DOI:
10.1039/D4MA01124D
(Paper)
Mater. Adv., 2025, Advance Article
Novel polydopamine/halloysite nanotube-reinforced brushite calcium phosphate cement for bone regeneration with synergistic regulation of mechanical/osteogenic capacity†
Received
14th November 2024
, Accepted 13th February 2025
First published on 15th February 2025
Abstract
Bone regeneration remains a clinical challenge with limited bone substitutes. Brushite calcium phosphate cements (Bru-CPCs), possessing good bioactivity and biocompatibility, are one of the widely studied bone graft materials. However, their further application in the long-term remodeling of bone is limited by the low compressive strength. Adding additives has been a promising strategy to solve the above problem. Herein, halloysite nanotubes (HNTs) with a unique rod-like structure and excellent biocompatibility were chosen as reinforced materials to fabricate bone repair materials. Inspired by the adhesive proteins in mussels, we modified the HNTs’ surface with polydopamine (PDA) to improve the inorganic–inorganic phase interfacial interactions between the HNTs and Bru-CPCs. Bru-CPCs, Bru-CPCs/1.5%HNTs and Bru-CPCs/1.5%HNTs@PDA were fabricated and the mechanical properties and biological activity of the bone repair materials were evaluated in detail. All the results indicated that Bru-CPCs incorporated with 1.5 wt% HNTs@PDA have good compressive strength and osteo-differentiation properties, making them a prospective biomaterial for bone-tissue repair.
Introduction
Large bone defect repair has always been a major challenge in the field of orthopedics.1–3 Autografts, allografts and xenografts are unable to meet the increasing demand for current clinical needs. Biomaterials have been extensively studied as bone grafts.4–7 Calcium phosphate cements (CPCs), with low-temperature self-setting, high biocompatibility, and similar constituents to bone, have attracted significant interest as implant materials in bone reconstruction surgery.8–12 Brushite cements (Bru-CPCs) possess superior biodegradability and osteoconductivity, but inferior mechanical strength, rendering them unsuitable for current clinical applications.13–16 Hence, it's important to improve the mechanical strength of Bru-CPCs before using them as bone repair materials.
To date, an efficiency strategy is adding additives, such as polymers (chitosan, cellulose ethers, collagen, starch, etc.), metal ions (Fe3+, Zn2+, Mg2+, Si2+, etc.) and nanoclay (montmorillonite (MMT), kaolinite (Kaol), LAPONITE® (Lap), halloysite nanotubes (HNTs), etc.) to CPCs.17–25 Several studies demonstrated the favorable effects of nanoclay on mechanical strength, cellular adhesion and proliferation. Compared to the other nanoclays, HNTs have been widely studied as biomaterials due to their excellent biocompatibility, unique tubular structure, selective drug loading, high mechanical strength and abundant resources.26–29 Research has explored the function of HNTs in modulating osteogenesis.30–33 Firstly, silicate ions released from HNTs stimulate osteogenic differentiation and increase the expression of ALP and OCN genes.31 Secondly, the nanotube structure of HNTs contributes to the stabilization of extracellular proteins, leading to an upregulation of ALP activity.32 Thirdly, HNTs that accumulate within cells promote osteogenesis by directly engaging in protein interactions and intracellular signaling pathways.33 Zhao et al. developed a hierarchical composite scaffold with a deferoxamine delivery system, DFO@GMs-PDA/PCL-HNTs (DGPN).32 They demonstrated that DGPN can promote bone regeneration and accelerate cranial defect healing. Wu et al. prepared HNT-based bone repair materials.30 The incorporation of HNTs led to an enhanced mechanical performance and upregulated the expression of osteogenic differentiation-related genes. Ji et al. developed a porous scaffold for bone regeneration by freeze-drying a mixture of nano-scale drug-loaded halloysite nanotubes (HNTs) and gelatin.34 The scaffold shows a porous structure and excellent biocompatibility. Compared with the gelatin scaffold, HNTs can significantly increase the mechanical properties of the composite scaffold by >300% and match natural cancellous bone. Hence, HNTs may be a promising additive for improving the mechanical properties of bone repair materials.
However, the above HNT-based bone repair materials were composed of inorganic HNTs and organic polymers together. Polymer chains can wrap around the HNTs, which benefits the modification of the interfacial interactions between the HNTs and the polymer, thereby improving the mechanical properties of bone repair materials.30,35,36 When introducing HNTs into Bru-CPCs, the interfacial interaction problem between inorganic HNTs and inorganic Bru-CPCs may hinder the mechanical properties of Bru-CPCs. Polydopamine (PDA) can be papered via the self-polymerization of dopamine (DA) in alkaline solution. The strong covalent or noncovalent interactions (hydrogen bonds or stacking interactions, between catechol moieties and substrates) endow extraordinary adhesive properties to PDA. Numerous studies have demonstrated that PDA coating is universal to nearly all substrates, and is like that of mussel adhesive protein, endowing the substrate with biocompatibility, post-functionality and other useful properties.37 The post-modification of the HNT surface with PDA may modify the interfacial interactions between the inorganic HNTs and inorganic Bru-CPCs.
Herein, aiming to improve the mechanical properties of bone repair materials, a novel Bru-CPCs/1.5%HNTs with HNTs was developed. The mechanical properties and biological activity of the Bru-CPCs/1.5%HNTs scaffold were investigated. Considering the interfacial interactions between HNTs and Bru-CPCs and the high adhesive property of PDA, the HNT surface was then coated by a PDA layer. Bru-CPCs/1.5%HNTs@PDA scaffolds were constructed. The effect of HNTs@PDA on the mechanical properties and biological activity of the scaffolds were assessed and discussed in detail. All the results demonstrated that adding HNTs@PDA can improve the compressive strength and bioactivity properties of Bru-CPCs/1.5%HNTs@PDA scaffolds for effective bone cell adhesion and growth, which may be widely used as bone repair materials in the further.
Results and discussion
Characterization of Bru-CPCs@HNTs
Bru-CPCs and modified Bru-CPCs/HNTs were first prepared, and the effect of HNT content on the mechanical properties of Bru-CPCs was investigated, as shown in Fig. 1B and Fig. S1 (ESI†). The results revealed that the mechanical properties increased initially and then decreased with increasing the content of HNTs. The optimal mechanical performance was achieved with adding 1.5% HNTs, reaching 12.16 MPa. These findings indicate that HNTs can enhance the mechanical properties of the modified Bru-CPCs/HNTs. But the weak interfacial interaction between HNTs and Bru-CPCs constrains the potential enhancement of mechanical properties. Additionally, we tested the setting time, pH, and degradation performance, as depicted in Fig. S2 (ESI†). The initial setting time for Bru-CPCs was found to be 10 minutes, and the final setting time was 21 minutes. For Bru-CPCs/1.5%HNTs, the initial setting time was 9 minutes, and the final setting time was 20 minutes. The addition of HNTs had a negligible effect on the setting time. After immersion in SBF solution for 12 hours, the pH of the samples was approximately 5.9. Since SBF was not a buffered system, the pH did not increase to 7.4 within 12 hours. The samples were then incubated for an additional 12 hours, and the pH reached 7.4. The pH values remained almost unchanged around 7.4 for all samples with further incubation. Regarding degradation performance, Bru-CPCs/1.5%HNTs exhibited a slightly slower degradation rate compared to Bru-CPCs. To further enhance the mechanical properties of Bru-CPCs and increase the interfacial interaction between HNTs and Bru-CPCs, we proposed to modify HNTs by introducing polydopamine to prepare HNTs@PDA. Subsequently, HNTs@PDA will be incorporated into Bru-CPCs to further improve their mechanical performance.
 |
| Fig. 1 (A) TGA curves of HNTs and HNTs@PDA. (B) Compressive strength of CPC scaffolds (incubated at 37 °C and 100% relative humidity for 2 days, **P < 0.01, ***P < 0.001). (C) Morphology of bone repair materials after mineralization. (D) Anti-washout property of the CPC scaffolds. Optical digital images of the CPC scaffolds after being injected into PBS solution and shaken at a speed of 60 rpm at 60 min. | |
Preparation procedures and thermogravimetric analysis and morphology of HNTs@PDA
The preparation process is shown in Scheme 1. Briefly, DA and HNTs were stirred in an alkaline environment and a PDA coating was formed on the surface of the HNTs by self-polymerization of DA to get HNTs@PDA. The morphological characters of HNTs and HNTs@PDA were revealed by TEM observations, which are shown in Fig. S3 (ESI†). The unmodified HNTs exhibit a cylindrical morphology with a distinct, empty central lumen. The HNT samples display an open-ended, hollow cylindrical structure with outer diameters ranging from 32–36 nm and inner diameters spanning 22–25 nm, respectively. The HNTs exhibit remarkably clear central channels, a testament to their double-layered nanotube architecture. Upon modification with dopamine, the HNTs@PDA display an enlarged outer diameter compared to the unmodified HNTs, indicative of a successful PDA coating on the exterior wall of the nanotubes.38 The HNTs@PDA exhibit a notable increase in wall thickness, and their outer surfaces appear roughened and coated with irregular materials, leading to an increase in the diameter of the HNTs significantly.39 The thickness of the HNTs@PDA layer (13–19 nm) is larger than that of the PDA layer (8–10 nm), indicating the successful coating of PDA modification.40
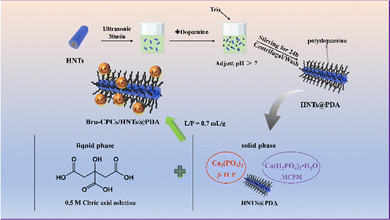 |
| Scheme 1 The preparation process of HNTs@PDA and the scaffolds. | |
The corresponding elemental maps displayed in Fig. S4 (ESI†) show the distribution of the constituent calcium element. The calcium mapping of the Ca-HNTs@PDA reveals an even distribution of the elemental signal along the entire length of the tube, indicating uniform enrichment of calcium ions. In contrast, the calcium mapping of the Ca-HNTs exhibits a random distribution pattern. The surface functionalization achieved through PDA coating enhances the accumulation of calcium ions within the material.32,41 The strong affinity between calcium ions and the catechol groups present in PDA suggests a significant interfacial interaction, which can contribute to the enhanced interface forces between HNTs@PDA and Bru-CPC.42
We used thermogravimetric analysis to analyze the grafting rate of PDA on HNTs (Fig. 1A). As shown in Fig. 1A, the weight decreased upon increasing the temperature from ambient temperature to 800 °C. The weight loss in the temperature range of room temperature to 405 °C was attributed to the adsorbed water and the chemically grafted silane existing in HNTs. The weight loss in the temperature range of 405 °C to 535 °C was attributed to the dihydroxylation of structural Al–OH groups of HNTs.43,44 The weight loss of the HNTs@PDA increases after coating with PDA. After 800 °C, the remaining weight was 85.0% and 81.6% for HNTs and HNTs@PDA, respectively, indicating that PDA has been successfully grafted onto HNTs. Moreover, a CCK-8 test was performed to investigate the cytocompatibility of the HNTs and HNTs@PDA by co-culturing MC3T3-E1 cells (Fig. S5, ESI†). The results showed that the number of cells in all groups increased over 24 hours, indicating that the HNTs and HNTs@PDA had good cell safety. After PDA functionalization, HNTs and HNTs@PDA were used as a solid additive to be incorporated into Bru-CPCs to obtain Bru-CPCs/1.5%HNTs and Bru-CPCs/1.5%HNTs@PDA (Scheme 1).
Compressive strength, fracture morphology, porosity, and anti-washout property
Compressive strength, fracture morphology, and porosity. The scaffolds were molded to obtain cylindrical forms (Scheme 1). The compressive strength and anti-washout ability of the CPCs, which were critical for actual clinical use, were systematically investigated. The compressive strengths of the pure Bru-CPCs, Bru-CPCs/1.5%HNTs and Bru-CPCs/1.5%HNTs@PDA scaffolds are shown in Fig. 1B and Fig. S1 (ESI†). The compressive strength of Bru-CPCs was 8.87 MPa after 2 days of immersion, which was consistent with the literature.14,45 As the content of HNTs increased to 1.5%, the value was increased to 12.16 MPa. The results showed that the addition of HNTs can improve the weak compressive strength of bone repair materials. A similar trend was observed for Bru-CPC/HNTs and Bru-CPC/HNTs@PDA as shown in Fig. S1 (ESI†). When adding 1.5 wt% HNTs@PDA, the compressive strength of Bru-CPCs/1.5%HNTs@PDA further increased to 18.14 MPa, which was about 2-fold higher than that of Bru-CPCs. The improvement of the compressive strength of bone repair materials may be attributed to the strong affinity between the catechol moieties of PDA and Ca2+ in Bru-CPCs. The fracture morphology of the scaffolds was characterized by SEM (Fig. 1C). The fracture morphology of the Bru-CPCs was irregular plate-like or flaky brushite crystals with micron to nanometer sizes. HNTs can act as crystal nuclei. Hence, with adding HNTs, the plate-like crystallization of Bru-CPCs/1.5%HNTs was more regular. After introducing HNTs@PDA, the crystals of Bru-CPCs/1.5%HNTs@PDA are more regularly stacked and the grain size becomes smaller. Some interfaces become blurred. These results may be beneficial to improving the mechanical properties. Fig. S6 (ESI†) exhibits the porosity of the CPCs. The porosity of Bru-CPCs was about 42.22 ± 0.75%. The porosities of Bru-CPCs/1.5%HNTs and Bru-CPCs/1.5%HNTs@PDA were 36.04 ± 0.67% and 38.31 ± 0.71%, respectively. The decrease in the porosity of the CPCs may lead to an increase in compressive strength.
Anti-washout property. The anti-washout property of the CPCs was determined by the appearance integrity after CPCs were injected into the PBS solution as given in Fig. 1D and Fig. S7 (ESI†). CPCs had good continuous shapes when injected into PBS solution. After 60 min of shaking cycles, Bru-CPCs/1.5%HNTs slightly disintegrated, while Bru-CPCs/1.5%HNTs@PDA showed more complete appearances. This might well be contributed by the chemical chelation of hydroxyl groups with Ca2+.46,47 The post-modification of the HNT surface with PDA modifies the interfacial interactions between inorganic HNTs and inorganic Bru-CPCs and enhances the anti-washout property of Bru-CPCs/1.5%HNTs@PDA.
Adhesive property, CCK-8 analysis, and live/dead staining analysis
Adhesive property. The adhesion and colonization of seed cells on the scaffolds are prerequisites for scaffold-dependent tissue engineering to promote bone regeneration.48 SEM images showed that the cells started to spread on the surface of Bru-CPCs, Bru-CPCs/1.5%HNTs and Bru-CPCs/1.5%HNTs@PDA. MC3T3-E1 cells on Bru-CPCs, Bru-CPCs/1.5%HNTs and Bru-CPCs/1.5%HNTs@PDA displayed by SEM observation appeared flat with intact, well-defined morphology and extending filopodia (Fig. 2A). This result indicated that all the scaffolds have good cytocompatibility.
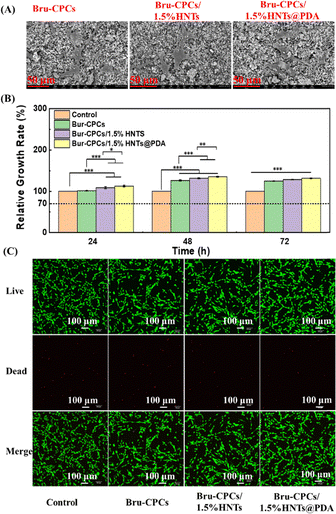 |
| Fig. 2 (A) SEM images of scaffolds co-cultured with cells for 24 h. (B) Cell viability of the scaffolds, in a CCK-8 test (*P < 0.05, **P < 0.01, ***P < 0.001). (C) Live/dead staining images of bone repair materials after co-culturing with cells for 24 hours. | |
CCK-8 analysis. CCK-8 analysis (Fig. 2B) and live/dead staining (Fig. 2C) were used to evaluate the proliferation of MC3T3-E1 cells on the scaffolds. As shown in Fig. 2B, the relative cell proliferation rates were all higher than 70% (the cell survival rate of the control group is defined as 100%), indicating that the cells proliferated well in each group. On the first day of cell culture, the relative cell proliferation rates in the Bru-CPCs, Bru-CPCs/1.5%HNTs, and Bru-CPCs/1.5%HNTs@PDA scaffolds were different, about 101%, 108%, and 112%, respectively. On the 3rd day, the relative cell proliferation rates in the Bru-CPCs, Bru-CPCs/1.5%HNTs, and Bru-CPCs/1.5%HNTs@PDA scaffolds were 124%, 128%, and 131%, respectively. The addition of HNTs@PDA increases the cell proliferation rate to a certain extent, which could be attributed to the comprehensive results of improved hydrophilicity, roughness, chemical composition, and morphology of the HNTs@PDA coating.49
Live/dead staining analysis. The results of the live/dead staining analysis further verified the results of CCK-8 analysis. As shown in Fig. 2C, the living MC3T3-E1 cells were stained green and displayed a normal shape. Compared with the control group and the pure Bru-CPCs scaffold, with the addition of HNTs and HNTs@PDA, the live cell (green fluorescence) density in the Bru-CPCs/1.5%HNTs scaffold and the Bru-CPCs/1.5%HNTs@PDA scaffold increased and few dead cells (red fluorescence) were observed. All the results demonstrated that the Bru-CPCs/1.5%HNTs@PDA scaffold promoted cell adhesion and proliferation and exhibited no adverse effects on cell viability and morphology.
ALP staining, ALP activity, and In vitro osteogenesis properties
ALP staining and ALP activity. Alkaline phosphatase (ALP) was an early osteogenic marker, which can reflect the differentiation trend and mineralization ability of osteoblasts.50 The osteogenic induction of the scaffolds was determined by ALP staining after 7 days of co-cultivation. The ALP expressions of all scaffolds and the control group were positive (Fig. 3A), indicating that all the scaffolds have the potential to promote early osteogenic differentiation. To more intuitively understand the differences in ALP activity of bone repair materials, ALP activity was detected (Fig. 3B). Compared with the control group, the ALP activities of Bru-CPCs, Bru-CPCs/1.5%HNTs, and Bru-CPCs/1.5%HNTs@PDA are all significantly increased.
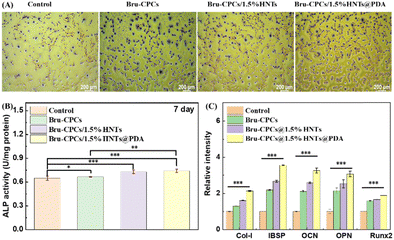 |
| Fig. 3 (A) ALP staining, (B) ALP activity and (C) osteogenesis-related gene expression test of the scaffolds (*P < 0.05, **P < 0.01, ***P < 0.001). | |
In vitro osteogenesis properties. To elucidate the moderating effect of HNTs and HNTs@PDA on osteogenic genes in MC3T3-E1 cells, we performed RT-qPCR for 5 related osteogenesic genes (Col-I, IBSP, OCN, OPN, and Runx2). Fig. 3C shows the expressions of Col-I, IBSP, OCN, OPN, and Runx2 of MC3T3-E1 after co-culturing with Bru-CPCs, Bru-CPCs/1.5%HNTs, and Bru-CPCs/1.5%HNTs@PDA. After culturing for 7 days, the different scaffolds upregulated the expression of 5 related osteogenesic genes to varying degrees. The cells in the group treated with Bru-CPCs/1.5%HNTs exhibited a higher expression of Col-I, IBSP, OCN, OPN and Runx2 than the control group and Bru-CPCs group in 7 days. Meanwhile, the expression levels of Col-I, IBSP, OCN and OPN in the Bru-CPCs/1.5%HNTs@PDA group had a significant difference with the other groups. Based on the higher expression levels of the four genes, it could be seen that the addition of HNTs@PDA into bone repair materials did not impair the osteoconductive function. Bru-CPCs/1.5%HNTs@PDA is conducive to the osteogenic differentiation and promotes bone repair.
Conclusions
In this study, HNTs were incorporated into Bru-CPCs as an additive to enhance the mechanical properties and osteogenic bioactivity of CPCs. The results indicated that the addition of HNTs led to an increase in the compressive strength of the CPCs. Additionally, the proliferation and in vitro osteogenic differentiation of MC3T3-E1 cells were also improved. The strong affinity between calcium ions and the catechol groups in PDA suggests significant interfacial interaction, which may contribute to the enhanced interface forces between HNTs@PDA and Bru-CPCBru-CPCs. The interfacial interactions between HNTs and Bru-CPCs are critical in further augmenting the compressive strength of the CPCs. Subsequently, the HNT surface was coated by a PDA layer and Bru-CPCs/1.5%HNTs@PDA was fabricated. The Bru-CPCs/1.5%HNTs@PDA scaffold exhibited superior compressive strength, cell proliferation, and osteogenic differentiation properties compared to both the Bru-CPCs and the Bru-CPCs/1.5%HNTs scaffolds. Based on these results, the newly designed Bru-CPCs/1.5%HNTs@PDA emerges as a promising candidate for bone-tissue repair applications. This work provides an efficient and universal strategy to design and construct high-performance personalized materials for bone-tissue regeneration. Further research will focus on in vivo studies and clinical evaluations, which are essential to ascertain the stability and regenerative potential of the fabricated scaffolds in the context of bone-tissue regeneration.
Data availability
Due to the confidentiality of data, the data including raw files used or analyzed during the current study are available from the corresponding authors on reasonable request.
Conflicts of interest
There are no conflicts to declare.
Acknowledgements
This study was supported by the National Natural Science Foundation of China (No. 22202055), the Natural Science Foundation of Hebei Province, China (No. C2022201038, H2022201017), the Foundation of Hebei Education Department (No. BJK2023004), Foundation of President of Hebei University (No. XZJJ202005), Medical Science Foundation of Hebei University (No. 2021B03), and the “Advanced Talents Incubation Program of the Hebei University”.
References
- J. Huang, A. C. Santos, Q. Tan, H. Bai, X. Hu, N. Mamidi and Z. Wu, J. Nanobiotechnol., 2022, 20, 522 CrossRef PubMed.
- N. Z. Laird, T. M. Acri, K. Tingle and A. K. Salem, Adv. Drug Delivery Rev., 2021, 174, 613–627 CrossRef CAS PubMed.
- L. Sun, H. Niu, Y. Wu, S. Dong, X. Li, B. Y. S. Kim, C. Liu, Y. Ma, W. Jiang and Y. Yuan, Bioact. Mater., 2024, 35, 208–227 CAS.
- X. He, Y. Li, D. Zou, H. Zu, W. Li and Y. Zheng, Bioact. Mater., 2024, 39, 456–478 CAS.
- L. Chen, J. Yang, Z. Cai, Y. Huang, P. Xiao, J. Wang, F. Wang, W. Huang, W. Cui and N. Hu, Adv. Funct. Mater., 2024, 34, 2314079 CrossRef CAS.
- J. D. Schwartzman, M. McCall, Y. Ghattas, A. S. Pugazhendhi, F. Wei, C. Ngo, J. Ruiz, S. Seal and M. J. Coathup, Biomaterials, 2024, 311, 122683 CrossRef CAS PubMed.
- M. Li, H. Wu, K. Gao, Y. Wang, J. Hu, Z. Guo, R. Hu, M. Zhang, X. Pang, M. Guo, Y. Liu, L. Zhao, W. He, S. Ding, W. Li and W. Cheng, Adv. Healthcare Mater., 2024, 13, 2402916 CrossRef CAS PubMed.
- A. Vezenkova and J. Locs, Bioact. Mater., 2022, 17, 109–124 CAS.
- H. H. K. Xu, P. Wang, L. Wang, C. Bao, Q. Chen, M. D. Weir, L. C. Chow, L. Zhao, X. Zhou and M. A. Reynolds, Bone Res., 2017, 5, 17056 CrossRef CAS PubMed.
- C. Cui, D. Liu, X. Xie, L. Wang, M. J. Lukic, X. Qiu, W. Chen, J. Shi, Y. Hong, B. Li, Z. Liu and S. Chen, Composites, Part B, 2024, 287, 111812 CrossRef CAS.
- W. Zhi, X. Wang, D. Sun, T. Chen, B. Yuan, X. Li, X. Chen, J. Wang, Z. Xie, X. Zhu, K. Zhang and X. Zhang, Bioact. Mater., 2022, 11, 240–253 CAS.
- Y. Feng, D. Wu, J. Knaus, S. Keßler, B. Ni, Z. Chen, J. Avaro, R. Xiong, H. Cölfen and Z. Wang, Adv. Healthcare Mater., 2023, 12, 2203411 CrossRef CAS PubMed.
- K. Hurle, J. M. Oliveira, R. L. Reis, S. Pina and F. Goetz-Neunhoeffer, Acta Biomater., 2021, 123, 51–71 CrossRef CAS PubMed.
- W. Gao, H. Wang, R. Liu, X. Ba, K. Deng and F. Liu, ACS Biomater. Sci. Eng., 2024, 10, 2062–2067 CrossRef CAS PubMed.
- L. Ding, H. Wang, J. Li, D. Liu, J. Bai, Z. Yuan, J. Yang, L. Bian, X. Zhao, B. Li and S. Chen, Biomater. Sci., 2023, 11, 96–107 RSC.
- G. Tripathi, M. Park, M. Hossain, S. B. Im and B. T. Lee, Int. J. Biol. Macromol., 2022, 221, 1536–1544 CrossRef CAS PubMed.
- N. Ribeiro, M. Reis, L. Figueiredo, A. Pimenta, L. F. Santos, A. C. Branco, A. P. Alves de Matos, M. Salema-Oom, A. Almeida, M. F. C. Pereira, R. Colaço and A. P. Serro, Ceram. Int., 2022, 48, 33361–33372 CrossRef CAS.
- P. A. Krokhicheva, M. A. Goldberg, A. S. Fomin, D. R. Khayrutdinova, O. S. Antonova, A. S. Baikin, A. A. Konovalov, A. V. Leonov, I. V. Mikheev, E. M. Merzlyak, V. A. Kirsanova, I. K. Sviridova, N. S. Sergeeva, S. M. Barinov and V. S. Komlev, Ceram. Int., 2023, 49, 19249–19264 CrossRef CAS.
- W. Liu, J. Zhang, P. Weiss, F. Tancret and J.-M. Bouler, Acta Biomater., 2013, 9, 5740–5750 CrossRef CAS PubMed.
- Y. Wan, H. Ma, Z. Ma, L. Tan and L. Miao, ACS Biomater. Sci. Eng., 2023, 9, 6084–6093 CrossRef CAS PubMed.
- G. H. Lee, P. Makkar, K. Paul and B. Lee, Mater. Sci. Eng. C, 2017, 77, 713–724 CrossRef CAS PubMed.
- M. Roozbahani and M. Kharaziha, Biomed. Mater., 2019, 14, 055008 CrossRef CAS PubMed.
- F. Kazemi-Aghdam, V. Jahed, M. Dehghan-Niri, F. Ganji and E. Vasheghani-Farahani, Carbohydr. Polym., 2021, 269, 118311 CrossRef CAS PubMed.
- L. Tong, Q. Liu, L. Xiong, P. Wang, M. Zhao, X. Li, J. Liang, Y. Fan, X. Zhang and Y. Sun, Sci. China Mater., 2024, 67, 2067–2079 CrossRef CAS.
- F. Doustdar, A. Olad and M. Ghorbani, Carbohydr. Polym., 2022, 282, 119127 CrossRef CAS PubMed.
- J. Liao, H. Wang, N. Liu and H. Yang, Adv. Colloid Interface Sci., 2023, 311, 102812 CrossRef CAS PubMed.
- E. Torres, V. Fombuena, A. Vallés-Lluch and T. Ellingham, Mater. Sci. Eng., C, 2017, 75, 418–424 CrossRef CAS PubMed.
- J. Varshosaz, Z. S. Sajadi-Javan, M. Kouhi and M. Mirian, Int. J. Biol. Macromol., 2021, 192, 869–882 CrossRef CAS PubMed.
- J. Zheng, F. Wu, H. Li and M. Liu, Mater. Sci. Eng., C, 2019, 105, 110072 CrossRef CAS PubMed.
- K. Huang, Q. Ou, Y. Xie, X. Chen, Y. Fang, C. Huang, Y. Wang, Z. Gu and J. Wu, ACS Biomater. Sci. Eng., 2019, 5, 4037–4047 CrossRef CAS PubMed.
- D. M. Reffitt, N. Ogston, R. Jugdaohsingh, H. F. J. Cheung, B. A. J. Evans, R. P. H. Thompson, J. J. Powell and G. N. Hampson, Bone, 2003, 32, 127–135 CrossRef CAS PubMed.
- R. Wang, X. Zha, J. Chen, R. Fu, Y. Fu, J. Xiang, W. Yang and L. Zhao, Adv. Healthcare Mater., 2024, 13, 2304232 CrossRef CAS PubMed.
- M. Mousa, N. D. Evans, R. O. C. Oreffo and J. I. Dawson, Biomaterials, 2018, 159, 204–214 CrossRef CAS PubMed.
- L. Ji, W. Qiao, Y. Zhang, H. Wu, S. Miao, Z. Cheng, Q. Gong, J. Liang and A. Zhu, Mater. Sci. Eng., C, 2017, 78, 362–369 CrossRef CAS PubMed.
- Y. Zhou, X. Gao, M. Zhao, L. Li and M. Liu, Compos. Sci. Technol., 2024, 250, 110537 CrossRef CAS.
- Q. Ou, K. Huang, C. Fu, C. Huang, Y. Fang, Z. Gu, J. Wu and Y. Wang, Chem. Eng. J., 2020, 382, 123019 CrossRef CAS.
- J. Wang, Y. Cui, B. Zhang, S. Sun, H. Xu, M. Yao, D. Wu and Y. Wang, Mater. Des., 2024, 238, 112655 CrossRef CAS.
- S. Choi, S. Chaudhari, H. Shin, K. Cho, D. Lee, M. Shon, S. Nam and Y. Park, J. Ind. Eng. Chem., 2022, 105, 158–170 CrossRef CAS.
- D. Gnanasekaran, A. Shanavas, W. W. Focke and R. Sadiku, RSC Adv., 2015, 5, 11272–11283 RSC.
- C. Chao, J. Liu, J. Wang, Y. Zhang, B. Zhang, Y. Zhang, X. Xiang and R. Chen, ACS Appl. Mater. Interfaces, 2013, 5, 10559–10564 CrossRef CAS PubMed.
- S. Kim and C. B. Park, Biomaterials, 2010, 31, 6628–6634 CrossRef CAS PubMed.
- N. Holten-Andersen, T. E. Mates, M. S. Toprak, G. D. Stucky, F. W. Zok and J. H. Waite, Langmuir, 2009, 25, 3323–3326 CrossRef CAS PubMed.
- Z. Wang, H. Wang, J. Liu and Y. Zhang, Desalination, 2014, 344, 313–320 CrossRef CAS.
- Y. Zhang, R. Meng, J. Zhou, X. Liu and W. Guo, Colloids Surf., A, 2022, 648, 129378 CrossRef CAS.
- H.-J. Lee, B. Kim, A. R. Padalhin and B.-T. Lee, Mater. Sci. Eng., C, 2019, 94, 385–392 CrossRef CAS PubMed.
- H. Shi, X. Ye, J. Zhang and J. Ye, ACS Biomater. Sci. Eng., 2019, 5, 262–271 CrossRef CAS PubMed.
- L. Ding, H. Wang, W. Zhang, J. Li, D. Liu, F. Han, S. Chen and B. Li, J. Mater. Chem. B, 2021, 9, 6802–6810 RSC.
- B. G. Sengers, M. Taylor, C. P. Please and R. O. C. Oreffo, Biomaterials, 2007, 28, 1926–1940 CrossRef CAS PubMed.
- Y. Yu, X. Li, J. Li, D. Li, Q. Wang and W. Teng, Mater. Sci. Eng., C, 2021, 131, 112473 CrossRef CAS PubMed.
- Y. Wang, C. J. E. Davey, K. van der Maas, R.-J. van Putten, A. Tietema, J. R. Parsons and G.-J. M. Gruter, Sci. Total Environ., 2022, 815, 152781 CrossRef CAS PubMed.
|
This journal is © The Royal Society of Chemistry 2025 |
Click here to see how this site uses Cookies. View our privacy policy here.