DOI:
10.1039/D4QO01127A
(Research Article)
Org. Chem. Front., 2025, Advance Article
Combination of the structural components of amino acids with fatty acids: access to an unknown class of “fatty” α-amino acids by palladium-catalyzed amidocarbonylation†
Received
25th November 2024
, Accepted 21st January 2025
First published on 23rd January 2025
Abstract
A new class of amino acids, so-called fatty amino acids, which integrate the typical structural motif of another important class of natural products, fatty acids, is presented. By applying palladium-catalyzed amidocarbonylation, diverse new N-acyl fatty amino acids are synthesized in one step in a 100% atom-efficient manner. Utilizing a small amount (0.5 mol%) of simple commercial Pd(OAc)2, the desired products can be synthesized in good to high yields, up to multi-g-scale. The shown products represent a combination of two essential classes of natural products and provide new bio-based building blocks with potential for many applications.
Introduction
α-Amino acids and their derivatives are a prerequisite for life on Earth.1–10 Apart from their manifold biological functions in humans, animals, and plants, they find numerous applications in diverse fields ranging from nutrition and pharmaceuticals to cosmetics. Another class of essential biomolecules are fatty acids, which are aliphatic monocarboxylic acids with longer, linear, and partially unsaturated carbon chains. Esters of fatty acids with glycerol are found in all natural fats and oils and represent an important renewable feedstock highly relevant for the chemical industry.11–14
Due to their importance, α-amino acids and fatty acids have been studied in detail by chemists, biologists, and many other scientists for more than a hundred years, and a large number of derivatives have been synthesized for numerous applications.15–23 Although both biochemically essential classes of compounds occur in all living organisms, surprisingly there are no “hybrid” molecules in nature, e.g. amino acids with longer (unsaturated) aliphatic chains in the α-position. Clearly, such extended alkyl groups will determine the hydrophobicity of the respective amino acid. In fact, hydrophobic interactions play a dominant role in stabilizing protein structures. Why has evolution avoided such chemical compounds? To answer this and to study the properties of “fatty” amino acids, it is important to provide synthetic methodologies, which allow a convenient and general preparation of such compounds. Very surprisingly, such derivatives (with alkyl chains >C9) have not been synthesized to the best of our knowledge (Fig. 1). Therefore, we set out to develop an efficient and environmentally friendly protocol to make such molecules conveniently available on a g-scale for further studies. A straightforward route to synthesize natural and unnatural N-acyl amino acids is the so-called amidocarbonylation of aldehydes (Fig. 2a).24 This one-pot, three-component reaction makes use of aldehydes, amides, and carbon monoxide in the presence of a suitable metal catalyst. Amidocarbonylations are generally catalyzed by cobalt and palladium complexes. As they are 100% atom-economical and use readily available substrates, they are also interesting from ecological and economic points of view.
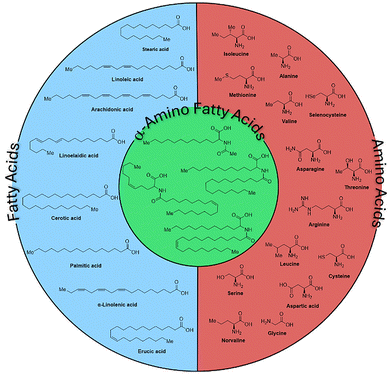 |
| Fig. 1 “Fatty” α-amino acids: a new class of compounds. | |
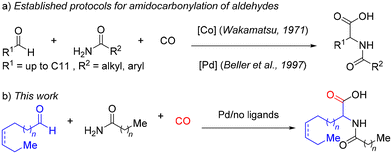 |
| Fig. 2 Amidocarbonylation of aldehydes and amides. | |
Originally in 1971, Wakamatsu reported the use of cobalt carbonyl complexes for this transformation.25 Later, researchers at Hoechst developed a palladium-catalysed variant, which works under milder reaction conditions.26 Interestingly, homogeneous27–30 and heterogeneous31–34 palladium catalysts have been applied in the latter and related cases.
The carbonylation of fatty aldehydes with CO in the presence of amides should in principle lead to unnatural long-chain amino acids, which also exhibit interesting amphiphilic (surface-active) properties.35–37 At this point, it is worth mentioning that the synthesis of new (bio-)surfactants with a hydrophilic head and a hydrophobic tail gained increasing attention in recent years,38–40 e.g. as part of modern vaccines.41
Against this background, we report here the first synthesis of a new class of unnatural amino acids. More specifically, we show that a variety of α-fatty amino acid derivatives are readily accessible by a palladium-catalysed amidocarbonylation reaction of the corresponding aldehydes in very good yields and selectively (Fig. 2b).
Results and discussion
We commenced our studies using commercially available dodecanal 1a and acetamide 2a as model substrates in the presence of 1 mol% Pd(OAc)2, 1 mol% H2SO4, and 35 mol% LiBr in N-methyl-2-pyrrolidone (NMP) as solvent at 100 °C under 60 bars of CO. Similar reaction conditions have been reported by some of us in the developmental studies of palladium-catalysed amidocarbonylation.24 Gratifyingly, the desired product was obtained in quantitative yield under these conditions.
To identify crucial reaction parameters, some variations of the metal precursor, temperature, pressure, additives, and solvent were made (Fig. 3). Notably, in all these experiments, a 1
:
1 ratio of amide and aldehyde was used, which is the most economical and avoids additional waste formation. Among the different palladium precursors, Pd(acac)2 and Pd(OAc)2 provided 3a in quantitative yield, while Pd2dba3 and PdBr2 gave lower product yield. Utilizing different solvents in the reaction furnished the product 3a in lower yield compared to NMP. In general, high product yields were obtained at lower CO pressure (20 bar) and lower temperature (60 °C). Decreasing the amount of catalyst proved to be viable and with only 0.1 mol% Pd(OAc)2, 80% yield of 3a was achieved. Furthermore, high catalyst turnover numbers (TON > 2000) in this transformation could be realized. From a mechanistic point of view, it is interesting that the reaction is quite sensitive to the amount of LiBr (35 mol%). As discussed below (see Fig. 5.), the formation of α-bromo-N-alkylamide seems to be crucial for the product formation. In agreement with this assumption, the replacement of LiBr with LiCl resulted in a lower yield of 3a. To better understand the amidocarbonylation of dodecanal, a kinetic profile of the model system was created under the optimized conditions. For this purpose, reactions were interrupted at precise moments and the crude reaction mixture was analysed. The first hour of the reaction time, 60% of product 3a was formed in addition to 40% of unreacted aldehyde 1a (Fig. 4 and Table S1, entry 2†), showing the rapid nature of the process. Interestingly, after 6 hours, neither aldehyde 1a nor amide 2a was observed, but the product yield still increased in the following 10 h until a nearly quantitative yield of 3a was obtained (Table S1, entry 5†). It is worth mentioning that the aldehyde and amide showed the same rate of consumption in the reaction and no intermediates could be observed. Adding new substrates and co-catalysts after 16 hours led to the formation of an additional 40% of 3a, indicating that the catalyst was still “alive”.
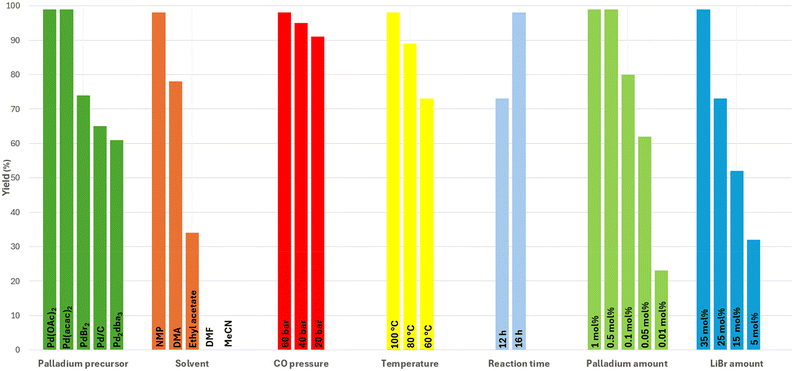 |
| Fig. 3 Palladium-catalysed amidocarbonylation of dodecanal with acetamide: variation of reaction conditions. Reactions were conducted on a 0.5 mmol scale and yields were determined by 1H-NMR analysis using 3,5-bis(trifluoromethyl)bromobenzene as an internal standard. | |
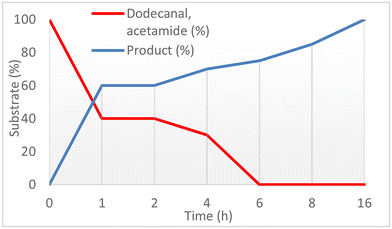 |
| Fig. 4 Consumption of dodecanal and acetamide, and formation of product 3a hour by hour. | |
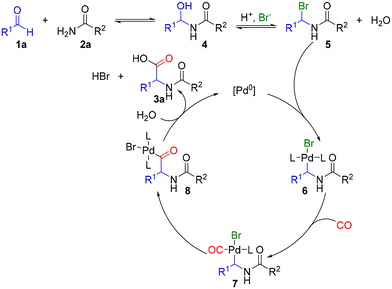 |
| Fig. 5 Proposed mechanism for the Pd-catalyzed amidocarbonylation of aldehydes. | |
Performing the model reaction under standard conditions but without CO showed significant conversion of dodecanal and acetamide; however, apart from an aldol condensation by-product, no other product was observed by GC-MS (see the ESI for details†). All these control experiments agree with the previously postulated mechanism for the Pd-catalyzed amidocarbonylation of aldehydes (Fig. 5).26 The process starts with the condensation between the aldehyde 1a and the amide 2a, resulting in the intermediate 4. Then, due to acidic conditions, protonation of the hydroxy group and further replacement by a bromide anion lead to intermediate 5, which is in equilibrium with 4. Then, oxidative addition of Pd0 species to intermediate 5 starts the catalytic cycle and leads to 6. Next, CO coordinates to the stabilized Pd+2 intermediate, furnishing 7. After ligand exchange, the desired product is formed by reductive elimination and the active Pd0 species is restored to start again the catalytic cycle.
To showcase the possibility to provide multi-gram-scale amounts of this novel class of compounds, e.g. for biological studies, we carried out upscaling experiments. As expected, product 3a could be easily isolated in around 8 g after filtration, solvent removal, and extraction without the necessity of column chromatography. Then, we started to prepare a variety of N-acyl amino acids with longer alkyl chains and investigated the scope of the reaction (Fig. 7). First, we performed reactions of the model aldehyde dodecanal 1a with different amides. Applying N-alkyl amides, such as acetamide 2a, butanamide 2b, and stearamide 2c, provided the corresponding products 3a, 3b, and 3c in good to high yields (84%, 90% and 78%, respectively). Similarly, cyclohexanecarboxamide 2g led to the desired product 3g in 75% yield. In humans, it is well known that saturated fatty acids have undesired biological properties. In contrast, unsaturated fatty acids have several health benefits, including potentially reducing the risk of heart disease. Hence, we tested unsaturated amides containing isolated double bonds in the alkyl chain, like oleamide 2d and erucamide 2e. To our delight, the reaction system is well tolerant towards olefins and the respective products 3d–e were furnished in good yields (68% and 71%). These latter results are noteworthy, as Pd-catalysed double bond isomerization or olefin carbonylation reactions could be expected. We also performed the reaction with industrially relevant methacrylamide containing a conjugated double bond, and the product 3f was obtained in 55% yield. For the first time, this substrate has been applied in amidocarbonlations and it was also possible to obtain suitable crystals for SC-XRD and its structure could be confirmed (Fig. 6, see the ESI for details†).42
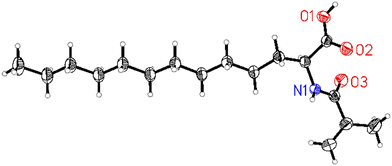 |
| Fig. 6 Molecular structure of 3f. Displacement ellipsoids correspond to 50% probability. | |
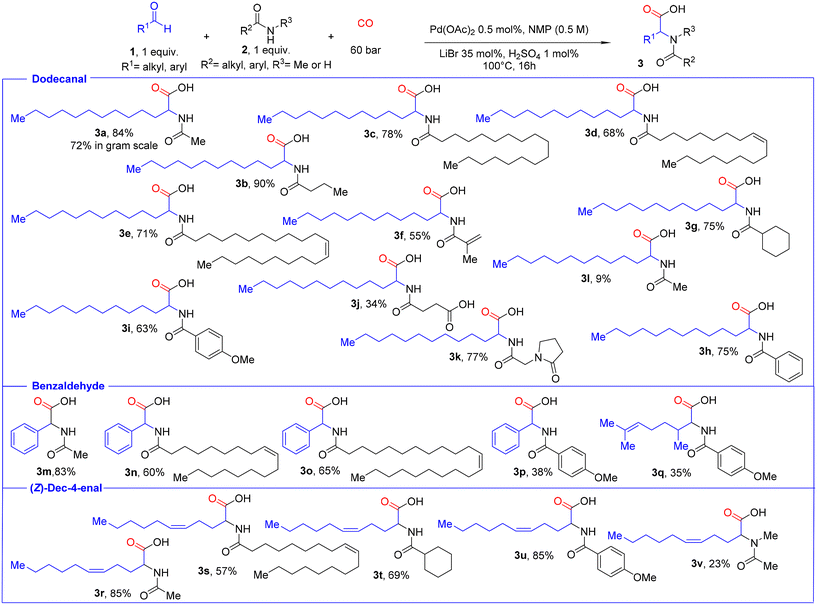 |
| Fig. 7 Synthesis of diverse N-acyl fatty α-amino acids. Reaction conditions: Pd(OAc)2 (0.5 mol%), aldehyde (0.5 mmol, 1 equiv.), amide (0.5 mmol, 1 equiv.), LiBr (35 mol%) and H2SO4 (1 mol%) in NMP (1 mL), CO (60 bar), 100 °C, and 16 h. Isolated yields from 0.5 mmol scale reactions. | |
Next, we turned our attention to the reaction of dodecanal with aromatic amides bearing different substituents. The parent benzamide 2 h gave the respective N-aryl-fatty amino acid 3h in 75% yield, while more electron-rich 4-methoxybenzamide 2i provided the desired product 3i in 63%. Amides containing other functional groups, such as carboxylic acid and cyclic amide, were also tolerated, and the respective 3j and 3k products were isolated in 34% and 77% yields. However, N-methyl acetamide 2l showed only low reactivity under these optimized conditions. As expected, aromatic aldehydes furnished the desired products 3p–m in 38–83% yields. Furthermore, other unsaturated aldehydes can be utilized to provide novel amino acids in one step. As an example, (Z)-dec-4-enal 1r reacted smoothly with acetamide 2r to afford product 3r in 85% yield, while the product 3s from the reaction with oleamide 2d was obtained in 57%. In addition, cyclohexanecarboxamide and 4-methoxybenzamide furnished the respective products 3t and 3u in 69% and 85% yields, respectively. Applying 2,6-dimethylhept-5-enal, a fragrance aldehyde also known as melonal 1q was obtained in 35% yield.
Apart from all these examples, other functionalized aldehydes including geranial, vanillin, 5-hydroxymethylfurfural (HMF), furfural, and syringaldehyde were tested. Unfortunately, under the standard reaction conditions in these cases no desired product was formed and unwanted side reactions such as oligomerization occurred.
Procedure and characterization
An 8 mL vial equipped with a stir bar was charged with Pd(OAc)2 (0.5 mol%), aldehyde (0.5 mmol, 1 equiv.), amide (0.5 mmol, 1 equiv.), LiBr (35 mol%) and H2SO4 (1 mol%) in NMP (1 mL). The reactional mixture was stirred under 60 bar CO at 100 °C for 16 h. After this time, the crude product was filtered through a small pad of Celite® with EtOAc and it was concentrated under reduced pressure. Then, NMP was removed from the crude product with distillation (1 mbar, 68 °C) and extraction methods or column chromatography were performed.
Conclusions
In summary, we have developed a protocol for the synthesis of an overlooked class of α-amino acid derivatives. It is very surprising that amino acids with long (unsaturated) alkyl chains in the α-position have never been described or found in living organisms or plants, although another important class of biomolecules, fatty acids, contain such structural units. We believe that it will be interesting to study the biological function of such amino acids and modified peptides or proteins. Therefore, it is necessary to provide synthetic protocols for their synthesis. We show that the known palladium-catalysed amidocarbonylation can be easily utilized to prepare a variety of so-called fatty amino acids in a 100% atom-efficient manner in the presence of low catalyst amounts.
All the obtained products have previously not been synthesized and present an interesting class of compounds, which combine two essential classes of natural products. Integrating such novel amino acids allows for the preparation of peptides and proteins with many potentially biological functions, as hydrophobic residues from amino acids contribute significantly to receptor activation, G protein coupling, and oligomerization processes. In addition, their physical properties as surfactants make this synthetic protocol interesting for several applications in chemistry and biology. Finally, it is worth mentioning that the developed procedure can be combined with subsequent enzyme-catalysed kinetic resolution, which should allow for a straightforward two-step synthesis of the respective amino acid enantiomers.42,43
Data availability
The data supporting this article have been included as part of the ESI.†
Conflicts of interest
There are no conflicts to declare.
Acknowledgements
We thank the analytical team of the Leibniz-Institut für Katalyse e.V. Also, we acknowledge the financial support from the São Paulo Research Foundation (FAPESP grants: 2019/25657-9; 2022/14575-4 [ELSS]; 2014/25770-6; 2014/07600-3; 2023/003839 [CRDC]) and the Brazilian National Research Council (CNPq) for partial support of this project (grant 406643/2018-0), as well as a research fellowship to CRDC (grant 306773/2018-0).
References
- B. E. Sleebs, T. Van Nguyen and A. B. Hughes, Recent Advances in Stereoselective Synthesis and Application of β-Amino Acids, Org. Prep. Proced. Int., 2000, 41, 429–478 CrossRef.
- R. Zhang, C. Zhang, J. Tan, Y. He, D. Zhuo, J. Zhang, Z. Luo, Q. Li, J. Yao, C. Ke, C. Tang, Y. Ye, S. He, X. Sheng and C. Liao, Enzymatic Synthesis of Noncanonical α-Amino Acids Containing γ-Tertiary Alcohols, Angew. Chem., 2024, 136, e202318550 CrossRef.
- D. S. Prendes, F. Papp, N. Sankaran, N. Sivendran, F. Beyer, C. Merten and L. J. Gooßen, Enantioselective Synthesis of Arylglycines via Pd-Catalyzed Coupling of Schöllkopf Bis-Lactim Ethers with Aryl Chlorides, Angew. Chem., Int. Ed., 2023, 62, e202309868 CrossRef CAS PubMed.
- M. Lubberink, W. Finnigan, C. Schnepel, C. R. Baldwin, N. J. Turner and S. L. Flitsch, One-Step Biocatalytic Synthesis of Sustainable Surfactants bySelective Amide Bond Formation, Angew. Chem., Int. Ed., 2022, 61, e202205054 CrossRef CAS PubMed.
- D. Gördes, H. Neumann, A. J. von Wangelin, C. Fischer, K. Drauz, H. Krimmer and M. Beller, The Amidocarbonylation of Aldehydes, Adv. Synth. Catal., 2003, 345, 510–516 CrossRef.
- Y. Zhang, J. Vanderghinste, J. Wang and S. Das, Challenges and recent advancements in the synthesis of α,α-disubstituted α-amino acids, Nat. Commun., 2024, 15, 1474 CrossRef CAS PubMed.
- F. J. A. Troyano, K. Merkens, K. Anwar and A. Gómez-Suárez, Radical-Based Synthesis and Modification of Amino Acids, Angew. Chem., Int. Ed., 2021, 60, 1098–1115 CrossRef PubMed.
- C. Najera and J. M. Sansano, Catalytic asymmetric synthesis of alpha-amino acids, Chem. Rev., 2007, 107, 4584–4671 CrossRef CAS PubMed.
- C. J. Moreno, K. Hernández, S. J. Charnok, S. Gittings, M. Bolte, J. Joglar, J. Bujons, T. Parella and P. Clapés, Synthesis of γ-Hydroxy-α-amino Acid Derivatives by Enzymatic Tandem Aldol Addition-Transamination Reactions, ACS Catal., 2021, 11, 4660–4669 CrossRef CAS PubMed.
- Y. Matsumoto, J. Sawamura, Y. Murata, T. Nishikata, R. Yazaki and T. Ohshima, Amino Acid Schiff Base Bearing Benzophenone Imine As a Platform for Highly Congested Unnatural α-Amino Acid Synthesis, J. Am. Chem. Soc., 2020, 142, 8498–8505 CrossRef CAS PubMed.
- A. S. Elgharbawy, W. Sadik, O. M. Sadek, M. A. Kasaby and J. Chil, A review on biodiesel feedstocks and production technologies, Chem. Soc., 2021, 66, 5098–5109 CAS.
- G. M. Mathew, D. Raina, V. Narisetty, V. Kumar, S. Saran, A. Pugazhendi, R. Sindhu, A. Pandey and P. Binod, Recent advances in biodiesel production: Challenges and solutions, Sci. Total Environ., 2021, 794, 148751 CrossRef CAS PubMed.
- W. Muramatsu, C. Manthena, E. Nakashima and H. Yamamoto, Peptide Bond-Forming Reaction via Amino Acid Silyl Esters: New Catalytic Reactivity of an Aminosilane, ACS Catal., 2020, 10, 9594–9603 CrossRef CAS.
- N. Tanaka, J. L. Zhu, O. L. Valencia, C. R. Schull and K. A. Scheidt, J, Cooperative Carbene Photocatalysis for β-Amino Ester Synthesis, Am. Chem. Soc., 2023, 145, 24486–24492 CAS.
- M. U. Ahmad, Fatty acids: Chemistry, synthesis, and applications, Elsevier, 2017 Search PubMed.
- J. Guo, L. Sun, F. Zhang, B. Sun, B. Xu and Y. Zhou, Progress in synthesis, properties and application
of amino acid surfactants, Chem. Phys. Lett., 2022, 794, 139499 CrossRef CAS.
- A. Vik and T. V. Hansen, Synthetic manipulations of polyunsaturated fatty acids as a convenient strategy for the synthesis of bioactive compounds, Org. Biomol. Chem., 2018, 16, 9319–9333 RSC.
- O. G. Mountanea, D. Limnios, M. G. Kokotou, A. Bourboula and G. Kokotos, Asymmetric Synthesis of Saturated Hydroxy Fatty Acids and Fatty Acid Esters of Hydroxy Fatty Acids, Eur. J. Org. Chem., 2019, 2010–2019 CrossRef CAS.
- A. F. Noisier and M. A. Brimble, C-H functionalization in the synthesis of amino acids and peptides, Chem. Rev., 2014, 114, 8775–8806 CrossRef CAS PubMed.
- J. A. Avendaño-Villarreal, F. G. Delolo, A. V. Granato, E. V. Gusevskaya and E. N. dos Santos, The first one-pot metathesis–hydroformylation procedure: a straight synthesis of 2-arylpropanals from renewable 1-propenylbenzenes, ChemCatChem, 2023, 15, e202201186 CrossRef.
- S. H. Park and S. Lee, Selective Synthesis of (Z)- and (E)-β-Fluoro-α,β-Unsaturated Amides Using Palladium-Catalyzed Aminocarbonylation, Org. Lett., 2023, 25, 6925–6930 CrossRef CAS PubMed.
- T. J. Fulton, A. Q. Cusumano, E. J. Alexy, Y. E. Du, H. Zhang, K. N. Houk and B. M. Stoltz, Global Diastereoconvergence in the Ireland-Claisen Rearrangement of Isomeric Enolates: Synthesis of Tetrasubstituted α-Amino Acids, J. Am. Chem. Soc., 2020, 142, 21938–21947 CrossRef CAS PubMed.
- W. Guo, Y. Luo, H. H.-Y. Sung, I. D. Williams, P. Li and J. Sun, Chiral Phosphoric Acid Catalyzed Enantioselective Synthesis of α-Tertiary Amino Ketones from Sulfonium Ylides, J. Am. Chem. Soc., 2020, 142, 14384–14390 CrossRef CAS PubMed.
- M. Beller and M. Ecker, Amidocarbonylation—An Efficient Route to Amino Acid Derivatives, Angew. Chem., Int. Ed., 2000, 39, 1010–1027 CrossRef CAS PubMed.
- H. Wakamatsu, J. Uda and N. Yamakami, Synthesis of N-acyl amino-acids by a carbonylation reaction, J. Chem. Soc. D, 1971, 1540–1540 RSC.
- M. Beller, M. Eckert, F. Vollmüller, S. Bogdanovic and H. Geissler, Palladium-Catalyzed Amidocarbonylation—A New, Efficient Synthesis of N-Acyl Amino Acid, Angew. Chem., Int. Ed. Engl., 1997, 36, 1494–1496 CrossRef CAS.
- Y. Ge, F. Ye, J. Yang, A. Spannenberg, R. Jackstell and M. Beller, Palladium-Catalyzed Domino Aminocarbonylation of Alkynols: Direct and Selective Synthesis of Itaconimides, JACS Au, 2021, 1, 1257–1265 CrossRef CAS PubMed.
- J. S. Quesnel and B. A. Arndtsen, Transition-metal-catalyzed multicomponent coupling reactions with imines and carbon monoxide, Pure Appl. Chem., 2013, 85, 377–384 CrossRef CAS.
- G. M. Torres, et al., Palladium-catalyzed, multicomponent approach to β-lactams via aryl halide carbonylation, J. Org. Chem., 2016, 81, 12106–12115 CrossRef CAS PubMed.
- M. Li, S. Li, D. Chen, F. Gao, Y. Qiu, X. Wang, Z. Quan and Y. Liang, Regioselective C–H Active Carbonylation via 1,4-Palladium Migration, Org. Lett., 2023, 25, 2761–2766 CrossRef CAS PubMed.
- S. Hancker, S. Kreft, H. Neumann and M. Beller, Synthesis of N-Lauroyl Sarcosine by Amidocarbonylation: Comparing Homogeneous and Heterogeneous Palladium Catalysts, Org. Process Res. Dev., 2017, 21, 2045–2051 CrossRef CAS.
- R. Akiyama, T. Sagae, M. Sugiura and S. Kobayashi, Efficient synthesis of N-acyl-α-amino acids via polymer incarcerated palladium-catalyzed amidocarbonylation, J. Organomet. Chem., 2004, 689, 3806–3809 CrossRef CAS.
- A. Hamasaki, X. Liu and M. Tokunaga, Amidocarbonylation of Aldehydes Utilizing Cobalt Oxide-supported Gold Nanoparticles as a Heterogeneous Catalyst, Chem. Lett., 2008, 37, 1292–1293 CrossRef CAS.
- T. T. Dang, Y. Zhu, J. S. Y. Ngiam, S. C. Ghosh, A. Chen and A. M. Seayad, Palladium Nanoparticles Supported on ZIF-8 As an Efficient Heterogeneous Catalyst for Aminocarbonylation, ACS Catal., 2013, 3, 1406–1410 CrossRef CAS.
- M. Connor, C. W. Vaughan and R. J. Vandenberg, N-Acyl, amino acids and N-acyl neurotransmitter conjugates: neuromodulators and probes for new drug targets, Br. J. Pharmacol., 2010, 160, 1857–1871 CrossRef CAS PubMed.
- L. Hanuš, E. Shohami, I. Bab and R. Mechoulam, N-Acyl, amino acids and their impact on biological processes, BioFactors, 2014, 40, 381–388 CrossRef PubMed.
- D. K. F. Santos, R. D. Rufino, J. M. Luna, V. A. Santos and L. A. Sarubbo, Open AccessReview.
- D. K. F. Santos, R. D. Rufino, J. M. Luna, V. A. Santos and L. A. Sarubbo, Int. J. Mol. Sci., 2016, 17, 401 CrossRef PubMed.
- C. E. Drakontis and S. Amin, Biosurfactants: Formulations, properties, and applications, Curr. Opin. Colloid Interface Sci., 2020, 48, 77–90 CrossRef CAS.
- D. G. Almeida, R. C. F. S. Silva, J. M. Luna, R. D. Rufino, V. A. Santos, I. M. Banat and L. A. Sarubbo, Biosurfactants: Promising Molecules for Petroleum Biotechnology Advances, Front. Microbiol., 2016, 7, 1718 Search PubMed.
- A. Hinzmann, S. S. Druhmann and H. Gröger, Synthesis of Bifunctional Molecules for the Production of Polymers Based on Unsaturated Fatty Acids as Bioderived Raw Materials, Sustainable Chem., 2020, 1, 18 Search PubMed.
- M. L. Smith, S. Gandolfi, P. M. Coshall and P. K. Rahman, Biosurfactants: a Covid-19 perspective, Front. Microbiol., 2020, 11, 1341 CrossRef PubMed.
- H. K. Chenault, J. Dahmer and G. M. Whitesides, Kinetic resolution of unnatural and rarely occurring amino acids: enantioselective hydrolysis of N-acyl amino acids catalyzed by acylase I, J. Am. Chem. Soc., 1989, 111, 6354–6364 CrossRef CAS.
Footnotes |
† Electronic supplementary information (ESI) available. CCDC 2332462. For ESI and crystallographic data in CIF or other electronic format see DOI: https://doi.org/10.1039/d4qo01127a |
‡ These authors contributed equally to the development of this paper. |
|
This journal is © the Partner Organisations 2025 |
Click here to see how this site uses Cookies. View our privacy policy here.