DOI:
10.1039/D4QI02653E
(Review Article)
Inorg. Chem. Front., 2025,
12, 11-38
Naphthalene diimide-based crystalline hybrid photochromic materials: structural types, photochromic mechanism, and applications
Received
21st October 2024
, Accepted 6th November 2024
First published on 6th November 2024
Abstract
Multifunctional stimuli-responsive photochromic materials have significantly expanded their application fields, including molecular switching, information storage and encryption, detection and sensing, etc. 1,4,5,8-Naphthalenediimides (NDIs) have been widely used for the construction of photochromic materials because of their excellent modification potential and high redox activity. As single organic NDI materials exhibit poor fatigue resistance and relatively simple functions, increasing interest in this field focuses on constructing multifunctional crystalline hybrid photochromic materials and exploring applications based on the electron-deficient features of NDIs. Nevertheless, the development of multifunctional naphthalene diimide-based crystalline hybrid photochromic materials (NCHPMs) remains a significant challenge. This paper summarizes structure design strategies for NCHPMs with different NDI ligands, the photochromic mechanism, applications, and future prospects. This article will provide a valuable reference for researchers studying NCHPMs.
1. Introduction
Multifunctional photochromic material, as an important branch of smart stimuli-responsive materials, has greatly expanded its application field and demonstrated promising prospects in information storage, ultraviolet detection, and sensors.1–11 Compared with pure organic or inorganic photochromic materials, inorganic–organic hybrid photochromic materials can not only maintain or enhance the characteristics of each component, but also expand their applicability in various fields, such as optical memory, photomagnetism, and multiple anticounterfeiting uses.12–20 The properties of multifunctional photochromic materials are not only determined by the molecular structure, but also by the packing mode and intermolecular interaction forces. Therefore, it is essential to accurately identify the structure and type of material. Research has demonstrated that these materials can be effectively synthesized through self-assembly to produce crystalline material, and a comprehensive analysis of the crystal structure can reveal the photochromic mechanisms and potential applications.21–24 However, self-assembly is an uncontrollable process, and the structure of the prepared compounds bears uncertainty, which is influenced by many factors including temperature, pH, solvents, etc. Moreover, it is difficult to precisely control the properties of photochromic materials. Hence, the construction of intelligent multifunctional photochromic materials remains a huge and significant challenge. At present, the production of multifunctional photochromic materials can be achieved by designing organic ligands with specific structures and functions, optimizing inorganic components, and combining different construction strategies with theoretical calculations.
Generally, the construction of multifunctional stimuli-responsive chromic materials requires appropriate insertion of stimuli-responsive units. 1,4,5,8-Naphthalenediimides (NDIs) as chromic materials have received extensive attention because of their large conjugate plane, good redox properties, good electron affinity and carrier mobility.25–29 However, individual organic NDI materials demonstrate poor fatigue resistance and relatively simple functions. The increasing interest in this field is focused on achieving the design of diverse naphthalene diimide-based crystalline hybrid photochromic materials (NCHPMs).30–32 Currently, the reported NCHPMs can be categorized based on their stacking mode: firstly, utilizing NDIs for bridging and metal coordination to form coordination polymers; and secondly, involving non-covalent interaction forces in the formation of supramolecular polymers during the stacking process. The photoinduced electron transfer mechanism is widely employed to elucidate the phenomenon of photochromism in NCHPMs. The electron transfer pathway primarily involves intermolecular interaction forces, such as π–π interaction, lone pair–π interaction force, anion–π interaction, and intermolecular hydrogen bonding. The bond length, bond angle, as well as the electron donor ability and interface relationship are crucial factors influencing the photochromic performance of NCHPMs.33–36 Despite significant progress in the research of NCHPMs, effectively regulating the interface relationship between electron donors (EDs) and electron acceptors (EAs) to control electron transfer remains a critical issue.
Thus far, for NCHPMs, no comprehensive review on the various types of structures, photochromic mechanism and their applications in this field has been reported yet. The review is divided into four main parts. The first part introduces the basic properties of NDIs and the discoloration mechanism of NDIs and some other photochromic materials. The second part summarizes the “structure–function” relationship of different types of NCHPMs by classifying different structural NDIs (Fig. 1). The third part is the application area of multifunctional NCHPMs. Finally, some personal views on the development prospects and problems faced by NCHPMs are put forward (Fig. 2). It is hoped that this paper can provide valuable guidance for researchers studying crystalline hybrid photochromic materials and promote further research.
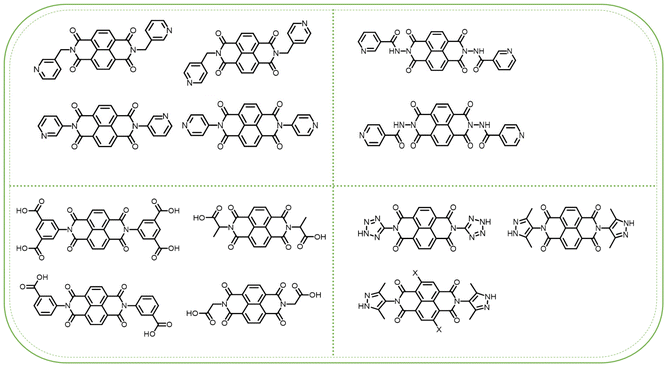 |
| Fig. 1 Organic units of NDIs with different structural types. | |
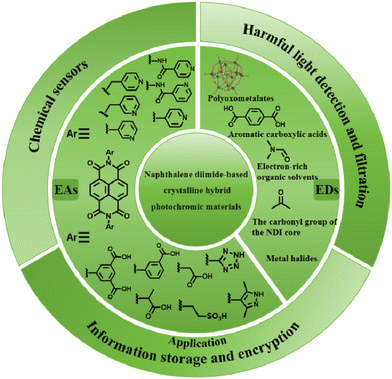 |
| Fig. 2 Schematic diagram of the structure types and applications of NCHPMs. | |
2. Basic properties of NDIs and photochromic mechanism
2.1 Basic properties of NDIs
NDIs are aromatic compounds with a planar rigid structure, electron deficiency characteristics, good thermal stability, and high crystallinity.37–39 NDI derivatives are typically prepared in two ways: one involves the functionalization of imide nitrogen, which is easily synthesized and has minimal impact on the optical and chemical properties of NDIs, only altering the solubility and molecular packing pattern.40,41 The other method involves the preparation of NDI derivatives by naphthalene core substitution (cNDIs), resulting in NDI derivatives with tunable absorption and fluorescence properties. The modified cNDIs exhibit a high fluorescence quantum yield (Φ ≈ ∼0.9) in organic solvents, and the uniformity of the yield is noteworthy.42–44 The presence of multiple modification sites on NDI contributes to its diverse species. NDIs are well suited for applications in light-responsive materials and electron transport devices due to their high electron affinity, low LUMO energy levels (ELUMO = −3.7 eV), and excellent reversibly reducible properties (ERed11/2 = −1.10 V and ERed21/2 = −1.51, vs. Fc/Fc+ in CH2Cl2).41,45,46
2.2 Photochromic mechanism
For a promising intelligent material, the photochromic mechanism must be investigated in depth. Currently, there are three primary types of photochromic reaction mechanisms that can be explained. The first involves visual discoloration caused by structural changes in response to light stimulation, such as the C–H chain cleavage of spiropyrans upon exposure to UV light.47–49 Azobenzene compounds undergo cis–trans isomerization reactions under photoexcitation.50–52 Dithiophene ethylene compounds undergo pericyclization reaction under photoinduction (Fig. 3a–c).53–56 Secondly, viologen and NDIs generate photoinduced electron transfer (PET) between EDs and EAs under photoexcitation, producing colored radicals (Fig. 3d and e).57–61 Thirdly, the visual discoloration is caused by the change of the valence state of metal ions, which mainly include polyoxometalates (POMs), transition metal oxides, metal halides, etc. (Fig. 3f).62–64 The regulation of photochromic properties and the study of the photochromic mechanism of NCHPMs are examined as an illustration. The electron-donor ability of EDs and the electron-accepting ability of EAs, as well as the electron transfer (ET) pathway, should be considered first in the construction of NCHPMs. Based on the unit of EDs and EAs, the ET pathways of NCHPMs are ultimately classified as ligand-to-ligand ET (LLET).4,65,66 ET usually occurs between ligand and ligand in the donor–acceptor (D–A) system when a specific direction and distance is satisfied. When NCHPMs are stimulated under light, electrons will transfer from EDs to EAs, forming a charge separation state. It is worth noting that the electron transfer in the D–A system is limited by the HOMO and LUMO orbital energy levels of EDs and EAs.67,68 In general, electron transfer occurs through non-covalent interactions such as π–π interactions and hydrogen bonding (Fig. 4a and b). When the electron-rich groups are closer to the NDI core, lone pair–π interactions and CH–π interactions will occur (Fig. 4c and d). Appropriate interface matching relationships will facilitate the generation of non-covalent interaction forces, thereby leading to the effective ET and photoresponsive behavior.69–71 In 2004, Andric mentioned that the π–π stacking and charge transfer between the electron-rich group and the colorless NDI ligand resulted in a colored D–A system.72 Furthermore, Saha's research group experimentally and theoretically demonstrated that lone pair–π interaction can facilitate electron transfer.73,74 It has been found that the distance between D–A and the amount of non-covalent interaction forces also significantly impact the photochromic performance of NCHPMs. Therefore, rational allocation of the interface relationship in the D–A system plays a crucial role in the development of NCHPMs.
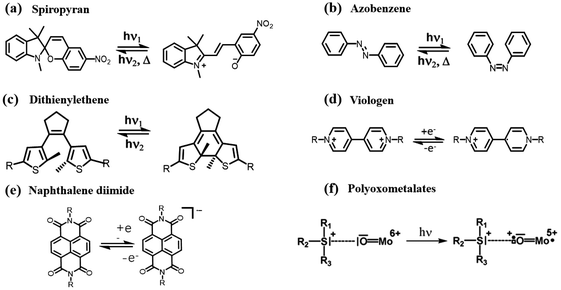 |
| Fig. 3 Common types of photochromic mechanisms. | |
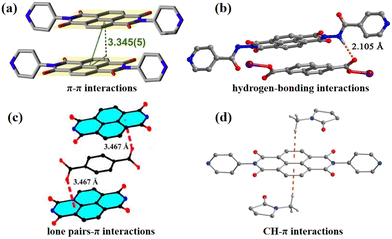 |
| Fig. 4 Some common non-covalent interaction forces in D–A systems (cited from ref. 75–78). | |
3. Structure types of NCHPMs
The construction of NDI units is crucial for the synthesis of NCHPMs, with functional design at the N position leading to structurally diverse NCHPMs that significantly impact interfacial contact between EDs and EAs. This paper introduces and summarizes assembly strategies, molecular structures, photochromic performance, and electron transfer pathways of NCHPMs by categorizing different NDI substituents in reported NCHPMs. NDIs can serve as both photoactive units and ligands in the construction of NCHPMs, offering a wide range of possibilities due to their diverse design and large π-conjugate planes. Typically, NCHPMs are synthesized using N-position-substituted NDIs with main substituents such as pyridine, pyridamide, carboxyl group, five-membered nitrogenous heterocyclic groups, and sulfonic acid groups. The following report details the construction of NCHPMs utilizing these types of NDIs, along with research findings on NDI homologs in photochromic materials.
3.1 NCHPMs based on pyridine-modified NDIs
Pyridine-based NDIs remain widely utilized in the synthesis of multifunctional photochromic materials due to the ability of N atoms’ lone pairs to form stable chemical bonds with metal ions, leading to diverse crystal packing patterns that enhance the photoinduced electron transfer (PET) mechanism and functionality of NCHPMs. From the perspective of spatial structure, the pyridine group has a smaller spatial occupancy in the coordination process, which makes NCHPMs have a tighter stacked structure and stronger non-covalent interactions force. Different N sites in the pyridyl group play an important role in regulating the size and structure of NCHPMs. Liu and Fu et al. have made great contributions to the synthesis and application of such NCHPMs.79–88
Coordination polymers (CPs), as a novel class of ET material, are widely used in the fabrication of photochromic materials. The incorporation of inorganic components not only enhances the durability and functionality of the material but also allows for the generation of diverse structures. By manipulating the structure of CPs and adjusting the strength of intermolecular interaction forces, it is possible to regulate the photochromic properties of the material.89,90 For example, in 2015, Lin et al. synthesized three 1D network coordination polymers with the same structure using three halogen atoms for encapsulation, [(DPNDI)ZnX2] (DPNDI = N,N′-di(4-pyridyl)-1,4,5,8-naphthalene diimide, X = Cl for 1, X = Br for 2 and X = I for 3).83 Due to the different intensities of lone pair–π interactions between halogen atoms and NDIs, only compounds 1 and 2 exhibited photochromism, while compound 3, with strong lone pair–π interactions, reduced the electron-withdrawing ability of DPNDI and thus could not achieve discoloration. This study offers a pathway for regulating NCHPMs by manipulating intermolecular interactions. The counter anions play a crucial role in determining the ultimate structure of the coordination polymer, thereby influencing the photochromic properties of the NCHPMs.91,92 In 2018, Liu's group reported the synthesis of three coordination polymers using DPNDI as an organic ligand and involving three different anions: [Cd(NO3)2(DPNDI) (CH3OH)]·CH3OH (4), [Cd(SCN)2(DPNDI)] (5), and [Cd(DPNDI)2 (DMF)2]·2ClO4 (6).84 In compound 4, NO3− participated in coordination, forming a one-dimensional network structure. Compound 5 featured SCN− anions participating in coordination and bridging to connect one-dimensional chains formed by Cd2+ and DPNDI into a two-dimensional network structure. 6 contained freely existing ClO4− within a stable 3D framework due to C–H⋯anion interactions. From a structural perspective, among the three anions, ClO4− exhibited poor coordination ability in these polymers and only existed in its free form. In terms of photochromic properties, compounds 4 and 6 underwent a color change from yellow to brown or dark brown under UV-vis light but faded in the dark over time; however, compound 5 showed photoinertness. This was attributed to the S atom in SCN− remotely supplying electrons to the electron-deficient NDI core of DPNDI, leading to decreased electron acceptance capacity which could cause energy level mismatches affecting the photosensitivity of the compounds.
MOFs, as a class of CPs, are known for their high stability and porosity. Functional versatility can be achieved by strategically designing and assembling MOF-type photochromic materials.93–95 The porous nature of MOFs allows electron-rich solvents to participate in the photochromic process as electron donors when such donors are absent within the MOFs. In 2021, Fu's group successfully synthesized a novel organic framework material {[Zn(3-DPMNI)0.5(NDC)]} (7, DPMNI = N,N′-bis(3-pyridylmethyl)-1,4,5,8-naphthalene diimide, H2NDC = 2,6-naphthalenedi-carboxylic acid), in which Zn2+ coordinated with four carboxyl groups to form a paddle-like [Zn2(COO)4] unit.85 The adjacent units were linked by NDC2+, resulting in a diamond-shaped layer, and ultimately connected by DPMNI to construct a column-layer structure (Fig. 5a and b). However, the photochromism of 7 was hindered by the absence of electron transfer pathways. Interestingly, compound 7 exhibited a color change after being immersed in DMF for three hours. Structural analysis revealed that the transition from crystal 7 to crystal 7@DMF ({[Zn(3-DPMNI)0.5(NDC)]·3DMF}) was attributed to the intervention of DMF molecules. The resulting 7@DMF displayed favorable color-changing behavior, which could be attributed to lone pair–π interactions between the O in DMF and NDI cores (Fig. 5c and d). Furthermore, when immersed in electron-rich solvents (DMA, DMSO, NMP), compound 7 demonstrated excellent detection capabilities for some electron-rich systems (Fig. 5e and f). Additionally, this NDI-based functionalized material also showed rapid response to small molecule amines. Overall, MOF materials are widely utilized for loading and adsorption of substances due to their exceptional thermal stability and pore structure.
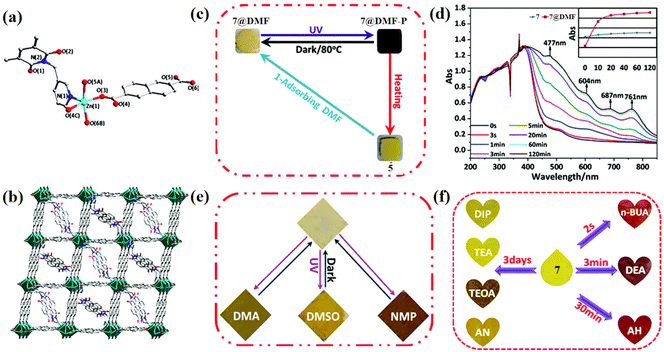 |
| Fig. 5 (a) Asymmetrical elements in the 7@DMF. (b) Three-dimensional frame structure of the 7@DMF. (c) Photochromic images of 7@DMF. (d) UV-vis patterns of 7@DMF as a function of illumination time. (e) Photochromic image of 7 after immersion in electron-rich solvent. (f) 7 responses to different amines. | |
By manipulating the interface relationship of the donor–acceptor system, different secondary linkers can alter the photochromic properties of MOFs.96,97 For example, in 2022, Liu et al. reported the construction of two 3-DPNDI-MOF materials using different auxiliary ligands: [Zn2(DPNDI)(2,6-NDC)2] (8) and [Zn2(DPNDI)(1,4-NDC)2] (9) (1,4-H2NDC = 1,4-naphthalenedicarboxylic acid, 2,6-H2NDC = 2,6-naphthalened-icarboxylic acid).86 In 8, Zn2+ was coordinated with four 2,6-NDC2− to form a paddle wheel [Zn2(COO)4] structural unit which further connected with 2,6-NDC2− to form a square two-dimensional layer. A three-dimensional porous structure was formed with DPNDI serving as a bridge between the layers. Compound 9 exhibited the same coordination pattern as 8 but with the position of the ligand replaced by 1,4-NDC instead of 2,6-NDC. Despite their structural similarities, compounds 8 and 9 exhibited different photosensitivity; while compound 8 did not change color under UV-vis irradiation and its spectrum remained unchanged before and after irradiation, compound 9 changed from yellow to brown under irradiation and new absorption peaks were generated at wavelengths of 520 and 645 nm due to the production of NDI free radicals, as evidenced by ESR profiles. This difference in photochromic performance can be attributed to the C–H⋯π interactions (8: 3.887 Å, 9: 3.272 Å).
The construction of NCHPMs can be accomplished through supramolecular polymerization, which manipulates molecules within the system using non-covalent interactions to create complex and ordered D–A systems.98,99 POMs, as an inorganic component with high redox activity and photoactivity, are utilized in the fabrication of various photochromic materials. The hybridization of POMs and NDIs can not only improve the stability and photochromic properties of compounds, but also provide more options for NCHPM applications.100,101 In 2015, Lu et al. reported two rare supramolecular photochromic materials featuring two distinct rigid NDIs as the host and polyoxometalate as the guest: [Cu2(DPNDI)2(H2O)7 (OH)·(PW12O40)]n (10) and [Cu(DPMNI)(C4H9NO)2 (CH3OH)·(HPW12O40)]n (11).87 In 10, Cu2+ was coordinated with rigid DPNDI to form a one-dimensional chain-like structure, while anions ([PW12O40]3−) acted as guest molecules passing through C–H⋯anion interactions and anion–π interactions were surrounded by four alternating horizontal and vertical chains, resulting in a 3D supramolecular network. In 11, the semi-rigid DPMNI was chosen as the primary component to coordinate with Cu2+ in order to generate a one-dimensional wave-shaped structure. The POM was flanked by two cis-DPMNI through C–H⋯O, C–H⋯anion interactions and anion–π interactions, resulting in the formation of a stable 3D supramolecular structure. Both compounds 10 and 11 displayed photochromic behavior under xenon lamp irradiation, with compound 10 exhibiting a faster light response. This could be attributed to the transformation of the NDI ligand from rigid to semi-rigid, leading to distinct modes of anion–π interactions. In 2021, Liu's group synthesized two POM-based NCHPM materials, [Cu3(DPMNI)2(PMo12O40)(DMA)12(H2O)2] (12), [Zn3(DPMNI)2 (PMo12O40)2(DMA)12(H2O)2] (13),88 both featuring similar structures. The metal cations were coordinated with DPMNI to form cationic one-dimensional chains, which were further arranged in a three-dimensional supramolecular structure with [PMo12O40]3− through anion–π interactions (2.990–3.201 Å) (Fig. 6a). Under Xe lamp irradiation both 12 and 13 exhibited remarkable color-changing properties (12 from blue to dark blue, 13 from light yellow to dark green) (Fig. 6b). Due to the synergistic effect of POM and NDI, they also demonstrated efficient photocatalytic degradation performance against the oncogenic dye BR9 in the presence of H2O2 (achieving a degradation rate of over 86% within two hours) (Fig. 6c and d).
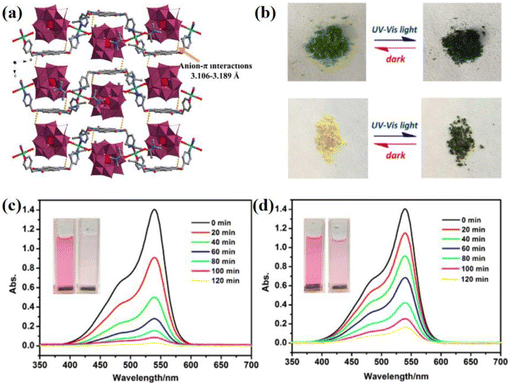 |
| Fig. 6 (a) Anion–π interactions in compound 12. (b) Photochromic images of compounds 12 and 13. (c) (d) UV-vis absorption spectra of BR9 after illumination in the presence of compounds 12 and 13. | |
Due to the strong coordination ability and electron deficiency of pyridyl NDIs, they have demonstrated remarkable efficacy in the construction of CPs and supramolecular polymers. By synergizing with various types of EDs, such as halogen atoms, anions, aromatic carboxylic acids, and POMs, they not only exhibit diverse structural assembly but also tunable photochromic properties. Summarizing these NCHPMs reveals that the photosensitivity of the compounds is influenced by non-covalent interactions in the D–A system and the electron-accepting capability of NDIs. It is worth noting that when the electron-donating ability of EDs is too strong, it may weaken the electron-deficient properties of NDIs, resulting in a decrease in their photochromic performance.
3.2 NCHPMs based on pyridinamide-modified NDIs
In order to prepare NCHPMs with superior performance, it is essential to consider the energy level matching and relative position matching between EDs and EAs. The presence of a carbonyl group in the pyridinamide group enhances the electron-deficient characteristics and coordination ability of NDIs, thereby expanding the non-covalent interaction types and coordination modes of NCHPMs. Through the synergistic effect of inorganic and organic compounds, it is possible to construct multifunctional NCHPMs; Zhang and Zheng et al. have achieved phased results.76,77,102–104 For example, in 2021, Zhang's research group successfully synthesized a novel Cd-MOF: [Cd(NDI-A)(ClO4)·H2O]n (14, NDI-A = N,N′-di(4-pyridylacylamino)-1,4,5,8-naphthalene diimide).102 The coordination of Cd2+ and ClO4− anions formed a wave-like chain structure, with NDI-A linking adjacent chains to create a palisade-like 2D framework. A three-dimensional supramolecular network was established through the interaction forces between ligands. Compound 14 demonstrated excellent photochromism and hydrochromism. Analysis revealed electron transfer from donor carbonyl oxygen to the acceptor via intermolecular lone pair–π interactions (3.355 Å). Furthermore, heating-induced loss of coordination water in the structure led to deepening of color in 14. Distinct luminescence properties between 14 and NDI suggested that changes in coordination mode influenced ligand fluorescence activity. Additionally, compound 14 exhibited discoloration properties when exposed to ammonia vapor. This study presents a new approach for designing and synthesizing functional MOF materials.
The utilization of auxiliary ligands with varying conjugations, shapes, and dimensions will modify the arrangement of NCHPMs, thus controlling the PET interface and modifying photochromic properties.105 The research conducted by the Fu and Xing groups has made significant contributions in this field, demonstrating that the structure and level of conjugation of EAs have a profound impact on NCHPMs. For example, in 2022, Fu's research group reported two new coordination polymers [Cd(NicNDI)(4,4′-SBC)] (15, 4,4′-SBC = 4,4′-stilbene dicarboxylic acid) and [Cd(NicNDI)(2,2′-BPC)] (16, 2,2′-BPC = 2,2′-biphenyl dicarboxylic acid), utilizing NicNDI (NicNDI = (3-pyridylacylam-ino)-1,4.5.8-naphthalene diimide) as the template and aromatic carboxylic acids as the connecting ligands.103 The photochromic behavior of the two complexes was significantly different, despite only differing in their auxiliary ligands. Complex 15 rapidly changed color from orange to brown under Hg lamp (300 W, 365 nm), while complex 16 only exhibited a slight deepening after irradiation. It was observed that complexes 15 and 16 possessed the same electron-accepting capability, but differed in their electron-donating ability due to the use of different auxiliary ligands. The utilization of distinct carboxylic acid ligands resulted in the formation of numerous interpenetrating structures, with 15 exhibiting four times more interpenetration than 16. This directly led to varying interface contacts between electron donors and acceptors within the system. In 2022, Xing's group synthesized nine cadmium-centered MOFs using NDI-A and seven different aliphatic dicarboxylic acids.104 Complexes 17–25 exhibited distinct structures due to the varying carboxylic acid ligands with differing chain lengths. Complex 17 exhibited a three-dimensional network structure, as depicted in Fig. 7, while complexes 18–25 formed a supramolecular network structure through hydrogen bonding. Cadmium adopted a hexacoordination mode in complexes 17, 18, 19 and 23, and a heptacoordination in complexes 20, 21, 22 and 24. Nine compounds were sensitive to amine vapor. It is important to note that the time of discoloration and the depth of color varied among these nine complexes, which could be attributed to the distance of the lone pair–π interaction and the number of N–H⋯O hydrogen bonds in each complex. Significantly, complexes 17–25 exhibited the ability to detect TNP through fluorescence quenching in ethanol solution, thereby enabling the development of a range of fluorescent sensors for TNP (Fig. 8). This research introduced a novel approach for the utilization of NDI-MOFs materials.
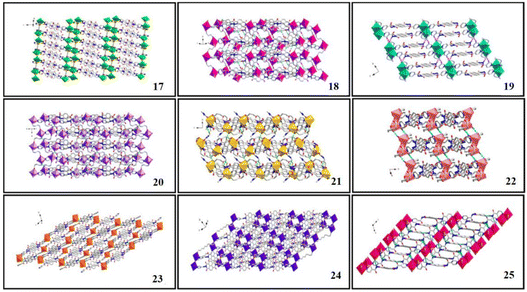 |
| Fig. 7 Network structure of compounds 17–25. | |
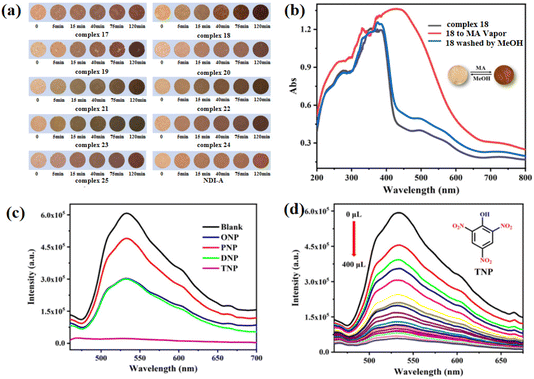 |
| Fig. 8 (a) Photochromic picture of complexes 17–25 and NDI ligand. (b) Vapor discoloration picture in MA and UV-vis pattern of 18. (c) The fluorescence intensity change of 18 to different nitrophenol compounds. (d) Fluorescence quenches with increasing TNP content in 18. | |
By changing the solvent ratio in the reaction system, the photochromic properties can be controlled. In 2022, Zheng et al. reported two Cd-based coordination polymers, [Cd0.5(NicNDI)(HBDC)]·H2O (26) and {[Cd(NicNDI)0.5 (BDC)]·MeCN}n (27, H2BDC = terephthalic acid).76 It was just a matter of the solvent molecules, but 26 and 27 showed obvious structural differences; 26 is a one-dimensional mononuclear structure, and 27 was a 3D network structure. 26 did not achieve photoinducibility, but under X-ray, visible light, and UV light 27 showed a light response (from yellow to black) (Fig. 9). This was due to the effect of the coplanarity of the NDI on ET (26 was non-coplanar, 27 was coplanar). Different solvent ratios could manipulate the conformation of NDI molecules, and this behavior enabled visual control of 27's discoloration rate and color. This was achieved by a change in lone pair–π interactions. Due to the electron deficiency characteristics, the host and guest could interact with amine compounds, and the detection could be achieved by color change (Fig. 10). This work revealed that the photochromic properties of NCHPMs can be modulated by manipulating molecular conformation.
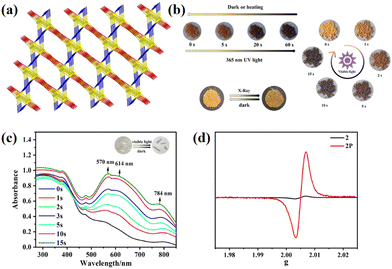 |
| Fig. 9 (a) 3D frame structure of compound 27. (b) Photochromism of 27 under UV light, X-ray, and visible light. (c) UV-vis absorption spectra of 27. (d) EPR spectra of 27 before and after irradiation. | |
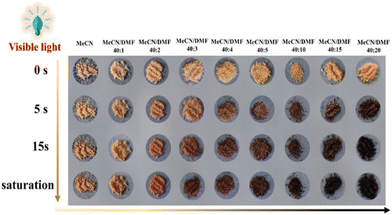 |
| Fig. 10 Compound 27 synthesized with different solvent ratios and photochromic properties. | |
In summary, the presence of asymmetric unit pyridinamide in NDIs may lead to distortion of their planar structure during coordination, impacting electron transfer efficiency and light response rate. The oxygen atom on pyridinamide can participate in photochromism through lone pair–π interactions. NCHPMs undergo structural changes and exhibit different weak intermolecular interactions when using various aromatic carboxylic acids, directly affecting their photochromic properties. Additionally, the conjugation degree of molecules in the D–A system is positively correlated with the color-changing properties of NCHPMs. Solvent ratio influences the molecular conformation of NDI ligands during NCHPM construction, allowing for regulation of color development and photochromism rate.
3.3 NCHPMs based on carboxylic acid-modified NDIs
Carboxylic acid-modified NDIs are crucial in the preparation of NCHPMs, as carboxylic acids exhibit flexible coordination modes that can be partially or completely deprotonated to greatly enhance geometric coordination configuration. The strong coordination ability of carboxylic acid ligands also contributes to the thermal stability and multifunctionality of NCHPMs. Additionally, the carboxyl group serves as a donor or acceptor for hydrogen bonding, complementing the rigid plane and carbonyl characteristics of NDI cores to facilitate the construction of supramolecular NCHPMs. These features significantly expand the construction mode and functionality of NCHPMs. Recently, the research groups of Kang, Xing, Kwak, Zhang and Fu et al. have made significant contributions to the in-depth investigation of NCHPMs utilizing carboxylic acid-modified NDIs.106–115 For example, in 2022, Kang et al. reported an NDI-based MOF, Nd2(BINDI)(NO3)(BA)(DMF)4 (28, H4BINDI = N,N′-bis(5-isophthalic acid)-1,4,5,8-naphthalenediimide, BA = benzoate).106 Under X-ray irradiation it changed from light yellow to black, and it was more sensitive (color changes within 7.2 s). There was a slight change in structure during color change (volume shrinkage from 6259.9 to 6083.8 Å3), mainly due to the formation of free radical anions that led to structural transitions, which were facilitated by the rotatable behavior of the auxiliary ligands (Fig. 11a). As the reaction temperature increased during synthesis for 28, the crystal color darkened, which was caused by the heat-induced generation of free radical anions (Fig. 11b). Since the absorption band of 28 (150-28) synthesized at 150 °C extended into the near-infrared region, 150-28 exhibited excellent photothermal conversion efficiency (31.37%) under IR irradiation (Fig. 11c). This marriage provides a new research idea for the preparation of new multi-stimulus-responsive functional materials. In addition, rare earth ions have the potential to introduce new properties into NDI-modified photochromic materials, such as fluorescence-responsive behaviors including turn-off fluorescence, turn-on fluorescence, and ratiometric fluorescence. The design and preparation of turn-on MOF fluorescent multifunctional sensors is a valuable pursuit. For example, in 2023, Xing's group discovered two NDI-modified lanthanide photochromic materials [Eu2(BINDI)(NO3)2(DMA)4]·2DMA (29) and [Sm2(BINDI)(NO3)2(DMA)4]·2DMA (30).107 Fluorescence detection in different solvents revealed that compound 29 displayed strong fluorescence and good stability in ethanol, making it suitable for fluorescence chemical sensing applications. Compound 29 was capable of detecting aromatic amines (OPD) and fatty amines (n-BA), each with varying fluorescence intensities, and test strips for amine vapor detection have been prepared. Additionally, by measuring the fluorescence intensity of certain pesticide components in an ethanol suspension prepared using compound 29, it was found that only thiamendazim (TBZ) affected its fluorescence intensity. This study contributes significantly to public health and safety by introducing new possibilities for developing NCHPM sensors with exceptional recognition capabilities (Fig. 12).
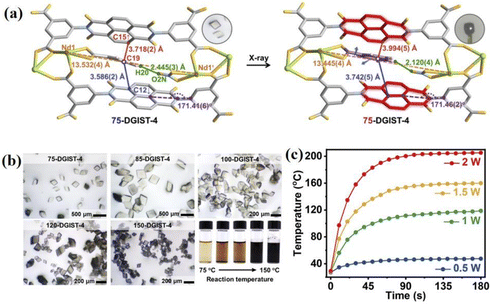 |
| Fig. 11 (a) Structural transformation and photochromism of compound 28 under X-ray irradiation. (b) Thermochromic image of 28. (c) Photothermal conversion of compound 150-28 at different powers. | |
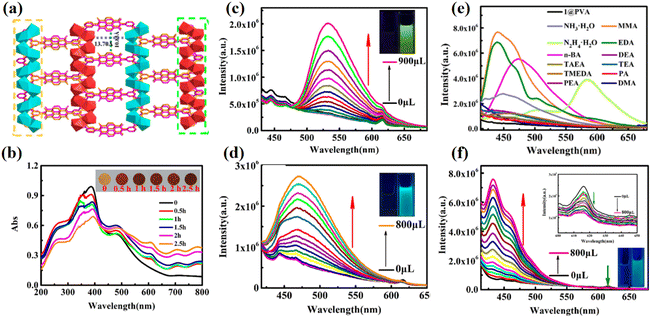 |
| Fig. 12 (a) Three-dimensional network structure of complex 29. (b) UV-vis pattern and photochromic picture of 29. (c) Fluorescence emission patterns of 29 with different concentrations of OPD. (d) Fluorescence emission patterns of compound 29 at different concentrations of n-BA. (e) Light response to aliphatic amines and aromatic amines of 29 test strip. (f) Fluorescence response of 29 to different amounts of TBZ. | |
The construction of NCHPMs based on carboxylic acid-modified NDIs is influenced not only by cations, but also by the addition of chiral molecules, amino acid derivative NDI ligands, or the introduction of multiple acids, all of which have a significant impact on the structure and photochromic properties of the compound. In 2019, Kwak's group reported AlaNDI-Cd (31) with both homochiral and heterochiral forms by coordinating with amino acid-modified NDIs and Cd (Fig. 13).108 Due to the production of NDI radicals, (Rac)-31 changed from yellow to black under UV light, and the photochromic process was accompanied by an increase in conductivity. It is noteworthy that doping hydrazine with (Rac)-31 significantly enhanced the photoresponse rate and conductivity (Fig. 14). When (Rac)-31 micro/nanocrystals were exposed to hydrazine gas for 5 minutes, the conductivity increased from 5.2 × 10−7 S m−1 to 1.3 × 10−3 S m−1, a 2500-fold increase. This enhancement was attributed to the reduction of energy gap upon introduction of hydrazine, leading to increased production of NDI radicals, thereby impacting photochromism and conductivity. This study presents a novel approach for enhancing the photoresponsivity and conductivity of NCHPMs. At the same time, it is proved that nano-sized NCHPMs have high potential in optoelectronic applications. By varying the size of the NDI nitrogen substituent, it is possible to control the photochromic properties. Building on this principle, in 2024, Fu et al. investigated coordination polymers based on NDI with different sizes; [Mn(CMNDI)(H2O)2] (32) and [Mn(CENDI)(H2O)2] (33) (H2CMNDI = N,N′-bis(carboxymethyl)-1,4,5,8-naphthalenediimide, H2CENDI = N,N′-bis(carboxyethyl)-naphthalenediimide) were constructed.109 Compound 32 and 33, featuring Mn2+ nodes and NDI bridges, have been synthesized as two-dimensional planar structures. Compound 33 formed a 3D supramolecular network with middle layers connected by hydrogen bonds. Compound 32 exhibited reversible color change from yellow to dark brown under both ultraviolet and visible light, with stable charge separation after heat treatment. On the other hand, compound 33 showed sensitivity to ultraviolet light, transitioning from light yellow to light brown. In addition to having the same intermolecular electron transfer pathway (π–π stacking, lone pair–π interactions), the NDI carboxyl group in 32 could achieve intramolecular electron transfer due to its short size (lone pair–π interactions is 3.476 Å). This multi-pathway electron transfer gave compound 32 faster photochromic and a wider range of light responses. At the same time, both compounds had electrochromic properties.
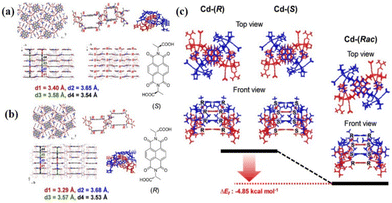 |
| Fig. 13 (a) Indicates the connection of the two NDI units in (Rac)-31 and the π–π stacking. (b) Indicates the connection mode of (R)-31 and the π–π accumulation between the centroids. (c) The formation energy calculation of chiral recognition in 31. | |
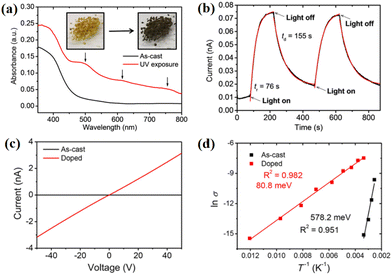 |
| Fig. 14 (a) Photochromic image and UV-vis spectrum of (Rac)-31. (b) Photocurrent profile of (Rac)-31. (c) I–V curves of hydrazine-doped (Rac)-31. (d) Conductivity Change under hydrazine doping in (Rac)-31. | |
NDI is known to be a rigid ligand with a large conjugation plane. Rigid ligands are generally relatively fixed during the coordination process, resulting in somewhat predictable structures assembled with metal ions. However, modifying the NDI with different carboxyl groups will result in a diverse topology due to a variety of coordination modes including single-tooth coordination, chelating coordination, and bridging coordination. At the same time, the diverse structures enable multifunctional development. For instance, the incorporation of POMs can provide luminescent materials with a wider range of functional options. In 2020, Lu's group successfully synthesized a novel material [(CH3)4N]4[Ln(L)1.5(H2O)3] (SiW12O40)·4H2O·3DMF (34),110 and it was discovered that the room-temperature phosphorescence in all compounds resulted from the generation of electron charge transfer states induced by photoexcitation. In 2024, Dai and Liu et al. demonstrated an NDI-based multifunctional hybrid material with POM as guest, Ce4(BINDI)2(DMA)16·SiMo12O40 (35).111 Ce3+ in 35 was bridged with H4BINDI to create a doubly interpenetrating 3D cation grid, and the POM([SiMo12O40]4−) anion was interspersed as a guest in the void to balance the charge (via anion–π interaction). Under UV-vis light irradiation, 35 changed from yellow to blue, and returned to its original color after 3 days in the dark, which was related to the photoreduction of POM anions and the production of NDI radicals by PET (Fig. 15a and b). The introduction of NDI made it possible to quickly generate stable charge separation states, and to improve near-infrared photothermal conversion (61.9 °C temperature increase in 100 seconds) after irradiation (Fig. 15c and d). Due to the long-lived radical anion and negative reduction potential of 35, it was able to act as a photocatalyst to increase the yield of aryl boron hydroxylation and thiocyanylation of indole (90–97%). This type of NCHPM demonstrates that the generation of radicals is not limited to color changes, but also the generation of highly stable radical anions can achieve photothermal conversion and enhance material conductivity. Stable radical anions can facilitate charge transfer and serve as catalysts to enhance the activity of certain chemical reactions.
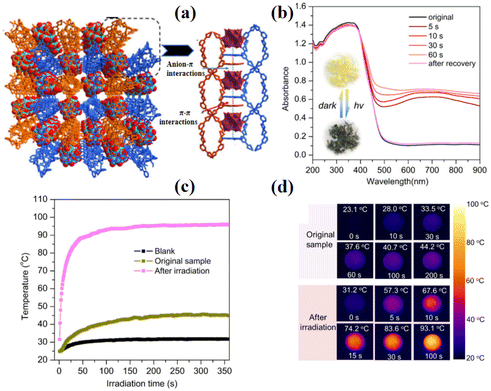 |
| Fig. 15 (a) 3D stacked structure of compound 35. (b) Photochromic image and UV-vis absorption spectra of 35. (c) Photothermal conversion curves of 35 and quartz glass under infrared light. (d) IR images of 35 before and after irradiation. | |
3.4 NCHPMs based on five-membered heterocyclic-modified NDIs
The design of organic ligands significantly influences the structure of NCHPMs. Compared with pyridines, multi-nitrogen five-membered heterocycles offer more coordination sites and stronger coordination capacity. Nitrogen-containing five-membered heterocycle-modified NDIs serve as bridging coordinators, allowing for diverse structural variations through free rotation and coordinated metal ions at appropriate angles and spaces. By combining the variable properties of the five-membered heterocyclic ring with the unique characteristics of NDI, NCHPMs can be applied in a wider range of applications. Han and Lu et al. have used this type of NCHPM to create new application fields.116–122 Multifunctional MOFs have attracted widespread attention due to their high porosity and specific surface area.123,124 Introducing NDIs into the construction of MOFs can result in unexpected effects. In 2015, Han's group synthesized a photochromic and electrochromic MOF [Zn(NDI-ATZ)(DMF)2]n (36) using NDI-ATZ as organic ligands, which was unprecedented.116 Compound 36 featured a 2D porous network structure and exhibited photochromism from yellow to dark green under sunlight due to the generation of photocharge transfer. In 2021, the group reported a 1D chain {[Zn4(NDI-ATZ)2(BDC)2(DMF)6]·(DMF)}n (37) with photochromic properties.117 Through experiments and theories, it was concluded that the strong π–π stacking between NDI ligands was closely associated with its photochromic performance. This suggests that NCHPMs could serve as potential optoelectronic switches and storage devices.
Room temperature phosphorescent (RTP) materials exhibit unique photoluminescence properties and are widely utilized in cryptographic anti-counterfeiting materials and light-emitting diodes due to their extended lifespan and broad response range.125,126 The combination of electron-deficient NDIs and highly negative-charge POMs can achieve high responsiveness and stability of NCHPMs, with the unique anion–π interaction between the two potentially inducing intermolecular charge transfer (ICT) states, thereby enhancing the intersystem crossing (ISC) process and facilitating RTP generation. In 2018, Lu's group first proposed the combination of polyoxometalate (PW12O403−) and NDI derivative H2NDI to prepare a new room-temperature phosphorescent material Zn4(H2NDI)2(NO3)2(DMAc)14·(MeCN)2·(PW12O40)2 (38, H2NDI = N,N′-bis(3,5-dimethylpyrazolyl)-1,4,5,8-naphthalenediimide), in which Zn2+ exhibited two different coordination modes (Fig. 16a).118 The compound was assembled through electrostatic interaction and anion–π interaction between two different zinc complexes and POM molecules. Each POM was surrounded by two different types, forming an arrangement of ABAB′ (Fig. 16b). Compound 38 underwent noticeable color changes after irradiation with visible and ultraviolet light (Fig. 16c and d). Through various tests and characterization methods, it was determined that the photoinduced charge transfer from POM to NDIs generated the NDIs’ radical and tungsten valence, explaining the photochromic behavior of compound 38. Notably, 38 exhibited room-temperature phosphorescence under UV excitation for the first time in a POM-based hybrid material (Fig. 16e and f). After analysis, this RTP phenomenon could be attributed to anion–π interaction-induced charge transfer and heavy atom effects. This study provides important support for the construction of POM-based CHPMs with RTP. Non-covalent interactions also play a crucial role in the formation of NCHPMs, serving not only as an avenue for electron transfer but also leading to energy changes within the system. In 2021, Lin's group reported the synthesis of two D–A hybrid crystals ((H4BDMPyBr2NDI)·(NMP)4HPW12O40) (39) and (H4BDMPy-I2NDI)·(HPW12O40) (40, H4BDMPy-X2NDI = N,N′-bis(3,5-dime-thylpyrazolyl)-2,6-X-1,4,5,8 -naphthalenediimide, X = Br, I), in which Br and I were introduced.119 In compounds 39 and 40, ligands and POMs were arranged alternately, exhibiting a two-dimensional supramolecular network structure. Compound 39 displayed a noticeable color change from yellow to black under light, while compound 40 showed no significant change. EPR analysis revealed that compound 39 was highly sensitive to light, leading to the production of NDI free radicals through electron transfer achieved via anion–π interactions and hydrogen bonding. It is noteworthy that both compounds 39 and 40 exhibited the RTP phenomenon. Comparison indicated that the introduction of halogen atoms could enhance the RTP quantum yield of these compounds, offering new prospects for D–A hybrid photochromic RTP materials.
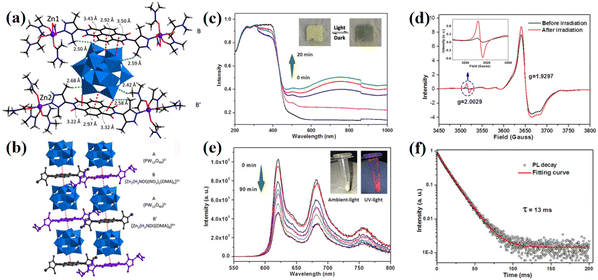 |
| Fig. 16 (a) Non-covalent interaction in compound 38. (b) Unique anion–π interactions in 38. (c) UV-vis pattern of 38. (d) EPR profile of 38. (e) PL spectrum of 38. (f) Luminescence attenuation of 38 at room temperature. | |
This section can offer new research directions in the study of NCHPMs. Firstly, the presence of a D–A system in NCHPMs allows for the promotion of delocalization and photogenerated charge transfer through the combination of ED and EA, enabling the exploration of optoelectronic devices. Secondly, when constructing NCHPMs, consideration can be given to strategies that enhance RTP, thereby achieving multi-functionality by combining photochromism and RTP.
3.5 Other structural types and congeners of NDIs
NCHPMs containing pyridine groups, carboxylic acid groups, and five-membered heterocyclic groups have been extensively utilized and documented. The photochromism of these materials involves electron transfer and subsequent radical formation. Currently, novel NDI ligands decorated by other functional groups, such as sulfonic acid groups, are gradually being developed. This section focuses on photochromic materials featuring some less common NDIs.
Anion–π and lone pair–π interactions are crucial in the development of photochromic NDI materials. These interactions enhance structural stability by restricting molecular movement and relaxation within the system. Additionally, they modify the electronic structure and π conjugation system to improve light response rate and range.127,128 For instance, in 2017, Lu's group synthesized a three-dimensional supramolecular coordination polymer Na(TauNDI)0.5(H2O)2 (41) via the solvothermal method using sulfonic acid-based-modified H2TuaNDI and NaNO3.129 The Na+ ions adopted a pentagonal bipyramidal coordination mode, while the NDI ligands were arranged in a herringbone pattern with adjacent NDIs connected by lone pair–π interactions (Fig. 17a). The middle layers of complex 41 were interconnected by C–H⋯π and hydrogen bonds, providing additional pathways for electron transfer. Under blue light, 41 exhibited abnormal photosensitivity and underwent color darkening. Furthermore, its resistivity sharply decreased after illumination, making it suitable for light-controlled switches and photovoltaic materials (Fig. 17b). Additionally, they prepared nanowire hydrogel (42) composed of Pb(NO3)2 and H2TuaNDI in the same year. The photochromic properties of 42 were attributed to charge transfer between NDI ligands in the gel state. Moreover, this hydrogel synthesis method can be utilized for selective detection of Pb2+. This study offers valuable insights into the diversification and versatility of NDIs as photochromic materials. In 2022, Ke et al. reported a multifunctional MOF with a flexible 3D frame structure, K7(DSPNDI)3(OH) (H2O)(DMSO)2·2(H2O) (43).130 The three adjacent NDI ligands in the structure were distorted, and the carbonyl group of the NDI formed a lone pair–π interaction (3.062 Å) with the adjacent NDI core, serving as a potential electron transfer pathway. Compound 43 exhibited photochromic properties under blue light (changing from yellow to brown), and free radicals remained present for up to 20 days, indicating the long-lived state of charge separation. In the near-infrared region, compound 43 showed a distinct absorption band, and the NDI radicals generated under blue light irradiation could be utilized for photothermal conversion (Fig. 18).
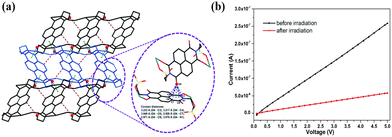 |
| Fig. 17 (a) Lone pair–π interactions between layers in compound 41. (b) Change in resistivity of compound 41 before and after irradiation. | |
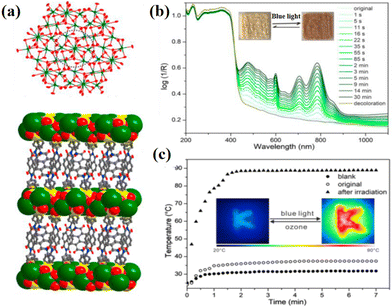 |
| Fig. 18 (a) 2D layer and 3D frame structure of compound 43. (b) Photochromic image and UV-vis absorption spectra of 43. (c) Photothermal conversion curves of 43 before and after illumination. | |
Combined with the high redox activity of NDIs and the structural designability of MOFs, it is possible to construct multifunctional MOFs. In 2023, Gu et al. reported a MOF: Zr-NDI-H2DPBP (44, H2DPBP = 5,15-di(pbenzoato)porphyrin) featuring porphyrin as donor and NDI as acceptor.131 The selection of H2DPBP was based on its similar molecular length to the NDI ligand, and the construction of an octahedral shape by replacing part of the reported Zr-NDI with H2DPBP (confirmed by color change and comparison of three porphyrins). Compound 44 exhibited photochromic behavior under xenon lamp irradiation and gradually reverted to its original color in air due to electron transfer between H2DPBP and NDI induced by light. This resulted in the generation of colored NDI radicals. Furthermore, 44 could serve as a photocatalyst for enhancing amine oxidation coupling (with a catalytic rate 17 times that of Zr-NDI), mainly attributed to efficient charge separation during the PET process along with improved photocatalytic activity facilitated by charge transfer and energy transfer mechanisms. Unfortunately, it could only achieve photochromism in the absence of oxygen.
Aromatic diamides (ADIs) are widely utilized in the fields of optics, electronics, catalysis, and biomedicine due to their extensive π acidic conjugation plane, high electron affinity, exceptional oxidation stability, and thermal stability.132–134 PMDIs (pyromellitic diimides), NDI and PDIs (perylene diimides) have received extensive attention as research hotspots among ADIs. Structurally, PMDI adopts a compact diamidimine structure with a benzene ring at its core, while NDI and PDI feature naphthalene and perylene rings, respectively, at their cores; all three compounds possess large planar rigid structures. In terms of size, similarity between PMDI and NDI is observed, with PDI being the largest. These compounds exhibit modifiable properties along with core electron-deficient characteristics and low LUMO energy levels (PMDI: ELUMO = ∼−3.6 eV, NDI: ELUMO = −3.7 eV, PDI: ELUMO = −3.8 eV), rendering them valuable as electron acceptors for constructing D–A-type stimulus response materials.46,135,136 Compared with NDIs, PMDIs and PDIs have limited reports on hybrid photochromic materials, which may be related to their π conjugation length and free radical stability. Some of the representative work is reported below. For example, in 2021, Fu et al. reported two positionally heterogeneous PMDI-based supramolecular polymers, 2-PMPMD (45) and 4-PMPMD (46) (2/4-PMPMD = N,N′-bis(2/4-pyridylmethyl)-pyromellitic diimide).137 In compounds 45 and 46, the carbonyl group in the PMDI ligand interacted with the adjacent ligand core to form a one-dimensional supramolecular chain through lone pair–π interactions. However, due to positional isomerism, compound 46 formed a 3D supramolecular network as the adjacent chains were linked through C–H⋯N hydrogen bond interactions. The intensity of the lone pair–π interaction in compound 46 exceeded that of compound 45 based on theoretical calculations. Under UV irradiation, compound 45 changed from colorless to light yellow in 30 minutes, while compound 46 changed color from colorless to green in just under a minute. This difference was attributed to the production of PMDI radicals.
In 2022, Li et al. synthesized two coordination polymers using NDIs and PMDIs as organic ligands, namely [Eu(H2BINDI)(BINDI)0.5 (H2O)2]·NH2(CH3)2·8H2O (47) and [Eu2(BIPMDI)(DMF)4 (NO3)2]·H2O·2DMF (48, H4BIPMDI = N,N′-bis(5-isophthalic acid)-pyromellitic diimide).138 Compound 47 used [Eu2(COO)8(H2O)2]2− as the secondary building unit (SBU), and was linked to the NDI ligand to form a 3D framework structure. Compound 48 took [Eu2(COO)4(NO3)2(DMF)4] as the SBU and was linked by PMDI ligands to form a 3D framework. The two compounds exhibited different light response behaviors: under ultraviolet irradiation, compound 47 changed from yellow to dark brown in 30 minutes, while compound 48 changed from light yellow to bright yellow in 45 minutes (Fig. 19). There exists a π–π interaction (3.439 Å) and a lone pair–π interaction (3.210/3.440 Å) between NDIs in compound 47; however, compound 48 may not have π–π interactions due to the small size of PMDI but only lone pair–π interactions between NO3− and PMDI. This differential D–A interface relationship and photoresponsive behavior may be related to the size of the π conjugated system between ligands.
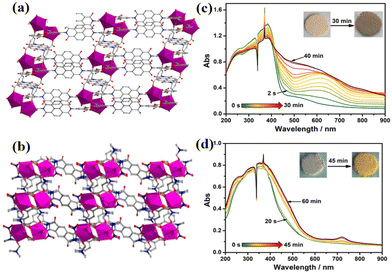 |
| Fig. 19 (a) and (b) Three-dimensional frame structure of compounds 47 and 48. (c) and (d) Photochromic properties and UV-vis absorption spectra of 47 and 48. | |
Due to the poor solubility and tendency for π–π coupling during self-assembly, PDIs are more inclined to form low-dimensional hybrid materials. This issue can be addressed by introducing substituents to alter the degree of distortion in PDIs. In 2019, Yin et al. successfully synthesized a 3D MOF by introducing a chloride substituent strategy to change the planarity of PDIs, [Zr6O4(OH)4(P-2COOH)4(CH3COO)4]·6H2O (49, P-2COOH = N,N′-di-(4-benzoic acid)-1,2,6,7-tetrachloroper-ylene-3,4,9,10-tetracarboxylic acid diimide).139 Compound 49 consisted of P-2COOH and Zr6(μ3-O)4(μ3-OH)4 metal clusters. Notably, compound 49 underwent a color change from red to black upon irradiation with blue light (455 nm) for 10 minutes in the presence of organic amines as electron donors (ED) (Fig. 20a–c). Further exposure to light resulted in the appearance of distinct radical anion characteristic peaks in the absorption and EPR spectra due to photoinduced electron transfer (PET). Owing to its compact and stable structure, compound 49 was able to sustain the existence of radical anions for over a month. The near-infrared absorption and radical stability of compound 49 enabled it to exhibit efficient photothermal conversion efficiency (52.3% conversion efficiency) (Fig. 20d), along with excellent infrared imaging capabilities. This study offers new insights into the design and application of photochromic materials featuring stable radicals. In 2021, Lin's group reported four PDI-based hybrid materials.140 The four compounds differed only in the POM anion (50–53, HnXM12O40, X = P or Si; M = W or Mo). Due to the introduction of chlorine atoms in the structure, the PDI ligand exhibited two enantiomers, which were connected by hydrogen bonds to form a racemic 1D network, and POM was linked to the adjacent 1D chain by anion–π interactions. As a result of anion–π interactions of varying intensities, the four compounds displayed controlled photochromism.
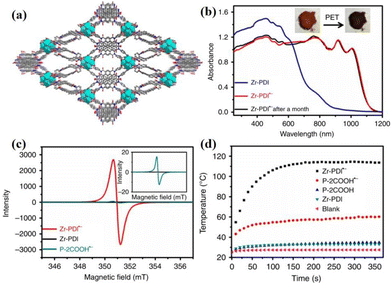 |
| Fig. 20 (a) Structural stacking diagram of compound 49. (b) Photochromic image and UV-vis-NIR absorption spectra of 49. (c) EPR spectra of compound 49 and P-2COOH. (d) Photothermal conversion curve of compound 49 under photoexcitation. | |
This section primarily introduces rare functionalized NCHPMs and their properties. It is observed that the synthesis of photoswitch conductive materials and photocatalytic materials can be achieved through the construction of a D–A system. The matching of EDs and EAs allows for extension of the absorption band, resulting in near-infrared absorption. Additionally, this section includes information on the basic properties of NDI congeners and reports on photochromic materials. Although limited reports make it difficult to clarify their effects on photochromism, it can be determined that π size of the conjugated system has an effect on the photochromic properties.
In conclusion, this section provides a summary of NCHPMs constructed by NDIs modified with various substituents (Table 1). It was observed that the photochromic performance of NCHPMs is influenced by several factors, including the size and configuration of NDIs, the electron-donating ability and conjugation degree of the auxiliary ligand, as well as the type and ratio of solvents. These factors can be manipulated by adjusting the intensity of non-covalent interactions at the D–A interface. It is noteworthy that lone pair–π interaction and anion–π interaction play a role in both construction and photochromic performance of NCHPMs. In addition, the physical and chemical properties of the material will change during the PET process, which is expected to enable the development of new multifunctional NCHPMs.
Table 1 NCHPMs based on ligands of NDIs of different structures
Complex |
Structure type |
Photochromic |
EDs |
ET pathway |
Ref. |
1
|
3D supramolecular |
Red → brownish black |
ODPNDI |
Lone pair–π |
83
|
2
|
3D supramolecular |
Red → brownish black |
ODPNDI |
Lone pair–π |
83
|
3
|
3D supramolecular |
Photoinertness |
ODPNDI |
Lone pair–π |
83
|
4
|
2D supramolecular |
Yellow → brown |
ODMF/MeOH |
Lone pair–π |
84
|
5
|
2D supramolecular |
Photoinertness |
ODMF/MeOH |
Lone pair–π |
84
|
6
|
3D supramolecular |
Yellow → dark brown |
ODMF/MeOH |
Lone pair–π |
84
|
7@DMF
|
3D MOF |
Yellow → reddish-brown |
ODMF |
Lone pair–π |
85
|
8
|
3D MOF |
Photoinertness |
ONDC |
C–H⋯π |
86
|
9
|
3D MOF |
Yellow → brown |
ONDC |
C–H⋯π |
86
|
10
|
3D supramolecular |
Blue → slight changes |
OPOM |
Anion–π |
87
|
11
|
3D supramolecular |
Blue → slight changes |
OPOM |
Anion–π |
87
|
12
|
3D supramolecular |
Blue → dark blue |
OPOM |
Anion–π |
88
|
13
|
3D supramolecular |
Yellow → dark |
OPOM |
Anion–π |
88
|
14
|
3D supramolecular |
Deep Yellow → dark reddish-brown |
ONDI-A |
Lone pair–π |
102
|
15
|
3D MOF |
Orange → brick red |
OSBC |
π–π |
103
|
16
|
3D MOF |
Orange → color deepening |
OBPC |
π–π |
103
|
17
|
3D MOF |
Light orange → dark brown |
ONDI-A |
Lone pair–π |
104
|
18–25
|
3D supramolecular |
Light orange → dark brown |
ONDI-A |
Lone pair–π |
104
|
26
|
3D MOF |
Photoinertness |
|
|
105
|
27
|
3D MOF |
Yellow → brown |
ONicNDI |
Lone pair–π |
105
|
28
|
3D MOF |
Yellow → dark |
OBINDI/BA |
Lone pair–π/hydrogen bonds |
106
|
29
|
3D MOF |
Yellow → reddish-brown |
— |
— |
107
|
30
|
3D MOF |
Pale pink → brown |
— |
— |
107
|
31
|
3D supramolecular |
Yellow → black |
— |
— |
108
|
32
|
2D MOF |
Yellow → dark brown |
OCMNDI |
Lone pair–π |
109
|
33
|
3D supramolecular |
Light yellow → light brown |
OCENDI |
Lone pair–π |
109
|
34
|
3D supramolecular |
Light yellow → brown |
OPOM |
Anion–π |
110
|
35
|
3D supramolecular |
Yellow → blue |
OPOM |
Anion–π |
111
|
36
|
2D MOF |
Yellowish →dark green |
— |
— |
116
|
37
|
1D stair-like |
Yellow brown → dark green |
OBDC |
π–π |
117
|
38
|
3D supramolecular |
Yellowish → green |
OPOM |
Anion–π |
118
|
39
|
2D supramolecules |
Yellow → black |
OPOM |
Anion–π |
119
|
40
|
2D supramolecules |
Orange → no change |
OPOM |
Anion–π |
119
|
41
|
3D supramolecules |
Olive → dark olive |
ONDI |
Lone pair–π |
129
|
43
|
3D MOF |
Yellow → brown |
ONDI |
Lone pair–π |
130
|
44
|
3D MOF |
Brown → slight changes |
ODPBP |
— |
131
|
45
|
1D supramolecules |
Colorless → light yellow |
OPMDI |
Lone pair–π |
137
|
46
|
3D supramolecules |
Colorless → green |
OPMDI |
Lone pair–π |
137
|
47
|
3D MOF |
Yellow → dark brown |
ONDI |
Lone pair–π |
138
|
π–π |
48
|
3D MOF |
Light yellow → bright yellow |
OPMDI |
Lone pair–π |
138
|
49
|
3D MOF |
Red → black |
Organic amines |
— |
139
|
49–51
|
3D supramolecules |
— |
OPOM |
Anion–π |
140
|
4. Application
Naphthalene diimides exhibit strong electrophilic ability, exceptional stability, redox properties, and diverse self-assembly, making them promising candidates for applications in molecular switches, semiconductor materials, and sensors.141–143 The synthesis of naphthalimide derivatives achieves the desired effect by modifying different functional groups to produce attractive materials. Modifying the nitrogen of the imide with functional groups and replacing the naphthyl nucleus are two common methods. However, recent research trends have focused on combining photochromic properties to explore other photochemical or photophysical properties. The introduction of an adjustable NDI-based organic ligand in NCHPMs retains their advantages and further optimizes their photochromic performance, realizing multifunctional photochromic materials. Recently developed novel multifunctional photochromic materials, such as NCHPMs, have demonstrated several advantages: (1) due to the low π electron density in NCHPMs, it is easy to form a D–A system and produce a variety of PET channels, resulting in excellent photochromic properties. Additionally, due to the participation of inorganic components, they have a long cycle life. (2) NCHPMs exhibit various coordination configurations and topologies accompanied by physical changes during color change processes, allowing for multi-functional development. (3) NCHPMs can form non-covalent interactions with electron-rich systems and can be detected and classified by changes in color and luminescence.
4.1 Harmful light detection and filtration
Exposure to purple light can have detrimental effects on human health, including skin conditions such as sunburn, premature aging, and an increased risk of skin cancer. Prolonged exposure to strong purple light may also lead to eye inflammation, conjunctivitis, and potential vision impairment. Additionally, purple light has the potential to trigger autoimmune diseases.144–146 Therefore, the selection of suitable materials for detecting and filtering purple light has become a primary concern. The NCHPMs for purple light detection and filtration are outlined below, chosen for their heightened sensitivity to light within the purple wavelength range, rapid response time, and excellent cycle life. In 2023, Zheng's group reported the discovery of a cadmium-based MOF material: {Cd3(NDI)3(IPA)3·3H2O}n (54).147 This compound underwent a crystal to crystal transition under UV irradiation, generating {[Cd2(NDI)2(IPA)2]·3H2O}n (54-P). The transformation involved the rotation and distortion of the NDI ring. Subsequent soaking in acetonitrile led to another crystal transformation, resulting in {[Cd3(NDI)3(IPA)3]·3H2O·0.5MeCN}n (55). This crystal transformation involved the addition of only half as many acetonitrile molecules, with no significant changes in other crystal parameters. It is noteworthy that this transformation enhanced electron transfer and accelerated the rate of photochromism. Upon irradiation with visible light, UV, and X-ray, it was observed that compounds 54 and 55 exhibited greater sensitivity to purple light compared with other light sources, resulting in shorter photochromic time and better coloring (Fig. 21a). Prolonged exposure to purple light can be harmful to human eyes and skin; however, this material has the ability to absorb purple light without causing harm. This discovery holds potential benefits for human life and health. Furthermore, compound 55 was also combined with glass to assess its effectiveness in filtering out purple light (Fig. 21b).
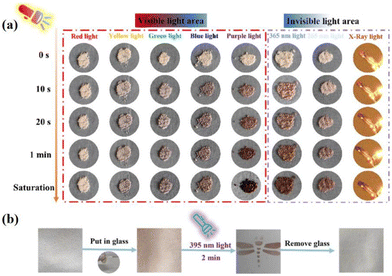 |
| Fig. 21 (a) The color change of compound 55 (b) The color change of the prepared 55-based glass under UV irradiation, and ink-free printing can be performed on the glass. | |
Blue light is ubiquitous in people's daily lives, but prolonged exposure to it can potentially harm the eyes and lead to retinal diseases. Additionally, blue light suppresses melatonin secretion, resulting in sleep disturbances.148–150 As a result, there is growing demand for the detection and filtration of blue light. In 2022, Fu's group utilized PMNDI as ligands to synthesize two types of NCHPMs, [Cd(3-PMNDI)(HNDC)2(DMF)] (56) and [Cd(4-PMNDI)(NDC)]·DMF (57).151 These compounds exhibited rapid response rates to light and were more sensitive to visible light compared with most NCHPMs (Fig. 22a and b). The unique light response of 56 and 57 may be attributed to variations in π–π interactions and lone pair–π interactions at the donor–acceptor interfaces. Upon irradiation with visible light of different wavelengths, it was observed that the photosensitivity range of 56 and 57 corresponded precisely with the wavelength range of blue light, making them promising materials for detecting and filtering blue light (Fig. 22c).
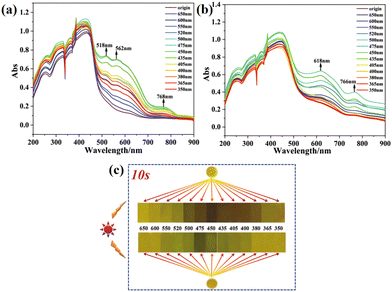 |
| Fig. 22 UV-vis spectra of 56 (a) and 57 (b) at different wavelengths of visible light. (c) Photochromism for 56 and 57 at different wavelengths of visible light. | |
4.2 Information storage and encryption
With the advancement of society, global attention has shifted towards environmental protection and sustainable development. Within the printing industry, traditional ink printing not only contributes to environmental pollution but also results in the wastage of natural resources. This necessitates the adoption of new technologies and materials to address these issues.152–154 Photochromic materials are well suited for this purpose due to their excellent color-changing properties, reversibility, and long-lasting color retention. Inkless printing offers a solution to reduce paper waste and minimize environmental harm during paper processing and printing.155–157
Conventional NCHPMs are not suitable for inkless printing applications due to their short photochromic lifetime. However, MOF materials can effectively prevent the accumulation of organic ligands and prolong the existence time of free radicals because of the three-dimensional network structure.158–161 For example, in 2016, Rahul Banerjee's research group successfully synthesized three types of NDI-based metal–organic framework (MOF) photochromic materials: Mg-NDI (58), Ca-NDI (59), and Sr-NDI (60).162 These materials were utilized in ink-free printing applications. Among them, the color of 58 was light yellow, while the other two MOFs were essentially colorless. Upon exposure to strong sunlight, 58 turned black-brown, whereas 59 and 60 turned green (Fig. 23). These MOFs could be applied as a coating on coated paper for ink printing purposes, with the coated paper maintaining good quality even after four uses. Furthermore, the printed content on the paper could be rapidly erased by placing it in an oxygen atmosphere. Additionally, these materials could be transformed into two-dimensional codes that were recognizable by electronic devices after color development, demonstrating their accuracy and readability in printing applications (Fig. 24). In 2022, Mo's group used H4BINDI to prepare a novel MOF, [Ca6(BINDI)3 (DMA)2(H2O)4] (61).163 Generally speaking, NDI is highly unstable and susceptible to quenching. The discoloration of 61 had a very long lifespan and could be stable for one month. Analysis revealed that the unique three-dimensional structure of 61 effectively isolated adjacent NDI ligands and prevented electron transfer between NDIs. Furthermore, the absence of a single electron in the 3d orbital of Ca2+ inhibited electron transfer between NDI and Ca. Additionally, 61 demonstrated a rapid light response rate. These characteristics, combined with its suitability for inkless printing, resulted in high-resolution printed content that remained clearly visible within 72 hours. It is noteworthy that 61 could be reused, and the coated paper prepared from it retained its integrity even after folding and twisting (Fig. 25).
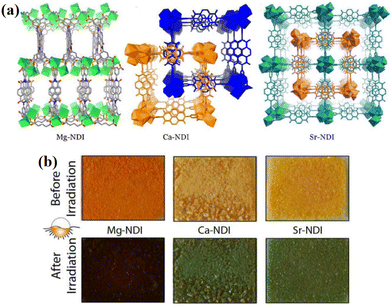 |
| Fig. 23 (a) The crystal structures of 58, 59 and 60, respectively. (b) Photochromic images of 58, 59 and 60, after strong light irradiation. | |
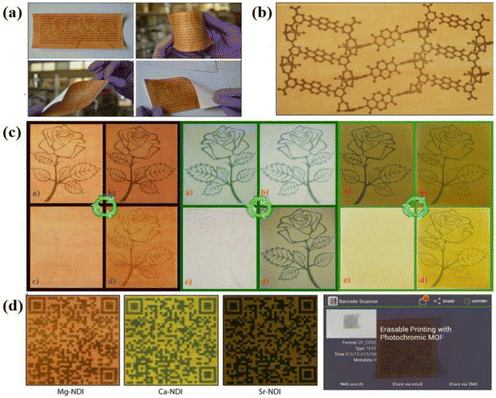 |
| Fig. 24 (a) Inkless printing paper prepared property of 58. (b) Print out the stick model diagram of 58. (c) Flowers patterns. (d) Two-dimensional codes printed on paper prepared by three MOFs materials and identified using electronic devices. | |
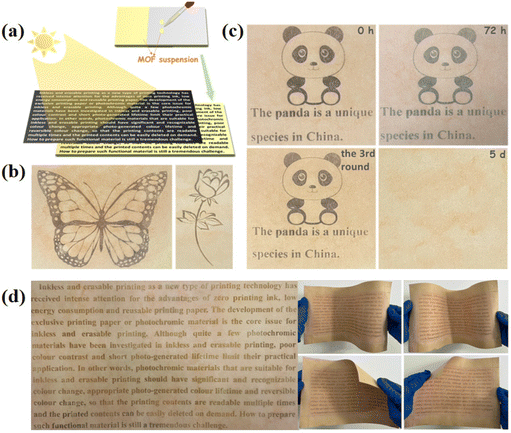 |
| Fig. 25 (a) Preparation process of coated paper for 61. (b) Printed butterfly and flower shapes. (c) Clarity and cycling test of the printed content at different times. (d) Stability test of coated paper. | |
The discovery and research of anti-counterfeiting materials play crucial roles in protecting patented technologies and personal interests. Superior anti-counterfeiting technology is essential in combating and restricting the proliferation of counterfeit and substandard products.164–167 Currently, the exceptional anti-counterfeiting performance of fluorescent photochromic materials has garnered widespread attention.168–171 In 2023, Zheng's group constructed a material based on NDI, (62 = N,N′-bis(2-butyric acid)-1,4,5,8-naphthalene diimide).172 It was observed that 62 exhibited diverse photochromic behaviors in different solvents and underwent simultaneous changes in fluorescence color. This unprecedented multichromatic fluorescence phenomenon in NDI photochromic materials could be attributed to the influence of solvents on the mobility of free radical anions (Fig. 26). Varying concentrations of 62 in solvent systems resulted in distinct luminescence states under ultraviolet excitation, with high concentrations leading to emission of 62 excimer and low concentrations resulting in emission of NDI˙−. Notably, the dynamic fluorescence emission was produced by 62 in DMF under UV irradiation. Furthermore, photochromic films incorporating polymethyl methacrylate (PMMA) with 62 exhibited dynamic information anti-counterfeiting properties and were reusable (Fig. 27).
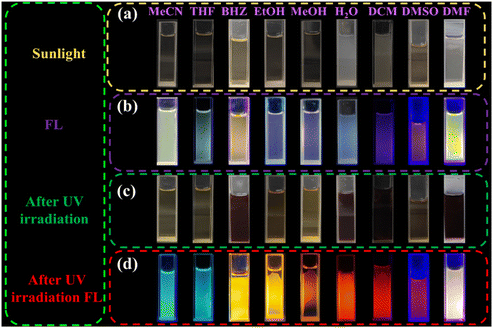 |
| Fig. 26 Color (a) and fluorescence (b) of 62 in different solvents. Photochromism (c) and fluorescence (d) of 62 in different solvents. | |
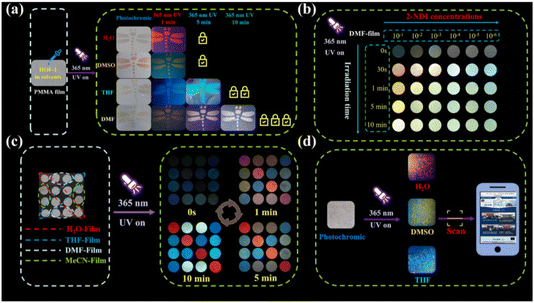 |
| Fig. 27 (a) Dynamic fluorescence exhibited by photochromic films prepared by 62 under different solvents. (b) Changes in fluorescence of DMF-membranes prepared at different concentrations of 62. (c) Thin films prepared with different solvents form squares. (d) Photochromic fluorescence color of membranes prepared with three different solvents. | |
4.3 Application of chemical sensors
Organic amines are commonly found in industrial and agricultural production. Despite their wide range of applications, organic amines pose certain health risks to humans, such as an increased risk of cancer with long-term exposure. Additionally, they are flammable and explosive substances, posing potential hazards to human safety and property.173–176 Therefore, the detection of organic amines is crucial. Organic amine sensors must exhibit high sensitivity and rapid response across different concentration ranges. Furthermore, these sensors should have a long service life and be cost-effective. Thus, utilizing NCHPMs that are more sensitive to amines can meet this demand. The primary principle of NCHPM chemical sensors involves leveraging the interaction between analytes and organic ligands to detect them through color or luminescence changes.177,178 In 2015, Rahul Banerjee's group prepared a naphthalene diimide MOF material Mg-NDI (58) (Fig. 28a and b). Due to the presence of the NDI core, 58 was an electron-deficient system.179 Treatment of 58 with various organic amines revealed that this MOF material could rapidly detect small molecules of organic amines through color change and fluorescence quenching, which was visible to the naked eye (Fig. 29). Additionally, 58 exhibited different colors in varying concentrations of amine vapor. As the concentration of amine solution increased, the photoluminescence properties of 58 were progressively quenched until completely disappearing. It is noteworthy that this MOF material also displayed solvent discoloration behavior (Fig. 28c), with 58 exhibiting negative solvent discoloration behavior as solvent polarity increased. These properties have potential applications in mixed solvent detection and sensing.
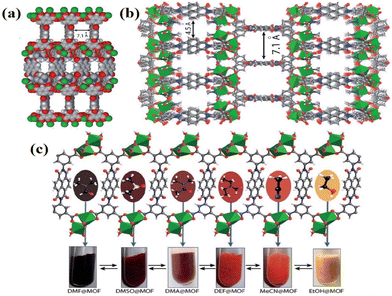 |
| Fig. 28 (a) The frame structure of 58. (b) The perspective of the extended structure of 58. (c) The solvent discoloration of 58 in different solvents resulted in a high color shift with the increase of solvent polarity. | |
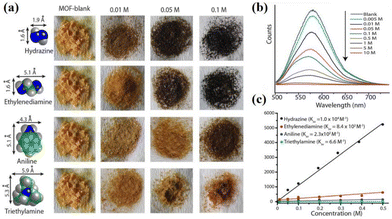 |
| Fig. 29 (a) 58 shows different discoloration in different concentrations of organic amines. (b) The fluorescence of 58 was quenched by increasing the concentration of aniline. (c) Stern–Volmer plots of 58 in different organic amines. | |
Sensors are widely utilized across various fields for their real-time detection, high-precision measurement, automatic control, and ability to enhance safety and conserve resources. As a result, sensors play a crucial role in the advancement of science, technology, and industry.180,181 The subsequent discussion will focus on photochromic material based on NDIs, which has diverse applications in sensor technology including optical switch sensing, photoluminescent sensing, and chemical resistance sensing. In 2017, Sang Kyu Kwak's research group synthesized the coordination polymer AlaNDI-Zn (63) with both homochiral and heterochiral forms.182 Compound 63 exhibited a three-dimensional supramolecular network structure through π–π interactions between NDI ligands. Interestingly, the heterochiral 63 underwent a color change from yellow to dark brown under UV light, attributed to the PET of the NDI ligand in the system. It is noteworthy that the conductivity of 63 increased upon UV irradiation, possibly due to the generation of extra carriers when NDI radicals were formed. Owing to its electron-deficient nature, compound 63 could detect electron-rich hydrazine through fluorescence changes, with a lower detection limit of 3.2 × 10−6 M. This phenomenon was associated with ET between 63 and hydrazine. Chiral 63 also demonstrated potential for detecting chiral neproxen. Furthermore, insulator 63 could detect electron-rich alcohols and amines by changes in resistivity, particularly exhibiting high sensitivity towards aniline (Fig. 30).
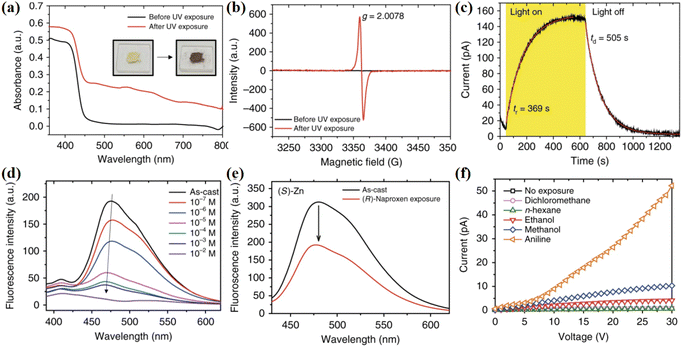 |
| Fig. 30 (a) UV-vis absorption spectra of compound (Rac)-63. (b) EPR spectra of (Rac)-63 before and after illumination. (c) Change in current during illumination of (Rac)-63. (d) (S)-63 and (R)-naproxen fluorescence quench in ethanol. (e) Fluorescence sensing curves of (S)-63 pairs (R)-naproxen and (S)-naipxen. (f) I–V curves of (Rac)-63 in different VOCs. | |
Bromine ion (Br−) is widely used in industrial manufacturing processes, and high concentrations of bromide can cause harm to the human body, such as respiratory tract and skin irritation.183–185 Additionally, bromide ions can also have detrimental effects on the environment and ecological stability. Currently, detecting bromide ions requires costly and complex processes, creating a demand for a convenient and rapid bromide ion detection device. Previous research has explored utilizing the fluorescence properties of NCHPMs to develop sensors for detecting various ions.186 In 2023, Zheng's group synthesized a novel NCHPM (64) featuring Bi(III) as the bridging link.187 Compound 64 exhibited reduced sensitivity to ultraviolet and X-rays, while demonstrating rapid response to visible light (Fig. 31a). Comparative analysis revealed that 64 displayed the highest fluorescence in aqueous solution and maintained relatively stable properties. Further experimentation with different anions in the aqueous solution containing 64 indicated distinct behavior of Br− compared with other anions. Specifically, detection of Br− resulted in a redshift and enhanced fluorescence emission in the aqueous solution, contrary to typical fluorescence quenching sensors, causing a change from light yellow to orange-yellow (Fig. 31b and c). The heightened sensitivity and anti-interference capabilities of 64 were attributed to the substitution of Br− for Cl− within its structure. Notably, the visual detection capability of 64 combined with its photochromic performance can be applied for information encryption purposes (Fig. 32).
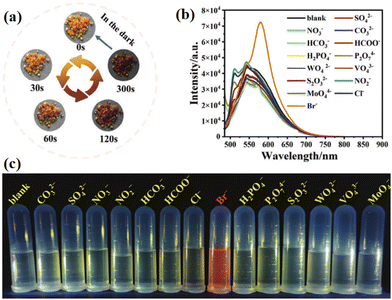 |
| Fig. 31 (a) Photochromic image of 64 in visible light. (b) Fluorescence emission spectra of different anions in an aqueous solution containing 64. (c) Selective detection of bromine ions. | |
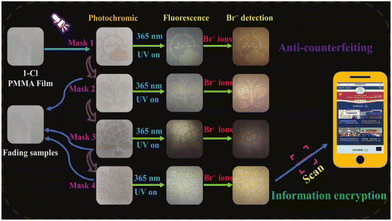 |
| Fig. 32 Photochromic combined with compound 64 and fluorescence detection of bromide ions for information encryption. | |
5. Conclusions and outlooks
In conclusion, we provide a comprehensive overview of the research progress on NDI-based photochromic materials. The classification of NCHPMs by different substituents is emphasized, along with the assembly strategies, packing mode of molecules, photochromic properties and influencing factors, and potential applications such as harmful light detection, information storage and encryption, and chemical sensors. We systematically elucidate the relationship between the structure of NCHPMs and their photochromic properties. Rational chemical structure design and adjustment of the interface relationship in the D–A system are crucial for achieving multifunctionality in NCHPMs. This review aims to offer insights into the rational design of new multifunctional NCHPMs in the future. Currently, some NCHPMs have potential applications in photocatalysis, photothermal conversion, and optoelectronic devices. However, key issues still need to be urgently addressed as research on multifunctional NCHPMs progresses.
1. Constructing new NDIs. Photochromic performance mainly relies on electron-deficient NDIs. The diversity of NDI ligands offers the possibility for constructing NCHPMs with different color-changing mechanisms and characteristics. By adjusting the structure and composition of NDIs, the function diversification of NCHPMs can be realized, highlighting the necessity to develop new NDIs.
2. Improving the response rate and range of photoresponsivity in NCHPMs. Researchers have proposed strategies such as enhancing the electron transfer pathway, minimizing the distance between electron donors and acceptors, and optimizing the energy level matching in the donor–acceptor system to improve the light response rate of NCHPMs. Despite some NCHPMs demonstrating rapid photoresponsivity, they remain insufficient for practical applications such as optical switches and real-time displays. Currently, the light response range of NCHPMs is mainly limited to the ultraviolet or visible light region, thus it is necessary to broaden the materials’ light sensitivity range to meet actual needs.
3. Enhancing the solubility and dispersion of NCHPMs is crucial, as they currently exhibit poor solubility in common organic solvents. This hinders the preparation of thin films and other materials through solution processing. Additionally, most NCHPMs exist in crystalline form, leading to issues such as poor adhesion to substrates and inadequate interface compatibility when applied to different substrates like gels or glasses. These challenges significantly impact their application performance.
4. Developing multifunctional NCHPMs. Multifunctional NCHPMs have unique color-changing properties and application value, which can meet the needs of new materials in the future. The development of multifunctional NCHPMs needs to consider many aspects such as component selection, design principles, synthesis methods, and performance optimization. At present, some NCHPMs have shown application prospects in the fields of near-infrared photothermal conversion, photocatalysis and optoelectronic devices.
Data availability
No primary research results, software or code have been included and no new data were generated or analysed as part of this review.
Conflicts of interest
The authors declare that they have no known competing financial interests or personal relationships that could have appeared to influence the work reported in this paper.
Acknowledgements
This study was supported by the Natural Science Foundation of Henan Province (242300420327 and NSFRF240702), Key Research Project of Higher Education Institutions of Henan Province of China (23A430025), Science and Technology Project of Henan Province (232102230004 and NSFRF230617) and the Fundamental Research Funds for the Universities of Henan Province of China (NSFRF220404).
References
- X.-S. Xing, C. Sun, L. Liu, M.-S. Wang and G.-C. Guo, Light-Responsive Proton Conductor: Record High Gain of Proton Conductivity Achieved by Photoinduced Electron-Transfer Strategy, CCS Chem., 2021, 3, 50–57 CrossRef CAS.
- L. Li, S.-H. Li, Z.-Y. Li, N.-N. Zhang, Y.-T. Yu, J.-G. Zeng and Y. Hua, Advances in viologen-based stimulus-responsive crystalline hybrid materials, Coord. Chem. Rev., 2024, 518, 216064 CrossRef CAS.
- R. Huo, T. Zhang, L.-X. Sun, L.-N. Zhao, Y.-H. Xing and F.-Y. Bai, Multiple stimulus responsive of a novel naphthalenediimide-based 2D alkaline-earth metal MOF material: Hydrochromism, amine-/acid-induced chromism and ionochromism as well as visual artificial film application, Chem. Eng. J., 2024, 488, 150917 CrossRef CAS.
- S.-D. Han, J.-X. Hu and G.-M. Wang, Recent advances in crystalline hybrid photochromic materials driven by electron transfer, Coord. Chem. Rev., 2022, 452, 214304 CrossRef CAS.
- C. Chen, J. Sun, Y. Zhang, X. Yang and J. Zhang, Flexible Viologen–Based Porous Framework Showing X-ray Induced Photochromism with Single-Crystal-to-Single-Crystal Transformation, Angew. Chem., Int. Ed., 2017, 56, 14458–14462 CrossRef CAS PubMed.
- P. Hao, W. Wang, L. Zhang, J. Shen and Y. Fu, Metal-dependent electronic and photochromic behaviors of dimethylbenzotriazolium iodometallate hybrids, Inorg. Chem. Front., 2019, 6, 287–292 RSC.
- T.-L. Yu, Y.-M. Guo, G.-X. Wu, X.-F. Yang, M. Xue, Y.-L. Fu and M.-S. Wang, Recent progress of d10 iodoargentate (I)/iodocuprate (I) hybrids: Structural diversity, directed synthesis, and photochromic/thermochromic properties, Coord. Chem. Rev., 2019, 397, 91–111 CrossRef CAS.
- S. Yu, A. Tian, Q. Lu, X. Xu, S. Ma, X. Wang and Z. Wang, Polyoxometalate-Viologen Thermochromic Hybrids for Organic Amine Detectors and Memristors with Temperature-Regulating Resistance Switching Characteristics, Inorg. Chem., 2023, 62, 1549–1560 CrossRef CAS PubMed.
- H. Yu, Y.-D. Lin, S.-L. Huang, X.-X. Li, C. Sun and S.-T. Zheng, Photochromic Polyoxoniobates with Photoinduced “D-f-A” Electron Transfer Mechanism, Angew. Chem., Int. Ed., 2023, 62, e202302111 CrossRef CAS PubMed.
- Q. Lu, A. Tian, S. Yu, X. Xu, S. Ma and M. Yang, Four Polyoxometalate-Viologen Discoloration Materials for UV Probing, Inkless and Erasable Printing and Visual Detection of Hg2+, Eur. J. Inorg. Chem., 2023, 26, e202200672 CrossRef CAS.
- X. Zhang, Z. Ma, X. Li, C. Qian, Y. Liu, S. Wang, X. Jia and Z. Ma, Multiresponsive Tetra-Arylethene-Based Fluorescent Switch with Multicolored Changes: Single-Crystal Photochromism, Mechanochromism, and Acidichromism, ACS Appl. Mater. Interfaces, 2021, 13, 40986–40994 CrossRef CAS PubMed.
- Y. Wang, N. Xu, J. Ma, H. Li, Y. Zhang, G. Liu and X. Wang, Stable Zinc(II) Coordination Polymer as a Rapid and Highly Sensitive Fluorescence Sensor for the Discriminative Sensing of Biomarker 2-(2-Methoxyethoxy) Acetic Acid, Inorg. Chem., 2022, 61, 7780–7786 CrossRef CAS PubMed.
- L. Li, Y.-T. Yu, N.-N. Zhang, S.-H. Li, J.-G. Zeng, Y. Hua and H. Zhang, Polyoxometalate (POM)-based crystalline hybrid photochromic materials, Coord. Chem. Rev., 2024, 500, 215526 CrossRef CAS.
- N.-N. Zhang, Y.-T. Liu, L. Li, X.-T. Liu, K. Xu, Z.-Y. Li and Y. Yan, Highly Efficient NIR-II Photothermal Conversion from A 2,2′-Biquinoline-4,4′-Dicarboxylate based Photochromic Complex, Inorg. Chem. Front., 2024, 11, 4867–4875 RSC.
- J. Ying, L. Jin, C. Sun, A. Tian and X. Wang, A Series
of Polyoxometalate–Viologen Photochromic Materials for UV Probing, Amine Detecting and Inkless and Erasable Printing, Chem. – Eur. J., 2022, 28, e202103268 CrossRef CAS PubMed.
- X.-Q. Yu, M.-S. Wang and G.-C. Guo, Single-Component Photochromic Smart Semiconductor with Photoswitchable Fast Bidirectional Electron Transfer, Adv. Funct. Mater., 2023, 33, 2212907 CrossRef CAS.
- D. Feng, Y. Mu, J. Li, S. De Han, J. Li, H. Sun, M. Pan, J. Hu and G. Wang, Light-Induced Electron Transfer Toward On/Off Room Temperature Phosphorescence in Two Photochromic Coordination Polymers, Adv. Funct. Mater., 2023, 33, 2305796 CrossRef CAS.
- J.-L. Zhao, M.-H. Li, Y.-M. Cheng, X.-W. Zhao, Y. Xu, Z.-Y. Cao, M.-H. You and M.-J. Lin, Photochromic crystalline hybrid materials with switchable properties: Recent advances and potential applications, Coord. Chem. Rev., 2023, 475, 214918 CrossRef CAS.
- Y.-P. Song, J.-N. Zhang, J.-R. Wang, K. Li, Y.-X. Yuan, B. Li and S.-Q. Zang, A fast responsive photochromic SCC-MOF for photoswitching and information encryption, Sci. China Mater., 2024, 67, 698–704 CrossRef CAS.
- T.-Y. Dou, C. Zhe-Hong, Y. Guan, Q.-X. Zeng, X.-X. Li and S.-T. Zheng, A novel guanidine-containing polyoxotantalate with reversible thermochromism property, J. Solid State Chem., 2024, 330, 124498 CrossRef CAS.
- A. Kar and C. P. Pradeep, Mixed Organic Counterion Strategy Modulates the Self-Assembly of Polyoxometalate Hybrids into Toroids and Affects Their Photochromic and Photocatalytic Properties, Inorg. Chem., 2022, 61, 20561–20575 CrossRef CAS PubMed.
- A. Mukhopadhyay, V. K. Maka, G. Savitha and J. N. Moorthy, Photochromic 2D metal-organic framework nanosheets (MONs): design, synthesis, and functional MON-Ormosil composite, Chem, 2018, 4, 1059–1079 CAS.
- M.-Q. Zhu, Both self-assembly and aggregation-induced emission are photoswitchable, Sci. China: Chem., 2018, 61, 1201–1202 CrossRef CAS.
- S. Hu, L. Lv, S. Chen, M. You and Z. Fu, Zn-MOF-Based Photoswitchable Dyad That Exhibits Photocontrolled Luminescence, Cryst. Growth Des., 2016, 16, 6705–6708 CrossRef CAS.
- M. B. Avinash, K. Swathi, K. S. Narayan and T. Govindaraju, Molecular Architectonics of Naphthalenediimides for Efficient Structure-Property Correlation, ACS Appl. Mater. Interfaces, 2016, 8, 8678–8685 CrossRef CAS.
- J.-Z. Liao, C. Wu, X.-Y. Wu, S.-Q. Deng and C.-Z. Lu, Exceptional photosensitivity of a polyoxometalatebased charge-transfer hybrid material, Chem. Commun., 2016, 52, 7394–7397 RSC.
- S. V. Bhosale, M. Al Kobaisi, R. W. Jadhav, P. P. Morajkar, L. A. Jones and S. George, Naphthalene diimides: perspectives and promise, Chem. Soc. Rev., 2021, 50, 9845–9998 RSC.
- S. Nussbaum, E. Socie, G. C. Fish, N. J. Diercks, H. Hempel, D. Friedrich, J.-E. Moser, J.-H. Yum and K. Sivula, Photogenerated charge transfer in Dion–Jacobson type layered perovskite based on naphthalene diimide, Chem. Sci., 2023, 14, 6052–6058 RSC.
- L. Zong, M. Zhang, Y. Song, Y. Xie, J. Feng, Q. Li and Z. Li, A red fluorescence probe based on naphthalene diimide for selective detection of sulfide by displacement strategy, Sens. Actuators, B, 2018, 257, 882–888 CrossRef CAS.
- L. Han, L. Qin, L. Xu, Y. Zhou, J. Sun and X. Zou, A novel photochromic calcium-based metal–organic framework derived from a naphthalene diimide chromophore, Chem. Commun., 2013, 49, 406–408 RSC.
- F. Wei, Y. Ye, W. Huang, Q. Lin, Z. Li, L. Liu, S. Chen, Z. Zhang and S. Xiang, A naphthalene diimide-based MOF with mog net featuring photochromic behaviors and high stability, Inorg. Chem. Commun., 2018, 93, 105–109 CrossRef CAS.
- J.-J. Liu, Y. Wang, Y.-J. Hong, M.-J. Lin, C.-C. Huang and W.-X. Dai, A photochromic naphthalene diimide coordination network sensitized by polyoxometalates, Dalton Trans., 2014, 43, 17908–17911 RSC.
- J.-J. Liu, Z.-J. Wang, S.-B. Xia, J. Liu and X. Shen, Photochromic and photocontrolled luminescence properties of two metal-organic frameworks constructed from a naphthalene diimide derivative, Dyes Pigm., 2020, 172, 107856 CrossRef CAS.
- A. M. Sanders, T. J. Magnanelli, A. E. Bragg and J. D. Tovar, Photoinduced Electron Transfer within Supramolecular Donor–Acceptor Peptide Nanostructures under Aqueous Conditions, J. Am. Chem. Soc., 2016, 138, 3362–3370 CrossRef CAS PubMed.
- M.-H. You, M.-H. Li, H.-H. Li, Y. Chen and M.-J. Lin, The impact of metal cations on the photochemical properties of hybrid heterostructures with infinite alkaline-earth metal oxide clusters, Dalton Trans., 2019, 48, 17381–17387 RSC.
- G.-P. Li, H. Zhu, P. Hao, J. Shen and Y. Fu, The modulation effect of carboxylic acid ligands on the electron transfer photochromism of NDI-derived coordination polymers, Dalton Trans., 2021, 50, 15153–15161 RSC.
- Q. Song, F. Li, Z.-Q. Wang and X. Zhang, A supramolecular strategy for tuning the energy level of naphthalenediimide: Promoted formation of radical anions with extraordinary stability, Chem. Sci., 2015, 6, 3342–3346 RSC.
- H. Hu, Y. Zhang, H. Ma, Y. Yang, S. Mei, J. Li, J. Xu and X. Zhang, A Supramolecular Naphthalene Diimide Radical Anion with Efficient NIR–II Photothermal Conversion for E. coli –Responsive Photothermal Therapy, Angew. Chem., Int. Ed., 2023, 62, e202308513 CrossRef CAS PubMed.
- X. Zhan, A. Facchetti, S. Barlow, T. J. Marks, M. A. Ratner, M. R. Wasielewski and S. R. Marder, Rylene and Related Diimides for Organic Electronics, Adv. Mater., 2011, 23, 268–284 CrossRef CAS PubMed.
- X. Peng, L. Wang and S. Chen, Donor–acceptor charge transfer assemblies based on naphthalene diimides (NDIs), J. Inclusion Phenom. Macrocyclic Chem., 2021, 99, 131–154 CrossRef CAS.
- M. Pan, X.-M. Lin, G.-B. Li and C.-Y. Su, Progress in the study of metal–organic materials applying naphthalene diimide (NDI) ligands, Coord. Chem. Rev., 2011, 255, 1921–1936 CrossRef CAS.
- F. O. Holtrup, G. R. J. Müller, H. Quante, S. De Feyter, F. C. De Schryver and K. Müllen, Terrylenimides: New NIR Fluorescent Dyes, Chem. – Eur. J., 1997, 3, 219–225 CrossRef CAS PubMed.
- F. C. De Schryver, T. Vosch, M. Cotlet, M. Van Der Auweraer, K. Müllen and J. Hofkens, Energy Dissipation in Multichromophoric Single Dendrimers, Acc. Chem. Res., 2005, 38, 514–522 CrossRef CAS.
- Y. Avlasevich, C. Li and K. Müllen, Synthesis and applications of core-enlarged perylene dyes, J. Mater. Chem., 2010, 20, 3814 RSC.
- V. Medabalmi, M. Sundararajan, V. Singh, M.-H. Baik and H. R. Byon, Naphthalene diimide as a two-electron anolyte for aqueous and neutral pH redox flow batteries, J. Mater. Chem. A, 2020, 8, 11218–11223 RSC.
- M. Al Kobaisi, S. V. Bhosale, K. Latham, A. M. Raynor and S. V. Bhosale, Functional Naphthalene Diimides: Synthesis, Properties, and Applications, Chem. Rev., 2016, 116, 11685–11796 CrossRef.
- X. Wang, B. Xu and W. Tian, A new function of photochromic spiropyran: an efficient photoinitiator for two-photon polymerization, Light: Sci. Appl., 2023, 12, 140 CrossRef CAS.
- Y. Yang, Y. Chen, Y. Li, Z. Wang and H. Zhao, Acid-, mechano- and photochromic molecular switches based on a spiropyran derivative for rewritable papers, Mater. Chem. Front., 2022, 6, 916–923 RSC.
- Z. Wang, Z. Ding, Y. Yang, L. Hu, W. Wu, Y. Gao, Y. Wei, X. Zhang and G. Jiang, Time-resolved encryption via photochromic and mechanochromic system based on silane-substituted spiropyran, Chem. Eng. J., 2023, 457, 141293 CrossRef CAS.
- Y. Li, B. Xue, J. Yang, J. Jiang, J. Liu, Y. Zhou, J. Zhang, M. Wu, Y. Yuan, Z. Zhu, Z. J. Wang, Y. Chen, Y. Harabuchi, T. Nakajima, W. Wang, S. Maeda, J. P. Gong and Y. Cao, Azobenzene as a photoswitchable mechanophore, Nat. Chem., 2024, 16, 446–455 CrossRef CAS PubMed.
- M. Yamauchi, K. Yokoyama, N. Aratani, H. Yamada and S. Masuo, Crystallization-Induced Emission of Azobenzene Derivatives, Angew. Chem., Int. Ed., 2019, 58, 14173–14178 CrossRef CAS PubMed.
- Z. Wu, R. Xue, M. Xie, X. Wang, Z. Liu, M. Drechsler, J. Huang and Y. Yan, Self-Assembly-Triggered Cis-to-Trans Conversion of Azobenzene Compounds, J. Phys. Chem. Lett., 2018, 9, 163–169 CrossRef CAS PubMed.
- H. Tian and S. Wang, Photochromic bisthienylethene as multi-function switches, Chem. Commun., 2007, 781–792 RSC.
- H. Tian and Y. Feng, Next step of photochromic switches, J. Mater. Chem., 2008, 18, 1617 RSC.
- C. P. Harvey and J. D. Tovar, Main-chain photochromic conducting polymers, Polym. Chem., 2011, 2, 2699 RSC.
- J. Zhang, Q. Zou and H. Tian, Photochromic Materials: More Than Meets The Eye, Adv. Mater., 2013, 25, 378–399 CrossRef CAS PubMed.
- L.-Z. Cai, Z.-Z. Yao, S.-J. Lin, M.-S. Wang and G.-G. Guo, Photoinduced Electron-Transfer (PIET) Strategy for Selective Adsorption of CO2 over C2H2 in a MOF, Angew. Chem., 2021, 133, 18371–18378 CrossRef.
- R. Huo, T. Zhang, G. Zeng, C. Wang, Y. H. Xing and F. Y. Bai, Construction of Naphthalenediimide Lanthanide(III)-MOFs and Composites Incorporated Electron-Rich Pyrene Derivative as Multifunctional Fluorescence Sensing for Nitro Aromatic Compounds and Aldehydes, Chin. J. Chem., 2024, 42, 283–293 CrossRef CAS.
- T. Gong, Q. Sui, P. Li, X.-F. Meng, L.-J. Zhou, J. Chen, J. Xu, L. Wang and E.-Q. Gao, Versatile and Switchable Responsive Properties of a Lanthanide-Viologen Metal–Organic Framework, Small, 2019, 15, 1803468 CrossRef PubMed.
- Y. Yan, N.-N. Zhang, L. M. Tauche, K. Thangavel, A. Pöppl and H. Krautscheid, Direct synthesis of a stable radical doped electrically conductive coordination polymer, Inorg. Chem. Front., 2022, 9, 5016–5023 RSC.
- N.-N. Zhang, Y.-N. Zhang, L. Li, Z.-Y. Li, Y.-T. Liu, Y. Dong, Y. Yan and M.-S. Wang, Photochromism and single-component white light emission from a metalloviologen complex based on 1,5-naphthyridine, Dalton Trans., 2024, 53, 6547–6555 RSC.
- H. Zheng, C. Wang, X. Zhang, Y. Li, H. Ma and Y. Liu, Control over energy level match in Keggin polyoxometallate-TiO2 microspheres for multielectron photocatalytic reactions, Appl. Catal., 2018, 234, 79–89 CrossRef CAS.
- M. Rouhani, J. Hobley, G. S. Subramanian, I. Y. Phang, Y. L. Foo and S. Gorelik, The influence of initial stoichiometry on the mechanism of photochromism of molybdenum oxide amorphous films, Sol. Energy Mater. Sol. Cells, 2014, 126, 26–35 CrossRef CAS.
- M. A. Shirif, M. Medhat, S. Y. El-Zaiat, A. M. Fayad and F. A. Moustafa, Optical Properties of Silver Halide Photochromic Glasses Doped with Cobalt Oxide, Silicon, 2018, 10, 219–227 CrossRef CAS.
- Q. Li, Q. Zhang, Z.-Z. Xue, J.-X. Hu and G.-M. Wang, Photoactive Anthracene-9,10-dicarboxylic Acid for Tuning of Photochromism in the Cd/Zn Coordination Polymers, Inorg. Chem., 2022, 61, 10792–10800 CrossRef CAS PubMed.
- M.-S. Wang, G. Xu, Z.-J. Zhang and G.-C. Guo, Inorganic–organic hybrid photochromic materials, Chem. Commun., 2010, 46, 361–376 RSC.
- G. Li, L. Xu, W. Zhang, K. Zhou, Y. Ding, F. Liu, X. He and G. He, Narrow–Bandgap Chalcogenoviologens for Electrochromism and Visible–Light–Driven Hydrogen Evolution, Angew. Chem., Int. Ed., 2018, 57, 4897–4901 CrossRef CAS PubMed.
- G. Sun, Y. Wei, Z. Zhang, J. Lin, Z. Liu, W. Chen, J. Su, P. Chou and H. Tian, Diversified Excited–State Relaxation Pathways of Donor–Linker–Acceptor Dyads Controlled by a Bent–to–Planar Motion of the Donor, Angew. Chem., Int. Ed., 2020, 59, 18611–18618 CrossRef CAS PubMed.
- Z.-W. Chen, G. Lu, P.-X. Li, R.-G. Lin, L.-Z. Cai, M.-S. Wang and G.-C. Guo, Influence of Supramolecular Interactions on Electron-Transfer Photochromism of the Crystalline Adducts of 4,4′-Bipyridine and Carboxylic Acids, Cryst. Growth Des., 2014, 14, 2527–2531 CrossRef CAS.
- F. M. Raymo and M. Tomasulo, Electron and energy transfer modulation with photochromic switches, Chem. Soc. Rev., 2005, 34, 327 RSC.
- L. Liu, Y. Cotelle, J. Klehr, N. Sakai, T. R. Ward and S. Matile, Anion-π catalysis: bicyclic products with four contiguous stereogenic centers from otherwise elusive diastereospecific domino reactions on π-acidic surfaces, Chem. Sci., 2017, 8, 3770–3774 RSC.
- G. Andric, J. F. Boas, A. M. Bond, G. D. Fallon, K. P. Ghiggino, C. F. Hogan, J. A. Hutchison, M. A.-P. Lee, S. J. Langford, J. R. Pilbrow, G. J. Troup and C. P. Woodward, Spectroscopy of Naphthalene Diimides and Their Anion Radicals, Aust. J. Chem., 2004, 57, 1011 CrossRef CAS.
- S. Guha and S. Saha, Fluoride Ion Sensing by an Anion−π Interaction, J. Am. Chem. Soc., 2010, 132, 17674–17677 CrossRef CAS PubMed.
- S. Guha, F. S. Goodson, S. Roy, L. J. Corson, C. A. Gravenmier and S. Saha, Electronically Regulated Thermally and Light-Gated Electron Transfer from Anions to Naphthalenediimides, J. Am. Chem. Soc., 2011, 133, 15256–15259 CrossRef CAS PubMed.
- X. Kuang, L. Meng and C.-Z. Lu, Unveiling electron transfer in a supramolecular aggregate for adaptive and autonomous photochromic response, iScience, 2021, 24, 102956 CrossRef CAS PubMed.
- Y.-S. Shi, D.-D. Yang, H.-W. Zheng, Q.-F. Liang, Y.-H. Fang, T. Xiao and X.-J. Zheng, Solvent-Modulated Self-Assembly of Naphthalenediimide-Based Cd(II) Complexes and the Controllable Photochromism via Conformational Isomerization, Inorg. Chem., 2022, 61, 15973–15982 CrossRef CAS PubMed.
- M.-L. Wang, C. Fu, L. Li and H. Zhang, A 2D photochromic zinc-based metal–organic framework with naphthalene diimide-type chromophore, Inorg. Chem. Commun., 2018, 94, 142–145 CrossRef CAS.
- J.-J. Liu, Y.-J. Hong, Y.-F. Guan, M.-J. Lin, C.-C. Huang and W.-X. Dai, Lone pair-π interaction-induced generation of non-interpenetrated and photochromic cuboid 3-D naphthalene diimide coordination networks, Dalton Trans., 2015, 44, 653–658 RSC.
- C. F. Leong, B. Chan, T. B. Faust, P. Turner and D. M. D'Alessandro, Electronic, Optical, and Computational Studies of a Redox-Active Napthalenediimide-Based Coordination Polymer, Inorg. Chem., 2013, 52, 14246–14252 CrossRef CAS PubMed.
- X. Kuang, S. Chen, L. Meng, J. Chen, X. Wu, G. Zhang, G. Zhong, T. Hu, Y. Li and C.-Z. Lu, Supramolecular aggregation of a redox-active copper-naphthalenediimide network with intrinsic electron conduction, Chem. Commun., 2019, 55, 1643–1646 RSC.
- M.-H. You, M.-H. Li, J.-Y. Liu and M.-J. Lin, Controlling molecular packing via diffusion methods for enhanced photochromic properties in D-A hybrid heterostructures, Dyes Pigm., 2021, 186, 109027 CrossRef CAS.
- H.-H. Zhu, P.-F. Hao, Q. Shen, J.-J. Shen, G.-P. Li, G.-Z. Zhao, H.-Y. Xing and Y.-L. Fu, The modulation effect of electron-rich solvents on the supramolecular networks and photochromic properties of naphthalene diimide molecules, CrystEngComm, 2021, 23, 3356–3363 RSC.
- J.-J. Liu, Y.-F. Guan, Y. Chen, M.-J. Lin, C.-C. Huang and W.-X. Dai, The impact of lone pair–π interactions on photochromic properties in 1-D naphthalene diimide coordination networks, Dalton Trans., 2015, 44, 17312–17317 RSC.
- J.-J. Liu, S.-B. Xia, Y.-L. Duan, T. Liu, F.-X. Cheng and C.-K. Sun, Anion-Controlled Architecture and Photochromism of Naphthalene Diimide-Based Coordination Polymers, Polymers, 2018, 10, 165 CrossRef PubMed.
- B.-H. Gao, P.-F. Hao, G.-P. Li, J.-J. Shen and Y.-L. Fu, An electron-deficient naphthalene diimide-based metal–organic framework for detecting electron-rich molecules through photo-/chemo-induced chromism, Dalton Trans., 2021, 50, 13993–14000 RSC.
- J.-J. Liu, J.-J. Fu, X. Shen, T. Liu and F.-X. Cheng, The effect of dicarboxylic acid isomer on the photochromism of naphthalenediimide-based metal-organic frameworks, J. Mol. Struct., 2022, 1265, 133346 CrossRef CAS.
- J.-Z. Liao, X.-Y. Wu, J.-P. Yong, H.-L. Zhang, W.-B. Yang, R. Yu and C.-Z. Lu, Anion−π Interaction-Directed Assembly of Polyoxometalate-Based Host–Guest Compounds and Its Contribution to Photochromism, Cryst. Growth Des., 2015, 15, 4952–4958 CrossRef CAS.
- J.-J. Liu, C. He, T. Liu, J. Liu and S.-B. Xia, Two photochromic hybrid materials assembled from naphthalene diimide as photocatalysts for the degradation of carcinogenic dye basic red 9 under visible light, J. Mol. Struct., 2021, 1243, 130804 CrossRef CAS.
- M.-Q. Zhu, L. Zhu, J. J. Han, W. Wu, J. K. Hurst and A. D. Q. Li, Spiropyran-Based Photochromic Polymer Nanoparticles with Optically Switchable Luminescence, J. Am. Chem. Soc., 2006, 128, 4303–4309 CrossRef CAS PubMed.
- J.-J. Liu, Three 1D coordination polymers based on bipyridinium carboxylate ligands: Photochromism, Dyes Pigm., 2019, 160, 476–482 CrossRef CAS.
- M. Du, X.-H. Bu, Z. Huang, S.-T. Chen and Y.-M. Guo, From metallacyclophanes to 1-D coordination polymers: Role of anions in self-assembly processes of copper(II) and 2,5-bis(3-pyridyl)-1,3,4-oxadiazole, Inorg. Chem., 2003, 42, 552–559 CrossRef CAS PubMed.
- D. Tanaka and S. Kitagawa, Template effects in porous coordination polymers, Chem. Mater., 2008, 20, 922–931 CrossRef CAS.
- J.-R. Li, J. Sculley and H.-C. Zhou, Metal–Organic Frameworks for Separations, Chem. Rev., 2012, 112, 869–932 CrossRef CAS PubMed.
- T. Fu, Y.-L. Wei, C. Zhang, L.-K. Li, X.-F. Liu, H.-Y. Li and S.-Q. Zang, A viologen-based multifunctional Eu-MOF: photo/electro-modulated chromism and luminescence, Chem. Commun., 2020, 56, 13093–13096 RSC.
- N. Sikdar, K. Jayaramulu, V. Kiran, K. V. Rao, S. Sampath, S. J. George and T. K. Maji, Redox–Active Metal–Organic Frameworks: Highly Stable Charge–Separated States through Strut/Guest–to–Strut Electron Transfer, Chem. – Eur. J., 2015, 21, 11701–11706 CrossRef CAS PubMed.
- J. Chen, S. Shen, Q. Liu and J. Liu, Photochromic Zn-MOFs derived from a flexible viologen carboxylate ligand and different dicarboxylic acids, J. Mol. Struct., 2024, 1318, 139278 CrossRef CAS.
- J.-J. Liu, S.-B. Xia, D. Liu, J. Hou, H. Suo and F.-X. Cheng, Multifunctional naphthalene diimide-based coordination polymers: Photochromism and solventchromism, Dyes Pigm., 2020, 177, 108269 CrossRef CAS.
- J.-Z. Liao, H.-L. Zhang, S.-S. Wang, J.-P. Yong, X.-Y. Wu, R. Yu and C.-Z. Lu, Multifunctional Radical-Doped Polyoxometalate-Based Host–Guest Material: Photochromism and Photocatalytic Activity, Inorg. Chem., 2015, 54, 4345–4350 CrossRef CAS PubMed.
- X. Ji, M. Ahmed, L. Long, N. M. Khashab, F. Huang and J. L. Sessler, Adhesive supramolecular polymeric materials constructed from macrocycle-based host–guest interactions, Chem. Soc. Rev., 2019, 48, 2682–2697 RSC.
- N. I. Gumerova and A. Rompel, Synthesis, structures and applications of electron-rich polyoxometalates, Nat. Rev. Chem., 2018, 2, 0112 CrossRef CAS.
- M.-H. You, M.-H. Li, Y.-M. Di, Y.-W. Wang and M.-J. Lin, Switching on room-temperature phosphorescence of photochromic hybrid heterostructures by anion-π interactions, Dyes Pigm., 2020, 173, 107943 CrossRef CAS.
- G.-S. Zhang, C. Fu, H.-Y. Zhang and H. Zhang, A multifunctional colorimetric sensor originating from a cadmium naphthalene diimide-based metal-organic framework: photochromism, hydrochromism, and vapochromism, CrystEngComm, 2021, 23, 4513–4521 RSC.
- S.-M. Zhang, W.-Y. Yu, P.-F. Hao, G.-P. Li, J.-J. Shen and Y.-L. Fu, The modulation effect of auxiliary ligands on photochromic properties of 3D naphthalene diimide coordination polymers, Dalton Trans., 2023, 52, 360–365 RSC.
- C. Wang, G. Zeng, Z.-X. You, Y.-H. Xing, F.-Y. Bai and L.-X. Sun, Stimuli-Responsive Naphthalenediimide Cd-MOFs Tuned by Different Aliphatic Dicarboxylic Acids with Extended Spacers, Inorg. Chem., 2022, 61, 10066–10078 CrossRef CAS PubMed.
- M. Kang, B.-H. Gao, S.-M. Zhang, P.-F. Hao, G.-P. Li, J.-J. Shen and Y.-L. Fu, The effect of conjugation degree of aromatic carboxylic acids on electronic and photo-responsive behaviors of naphthalenediimide-based coordination polymers, Dalton Trans., 2023, 52, 12030–12037 RSC.
- S. Park, J. Lee, H. Jeong, S. Bae, J. Kang, D. Moon and J. Park, Multi-stimuli-engendered radical-anionic MOFs: Visualization of structural transformation upon radical formation, Chem, 2022, 8, 1993–2010 CAS.
- R. Huo, C. Wang, M.-Y. Wang, M.-Y. Sun, S. Jiang, Y.-H. Xing and F.-Y. Bai, Preparation of Naphthalenediimide-Decorated Electron-Deficient Photochromic Lanthanide (III)-MOF and Paper Strip as Multifunctional Recognition and Ratiometric Luminescent Turn-On Sensors for Amines and Pesticides, Inorg. Chem., 2023, 62, 6661–6673 CrossRef CAS PubMed.
- X.-B. Shang, I. Song, J. H. Lee, W. Choi, H. Ohtsu, G. Y. Jung, J. Ahn, M. Han, J. Y. Koo, M. Kawano, S. K. Kwak and J. H. Oh, Heterochiral
Doped Supramolecular Coordination Networks for High-Performance Optoelectronics, ACS Appl. Mater. Interfaces, 2019, 11, 20174–20182 CrossRef CAS.
- S. Zhang, P.-F. Hao, Y. Zhang, G. Li, J. Shen and Y.-L. Fu, The simultaneous modulation effect of N -substituents on the photochromic and electrochromic properties of naphthalenediimide-based coordination polymers, Inorg. Chem. Front., 2024, 11, 1226–1237 RSC.
- X.-Y. Wu, H.-L. Zhang, S.-S. Wang, W. Wu, L. Lin, X.-Y. Jiang and C.-Z. Lu, Polyoxometalate-based room-temperature phosphorescent materials induced by anion-π interactions, Dalton Trans., 2020, 49, 3408–3412 RSC.
- W.-J. Dai, C.-X. He, S.-R. Li, Y.-R. Xu, F.-X. Cheng and J.-J. Liu, A persistent radical anion naphthalenediimide-based hybrid material for near-infrared photothermal conversion and photocatalytic oxidative organic transformations, Inorg. Chem. Front., 2024, 11, 5185–5195 RSC.
- Z.-X. You, Y. Xiao, T. Zhang, Q.-L. Guan, Y.-H. Xing and F.-Y. Bai, Design and Construction of the Uranyl Coordination Polymer with Multifunction Stimulus Response: Fluorescent Sensors for Halide Ions and Photochromism, Inorg. Chem., 2024, 63, 9823–9830 CrossRef CAS.
- W. Dai, W. Wei, Z. Yao, S. Xiang and Z. Zhang, A photochromic NDI-based framework for the facile hydrazine sensor, Inorg. Chem. Commun., 2022, 141, 109497 CrossRef CAS.
- Z. You, C. Wang, Y. Xiao, Q. Guan, J. Li, Y. Xing, H. Gao, L. Sun and F. Bai, Integrated Photoresponsive Alkaline Earth Metal Coordination Networks: Synthesis, Topology, Photochromism and Photoluminescence Investigation, Chem. – Eur. J., 2021, 27, 9605–9619 CrossRef CAS PubMed.
- G. Radha, S. Roy, C. Chakraborty and H. Aggarwal, Electrochromic and photochromic behaviour in a single metal–organic framework containing a redox-active linker, Chem. Commun., 2022, 58, 4024–4027 RSC.
- Y.-X. Xie, W.-N. Zhao, G.-C. Li, P.-F. Liu and L. Han, A Naphthalenediimide-Based Metal–Organic Framework and Thin Film Exhibiting Photochromic and Electrochromic Properties, Inorg. Chem., 2016, 55, 549–551 CrossRef CAS.
- Y. Zhou, L. Han and W.-J. Chen, Inter-ligand charge-transfer interactions in a photochromic and redox active zinc-organic framework, CrystEngComm, 2021, 23, 5982–5988 RSC.
- J.-Z. Liao, L.-Y. Meng, J.-H. Jia, D. Liang, X.-L. Chen, R.-M. Yu, X.-F. Kuang and C.-Z. Lu, Anion-π Interaction–Induced Room–Temperature Phosphorescence of a Polyoxometalate–Based Charge–Transfer Hybrid Material, Chem. – Eur. J., 2018, 24, 10498–10502 CrossRef CAS.
- Y.-M. Di, M.-H. Li, M.-H. You, S.-Q. Zhang and M.-J. Lin, Photochromic and Room Temperature Phosphorescent Donor–Acceptor Hybrid Crystals Regulated by Core-Substituted Naphthalenediimides, Inorg. Chem., 2021, 60, 16233–16240 CrossRef CAS PubMed.
- Y.-M. Di, M.-H. Li, S.-Q. Zhang, M.-H. You and M.-J. Lin, Photochromic and Room-Temperature Phosphorescent D–A Hybrid Crystals Induced by Anion−π Interactions, Cryst. Growth Des., 2021, 21, 3511–3520 CrossRef CAS.
- Z. Li, J. Guo, F. Xiang, Q. Lin, Y. Ye, J. Zhang, S. Chen, Z. Zhang and S. Xiang, Photochromic naphthalene diimide Cd-MOFs based on different second dicarboxylic acid ligands, CrystEngComm, 2018, 20, 7567–7573 RSC.
- G.-P. Li, J. Zhang, W.-W. Ren, S.-N. Wang, Y.-X. Wang, Y.-L. Fu and Y.-Y. Wang, Electron density effect of aromatic carboxylic acids in naphthalenediimide-based coordination polymers: from thermal electron transfer and charge transfer to photoinduced electron transfer, Dalton Trans., 2023, 52, 16184–16188 RSC.
- H. C. Gulbalkan, Z. P. Haslak, C. Altintas, A. Uzun and S. Keskin, Assessing CH4/N2 separation potential of MOFs, COFs, IL/MOF, MOF/Polymer, and COF/Polymer composites, Chem. Eng. J., 2022, 428, 131239 CrossRef CAS.
- L. Cai, X.-Y. Yu, M.-S. Wang, D. Yuan, W. Chen, M. Wu and G.-C. Guo, In Situ Stimulus Response Study on the Acetylene/Ethylene Purification Process in MOFs, Angew. Chem., Int. Ed., 2024, e202417072 Search PubMed.
- L. Gu, H. Shi, M. Gu, K. Ling, H. Ma, S. Cai, L. Song, C. Ma, H. Li, G. Xing, X. Hang, J. Li, Y. Gao, W. Yao, Z. Shuai, Z. An, X. Liu and W. Huang, Dynamic Ultralong Organic Phosphorescence by Photoactivation, Angew. Chem., 2018, 130, 8561–8567 CrossRef.
- J. Wang, Z. Huang, X. Ma and H. Tian, Visible–Light–Excited Room–Temperature Phosphorescence in Water by Cucurbit[8]uril–Mediated Supramolecular Assembly, Angew. Chem., 2020, 132, 10014–10019 CrossRef.
- S. Guha, F. S. Goodson, L. J. Corson and S. Saha, Boundaries of Anion/Naphthalenediimide Interactions: From Anion−π Interactions to Anion-Induced Charge-Transfer and Electron-Transfer Phenomena, J. Am. Chem. Soc., 2012, 134, 13679–13691 CrossRef CAS PubMed.
- Y. Chen, J.-J. Liu, C.-R. Fan, J.-Q. Li and M.-J. Lin, The catassembled generation of naphthalene diimide coordination networks with lone pair-π interactions, Sci. China: Chem., 2016, 59, 1492–1497 CrossRef CAS.
- J.-Z. Liao, J.-F. Chang, L. Meng, H.-L. Zhang, S.-S. Wang and C.-Z. Lu, Lone pair-π interaction-induced generation of photochromic coordination networks with photoswitchable conductance, Chem. Commun., 2017, 53, 9701–9704 RSC.
- J.-Z. Liao, Y. Jiang, F.-F. He, L.-L. Jiang, X.-M. Zhu and H. Ke, Photo-induced organic radical species in naphthalenediimide-based metal-organic framework for reversible photochromism and near-infrared photothermal conversion, Mater. Today Chem., 2023, 27, 101324 CrossRef CAS.
- W.-J. Xu, B.-X. Huang, G. Li, F. Yang, W. Lin, J.-X. Gu, H.-G. Deng, Z.-G. Gu and H.-G. Jin, Donor–Acceptor Mixed-Naphthalene Diimide-Porphyrin MOF for Boosting Photocatalytic Oxidative Coupling of Amines, ACS Catal., 2023, 13, 5723–5732 CrossRef CAS.
- P. Singh, A. Hirsch and S. Kumar, Perylene diimide-based chemosensors emerging in recent years: From design to sensing, TrAC, Trends Anal. Chem., 2021, 138, 116237 CrossRef CAS.
- S. Matile, A. V. Jentzsch, J. Montenegro and A. Fin, Recent synthetic transport systems, Chem. Soc. Rev., 2011, 40, 2453 RSC.
- S. K. Keshri, D. Asthana, S. Chorol, Y. Kumar and P. Mukhopadhyay, Appending Diverse π–Extended Acceptors with Tetrathiafulvalene/Dithiafulvalene Donors: Multistate Redox Properties, Radical Ion Generation and Mid–IR–Absorbing Mixed–Valence States, Chem. – Eur. J., 2018, 24, 1821–1832 CrossRef CAS PubMed.
- C.-H. Luo, Z.-T. Shen, X.-J. Meng, L. Han, S. Sun, T. Lin, J.-L. Sun, H. Peng and J.-H. Chu, Design and synthesis of pyromellitic diimide-based donor-acceptor conjugated polymers for photovoltaic application, Polym. Adv. Technol., 2014, 25, 809–815 CrossRef CAS.
- Q. Shi, J. Wu, X. Wu, A. Peng and H. Huang, Perylene Diimide–Based Conjugated Polymers for All–Polymer Solar Cells, Chem. – Eur. J., 2020, 26, 12510–12522 CrossRef CAS.
- P.-F. Hao, Y. Xu, J. Shen and Y.-L. Fu, Effect of positional isomerism on electron-transfer photochromism and photoluminescence of two pyromellitic diimide-based organic molecules, Dyes Pigm., 2021, 186, 108941 CrossRef CAS.
- G.-P. Li, H.-F. Xie, P.-F. Hao, Y.-L. Fu, K. Zhang, J.-J. Shen and Y.-Y. Wang, Size Effect of Arylenediimide π-Conjugate Systems on the Photoresponsive Behaviors in Eu3+-Based Coordination Polymers, Inorg. Chem., 2022, 61, 6403–6410 CrossRef CAS.
- B. Lü, Y. Chen, P. Li, B. Wang, K. Müllen and M. Yin, Stable radical anions generated from a porous perylenediimide metal-organic framework for boosting near-infrared photothermal conversion, Nat. Commun., 2019, 10, 767 CrossRef.
- M.-H. You, M.-H. Li, Y.-M. Di, S.-Q. Zhang and M.-J. Lin, Photochromic Polyoxometalate/Perylenediimide Donor–Acceptor Hybrid Crystals with Interesting Luminescent Properties, Inorg. Chem., 2022, 61, 105–112 CrossRef CAS PubMed.
- S. Kumar, M. R. Ajayakumar, G. Hundal and P. Mukhopadhyay, Extraordinary Stability of Naphthalenediimide Radical Ion and Its Ultra-Electron-Deficient Precursor: Strategic Role of the Phosphonium Group, J. Am. Chem. Soc., 2014, 136, 12004–12010 CrossRef CAS PubMed.
- E. Castaldelli, K. D. G. I. Jayawardena, D. C. Cox, G. J. Clarkson, R. I. Walton, L. Le-Quang, J. Chauvin, S. R. P. Silva and G. J.-F. Demets, Electrical semiconduction modulated by light in a cobalt and naphthalene diimide metal-organic framework, Nat. Commun., 2017, 8, 2139 CrossRef.
- C. Li, Z. Lin, Y. Li and Z.-H. Wang, Synthesis and Applications of π-Extended Naphthalene Diimides, Chem. Rec., 2016, 16, 873–885 CrossRef CAS PubMed.
- J.-H. Lu, B.-H. Jiang, F.-C. Hsiao, Y.-C. Peng, Y.-W. Su, Y.-R. Lin, T.-H. Tsai, M.-N. Shiu, C.-Y. Lin, Y.-T. Fang and C.-P. Chen, High-Performance organic photodiodes for Blue-Light hazard detection, Chem. Eng. J., 2022, 437, 135327 CrossRef CAS.
- J. Wang, A. S. Jeevarathinam, A. Jhunjhunwala, H. Ren, J. Lemaster, Y. Luo, D. P. Fenning, E. E. Fullerton and J. V. Jokerst, A Wearable Colorimetric Dosimeter to Monitor Sunlight Exposure, Adv. Mater., 2018, 3, 1800037 Search PubMed.
- Y. Zhang, H. Liu, S. Liu, Q. Gong, Y.-S. Liu, D. Tian and L.-X. Kang, Narrowband photoresponse of a self-powered CuI/SrTiO3 purple light detector with an ultraviolet-shielding effect, J. Mater. Chem. C, 2023, 11, 127–133 RSC.
- Y.-S. Shi, D.-D. Yang, T. Xiao, Y.-H. Fang, Z.-G. Xia and X.-J. Zheng, Naphthalenediimide-based photochromic MOFs: Structure visualization upon radical formation, and applications in purple light detection, inkless printing and anti-counterfeiting, Chem. Eng. J., 2023, 462, 142275 CrossRef CAS.
- K. Hiramoto, S. Kubo, K. Tsuji, D. Sugiyama and H. Hamano, Induction of Skin Cancer by Long-Term Blue Light Irradiation, Biomedicines, 2023, 11, 2321 CrossRef CAS PubMed.
- K.-T. Shih, J.-S. Liu, F. Shyu, S.-L. Yeh and H. H. Chen, Blocking harmful blue light while preserving image color appearance, ACM Trans. Graphics, 2016, 36, 1–10 Search PubMed.
- G.-H. Huang, Y.-P. Huang, W. Xu, Q.-H. Yao, X.-F. Liu, C.-F. Ding and X. Chen, Cesium lead halide perovskite nanocrystals for ultraviolet and blue light blocking, Chin. Chem. Lett., 2019, 30, 1021–1023 CrossRef CAS.
- P.-F. Hao, B.-H. Gao, G.-P. Li, J.-J. Shen and Y.-L. Fu, Ultrafast visible-light photochromic properties of naphthalenediimide-based coordination polymers for the visual detection/filtration of blue light, Inorg. Chem. Front., 2022, 9, 2852–2861 RSC.
- A. A. Koutinas, A. Vlysidis, D. Pleissner, N. Kopsahelis, I. L. Garcia, I. K. Kookos, S. Papanikolaou, T. H. Kwan and C. S. K. Lin, Valorization of industrial waste and by-product streams via fermentation for the production of chemicals and biopolymers, Chem. Soc. Rev., 2014, 43, 2587 RSC.
- S. Hassanzadeh, N. Aminlashgari and M. Hakkarainen, Microwave-Assisted Recycling of Waste Paper to Green Platform Chemicals and Carbon Nanospheres, ACS Sustainable Chem. Eng., 2015, 3, 177–185 CrossRef CAS.
- V. Nagy, I. Suleimanov, G. Molnár, L. Salmon, A. Bousseksou and L. Csóka, Cellulose-spin crossover particle composite papers with reverse printing performance: a proof of concept, J. Mater. Chem. C, 2015, 3, 7897–7905 RSC.
- H. Nishi, T. Namari and S. Kobatake, Photochromic polymers bearing various diarylethene chromophores as the pendant: synthesis, optical properties, and multicolor photochromism, J. Mater. Chem., 2011, 21, 17249 RSC.
- T. Panda, D. K. Maiti and M. K. Panda, Inkless Writing and Self-Erasing Security Feature of (Z)-1,2-Diarylacrylonitrile-Based Materials: A Confidential Data Communication, ACS Appl. Mater. Interfaces, 2018, 10, 29100–29106 CrossRef CAS.
- Y.-T. Yu, S.-H. Li, Z.-Y. Li, J.-G. Zeng, C.-J. Liu and L. Li, A novel viologen-based hybrid crystalline material for photochromic glass films, information storage and anti-counterfeiting, Dalton Trans., 2024, 53, 17565–17570 RSC.
- B. Garai, A. Mallick and R. Banerjee, Photochromic metal-organic frameworks for inkless and erasable printing, Chem. Sci., 2016, 7, 2195–2200 RSC.
- W.-G. Lu, Z.-W. Wei, Z.-Y. Gu, T.-F. Liu, J. Park, J. Park, J. Tian, M. Zhang, Q. Zhang, T. Gentle III, M. Bosch and H.-C. Zhou, Tuning the structure and function of metal-organic frameworks via linker design, Chem. Soc. Rev., 2014, 43, 5561–5593 RSC.
- J.-K. Sun, L.-X. Cai, Y.-J. Chen, Z.-H. Li and J. Zhang, Reversible luminescence switch in a photochromic metal–organic framework, Chem. Commun., 2011, 47, 6870 RSC.
- J.-P. Zhang, Y.-B. Zhang, J.-B. Lin and X.-M. Chen, Metal Azolate Frameworks: From Crystal Engineering to Functional Materials, Chem. Rev., 2012, 112, 1001–1033 CrossRef CAS.
- K.-B. Wang, R. Bi, M.-L. Huang, B. Lv, H.-J. Wang, C. Li, H. Wu and Q.-C. Zhang, Porous Cobalt Metal-Organic Frameworks as Active Elements in Battery–Supercapacitor Hybrid Devices, Inorg. Chem., 2020, 59, 6808–6814 CrossRef CAS PubMed.
- W.-B. Li, X.-H. Chen, J.-Z. Chen, R. Huang, J.-W. Ye, L. Chen, H.-P. Wang, T. Yang, L.-Y. Tang, J. Bai, Z.-W. Mo and X.-M. Chen, Photochromic Metal-Organic Framework for High-Resolution Inkless and Erasable Printing, ACS Appl. Mater. Interfaces, 2022, 14, 8458–8463 CrossRef CAS PubMed.
- J.-W. Zhang, Z.-J. Wang, X.-X. Huo, X. Meng, Y. Wang, H. Suo and P.-L. Li, Anti–Counterfeiting Application of Persistent Luminescence Materials and Its Research Progress, Laser Photonics Rev., 2024, 18, 2300751 CrossRef CAS.
- J.-M. Wan, J. Xu, S.-Y. Zhu, J. Li and K.-F. Chen, Multicolor photochromic material with dual protection of anti-counterfeiting and waterproofing, Chem. Eng. J., 2023, 473, 145500 CrossRef CAS.
- Y.-H. Shi, J.-L. Han, X. Jin, W.-G. Miao, Y. Zhang and P.-F. Duan, Chiral Luminescent Liquid Crystal with Multi-State-Reversibility: Breakthrough in Advanced Anti-Counterfeiting
Materials, Adv. Sci., 2022, 9, 2201565 CrossRef CAS.
- W.-B. Dong, W.-X. Wang, M.-Y. Wang, J.-C. Zhang and Y.-H. Wang, A dynamic irradiation-responsive material for advanced anti-counterfeiting in dark and bright fields, J. Mater. Chem. C, 2022, 10, 4218–4223 RSC.
- B. Wu, X.-T. Xu, Y.-Y. Tang, X.-L. Han and G.-J. Wang, Multifunctional Optical Polymeric Films with Photochromic, Fluorescent, and Ultra-Long Room Temperature Phosphorescent Properties, Adv. Opt. Mater., 2021, 9, 2101266 CrossRef CAS.
- A. Singh, P. Verma, S. Laha, D. Samanta, S. Roy and T. K. Maji, Photochromic Conjugated Microporous Polymer Manifesting Bio-Inspired pcFRET and Logic Gate Functioning, ACS Appl. Mater. Interfaces, 2020, 12, 20991–20997 CrossRef CAS PubMed.
- J. Tang, Y. Tian, Z. Lin, C.-H. Zhang, P.-S. Zhang, R.-J. Zeng, S. Wu, X.-D. Chen and J. Chen, Supramolecular Polymers with Photoswitchable Multistate Fluorescence for Anti-Counterfeiting and Encryption, ACS Appl. Mater. Interfaces, 2023, 15, 2237–2245 CrossRef CAS PubMed.
- J.-Q. Zhang, Q.-C. Wang, L. Zou and C.-Y. Jia, Synthesis and properties of novel photochromic bisthienylethenes containing imidazole and imidazolium derivatives, Chin. Chem. Lett., 2014, 25, 762–766 CrossRef CAS.
- Y.-S. Shi, D.-D. Yang, T. Xiao, Z.-G. Xia, Y.-H. Fang and X.-J. Zheng, Photoinduced multi-color emission of naphthalenediimide radical in different solvents and dynamic anti-counterfeiting film, Chem. Eng. J., 2023, 472, 145152 CrossRef CAS.
- G. Das, B. Garai, T. Prakasam, F. Benyettou, S. Varghese, S. K. Sharma, F. Gándara, R. Pasricha, M. Baias, R. Jagannathan, N. Saleh, M. Elhabiri, M. A. Olson and A. Trabolsi, Fluorescence turn on amine detection in a cationic covalent organic framework, Nat. Commun., 2022, 13, 3904 CrossRef CAS PubMed.
- R.-J. Hu, S.-Y. Lin, D.-N. Hu, H.-Y. Huang, M.-S. Wang, R.-X. Li, M. Tian, Z.-G. Shuai and Y. Wei, “Turn-on” fluorescence sensor for organic amines fabricated via sustainable processing, Mater. Chem. Front., 2023, 7, 153–159 RSC.
- M. Kataria, J. Hwang, H. D. Chau, N. Y. Kwon, D. W. Lee, T. Kim, K. Lee, Y. Kim, S. Park and D. H. Choi, Pyrazine-based hollow spherical self-assemblies: A portable tool for detection of volatile organic amines, Sens. Actuators, B, 2021, 343, 130110 CrossRef CAS.
- T.-X. Wang, W. Liu, L. Chen and X.-X. Li, Carboxyl-functionalized covalent organic frameworks for the efficient adsorption of foodborne heterocyclic aromatic amines, Chem. Eng. J., 2023, 475, 146044 CrossRef CAS.
- Q. Sui, P. Li, N.-N. Yang, T. Gong, R. Bu and E.-Q. Gao, Differentiable Detection of Volatile Amines with a Viologen-Derived Metal–Organic Material, ACS Appl. Mater. Interfaces, 2018, 10, 11056–11062 CrossRef CAS PubMed.
- S.-L. Yao, S.-J. Liu, X.-M. Tian, T.-F. Zheng, C. Cao, C.-Y. Niu, Y.-Q. Chen, J.-L. Chen, H. Huang and H.-R. Wen, A Zn II -Based Metal–Organic Framework with a Rare tcj Topology as a Turn-On Fluorescent Sensor for Acetylacetone, Inorg. Chem., 2019, 58, 3578–3581 CrossRef CAS PubMed.
- A. Mallick, B. Garai, M. A. Addicoat, P. St. Petkov, T. Heine and R. Banerjee, Solid state organic amine detection in a photochromic porous metal organic framework, Chem. Sci., 2015, 6, 1420–1425 RSC.
- T. Du, X. Han, X.-L. Yan, J.-W. Shang, Y. Li and J.-B. Song, MXene-Based Flexible Sensors: Materials, Preparation, and Applications, Adv. Mater., 2023, 8, 2202029 CAS.
- Y.-P. Liu, L.-L. Wang, Y.-Y. Mi, S.-S. Zhao, S.-M. Qi, M. Sun, B. Peng, Q. Xu, Y.-C. Niu and Y. Zhou, Transparent stretchable hydrogel sensors: materials, design and applications, J. Mater. Chem. C, 2022, 10, 13351–13371 RSC.
- X. Shang, I. Song, G. Y. Jung, W. Choi, H. Ohtsu, J. H. Lee, J. Y. Koo, B. Liu, J. Ahn, M. Kawano, S. K. Kwak and J. H. Oh, Chiral self-sorted multifunctional supramolecular biocoordination polymers and their applications in sensors, Nat. Commun., 2018, 9, 3933 CrossRef PubMed.
- I. Cohen, B. Shapira, E. Avraham, A. Soffer and D. Aurbach, Bromide Ions Specific Removal and Recovery by Electrochemical Desalination, Environ. Sci. Technol., 2018, 52, 6275–6281 CrossRef CAS PubMed.
- F. X. R. Van Leeuwen, B. Sangster and A. G. Hildebrandt, The Toxicology of Bromide Ion, Crit. Rev. Toxicol, 1987, 18, 189–213 CrossRef CAS PubMed.
- Q. Tang, L. Deng, Y.-Y. Mao, S. Fu, W. Luo, T.-T. Huang, J. Hu and R. P. Singh, Formation and toxicity alteration of halonitromethanes from Chlorella vulgaris during UV/chloramination process involving bromide ion, J. Environ. Manage., 2024, 359, 121034 CrossRef CAS PubMed.
- J. Kim, S. Lee and M. S. Han, pH-guided fluorescent sensing probe for the discriminative detection of Cl− and Br− in human serum, Anal. Chim. Acta, 2022, 1210, 339879 CrossRef CAS PubMed.
- Y.-S. Shi, T. Xiao, D.-D. Yang, Z.-G. Xia and X.-J. Zheng, Dynamic Fluorescence Sensing of Bromide Ions by Photochromic Bi(III)–Coordination Polymers Based on a Ligand Integrated by Naphthalene Diimides and Pyridinium in Solution and Films, Small, 2024, 20, 2309076 CrossRef CAS PubMed.
|
This journal is © the Partner Organisations 2025 |
Click here to see how this site uses Cookies. View our privacy policy here.