DOI:
10.1039/D5QI00038F
(Research Article)
Inorg. Chem. Front., 2025, Advance Article
Superacidic aluminum azido species: precursors to triazolyl dimers, trimers and tetramers†
Received
5th January 2025
, Accepted 10th February 2025
First published on 13th February 2025
Abstract
The aluminum-azido species (Me3SiN3)Al(C6F5)3 thermally converts to [(C6F5)2Al(μ-N3)]2 2. Further reactions with Me3SiN3 afford [(C6F5)2Al(μ-N3)2]3Al which contains unique four Al centers bound to six bridging azides. Compound 2 is shown to be a Lewis superacid both experimentally and theoretically, forming dimeric products in reactions with diphenylphosphine oxide or tetrahydrofuran and a monomeric adduct with an NHC donor. Compound 2 also undergoes “click” reactions with alkynes to give dimeric, trimeric and tetrameric products depending on the nature of the substitution on the alkyne, which demonstrates the ability of the reagent to control the nature of aggregation.
Introduction
Since the first synthesis of azido benzene by Griess in 1864,1,2 organic azides have been extensively studied for applications in organic synthesis, bioorthogonal chemistry and materials science.3–13 Polyazido compounds are high-energy materials thanks to the high nitrogen content and the energetically favorable release of N2.14 As a result they often exhibit poor thermal stability and are sensitive to mechanical stimuli.15–20 Thus, a major theme in the study of azido compounds has been to probe their reactivity and stability.11,19,21–23 The incorporation of strongly Lewis acidic or steric bulky moieties has been shown to significantly increase the stability of azido compounds, leading to the formation of isolable molecular species.24–26 While monoazido boranes such as R(3−n)B(N3)n19,27 1-azido-2,3,4,5-tetraphenylborole28 and carbene-stabilized azidoborane,29–31 have been reported, more electrophilic boranes generally form more diverse molecular structures. For example, the trimers (Cl2B(μ-N3))3 and (Br2B(μ-N3))332,33 were reported in the 1960s, while Klapoke reported the dimer ((C6F5)2B(μ-N3))224,25 in 2000. Subsequently Bettinger et al. described the trimeric 9-azido-9-borafluorene (Scheme 1)34,35 while Braunschweig et al. synthesized the diborane(4) diazides (Scheme 1).36,37 The reactivity of borane–azides has drawn limited attention. We previously reported the use of ((C6F5)2B(μ-N3))2 in uncatalyzed “click” reactions with various alkynes.38–40 Ye et al. reported the reactivity of the super Lewis acid carborane-derived 9,10-diazido-9,10-dibora-anthracene (Scheme 1)21 with B–H, Si–H and Ge–H bonds. Most recently, Guan, Lin, Shi and co-workers published the use of bis-azidoboranes fused by biphenylene/xanthrene (Scheme 1)41 to generate transient nitrene species for further reactions.
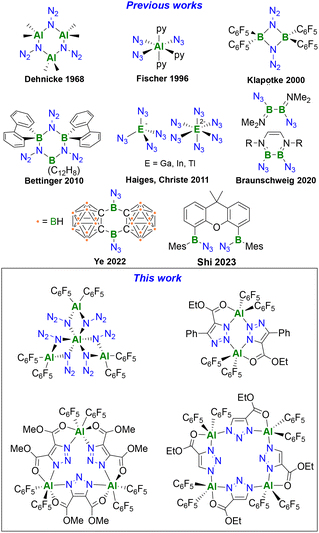 |
| Scheme 1 Selected examples of group 13 azides. | |
Heavier analogues of group 13 polyazides are generally less studied. For gallium, indium and thallium, the polyazido species M(N3)3, (CH3CN)M(N3)3 (M = Ga, In, Tl), [Ga(N3)5]2−, and [M(N3)6]3− (M = In, Tl) (Scheme 1) have been fully characterized by Haiges, Christe and co-workers (Scheme 1).42 In the case of aluminum, while the first Al-azide (Me2Al(μ-N3))3 (Scheme 1) was reported by Dehnicke in 1968, only a few other Al-azides have been described since. The Al-monoazides LAlN3 (L = [(Me3SiNCH2CH2)2NR]2−) and AriPr8Al(N3)[N(SiMe3)2] have been described by Bertrand et al.43 and Power et al.,44 respectively, while Uhl et al. and Fischer and coworkers reported the trimeric [(CH3Si)2CH2Al(μ-N3)]3
45 and the monomeric triazide py3Al(N3)3
46 (Scheme 1), respectively. Apart from the use of azido-alane Et2AlN3
47 as an N3 source in the preparation of N-rich compounds and materials,48–52 little is known about the reactivity of Al-polyazides.
We reasoned that the incorporation of strong electron withdrawing groups on aluminum would enhance the stability and Lewis acidity of polyazido species and thus favor expanded coordination geometries for aggregation. Herein, we report the preparation of aluminum-azido derivatives incorporating C6F5 substituents and demonstrated that such species provide access to polytriazolyl derivatives via “click” reactions resulting in dimeric, trimeric and tetrameric aluminum species, where the reagent determines the nature of the aggregation.
Results and discussion
We initiated our investigation with the reaction of trimethylsilylazide (Me3SiN3) and Al(C6F5)3 in a ratio of 1
:
1 at room temperature. A white solid 1 was obtained and isolated in 90% yield (Scheme 2). This species exhibited 19F NMR signals at −123.0, −150.0 and −160.1 ppm, inferring a tetracoordinated Al center based on the gap between the signal for the meta- and para-fluorines.53–55 The IR absorption peaks of 1 at 2170 and 1358 cm−1 confirm the presence of the azido moiety.56 Single crystal X-ray diffraction (XRD) analysis of the colorless crystals of 1 revealed the presence of the azide–alane adduct (Me3SiN3)Al(C6F5)3 (Fig. 1a) with a N–Al bond of 1.9925(14) Å. The two N–N bond lengths in the azido-fragment were 1.258(2) and 1.113(2) Å, which are similar to those found in the known boron analogue [1.259(1) and 1.117(2) Å].57,58
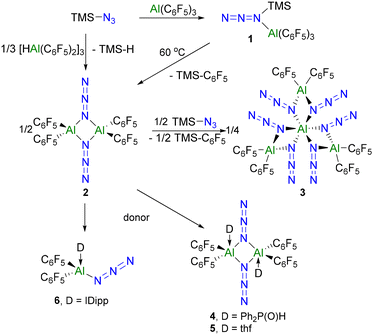 |
| Scheme 2 Synthesis of 1–6. | |
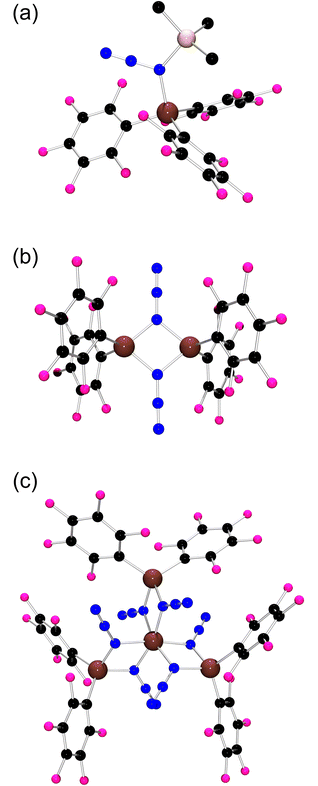 |
| Fig. 1 POV-ray depiction of (a) 1, (b) 2, and (c) 3; C: black, Al: brown, F: pink, N: blue, and Si: salmon. Hydrogen atoms have been omitted for clarity. | |
Heating 1 in a toluene solution at 60 °C for 12 h led to the observation of two new sets of peaks at −124.3, −146.7 and −157.7 ppm and −128.0, −152.4 and −161.7 ppm in the 19F NMR spectrum. The latter signals affirm the generation of Me3Si–C6F5.59 The remaining signals were attributed to 2 which was isolated as colorless crystals upon recrystallization from a toluene solution (Scheme 2). The persistence of IR absorptions at 2174 and 1365 cm−1 suggests the retention of the azido-fragment. Upon redissolution, a 19F–19F DOSY NMR spectrum confirmed a single species in solution. In addition, compound 2 was found to be unstable in dichloromethane and chloroform giving a complex mixture of products. X-ray crystallography revealed 2 as the dimer [(C6F5)2Al(μ-N3)]2 (Fig. 1b) structurally similar to the boron analog,25 in which two azido groups bridge the two Al atoms. The four Al–N bond lengths are found to be 1.914(2), 1.918(2), 1.918(2) and 1.925(2) Å.
The formation of 2 from 1 is thought to occur via a formal β-elimination of Me3SiC6F5. This notion prompted us to probe the corresponding reaction of [HAl(C6F5)2]3 with Me3SiN3. Indeed upon β-elimination of the silane, HSiMe3 was evidenced by the 1H NMR resonances at 4.17 (Si–H) and 0.01 (SiCH3) ppm and the product 2 could be isolated in 90% yield.
Heating compound 2 in the presence of additional Me3SiN3 at 80 °C for 3 days resulted in the formation of a new species 3 as evidenced by a new set of 19F NMR signals and the resonances attributable to Me3SiC6F5. Species 3 was isolated in 73% yield (Scheme 2) and showed IR absorptions at 2169 and 1360 cm−1. An X-ray crystallographic study confirmed the formulation of 3 as [(C6F5)2Al(μ-N3)2]3Al (Fig. 1c) featuring a pseudo-octahedral Al center surrounded by six azido fragments, each of which bridges three other Al centers. The twelve Al–N bond lengths range from 1.893(2) to 1.996(2) Å. Each of the three Al2N2 rings are nearly planar with the N–Al–N angles ranging from 76.23(8)° to 80.8(1)°. Compound 3 represents an unusual polyazido-alane, incorporating an unprecedented hexaazido-aluminum center.
The Lewis acidity of 2 was assessed by Gutmann–Beckett Lewis acidity test revealing an acceptor number (AN) of 82.4 for 2. This is significantly higher than that observed for B(C6F5)3 (AN = 76.0).55,60 Density functional theory (DFT) calculations performed at the BP86-D3/def2-SVP level of theory revealed that the gas-phase/solvent-corrected (toluene) isodesmic fluoride ion affinity values (FIA) of 545.1 kJ mol−1 and 387.6 kJ mol−1 exceed those of SbF5 (FIA: 483.2 kJ mol−1; FIAtoluene: 383.5 kJ mol−1). Similarly, the hydride ion affinities (HIA) of 487.7 kJ mol−1 and 324.5 kJ mol−1 for 2 are similar to those of B(C6F5)3 (HIA: 488.1 kJ mol−1; HIAtoluene: 286.4 kJ mol−1). Collectively these data suggest that 2 is a Lewis superacid,61–63 with the lowest unoccupied molecular orbital (LUMO) of 2 partly associated with the empty p-orbital on Al (Fig. 2).
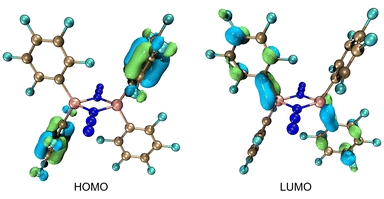 |
| Fig. 2 Selected molecular orbitals for 2 (dimer) at the M062X/def2-SVP level. Isovalue: 0.05. | |
Treatment of 2 with Ph2P(O)H afforded colorless crystals of 4 (Scheme 2) which exhibited a 31P NMR doublet at −34.5 ppm, with a P–H coupling constant of 539.2 Hz. The molecular structure of 4 (Scheme 2 and Fig. 3a) confirmed the coordination of a phosphine-oxide to each of the Al centers of 2, with ((C6F5)2Al(OP(H)Ph2)(μ-N3))2 generating five coordinate Al centers. The analogous tetrahydrofuran adduct ((C6F5)2Al(thf)(μ-N3))2, 5 was also isolated (Scheme 2, see the ESI†). In contrast, the reaction of 2 with the N-heterocyclic carbene [1,3-bis-(2,6-diisoproylphenyl)imidazol-2-ylidene] (IPr) gave the product 6 (Scheme 2) which was shown crystallographically to be the monomeric species (IPr)Al(N3)(C6F5)2 (Fig. 3b). This contrasting reactivity is thought to result from both the strong donor ability and the steric demands of the IPr ligand.
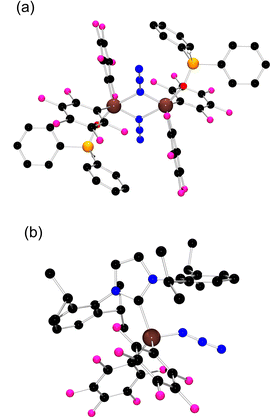 |
| Fig. 3 POV-ray depiction of (a) 4 and (b) 6; C: black, Al: brown, F: pink, N: blue, O: red, and P: orange. Hydrogen atoms have been omitted for clarity. | |
We speculated that alkynes react with 2 in a ‘click reaction’ and that the superacidity of 2 and the nature of the alkyne substitution would have an impact on the degree of aggregation. To probe this, 2 was reacted with ethyl phenylpropiolate in a toluene solution. The in situ 19F NMR spectrum showed new resonances at −123.1, −153.6 and −161.5 ppm (Scheme 3). An X-ray crystallographic study of the product 7 showed it to be the dimeric species [(C6F5)2Al(μ-EtO2CC2PhN3)]3 (Fig. 4a) in which two penta-coordinate Al centers are bridged by two triazole rings in a 1,3-fashion with Al–N bond lengths of 1.9547(14) and 2.0337(15) Å.
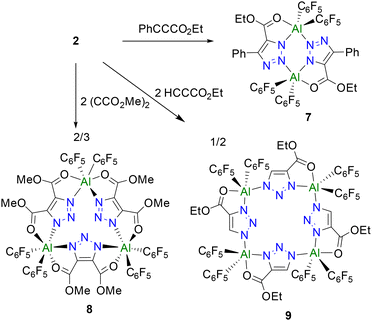 |
| Scheme 3 Synthesis of 7–9. | |
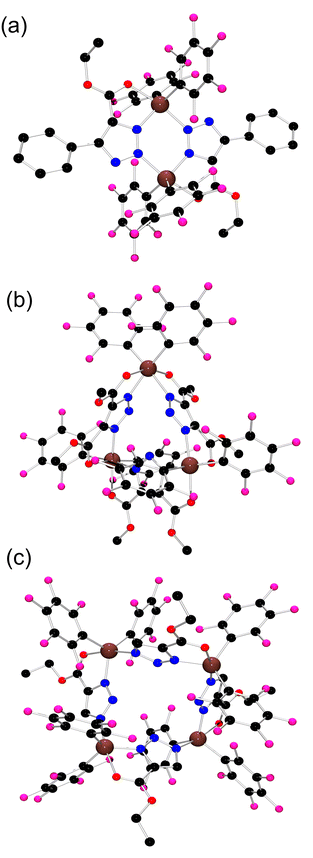 |
| Fig. 4 POV-ray depiction of (a) 7, (b) 8, and (c) 9; C: black, Al: brown, F: pink, N: blue, and O: red. Hydrogen atoms have been omitted for clarity. | |
The analogue reaction of 2 with dimethyl acetylenedicarboxylate in toluene solution led to the formation of a new species 8 which exhibited 19F NMR signals at −139.1, −154.0 and −162.3 ppm. Colourless crystals of 8 (Scheme 3) suitable for single-crystal XRD analysis were obtained by storing a C6H6/hexane solution of 8 at −30 °C. The molecular structure showed 8 to be the trimeric species ((C6F5)2Al((μ-MeO2C)2C2N3))3 (Fig. 4b). In this case, three triazole rings bridged three Al(C6F5)2 moieties with Al–N bond lengths ranging from 2.030(3) to 2.118(2) Å. The Al centers are also coordinated to C
O fragments of the ester with Al–O distances of 1.9804(19) to 2.155(2) Å generating a pseudo-octahedral Al coordination sphere.
Compound 2 also reacted with an equivalent of ethyl propiolate in a benzene solution, affording colorless crystals of 9 in a yield of 76%. This species exhibited 19F NMR signals at −123.2, 125.3, −151.7, 156.2, −160.1, and 162.9, inferring a tetracoordinated Al center with the inequivalent C6F5 groups. A single-crystal X-ray diffraction study of 9 (Scheme 3 and Fig. 4c) revealed a tetrameric formulation ((C6F5)2Al(μ-EtO2CC2HN3))4 in which the four triazole rings bridged four Al atoms in a 1, 3-fashion with Al–N bond lengths of 1.936(3) and 2.002(3) Å. Coordination of the ester's carbonyl fragment to Al affords penta-coordinate Al centers.
In contrast to boron azides which undergo “click reactions”, affording four coordinate boron centers,38–40 the present reactions of superacidic aluminum centers incorporated in 7–9 provide interactions with the carbonyl substituents to give penta- or hexa-coordinated Al centers. As a consequence, the nature of the substituents on the alkyne determines the degree of aggregation, permitting the formation of dimers, trimers and tetramers, 7–9. Thus, the super-Lewis acidity of the Al centers in 2 and the nature of the substitutions on the alkynes can be tuned to control the nature of aggregates formed.
Conclusions
In summary, we have reported the synthesis of the aluminum azido derivative 1 and its thermal conversion to 2. In addition, 2 was shown to react with excess Me3SiN3 to give 3, a species containing an unusual hexaazido-Al center. The superacidity of 2 was confirmed experimentally and computationally while reactions with donors revealed that steric demands have an impact on the nature of the resulting adducts. Exploiting compound 2 for click reactions with substituted alkynyl esters afforded oligomeric aluminum triazole derivatives 7–9. These findings demonstrate the ability of highly electron deficient aluminum-azides to access a range of structurally diverse aggregates, the nature of which is determined by the steric demands of the substitution on the alkyne. This provides a strategy to control the degree of aggregation. Further exploration of the utility of such Lewis superacidic aluminum azides in organic and materials chemistry is the subject of ongoing work.
Author contributions
S. J., J. T. and T. C. performed the experiments; S. J. performed DFT calculations; T. C., W. Y and N. X. assisted S. J. and J. T. with data collection; D. W. S. and Y. W. prepared the manuscript and managed the project.
Data availability
The data supporting this article have been included as part of the ESI.† Deposition numbers 2164107 (2), 2329987 (3), 2329988 (1), 2329989 (8), 2329990 (5), 2329991 (6), 2329992 (4), 2356544 (7), and 2359713 (9) contain the supplementary crystallographic data for this paper.†
Conflicts of interest
The authors declare no competing financial interests.
Acknowledgements
We gratefully acknowledge financial support from the National Natural Science Foundation of China (No. 22401163, 42388101 and 92256203), Ningbo Natural Science Foundation (No. 2022J108) and the Technology and Engineering Center for Space Utilization, the Chinese Academy of Sciences (No. KJZ-YY-NSM0406). We acknowledge the Analysis Center of Institute of Drug Discovery Technology for collecting spectral data.
References
- J. P. Griess and A. W. V. Hofmann, XVIII. On a new series of bodies in which nitrogen is substituted for hydrogen, Philos. Trans. R. Soc. London, 1864, 154, 667–731 CrossRef.
- J. P. Griess and A. W. V. Hofmann, XX. On a new class of compounds in which nitrogen is substituted for hydrogen, Proc. R. Soc. London, 1864, 13, 375–384 CrossRef.
- E. F. V. Scriven and K. Turnbull, Azides: their preparation and synthetic uses, Chem. Rev., 1988, 88, 297–368 CrossRef CAS.
- L.-H. Liu and M. Yan, Perfluorophenyl Azides: New Applications in Surface Functionalization and Nanomaterial Synthesis, Acc. Chem. Res., 2010, 43, 1434–1443 CrossRef CAS PubMed.
- C. Wentrup, Flash Vacuum Pyrolysis of Azides, Triazoles, and Tetrazoles, Chem. Rev., 2017, 117, 4562–4623 CrossRef CAS PubMed.
- J. C. Jewett and C. R. Bertozzi, Cu-free click cycloaddition reactions in chemical biology, Chem. Soc. Rev., 2010, 39, 1272–1279 RSC.
- P. Thirumurugan, D. Matosiuk and K. Jozwiak, Click Chemistry for Drug Development and Diverse Chemical–Biology Applications, Chem. Rev., 2013, 113, 4905–4979 CrossRef CAS PubMed.
- G. C. Tron, T. Pirali, R. A. Billington, P. L. Canonico, G. Sorba and A. A. Genazzani, Click chemistry reactions in medicinal chemistry: Applications of the 1,3-dipolar cycloaddition between azides and alkynes, Med. Res. Rev., 2008, 28, 278–308 CrossRef CAS PubMed.
- J. Lahann, Click chemistry for biotechnology and materials science, John Wiley & Sons, 2009 Search PubMed.
- M. F. Debets, C. W. J. van der Doelen, F. P. J. T. Rutjes and F. L. van Delft, Azide: A Unique Dipole for Metal-Free Bioorthogonal Ligations, ChemBioChem, 2010, 11, 1168–1184 CrossRef CAS PubMed.
- S. Xie, M. Sundhoro, K. N. Houk and M. Yan, Electrophilic Azides for Materials Synthesis and Chemical Biology, Acc. Chem. Res., 2020, 53, 937–948 CrossRef CAS PubMed.
- S. Chiba, Application of Organic Azides for the Synthesis of Nitrogen-Containing Molecules, Synlett, 2012, 21–44 CrossRef CAS.
- S. Bräse, C. Gil, K. Knepper and V. Zimmermann, Organic Azides: An Exploding Diversity of a Unique Class of Compounds, Angew. Chem., Int. Ed., 2005, 44, 5188–5240 CrossRef PubMed.
- O. T. O'Sullivan and M. J. Zdilla, Properties and Promise of Catenated Nitrogen Systems As High-Energy-Density Materials, Chem. Rev., 2020, 120, 5682–5744 CrossRef PubMed.
- I. C. Tornieporth-Oetting and T. M. Klapötke, Covalent Inorganic Azides, Angew. Chem., Int. Ed. Engl., 1995, 34, 511–520 CrossRef CAS.
- R. P. Singh, R. D. Verma, D. T. Meshri and J. N. M. Shreeve, Energetic Nitrogen-Rich Salts and Ionic Liquids, Angew. Chem., Int. Ed., 2006, 45, 3584–3601 CrossRef CAS PubMed.
- G. Zhang, G. Chen, J. Li, S. Sun, Y. Luo and X. Li, High Azide Content Hyperbranched Star Copolymer as Energetic Materials, Ind. Eng. Chem. Res., 2018, 57, 13962–13972 CrossRef CAS.
- Y. Huang, J. Gao, H. Gao, Y. Chen, Y. Zang, J. Sun and C. Zhang, Azide Polyether with a Highly Flexible Main Chain as Energetic Materials, ACS Appl. Polym. Mater., 2023, 5, 3096–3103 CrossRef CAS.
- P. Portius and M. Davis, Recent developments in the chemistry of homoleptic azido complexes of the main group elements, Coord. Chem. Rev., 2013, 257, 1011–1025 CrossRef CAS.
- T. M. Klapötke and B. Krumm, Azide-Containing High Energy Materials, in Organic Azides, ed. K. B. Stefan Bräse, 2009, pp. 389–411 Search PubMed.
- C. Zhang, X. Liu, J. Wang and Q. Ye, A Three-Dimensional Inorganic Analogue of 9,10-Diazido-9,10-Diboraanthracene: A Lewis Superacidic Azido Borane with Reactivity and Stability, Angew. Chem., Int. Ed., 2022, 61, e202205506 CrossRef CAS PubMed.
- L. Zhu and R. Kinjo, Reactions of main group compounds with azides forming organic nitrogen-containing species, Chem. Soc. Rev., 2023, 52, 5563–5606 RSC.
- F. A. Carey and R. J. Sundberg, Structural Effects on Stability and Reactivity, in Advanced Organic Chemistry: Part A: Structure and Mechanisms, Springer US, Boston, MA, 2007, pp. 253–388 Search PubMed.
- W. Fraenk, T. M. Klapötke, B. Krumm, H. Nöth, M. Suter and M. Warchhold, Oligomeric pentafluorophenylboron azides, J. Chem. Soc., Dalton Trans., 2000, 4635–4638 RSC.
- W. Fraenk, T. M. Klapötke, B. Krumm and P. Mayer, Bis(pentafluorophenyl)boron azide: synthesis and structural characterization of the first dimeric boron azide, Chem. Commun., 2000, 667–668 RSC.
- W. Fraenk and T. M. Klapötke, Theoretical studies on the thermodynamic stability and trimerization of BF2N3, J. Fluorine Chem., 2001, 111, 45–47 CrossRef CAS.
- M. F. Lappert and H. Pyszora, Pseudohalides of Group IIIB and IVB Elements, in Advances in Inorganic Chemistry and Radiochemistry, ed. H. J. Emeléus and A. G. Sharpe, Academic Press, 1966, vol. 9, pp. 133–184 Search PubMed.
- F. Lindl, F. Fantuzzi, L. Mailänder, C. Hörl, G. Bélanger-Chabot and H. Braunschweig, Azidoborolate anions and azidoborole adducts: isolable forms of an unstable borole azide, Chem. Commun., 2022, 58, 4735–4738 RSC.
- A. K. Swarnakar, C. Hering-Junghans, K. Nagata, M. J. Ferguson, R. McDonald, N. Tokitoh and E. Rivard, Encapsulating Inorganic Acetylene, HBNH, Using Flanking Coordinative Interactions, Angew. Chem., Int. Ed., 2015, 54, 10666–10669 CrossRef CAS PubMed.
- A. K. Swarnakar, C. Hering-Junghans, M. J. Ferguson, R. McDonald and E. Rivard, Reactivity of a coordinated inorganic acetylene unit, HBNH, and the azidoborane cation [HB(N3)]+, Chem. Sci., 2017, 8, 2337–2343 RSC.
- E. Merling, V. Lamm, S. J. Geib, E. Lacôte and D. P. Curran, [3 + 2]-Dipolar Cycloaddition Reactions of an N-Heterocyclic Carbene Boryl Azide, Org. Lett., 2012, 14, 2690–2693 CrossRef CAS PubMed.
- P. I. Paetzold, Beiträge zur Chemie der Bor-Azide. I. Zur Kenntnis von Dichlorborazid, Z. Anorg. Allg. Chem., 1963, 326, 47–52 CrossRef CAS.
- P. I. Paetzold, M. Gayoso and K. Dehnicke, Darstellung, Eigenschaften und Schwingungsspektren der trimeren Bordihalogenidazide (BCl2N3)3 und (BBr2N3)3, Chem. Ber., 1965, 98, 1173–1180 CrossRef CAS.
- M. Müller, C. Maichle-Mössmer and H. F. Bettinger, BN-Phenanthryne: Cyclotetramerization of an 1,2-Azaborine Derivative, Angew. Chem., Int. Ed., 2014, 53, 9380–9383 CrossRef PubMed.
- S. Biswas, I. M. Oppel and H. F. Bettinger, Synthesis and Structural Characterization of 9-Azido-9-Borafluorene: Monomer and Cyclotrimer of a Borole Azide, Inorg. Chem., 2010, 49, 4499–4506 CrossRef CAS PubMed.
- T. Thiess, G. Bélanger-Chabot, F. Fantuzzi, M. Michel, M. Ernst, B. Engels and H. Braunschweig, Diborane(4) Azides: Surprisingly Stable Sources of Transient Iminoboranes, Angew. Chem., Int. Ed., 2020, 59, 15480–15486 CrossRef CAS PubMed.
- D. Prieschl, G. Bélanger-Chabot, X. Guo, M. Dietz, M. Müller, I. Krummenacher, Z. Lin and H. Braunschweig, Synthesis of Complex Boron–Nitrogen Heterocycles Comprising Borylated Triazenes and Tetrazenes Under Mild Conditions, J. Am. Chem. Soc., 2020, 142, 1065–1076 CrossRef CAS PubMed.
- R. L. Melen, A. J. Lough and D. W. Stephan, Boron azides in Staudinger oxidations and cycloadditions, Dalton Trans., 2013, 42, 8674–8683 RSC.
- R. L. Melen and D. W. Stephan, Cycloaddition reactions between dicyclohexylboron azide and alkynes, Dalton Trans., 2013, 42, 4795–4798 RSC.
- R. L. Melen and D. W. Stephan, Cycloaddition reactions of (C6F5)2BN3 with dialkyl acetylenedicarboxylates, Dalton Trans., 2015, 44, 5045–5048 RSC.
- T.-T. Liu, J. Chen, B.-T. Guan, Z. Lin and Z.-J. Shi, Distance-Triggered Distinct Aryl Migrations on Azidodiboranes, Chem. – Eur. J., 2023, 29, e202203676 CrossRef CAS PubMed.
- R. Haiges, J. A. Boatz, J. M. Williams and K. O. Christe, Preparation and Characterization of the Binary Group 13 Azides M(N3)3 and M(N3)3·CH3CN (M=Ga, In, Tl), [Ga(N3)5]2−, and [M(N3)6]3− (M=In, Tl), Angew. Chem., Int. Ed., 2011, 50, 8828–8833 CrossRef CAS PubMed.
- N. Emig, F. P. Gabbaï, H. Krautscheid, R. Réau and G. Bertrand, The Azide-Nitrilimine Analogy in Aluminum Chemistry, Angew. Chem., Int. Ed., 1998, 37, 989–992 CrossRef CAS PubMed.
- J. D. Queen, S. Irvankoski, J. C. Fettinger, H. M. Tuononen and P. P. Power, A Monomeric Aluminum Imide (Iminoalane) with Al–N Triple-Bonding: Bonding Analysis and Dispersion Energy Stabilization, J. Am. Chem. Soc., 2021, 143, 6351–6356 CrossRef CAS PubMed.
- W. Uhl, R. Gerding, S. Pohl and W. Saak, Reaktionen von R2Al-AlR2 (R=CH(SiMe3)2) mit Trimethylsilylazid—Insertion in die Al-Al-Bindung und Abbau zum trimeren Dialkylaluminiumazid, Chem. Ber., 1995, 128, 81–85 CrossRef CAS.
- R. A. Fischer, A. Miehr, H. Sussek, H. Pritzkow, E. Herdtweck, J. Müller, O. Ambacher and T. Metzger, Structures of (C5H5N)3Al(N3)3, [Me2N(CH2)3]2Al(N3) and Me2(N3)Al(H2NBut). Low-temperature OMVPE of AlN in the absence of ammonia, Chem. Commun., 1996, 2685–2686 RSC.
- J. Müller and K. Dehnicke, Darstellung, eigenschaften und schwingungsspektren von dialkylmetall-aziden der elemente aluminum, gallium, indium und thallium, J. Organomet. Chem., 1968, 12, 37–47 CrossRef.
- K. Haraguchi, Y. Kubota and H. Tanaka, Ring Opening of Nucleoside 1′,2′-Epoxides with Organoaluminum Reagents: Stereoselective Entry to Ribonucleosides Branched at the Anomeric Position, J. Org. Chem., 2004, 69, 1831–1836 CrossRef CAS PubMed.
- H. L. Ricks-Laskoski, B. L. Chaloux, S. M. Deese, M. Laskoski, J. B. Miller, M. A. Buckley, J. W. Baldwin, M. A. Hickner, K. M. Saunders and C. M. Christensen, Tetrazolation of Side Chains and Anhydrous Conductivity in a Hydrophobic Polymer, Macromolecules, 2014, 47, 4243–4250 CrossRef CAS.
- S. Monticelli and V. Pace, Diethylaluminium Azide: A Versatile Reagent in Organic Synthesis, Aust. J. Chem., 2015, 68, 703–706 CrossRef CAS.
- V. Aureggi and G. Sedelmeier, 1,3-Dipolar Cycloaddition: Click Chemistry for the Synthesis of 5-Substituted Tetrazoles from Organoaluminum Azides and Nitriles, Angew. Chem., Int. Ed., 2007, 46, 8440–8444 CrossRef CAS PubMed.
- V. H. Rawal and H. M. Zhong, One-step conversion of esters to acyl azides using diethylaluminum azide, Tetrahedron Lett., 1994, 35, 4947–4950 CrossRef CAS.
- J. M. Blackwell, W. E. Piers and M. Parvez, Mechanistic Studies on Selectivity in the B(C6F5)3-Catalyzed Allylstannation of Aldehydes: Is Hypercoordination at Boron Responsible?, Org. Lett., 2000, 2, 695–698 CrossRef CAS PubMed.
- W. E. Piers, The chemistry of perfluoroaryl boranes, Adv. Organomet. Chem., 2005, 52, 1–76 CAS.
- T. Beringhelli, D. Donghi, D. Maggioni and G. D'Alfonso, Solution structure, dynamics and speciation of perfluoroaryl boranes through 1H, 11B and 19F NMR spectroscopy, Coord. Chem. Rev., 2008, 252, 2292–2313 CrossRef CAS.
- D. Volz, T. Baumann, H. Flügge, M. Mydlak, T. Grab, M. Bächle, C. Barner-Kowollik and S. Bräse, Auto-catalysed crosslinking for next-generation OLED-design, J. Mater. Chem., 2012, 22, 20786–20790 RSC.
- K. Bläsing, J. Bresien, R. Labbow, D. Michalik, A. Schulz, M. Thomas and A. Villinger, Borane Adducts of Hydrazoic Acid and Organic Azides: Intermediates for the Formation of Aminoboranes, Angew. Chem., Int. Ed., 2019, 58, 6540–6544 CrossRef PubMed.
- K. Bläsing, J. Bresien, S. Maurer, A. Schulz and A. Villinger, Trimethylsilyl Pseudohalide Adducts of GaCl3 and B(C6F5)3, Eur. J. Inorg. Chem., 2021, 2021, 1913–1920 CrossRef.
- M. R. Elsby and S. A. Johnson, Nickel-Catalyzed C–H Silylation of Arenes with Vinylsilanes: Rapid and Reversible β-Si Elimination, J. Am. Chem. Soc., 2017, 139, 9401–9407 CrossRef CAS PubMed.
- M. Mewald, R. Fröhlich and M. Oestreich, An Axially Chiral, Electron-Deficient Borane: Synthesis, Coordination Chemistry, Lewis Acidity, and Reactivity, Chem. – Eur. J., 2011, 17, 9406–9414 CrossRef CAS PubMed.
- K. O. Christe, D. A. Dixon, D. McLemore, W. W. Wilson, J. A. Sheehy and J. A. Boatz, On a quantitative scale for Lewis acidity and recent progress in polynitrogen chemistry, J. Fluorine Chem., 2000, 101, 151–153 CrossRef CAS.
- H. Böhrer, N. Trapp, D. Himmel, M. Schleep and I. Krossing, From unsuccessful H2-activation with FLPs containing B(Ohfip)3 to a systematic evaluation of the Lewis acidity of 33 Lewis acids based on fluoride, chloride, hydride and methyl ion affinities, Dalton Trans., 2015, 44, 7489–7499 RSC.
- L. Greb, Lewis Superacids: Classifications, Candidates, and Applications, Chem. – Eur. J., 2018, 24, 17881–17896 CrossRef CAS PubMed.
Footnotes |
† Electronic supplementary information (ESI) available: synthesis and characterization of all compounds, NMR spectra, crystallographic data, and details of the DFT calculations. CCDC 2164107, 2329987–2329992, 2356544 and 2359713. For ESI and crystallographic data in CIF or other electronic format see DOI: https://doi.org/10.1039/d5qi00038f |
‡ These authors contributed equally to this work. |
|
This journal is © the Partner Organisations 2025 |
Click here to see how this site uses Cookies. View our privacy policy here.