Bindone-based polymer for colorimetric detection of volatile amines†
Received
22nd January 2025
, Accepted 28th March 2025
First published on 4th April 2025
Abstract
Effective detection and monitoring of volatile amines are crucial for protecting human health and the environment, particularly in areas such as disease diagnosis and food spoilage detection. Traditional gas sensors, including electrochemical, semiconductor, and photochemical types, often suffer from limited selectivity, sensitivity and require complex and expensive synthesis and detection equipment. Colorimetric sensors, which are easily interpreted through visible colour changes, have recently gained attention for their simplicity and real-time detection capabilities. In this study, we present a chemosensing system based on the bindone motif, both as a small molecule (Bin) and embedded in a polymer backbone (PBin), for the effective colorimetric detection of volatile amines. Our system exhibits high selectivity and sensitivity, with detection limits as low as 0.04 ppm for Bin and 1.57 ppm for PBin. The colour change, driven by amine-induced tautomerisation, was confirmed through UV-Vis spectroscopy, NMR spectroscopy, and TD-DFT calculations. pH dependent studies reveal the importance of basicity on the mechanism and selectivity. By incorporating the bindone moiety into the polymer backbone, its thermal stability was significantly enhanced. The versatility of the sensor was demonstrated in solution, and paper-based film formats, with successful application in detecting amines released during fish spoilage. This work highlights the potential of the bindone-based chemosensor as a cost-effective, portable, and efficient tool for monitoring food freshness and other applications requiring the detection of volatile amines.
Introduction
Amines play a crucial role both in nature and industrial production.1 Of this class of compounds, many are volatile, corrosive, and hazardous. Therefore, it is essential to sense and monitor the presence of volatile amines to protect human health and the environment.2–6 Detecting volatile amines is vital across various fields, including biogenic amines as analytes for the early diagnosis of diseases.7,8 Additionally, during food spoilage, microorganisms break down free amino acids into biogenic amines like histamine, cadaverine, putrescine, and trimethylamine, which can significantly harm human health.9–11 Thus, developing highly sensitive detection methods for volatile amines is imperative for a range of practical applications.12,13
Previous reports on gas sensors have included electrochemical sensors, semiconductor sensors, and photochemical sensors.14 These typically utilize highly sensitive metal oxides, however they suffer from inadequate selectivity, instability, and degradation when exposed to moisture or during prolonged use.15 Advancements in methods that monitor changes in resistivity and luminescence have enhanced the sensitivity and selectivity of chemiresistors and fluorescence sensors, achieving detection limits below parts per million (sub-ppm).16–18 Despite these improvements, these systems often necessitate complex or expensive synthetic processes and auxiliary equipment for amine identification.19,20
Colorimetric sensors are attractive as their sensing response is easily understood through a visible colour change and do not require expensive spectroscopic techniques or instrumentation to interpret.21–23 Recently, naked-eye colorimetric chemosensors have achieved significant attention as simple, cost-effective, and real-time sensing for amines and food spoilage detection due to the unambiguous colour change when exposed to the target analyte.24–27 Materials that undergo a colour change when exposed to applied stimuli are observed in many types of materials, however, the high tuneability of organic materials with respect to their optoelectronic properties offers an advantage. The limit of detection (LOD), which marks the onset of the colour change, and the sensitivity of these sensors can be potentially fine-tuned in organic materials, though this largely depends on the specific sensing mechanism used.
An emerging design strategy for amine sensing is to embed receptors into the backbone of polymers with high electron affinity which helps to promote interaction with electron rich amines. A wide variety of polymer types and detection mechanisms have been utilized for amine detection (Table S2†). One strategy involves grafting receptors onto bioderived and biodegradable polymers such cellulose, polylactic acid and polycaprolactone.15,28–31 Conjugated polymers have also been employed, particular for the detection of aromatic amines, which can alter the polymer optoelectronics through π–π interactions.32–34 Recently, polymer networks have also been deployed as an attractive class of materials due to large surface area and luminescence properties.35,36 Metal organic frameworks are another exciting class of porous polymer with use in amine detection.37–40 Polymers of intrinsic microporosity (PIMs) are a valuable class of materials for sensing applications due to the high surface area which leads to efficient interaction with analyte species.41–43 Another desirable feature of PIMs is the highly rigid and contorted backbone leading to both good solubility and processability of thin films.44,45
Biindenylidene-3,10,30-trione (bindone) is reported to display a colorimetric response towards amines at high temperature and has been reported historically as a crude test for amines.46,47 Contemporary reports have also utilised bindone based molecules as chemosensors for heavy metal sensing and pH detection.48,49 The bindone structure possesses an activated CH2 position, rendered slightly acidic by adjacent electron-withdrawing carbonyl groups, making it an ideal candidate for sensing mechanisms involving proton exchange. In this study we present a chemosensing bindone moiety for effective colorimetric detection of volatile amines in solution and in vapor form. As a small molecule (tetrafluorobiindenylidene-3,10,30-trione, Bin) and embedded in a polymer backbone (PBin), we demonstrate a versatile detection system with high selectivity. Through mechanistic studies, we deduce that in the presence of our target analyte, the bindone moiety tautomerizes which invokes a definite colour change. A variety of analytes and different classes of amines were screened to elucidate the selectivity. The unambiguous colorimetric response to amine vapour generated from spoiled fish samples, along with its detection capabilities under various conditions—such as in solution, and dyed paper—demonstrates the practicality of the bindone scaffold for detecting amines for a wide variety of applications. Thus, we developed a new cost effective and versatile chemosensor with structural simplicity as an attractive colorimetric detection tool for volatile amines with potential for application in food packaging (Scheme 1).
 |
| Scheme 1 Synthesis of Bin and PBin. | |
Results and discussion
Synthesis and characterisation
The synthesis was carried out without any chlorinated solvents or expensive catalysts and proceeded from cheap, commercially available starting materials. 4,5-Difluorophthalic anhydride (1), was converted into 5,6-difluoro-1H-indene-1,3(2H)-dione (2) in the presence of tert-butyl acetoacetate, triethylamine and acetic anhydride, as reported in the literature.50 Following this, the compound underwent a base catalysed dimerization in ethanol to afford small molecule target, Bin with 84% yield. The monomer was reacted further to generate polymer PBinvia the SnAr polymerisation with 3,3,3′,3′-tetramethyl-1,1′-spirobiindane-5,5′,6,6′-tetraol. This form of SnAr polymerization, involving the formation of dibenzodioxin linkages, is well-documented and has been shown to proceed efficiently between biscatechol derivatives and electron-deficient tetrafluorinated monomers, as exemplified in the synthesis of PIM-1.51 After workup and subsequent purification via Soxhlet extractions using dichloromethane and methanol to remove small molecule side-products, a moderate yield of 50% by weight was attained. To confirm the desired product, NMR, IR, and elemental analysis was performed on PBin. The proton NMR revealed a new collection of peaks corresponding to the spirobiindane and bindone moieties. The peak broadening can be attributed to the varying electronic environments due to the contorted polymer backbone induced by racemic nature of the spirobiindane monomer. Crucially, the IR spectra of PBin contained peaks at 1700 cm−1 characteristic of carbonyl group stretches. Elemental analysis of PBin revealed carbon and hydrogen composition in close agreement with predicted monomer unit, with the slight discrepancy attributed to the polymer end groups.
The polymerisation was also confirmed using GPC and weight average molecular weight (mw) of around 16 kg mol−1 observed with polydispersity index of 1.3. Despite the contorted spirobisindane moiety along the polymer backbone, the microporosity of PBin was modest and gas sorption analysis revealed low nitrogen adsorption at 77 K (Fig. S14†). Conversely, PBin did display significant CO2 uptake (22 cm3 g−1 at 273 K) suggesting the presence of micropores smaller than 0.7 nm in diameter. This is further supported by the NLDFT pore size distribution derived from the CO2 adsorption isotherm at 273 K, which shows that the majority of the micropore volume is below 0.8 nm confirming the materials potential to uptake certain gaseous analytes (Fig. S13†). SEM imaging was used to give insight into the morphology of the two materials. PBin revealed macroporous cavities whereas Bin appears as a crystalline solid (Fig. S15†). The thermal stability of Bin and PBin were investigated and through TGA analysis it was revealed that Bin commenced sharp decomposition at 200 °C whereas the polymeric PBin remained stable up to 400 °C without significant mass loss and has glass transition temperature (Tg) of 210 °C (Fig. S10 and 11†). The findings reveal that embedding the bindone moiety into the polymer backbone greatly improved its thermal stability, increasing its practical utility.
Optical response
Firstly, extensive UV-Vis absorption studies were carried out with the small molecule Bin to understand the selectivity of the colour change. Solutions of Bin were prepared in tetrahydrofuran and mixed with 0.1 equivalents of a variety of different amines, namely primary, secondary and tertiary amines, alkyl as well as aromatic amines, and key biogenic amines. Pyridine and hydrazine were also investigated (Fig. 1).
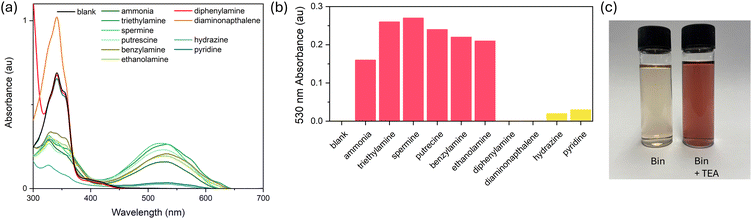 |
| Fig. 1 (a) UV-Vis absorption spectra of solutions of Bin (1 × 10−5 mol L−1, THF) with addition of 0.1 eq. of amine. (b) Relative absorbance of Bin at 530 nm for each amine added (c) image of solutions of Bin before and after treatment with base. | |
Before any amine is added, Bin exists mainly as the keto isomer and absorbs in the UV region with a λmax at 340 nm. There is an additional broad low intensity absorption centred around 400 nm which crosses into the visible region, which is responsible for the pale-yellow colour of the solution. Upon adding small quantities of alkyl amines or ammonia (triethylamine, spermine, putrescine, ammonia, ethanolamine, benzylamine), there is an instant change in the absorption, with a new strong absorption at 530 nm, while the peak at 340 nm is diminished. This corresponds to a stark colour change from pale-yellow to purple. For aromatic amines, 1,5-diaminonaphthalene and diphenylamine, the colour change is not observed, and the changes to the UV absorption can be attributed to the absorption of the aromatic compounds themselves. For the nitrogen containing non-amine compounds, pyridine and hydrazine, a peak is observed at 530 nm, however the intensity is very weak. It was noted that with hydrazine, the absorption at 340 nm is also diminished, perhaps due to highly reactive hydrazine, which may undergo a side reaction with Bin. From this study it is clear that the bindone system utilized is selective for alkyl amines and ammonia over aromatic amines.
An analogous study was conducted with the polymeric counterpart PBin and a similar result was observed. When PBin was exposed to small amounts of alkyl amine, the absorption around 400 nm decreased, revealing a new peak at approximately 550 nm. The colour change was less distinctive, and this is due to the broadening of the absorption bands due to the polymeric nature. Analogous to Bin, PBin did not undergo a large change in presence of the aromatic amines and showed selectivity for alkyl amines over aryl amines and pyridine. The λmax of peaks in PBin compared with corresponding peaks in Bin are redshifted, presumably due to the change from electron withdrawing fluorine moieties to the more electron rich oxygen units (Fig. 2).
 |
| Fig. 2 (a) UV-Vis absorption spectra of solutions of PBin (0.1 mg mL−1, THF) with addition of 0.1 eq. of amine. (b) Relative absorbance of PBin at 530 nm for each amine added. (c) Image of solutions of PBin before and after treatment with base. | |
The fluorescence response was monitored upon addition to a selection of amines (Fig. S16†), however, both materials exhibited very low fluorescence before and after exposure to amines therefore we report that the system is not suitable as a fluorescent sensor for amines.
To understand the selectivity of our chemosensor, Bin and PBin, were exposed to a range of anions in THF solution (Fig. S17†). Both materials exhibited a very minor response to water, chloride and phosphate anions. Bin and PBin did exhibit a strong response when exposed to sodium hydroxide solution, implying that the chemosensor is also selective towards strong bases. This correlates with our hypothesised mechanism involving a keto–enol(ate) tautomerism, which can also be promoted under strongly basic conditions.
The sensitivity of the bindone chemosensor was evaluated by exposing the sensor to extremely low concentrations of triethylamine to elucidate the onset of the colorimetric response. Bin displayed very high sensitivity to triethylamine and a slight increase in the absorption at 530 nm was noted at as low as 0.0001 ppm (Fig. 3). At this low concentration range, below 1 ppm, the colour change was not obvious to the human eye. At 10 ppm and above there was a large increase in absorption at 530 nm and a discernible colour change was observed. At concentrations above 10 ppm, the 530 nm absorption plateaus and no further increase is observed. PBin was found to be less sensitive than the small molecule counterpart and only minor spectral changes were observed up to 100 ppm concentration of triethylamine. A noticeable colour change was observed at 0.1% triethylamine and above and the absorption reached a plateau at 1% with no increase with higher concentrations. In addition, the low limit of detection (LOD) was estimated for Bin and PBin as 0.04 ppm and 1.57 ppm respectively using the following equation:
where
σ is the standard deviation of the blank solution and
σ is the slope of the calibration curve (Fig. S18
†). The results demonstrate the excellence of the bindone moiety in detecting amines with high sensitivity and resolution with LOD on the ppm scale, comparable to state-of-the-art amine sensing polymers (Table S2
†).
 |
| Fig. 3 UV-Vis absorption spectra of Bin (a) and PBin (b) upon exposure to low levels of triethylamine (TEA) to elucidate detection threshold for amine sensing. Solutions were prepared as 1 × 10−5 mol L−1 THF and 0.1 mg mL−1 THF for Bin and PBin respectively. | |
The disparity in detection limit between Bin and PBin can be explained by the variation in electronic environment of the bindone moiety. The electron withdrawing fluorine atoms in Bin serve to encourage the deprotonation of the keto form, likely stabilising the negative charge generated through the tautomerisation process. In the polymer backbone of PBin, the fluorine atoms are replaced with electron donating oxygen atoms which render the process slightly less favourable.
Mechanism of optical sensing
As mentioned in the previous section, the proposed mechanism responsible for the colour change is attributed to the amine induced keto to enol(ate) tautomerisation via deprotonation of the bindone moiety. The underpinning mechanism is supported by NMR spectroscopy and computational investigation. A sample of Bin in dichloromethane-d2 was deliberately contaminated with 5 μL of triethylamine and the NMR spectra before and after were compared. Upon addition of base, the CH2 peak at 4.1 ppm disappeared and is replaced by a broad singlet at 6.7 ppm with relative intensity of 1 proton suggesting tautomerisation to enol. A 2D NMR (HSQC) experiment was carried out and confirmed the peak at 6.7 ppm to be a CH signal in the expected alkene region (Fig. S5†). The OH signal is absent from the spectra likely due to the rapid proton exchange with the base as the molecule exchanges between the enol and enolate form. The 2D NMR experiment was performed in acetonitrile-d3 to increase solubility of enol facilitating better resolution. Due to the triketone nature of the bindone moiety, there exists three possible isomers of the enol form. Using DFT calculations, the energy of each isomer was calculated to ascertain the lowest energy (Fig. S19†). It was found that the carbonyl group adjacent to the methylene group is converted to the hydroxyl group during tautomerisation resulting in the lowest energy structure. Using TD-DFT, the UV/Vis absorption of Bin was simulated, and it was found to correlate with the experimentally observed spectra with the absorption of the enol isomer shifted to approximately 500 nm compared to the keto isomer at 350 nm (Fig. 4).
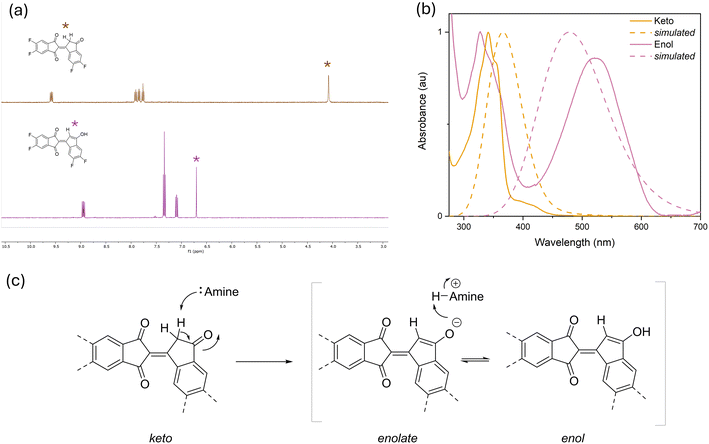 |
| Fig. 4 (a) NMR spectra of Bin before and after exposure to TEA, (b) experimental vs. simulated UV/Vis absorption spectra of Binketo and Binenol (TD-DFT B3LYP 6311G(dp)), and (c) proposed amine promoted keto–enol(ate) tautomerisation mechanism. | |
To further validate the tautomerisation mechanism, a pH dependant UV–Vis study was carried out to understand the acid/base sensitivity of the bindone system. Due to the low solubility of PBin in aqueous solution, Bin was studied as a model small molecule. DMSO/H2O solutions of Bin were prepared with HEPES buffer adjusted to pH 1 to 11 using HCl and NaOH respectively, then absorption spectra of each sample were acquired (Fig. 5). Under acidic conditions an absorption at 350 nm was observed, and as the pH was increased towards pH 7 a new band became visible at 528 nm. From pH 7 to 9 a significant sharp increase in the absorption was observed including a decrease in the absorption at 350 nm. The pH sensitivity confirms the colorimetric response towards amines is due to the basicity of the amino analytes. To quantify basicity, the pKa of the conjugate acids (pKaH) of each amine is compiled and used as an evaluation of basicity (Table S1†). The selectivity towards aliphatic amines over aromatic amines is a result of the higher pKaH of aliphatic amines. Ammonia, triethylamine, spermine, putrescine, benzylamine, ethanolamine and hydrazine all have pKaH between 8.1 and 10.8 whereas the aromatic amines studied (diphenylamine, 1,5-diaminonaphthalene and pyridine) have values below 5.1.
 |
| Fig. 5 (a) pH dependent UV-Vis absorption spectra of Bin at pH 1–11 (5 × 10−5 mol L−1 DMSO/H2O (4 : 6, v/v) HEPES-buffered solutions) and 528 nm absorption of Bin at varying pH. (b) Reversibility study of Bin and PBin solutions showing absorption at 530 nm after exposure to triethylamine (1 μL 7.2 M), then HCl (1 μL, 7.2 M, aq.) over 6 cycles (1 × 10−5 mol L−1 THF and 0.1 mg mL−1 THF solutions of Bin and PBin respectively). | |
The stability and reversibility of the chemosensor was evaluated via analyte exposure and regeneration cycles. Solutions of Bin and PBin were prepared in THF and small quantities of triethylamine followed by hydrochloric acid solution were added and UV-Vis absorption measured upon each addition. The solutions of Bin and PBin exhibited instant colour change after exposure to triethylamine and producing an intense absorption at 530 nm in the UV-Vis spectra as expected. Upon subsequent addition of acid, the colour of the solution reverted and absorption at 530 nm completely diminished. The compound revealed stable cycling stability over 6 amine/acid cycles highlighting the reversibility of the bindone motif and the potential in molecular logic switches.52,53
Paper-based sensing of amine vapor
To determine the capability of the bindone based materials to detect volatile amine vapours, paper films were prepared via dip coating test strips from THF solutions of Bin and PBin.54–56 After leaving to dry, the paper films were exposed to an ammonia atmosphere generated in sealed chambers using solutions of varying concentrations ammonium hydroxide for 10 minutes. Bin displayed high sensitivity to ammonia vapour, exhibiting a stark colour change when exposed to even trace amounts. A colour change from off white to purple was observed on the test trips for ammonium hydroxide concentration 0.01%–5%. In contrast, PBin displayed a less immediate and contrasting colour change from yellow to dark purple when exposed to the ammonia vapor. The onset of the detection was just below 0.1% ammonium hydroxide concentration and is at least an order of magnitude less sensitive than Bin. The colour change for PBin test strips gradually increased intensity with increasing ammonia atmosphere, whereas Bin test strips exhibit obvious and intense colour change even in low concentrations of ammonia (Fig. 6).
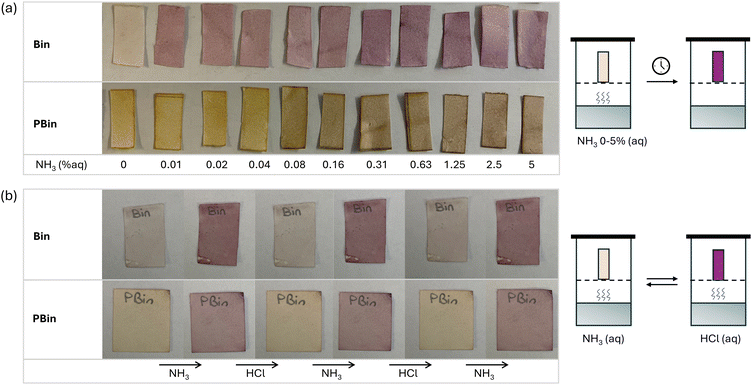 |
| Fig. 6 (a) Paper test strips, dip coated from THF solutions of Bin and PBin then exposed to ammonia atmosphere for 10 minutes generated by varying amounts of ammonium hydroxide solution in a sealed environment, (b) reversibility of Bin and PBin paper test strips exposed to consecutive ammonia and hydrochloric acid vapor. | |
The stability and reversibility of paper test strips was evaluated. Paper test strips were coated in Bin and PBin (dip coated from 1 × 10−5 mol L−1 and 0.1 mg mL−1 THF solutions, respectively) and exposed to ammonia vapour (30% aq) invoking clear colorimetric response. Test strips were removed from the amine vapour chamber and left to stand in air for several days with no observable change, revealing that the colour change was irreversible under ambient conditions. The strips were then exposed to concentrated HCl vapour (12 M aq), and the colour change instantly reversed. The paper test strips remained stable through multiple consecutive exposures to amine and HCl vapour, consistently displaying a clear colorimetric response across numerous cycles. This underscores the potential for reusing PBin and Bin paper test strips, enhancing the sustainability and versatility in various applications.
Real world application of colorimetric sensor
The production of biogenic amines through microbial fermentation in food is a key indicator of freshness. Consequently, developing a simple, portable detector for reliable, fast, and real-time food freshness assessment would be highly advantageous. In this study we present a real-world example of food freshness testing using the newly developed chemosensor. Paper test strips were dip coated from Bin and PBin solutions and dried. The test strips were placed inside sealed containers under argon with pieces of cod and left for five days at temperatures of −20 °C, 5 °C and 20 °C (Fig. 7). Fish samples incubated at −20 °C and 5 °C did not induce an obvious response in the test strips, however the fish sample stored at 20 °C had significantly deteriorated and a clear colour change was observed on both the Bin and PBin test strips, indicating practical use in food quality detection. Due to the complexity of variables involved in real-world food spoilage detection, further studies are required to understand the effects of humidity and competing analytes on the detection mechanism, however the study clearly highlights the potential for the bindone moiety to be utilised in food packaging for the monitoring of food spoilage.
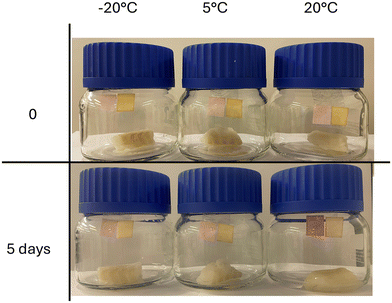 |
| Fig. 7 Food spoilage study using samples of cod incubated at temperatures −20 °C, 5 °C and 20 °C for five days with paper test strips of Bin (left) and PBin (right) displaying colorimetric response to food spoilage. | |
Conclusion
In this study we developed a highly sensitive, selective and rapid chemosensing bindone motif, providing a small molecule and polymeric material that both exhibit a clear colorimetric response and selectivity to alkyl amines. Both Bin and PBin displayed changes in absorption spectra, with an unambiguous colour change underpinned by an amine catalysed keto–enol(ate) tautomerisation mechanism. The mechanism was confirmed through UV-Vis spectroscopy, 1D and 2D NMR, and computational studies. pH dependent experiments confirm that the basicity of amines is intrinsic to deprotonation mechanism, and it was revealed that the pKa of analytes is inherent to selectivity. The tautomerisation process was found to be highly reversible and cycled between both states without loss of function. Detection limits as low as 0.04 ppm for Bin and 1.57 ppm for PBin were achieved, demonstrating exceptional sensitivity. By incorporating the chemosensing motif into the polymer backbone, its thermal stability was significantly enhanced, extending its practical utility. The versatility of the sensing platform was outlined by using solution detection, as well as the detection of vapours on paper substrates, which could be regenerated and reused multiple times without degradation. A food spoiling experiment was carried out highlighting the potential for real-world food packaging application. A key advantage of the bindone chemosensor over many state-of-the-art sensors is its capability for naked-eye detection, along with its straightforward and cost-effective synthesis. Due to the ease of preparation, portability, simple operation, selectivity, sensitivity and reversibility, the bindone chemosensor has the potential to be used for the detection of amines for wide variety of applications.
Data availability
Data supporting the study “Bindone-Based Polymer for Colorimetric Detection of Volatile Amines” are provided in the manuscript and ESI.†
Additional raw data supporting the study are available under a licence from the University of Glasgow Enlighten Research Data Repository at https://dx.doi.org/10.5525/gla.researchdata.1859.
Conflicts of interest
The authors declare no conflicts of interest.
Acknowledgements
DW (EP/V027425/1) and DT (EP/V027735/1) thank the EPSRC for funding. GC thanks the Leverhulme Trust for the award of a research fellowship.
References
-
S. A. Lawrence, Amines: synthesis, properties and applications, Cambridge University Press, 2004 Search PubMed
.
- S. K. Kannan, B. Ambrose, S. Sudalaimani, M. Pandiaraj, K. Giribabu and M. Kathiresan, A review on chemical and electrochemical methodologies for the sensing of biogenic amines, Anal. Methods, 2020, 12(27), 3438–3453 RSC
.
- N. Kaur, S. Chopra, G. Singh, P. Raj, A. Bhasin, S. K. Sahoo, A. Kuwar and N. Singh, Chemosensors for biogenic amines and biothiols, J. Mater. Chem. B, 2018, 6(30), 4872–4902 RSC
.
- P. R. Lakshmi, B. Mohan, P. Kang, P. Nanjan and S. Shanmugaraju, Recent advances in fluorescence chemosensors for ammonia sensing in the solution and vapor phases, Chem. Commun., 2023, 59(13), 1728–1743 CAS
.
- B. Liu, J. Zhuang and G. Wei, Recent advances in the design of colorimetric sensors for environmental monitoring, Environ. Sci.:Nano, 2020, 7(8), 2195–2213 RSC
.
- L. Wang, X. Ran, H. Tang and D. Cao, Recent advances on reaction-based amine fluorescent probes, Dyes Pigm., 2021, 194, 109634 CAS
.
- H. Vasconcelos, L. C. C. Coelho, A. Matias, C. Saraiva, P. A. S. Jorge and J. M. M. M. de Almeida, Biosensors for Biogenic Amines: A Review, Biosensors, 2021, 11(3), 82 CAS
.
- C. Dincer, R. Bruch, E. Costa-Rama, M. T. Fernández-Abedul, A. Merkoçi, A. Manz, G. A. Urban and F. Güder, Disposable Sensors in Diagnostics, Food, and Environmental Monitoring, Adv. Mater., 2019, 31(30), 1806739 Search PubMed
.
- R. S. Andre, L. A. Mercante, M. H. M. Facure, R. C. Sanfelice, L. Fugikawa-Santos, T. M. Swager and D. S. Correa, Recent Progress in Amine Gas Sensors for Food Quality Monitoring: Novel Architectures for Sensing Materials and Systems, ACS Sens., 2022, 7(8), 2104–2131 CrossRef CAS PubMed
.
- M. J. Grant, K. M. Wolfe, C. R. Harding and G. C. Welch, Biogenic amine sensors using organic π-conjugated materials as active sensing components and their commercialization potential, J. Mater. Chem. C, 2023, 11(29), 9749–9767 CAS
.
- J. Zhang, Y. Yang, L. Zeng and J. Wang, A ratiometric fluorescence platform for on-site screening meat freshness, Food Chem., 2024, 436, 137769 CAS
.
- Z. Li, S. Hou, H. Zhang, Q. Song, S. Wang and H. Guo, Recent advances in fluorescent and colorimetric sensing for volatile organic amines and biogenic amines in food, Adv. Agrochem, 2023, 2(1), 79–87 CrossRef CAS
.
- A. Naila, S. Flint, G. Fletcher, P. Bremer and G. Meerdink, Control of Biogenic Amines in Food—Existing and Emerging Approaches, J. Food Sci., 2010, 75(7), R139–R150 CrossRef CAS PubMed
.
- X. Zhou, S. Lee, Z. Xu and J. Yoon, Recent Progress on the Development of Chemosensors for Gases, Chem. Rev., 2015, 115(15), 7944–8000 CrossRef CAS PubMed
.
- T. Siripongpreda, K. Siralertmukul and N. Rodthongkum, Colorimetric sensor and LDI-MS detection of biogenic amines in food spoilage based on porous PLA and graphene oxide, Food Chem., 2020, 329, 127165 CrossRef CAS PubMed
.
- Y. J. Diaz, Z. A. Page, A. S. Knight, N. J. Treat, J. R. Hemmer, C. J. Hawker and J. Read de Alaniz, A Versatile and Highly Selective Colorimetric Sensor for the Detection of Amines, Chem. – Eur. J., 2017, 23(15), 3562–3566 CrossRef CAS PubMed
.
- S. Ulrich, S. O. Moura, Y. Diaz, M. Clerc, A. G. Guex, J. R. de Alaniz, A. Martins, N. M. Neves, M. Rottmar, R. M. Rossi, G. Fortunato and L. F. Boesel, Electrospun colourimetric sensors for detecting volatile amines, Sens. Actuators, B, 2020, 322, 128570 CrossRef CAS
.
- M. K. Noushija, A. V. Krishna, R. T. M. Ali and S. Shanmugaraju, Reactivity-based small-molecule fluorescence probes for sensing biogenic amine cadaverine – a biomarker to determine food freshness, Sens. Diagn., 2025 Search PubMed
, Advance Article.
- M. Sabela, S. Balme, M. Bechelany, J.-M. Janot and K. Bisetty, A Review of Gold and Silver Nanoparticle-Based Colorimetric Sensing Assays, Adv. Eng. Mater., 2017, 19(12), 1700270 CrossRef
.
- Z. Tang, J. Yang, J. Yu and B. Cui, A Colorimetric Sensor for Qualitative Discrimination and Quantitative Detection of Volatile Amines, Sensors, 2010, 10(7), 6463–6476 CrossRef CAS PubMed
.
- B. M. Oh, N. Y. Cho, E. H. Lee, S. Y. Park, H. J. Eun and J. H. Kim, Colorimetric and fluorometric bimodal amine chemosensor based on deprotonation-induced intramolecular charge transfer: Application to food spoilage detection, J. Hazard. Mater., 2024, 465, 133150 CrossRef CAS PubMed
.
- X. Zhong, D. Huo, H. Fa, X. Luo, Y. Wang, Y. Zhao and C. Hou, Rapid and ultrasensitive detection of biogenic amines with colorimetric sensor array, Sens. Actuators, B, 2018, 274, 464–471 CrossRef CAS
.
- V. S. A. Piriya, P. Joseph, S. C. G. K. Daniel, S. Lakshmanan, T. Kinoshita and S. Muthusamy, Colorimetric sensors for rapid detection of various analytes, Mater. Sci. Eng., C, 2017, 78, 1231–1245 CrossRef PubMed
.
- M. Saravanakumar, B. Umamahesh, R. Selvakumar, J. Dhanapal, S. K. Ashok Kumar and K. I. Sathiyanarayanan, A colorimetric and ratiometric fluorescent sensor for biogenic primary amines based on dicyanovinyl substituted phenanthridine conjugated probe, Dyes Pigm., 2020, 178, 108346 CrossRef CAS
.
- L. Chen, H. Jiang, M. Zheng, Z. Li, N. Li, S. Zhao and X. Liu, Fly-antennae-inspired biomass-based fluorescent platform for NH3 quantitative detection and visual real-time monitoring of seafood spoilage, J. Hazard. Mater., 2022, 434, 128868 CrossRef CAS PubMed
.
- H. Li, Q. Chen, J. Zhao and Q. Ouyang, Non-destructive evaluation of pork freshness using a portable electronic nose (E-nose) based on a colorimetric sensor array, Anal. Methods, 2014, 6(16), 6271–6277 RSC
.
- X. Chen, Y. Tu, S. Cheng, X. Guo, T. Lu, Y. Guo, X. Huang, Y. Xiong and B. Z. Tang, A colorimetric and ratiometric fluorescent paper chip for biogenic amine monitoring based on a simple pH-sensitive AIEgen, Chem. Eng. J., 2022, 450, 137928 CrossRef CAS
.
- A. Lopera-Valle and A. Elias, Colorimetric indicators for volatile amines based on succinic anhydride (SAh)-grafted poly (lactic acid) (PLA), Anal. Methods, 2020, 12(19), 2499–2508 CAS
.
- H. Nawaz, J. Zhang, W. Tian, K. Jin, R. Jia, T. Yang and J. Zhang, Cellulose-based fluorescent sensor for visual and versatile detection of amines and anions, J. Hazard. Mater., 2020, 387, 121719 CAS
.
- D. Kossyvaki, A. Barbetta, M. Contardi, M. Bustreo, K. Dziza, S. Lauciello, A. Athanassiou and D. Fragouli, Highly Porous Curcumin-Loaded Polymer Mats for Rapid Detection of Volatile Amines, ACS Appl. Polym. Mater., 2022, 4(6), 4464–4475 CAS
.
- H. Nawaz, X. Zhang, S. Chen, T. You and F. Xu, Recent studies on cellulose-based fluorescent smart materials and their applications: A comprehensive review, Carbohydr. Polym., 2021, 267, 118135 CAS
.
- Y.-J. Jin and G. Kwak, Detection
of biogenic amines using a nitrated conjugated polymer, Sens. Actuators, B, 2018, 271, 183–188 CAS
.
- S. Rochat and T. M. Swager, Fluorescence Sensing of Amine Vapors Using a Cationic Conjugated Polymer Combined with Various Anions, Angew. Chem., Int. Ed., 2014, 53(37), 9792–9796 CAS
.
- Y.-J. Zhao, K. Miao, Z. Zhu and L.-J. Fan, Fluorescence Quenching of a Conjugated Polymer by Synergistic Amine-Carboxylic Acid and π–π Interactions for Selective Detection of Aromatic Amines in Aqueous Solution, ACS Sens., 2017, 2(6), 842–847 CrossRef CAS PubMed
.
- Anamika, N. Gupta, A. Maurya and B. K. Kuila, Highly Fluorescent Conjugated Polymer Network as Reversible and Colorimetric Probe for Ultrafast Detection of BF3 and Amine, ACS Appl. Polym. Mater., 2025, 7(4), 2584–2594 CrossRef CAS
.
- J. Yu and C. Zhang, Fluorescent sensing for amines with a low detection limit based on conjugated porous polymers, J. Mater. Chem. C, 2020, 8(46), 16463–16469 RSC
.
- J. Othong, J. Boonmak, N. Wannarit, F. Kielar, T. Puangmali, W. Phanchai and S. Youngme, Dual mode in a metal-organic framework based mixed matrix membrane for discriminative detection of amines: Vapoluminescent and vapochromic response, Sens. Actuators, B, 2021, 343, 130066 CrossRef CAS
.
- C. Zhou, Y. Liu, Z. Liu, Z. Qiu, Z. Sun, M. Chen, B. Xie, H.-M. Wen and J. Hu, A highly sensitive MOF fluorescence probe for discriminative detection of aliphatic and aromatic amines, J. Mater. Chem. C, 2025, 13, 5611–5616 RSC
.
- T. Leelasree and H. Aggarwal, MOF sensors for food safety: ultralow detection of putrescine and cadaverine in protein rich foods, J. Mater. Chem. C, 2022, 10(6), 2121–2127 RSC
.
- S. Chen, X. Duan, C. Liu, S. Liu, P. Li, D. Su, X. Sun, Y. Guo, W. Chen and Z. Wang, La-Ce-MOF nanocomposite coated quartz crystal microbalance gas sensor for the detection of amine gases and formaldehyde, J. Hazard. Mater., 2024, 467, 133672 CrossRef CAS PubMed
.
- M. W. Alam, S. I. Bhat, H. S. Al Qahtani, M. Aamir, M. N. Amin, M. Farhan, S. Aldabal, M. S. Khan, I. Jeelani, A. Nawaz and B. Souayeh, Recent Progress, Challenges, and Trends in Polymer-Based Sensors: A Review, Polymers, 2022, 14(11), 2164 CrossRef CAS PubMed
.
- F. Marken, L. Wang, Y. Zhao, Z. Li, M. Amiri and H. Imanzadeh, Polymers of intrinsic microporosity (PIMs) in sensing and in electroanalysis, Curr. Opin. Chem. Eng., 2022, 35, 100765 CrossRef
.
- F. Topuz, M. H. Abdellah, P. M. Budd and M. A. Abdulhamid, Advances in Polymers of Intrinsic Microporosity (PIMs)-Based Materials for Membrane, Environmental, Catalysis, Sensing and Energy Applications, Polym. Rev., 2024, 64(1), 251–305 CrossRef CAS
.
- N. B. McKeown, The synthesis of polymers of intrinsic microporosity (PIMs), Sci. China: Chem., 2017, 60(8), 1023–1032 CrossRef CAS
.
- N. B. McKeown, Polymers of Intrinsic Microporosity (PIMs), Polymer, 2020, 202(1), 122736 CrossRef CAS
.
-
M. Večeřa and J. Gasparič, Amines, in Detection and Identification of Organic Compounds, ed. M. Večeřa and J. Gasparič, Springer US, Boston, MA, 1971, pp. 317–353 Search PubMed
.
- Y. R. Uldrikis and G. Y. Dubur, Detection of 5-aminopyrimidines, Chem. Heterocycl. Compd., 1970, 4(2), 260–260 CrossRef
.
- S.-Q. Cai, X.-H. Cai and K.-F. Zhang, Biindenylidene-3,10,30-trione: An Interesting Solvatochromic Molecule and Its Applications for Visual pH Detection and DCM Identification, J. Solution Chem., 2024, 53(11), 1476–1491 CrossRef
.
- Y.-J. Kim, S.-Y. Gwon and S.-H. Kim, [1,2′]Biindenylidene-3,1′-3′-trion(bindone): Colorimetric detection of volatile organic compounds(VOCs) ethylamine using highly selective Hg2+ chemosensor in aqueous solution, Dyes Pigm., 2016, 133, 184–188 CrossRef CAS
.
- H. Yao, Y. Cui, R. Yu, B. Gao, H. Zhang and J. Hou, Design, Synthesis, and Photovoltaic Characterization of a Small Molecular Acceptor with an Ultra-Narrow Band Gap, Angew. Chem., Int. Ed., 2017, 56(11), 3045–3049 CrossRef CAS PubMed
.
- N. B. McKeown, Polymers of Intrinsic Microporosity (PIMs), Polymer, 2020, 202, 122736 CrossRef CAS
.
- L. Liu, P. Liu, L. Ga and J. Ai, Advances in Applications of Molecular Logic Gates, ACS Omega, 2021, 6(45), 30189–30204 CrossRef CAS PubMed
.
- J. Andréasson and U. Pischel, Molecules with a sense of logic: a progress report, Chem. Soc. Rev., 2015, 44(5), 1053–1069 RSC
.
- A. W. Martinez, S. T. Phillips, G. M. Whitesides and E. Carrilho, Diagnostics for the Developing World: Microfluidic Paper-Based Analytical Devices, Anal. Chem., 2010, 82(1), 3–10 CrossRef CAS PubMed
.
- S. K. Mahadeva, K. Walus and B. Stoeber, Paper as a Platform for Sensing Applications and Other Devices: A Review, ACS Appl. Mater. Interfaces, 2015, 7(16), 8345–8362 CrossRef CAS PubMed
.
- D. D. Liana, B. Raguse, J. J. Gooding and E. Chow, Recent Advances in Paper-Based Sensors, Sensors, 2012, 12(9), 11505–11526 CrossRef CAS PubMed
.
|
This journal is © The Royal Society of Chemistry 2025 |
Click here to see how this site uses Cookies. View our privacy policy here.