of Sulforaphane on ER-induced Apoptosis and Inflammation in Necrotizing Enterocolitis Mice, Comb. Chem. High Throughput Screening, 2023, 26(6), 1186–1195 CrossRef CAS PubMed.
- T. Liu, H. Zong and X. Chen, et al., Toll-like receptor 4-mediated necroptosis in the development of necrotizing enterocolitis, Pediatr. Res., 2022, 91(1), 73–82 CrossRef CAS PubMed.
- E. Moliner-Calderón, S. Verd, A. Leiva, G. Ginovart, P. Moll-McCarthy and J. Figueras-Aloy, The role of human milk feeds on inotrope use in newborn infants with sepsis, Front. Pediatr., 2023, 11, 1172799 CrossRef PubMed.
- L. R. Sorce, M. A. Q. Curley, R. Kleinpell, B. Swanson and P. P. Meier, Mother's Own Milk Feeding and Severity of Respiratory Illness in Acutely Ill Children: An Integrative Review, J. Pediatr. Nurs., 2020, 50, 5–13 CrossRef PubMed.
- L. Harris, S. Lewis and S. Vardaman, Exclusive Human Milk Diets and the Reduction of Necrotizing Enterocolitis, Adv. Neonatal. Care, 2024, 24(5), 400–407 CrossRef PubMed.
- Y. Lyu, B. J. Kim, J. S. Patel, D. C. Dallas and Y. Chen, Human Milk Protein-Derived Bioactive Peptides from In Vitro-Digested Colostrum Exert Antimicrobial Activities against Common Neonatal Pathogens, Nutrients, 2024, 16(13), 2040 CrossRef CAS PubMed.
- Z. Fan and W. Jia, Lactobacillus casei-Derived Postbiotics Elevate the Bioaccessibility of Proteins via Allosteric Regulation of Pepsin and Trypsin and Introduction of Endopeptidases, J. Agric. Food Chem., 2023, 71(28), 10647–10669 CrossRef CAS PubMed.
- C. L. Leaphart, F. Qureshi and S. Cetin, et al., Interferon-gamma inhibits intestinal restitution by preventing gap junction communication between enterocytes, Gastroenterology, 2007, 132(7), 2395–2411 CrossRef CAS PubMed.
- M. Good, R. H. Siggers and C. P. Sodhi, et al., Amniotic fluid inhibits Toll-like receptor 4 signaling in the fetal and neonatal intestinal epithelium, Proc. Natl. Acad. Sci. U. S. A., 2012, 109(28), 11330–11335 CrossRef CAS PubMed.
- C. R. Pizzarello, A. Nelson, I. Verekhman, A. E. Seppo and K. M. Järvinen, Human milk affects TLR4 activation and LPS-induced inflammatory cytokine expression in Caco-2 intestinal epithelial cells, Sci. Rep., 2024, 14, 13448 CrossRef CAS PubMed.
- Q.-Y. Zhang, M.-T. Zhong and M. Gi, et al., Inulin alleviates perfluorooctanoic acid-induced intestinal injury in mice by modulating the PI3K/AKT/mTOR signaling pathway, Environ. Pollut., 2024, 342, 123090 CrossRef CAS PubMed.
- E. Altobelli, P. M. Angeletti, A. Verrotti and R. Petrocelli, The Impact of Human Milk on Necrotizing Enterocolitis: A Systematic Review and Meta-Analysis, Nutrients, 2020, 12(5), 1322 CrossRef PubMed.
- X. Xiong, Y. Wang and X. Chen, et al., Mixed Feedings and Necrotizing Enterocolitis: The Proportion of Human Milk Matters, Breastfeed Med., 2023, 18(6), 469–474 CrossRef PubMed.
- B. Liu, W. Qiao, M. Zhang, Y. Liu, J. Zhao and L. Chen, Bovine milk with variant β-casein types on immunological mediated intestinal changes and gut health of mice, Front. Nutr., 2022, 9, 970685 CrossRef PubMed.
- Y. Li, X. Cui and X. Wang, et al. Human milk derived peptide AOPDM1 attenuates obesity by restricting adipogenic differentiation through MAPK signalling, Biochim. Biophys. Acta Gen. Subj., 2021, 1865(3), 129836 CrossRef CAS PubMed.
- D. Shen, J. F. Gao and J. Xia, et al., Liposome-encapsulated peptide PDBSN ameliorates high-fat-diet-induced obesity and improves metabolism homeostasis, Biochem. Biophys. Res. Commun., 2020, 533(1), 181–187 CrossRef CAS PubMed.
- J. Cai, X. Li, H. Du, C. Jiang, S. Xu and Y. Cao, Immunomodulatory significance of natural peptides in mammalians: Promising agents for medical application, Immunobiology, 2020, 225(3), 151936 CrossRef CAS PubMed.
- S. Seo, H. Miyake and M. Alganabi, et al., Vasoactive intestinal peptide decreases inflammation and tight junction disruption in experimental necrotizing enterocolitis, J. Pediatr. Surg., 2019, 54(12), 2520–2523 CrossRef PubMed.
- L. W. Kaminsky, R. Al-Sadi and T. Y. Ma, IL-1β and the Intestinal Epithelial Tight Junction Barrier, Front. Immunol., 2021, 12, 767456 CrossRef CAS PubMed.
- G. Noel, J. G. In and J. M. Lemme-Dumit, et al., Human Breast Milk Enhances Intestinal Mucosal Barrier Function and Innate Immunity in a Healthy Pediatric Human Enteroid Model, Front. Cell Dev. Biol., 2021, 9, 685171 CrossRef PubMed.
- Y. Yang, T. Zhang and G. Zhou, et al., Prevention of Necrotizing Enterocolitis through Milk Polar Lipids Reducing Intestinal Epithelial Apoptosis, J. Agric. Food Chem., 2020, 68(26), 7014–7023 CrossRef CAS PubMed.
- A. Z. Spitz and E. Gavathiotis, Physiological and pharmacological modulation of BAX, Trends Pharmacol. Sci., 2022, 43(3), 206–220 CrossRef CAS PubMed.
- W.-T. Kuo, L. Shen and L. Zuo, et al., Inflammation-induced Occludin Downregulation Limits Epithelial Apoptosis by Suppressing Caspase-3 Expression, Gastroenterology, 2019, 157(5), 1323–1337 CrossRef CAS PubMed.
- C. L. Moore, A. V. Savenka and A. G. Basnakian, TUNEL Assay: A Powerful Tool for Kidney Injury Evaluation, Int. J. Mol. Sci., 2021 Jn 2, 22(1), 412 Search PubMed.
- M. Akisu, B. Durmaz and O. A. Koroglu, et al., The effects of IGF-1 and erythropoietin on apoptosis and telomerase activity in necrotizing enterocolitis model, Pediatr. Res., 2021, 90(3), 559–564 CrossRef CAS PubMed.
- J. Huo, R. Zhang and X. Wu, et al., Active polypeptide MDANP protect against necrotizing enterocolitis (NEC) by regulating the PERK-eIF2α-QRICH1 axis, Sci. Rep., 2023, 13, 22912 CrossRef CAS PubMed.
- D. J. Hackam and C. P. Sodhi, Bench to bedside - new insights into the pathogenesis of necrotizing enterocolitis, Nat. Rev. Gastroenterol. Hepatol., 2022, 19(7), 468–479 CrossRef CAS PubMed.
- X. Zhang, Y. Zhang, Y. He, X. Zhu, Q. Ai and Y. Shi, β-glucan protects against necrotizing enterocolitis in mice by inhibiting intestinal inflammation, improving the gut barrier, and modulating gut microbiota, J. Transl. Med., 2023, 21(1), 14 CrossRef PubMed.
- D. Huang, P. Wang and J. Chen, et al., Selective targeting of MD2 attenuates intestinal inflammation and prevents neonatal necrotizing enterocolitis by suppressing TLR4 signaling, Front. Immunol., 2022, 13, 995791 CrossRef CAS PubMed.
- C. Wang, M. Zhang and H. Guo, et al., Human Milk Oligosaccharides Protect against Necrotizing Enterocolitis by Inhibiting Intestinal Damage via Increasing the Proliferation of Crypt Cells, Mol. Nutr. Food Res., 2019, 63(18), e1900262 CrossRef PubMed.
- Y. Zhang, Q. Liu and K. Gao, et al., Remote Ischemic Conditioning Relieves Necrotizing Enterocolitis Through Regulation of Redox and Inflammation, J. Interferon Cytokine Res., 2023, 43(5), 216–228 CrossRef CAS PubMed.
- B. Liu, X. Piao and W. Niu, et al., Kuijieyuan Decoction Improved Intestinal Barrier Injury of Ulcerative Colitis by Affecting TLR4-Dependent PI3K/AKT/NF-κB Oxidative and Inflammatory Signaling and
Gut Microbiota, Front. Pharmacol., 2020, 11, 1036 CrossRef CAS PubMed.
- X. Yan, Y. Cao and W. Chen, et al., Peptide Tat(48–60) YVEEL protects against necrotizing enterocolitis through inhibition of toll-like receptor 4-mediated signaling in a phosphatidylinositol 3-kinase/AKT dependent manner, Front. Nutr., 2022, 9, 992145 CrossRef PubMed.
- F. Ullah, D. Bhattarai and Z. Cheng, et al., Comparative Analysis of V-Akt Murine Thymoma Viral Oncogene Homolog 3 (AKT3) Gene between Cow and Buffalo Reveals Substantial Differences for Mastitis, BioMed Res. Int., 2018, 2018, 1463732 Search PubMed.
- N. K. Saeed, M. Al-Beltagi, A. S. Bediwy, Y. El-Sawaf and O. Toema, Gut microbiota in various childhood disorders: Implication and indications, World J. Gastroenterol., 2022, 28(18), 1875–1901 CrossRef CAS PubMed.
- J. Aires, Z. E. Ilhan and L. Nicolas, et al., Occurrence of Neonatal Necrotizing Enterocolitis in Premature Neonates and Gut Microbiota: A Case-Control Prospective Multicenter Study, Microorganisms, 2023, 11(10), 2457 CrossRef CAS PubMed.
- S. Zeng, J. Ying, S. Li, Y. Qu, D. Mu and S. Wang, First 1000 Days and Beyond After Birth: Gut Microbiota and Necrotizing Enterocolitis in Preterm Infants, Front. Microbiol., 2022, 13, 905380 CrossRef PubMed.
- F. Di Vincenzo, A. Del Gaudio, V. Petito, L. R. Lopetuso and F. Scaldaferri, Gut microbiota, intestinal permeability, and systemic inflammation: a narrative review, Intern. Emerg. Med., 2024, 19(2), 275–293 CrossRef PubMed.
- C. Mullineaux-Sanders, J. Sanchez-Garrido, E. G. D. Hopkins, A. R. Shenoy, R. Barry and G. Frankel, Citrobacter rodentium–host–microbiota interactions: immunity, bioenergetics and metabolism, Nat. Rev. Microbiol., 2019, 17(11), 701–715 CrossRef CAS.
- E. Dempsey and S. C. Corr, Lactobacillus spp. for Gastrointestinal Health: Current and Future Perspectives, Front. Immunol., 2022, 13, 840245 CrossRef CAS.
- V. Notarbartolo, M. Giuffrè, C. Montante, G. Corsello and M. Carta, Composition of Human Breast Milk Microbiota and Its Role in Children's Health, Pediatr. Gastroenterol., Hepatol. Nutr., 2022, 25(3), 194–210 CrossRef PubMed.
- K. Vongbhavit, L. K. Salinero and K. M. Kalanetra, et al., A comparison of bacterial colonization between nasogastric and orogastric enteral feeding tubes in infants in the neonatal intensive care unit, J. Perinatol., 2022, 42(11), 1446–1452 CrossRef CAS.
|
This journal is © The Royal Society of Chemistry 2025 |
Click here to see how this site uses Cookies. View our privacy policy here.