DOI:
10.1039/D4QO02211D
(Review Article)
Org. Chem. Front., 2025, Advance Article
Advances in synthetic strategies for two-dimensional conjugated polymers
Received
23rd November 2024
, Accepted 18th January 2025
First published on 28th January 2025
Abstract
Two-dimensional conjugated polymers (2D CPs) are typically represented by 2D conjugated covalent organic frameworks (COFs) that consist of covalently cross-linked linear conjugated polymers, which possess extended in-plane π-conjugation and out-of-plane electronic couplings. The precise incorporation of molecular building blocks into ordered polymer frameworks through (semi)reversible 2D polycondensation methodologies enables the synthesis of novel polymer semiconductors with designable and predictable properties for various (opto)electronic, spintronic, photocatalytic, and electrochemical applications. Linkage chemistry lays the foundation for this class of synthetic materials and provides a library for subsequent investigations. In this review, we summarize recent advances in synthetic strategies for 2D CPs. By exploring synthetic approaches and the intricate interplay between chemical structure, the efficiency of 2D conjugation, and related physicochemical properties, we are expected to guide readers with a general background in synthetic chemistry and those actively involved in electronic device research. Furthermore, the discussion will appeal to researchers intrigued by the prospect of uncovering novel physical phenomena or mechanisms inherent in these emerging polymer semiconductors. Finally, future research directions and perspectives of highly crystalline and processable 2D CPs for electronics and other cutting-edge fields are discussed.
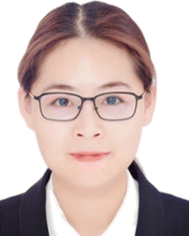 Ruyan Zhao | Ruyan Zhao received her PhD from the University of Science and Technology of China in 2019 under the supervision of Prof. Jun Liu. She then joined the University of Toronto as a postdoctoral fellow, working with Prof. Dwight S. Seferos from February 2020 to June 2022. In 2022, she joined Technische Universität Dresden as a postdoctoral researcher under the supervision of Prof. Xinliang Feng. In 2023, she moved to the Max Planck Institute of Microstructure Physics in Halle to continue her postdoctoral research. Her research interests include the synthesis and application exploration of novel conjugated polymers and hybrid semiconductors. |
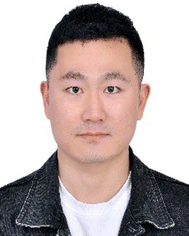 Guoqin Liu | Guoqin Liu received his Master's degree from the University of Science and Technology of China, under the supervision of Prof. Guhuan Liu and Dr Guoying Zhang. He is currently pursuing a PhD at TU Dresden, under the supervision of Prof. Xinliang Feng and Dr Mingchao Wang. His research focuses on the design and synthesis of novel 2D conjugated polymers (2D CPs) and their applications in semiconductor devices and spin dynamics. |
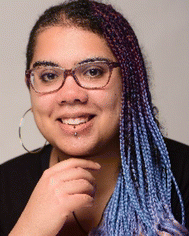 Philomène Leonore Koko | Philomène Leonore Koko received her Master's degree from the Dresden University of technology of Germany, under the supervision of Prof. Xinliang Feng and Dr Mingchao Wang. She is currently pursuing a PhD at TU Dresden under the supervision of Prof. Xinliang Feng and Dr Mingchao Wang. Her research focuses on the design and synthesis of novel 2D conjugated polymers (2D CPs) and their applications in semiconductor devices. |
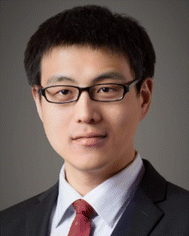 Mingchao Wang | Mingchao Wang is a research group leader at Technische Universität Dresden (TUD) and Max Planck Institute for Microstructure Physics. He received his BSc from Shandong University and MSc as well as PhD from TUD in chemistry. After that, he worked as a research associate at TUD and was elected research group leader in 2022. His current research interests focus on the chemistry and physics of 2D conjugated (covalent or coordination) polymers, covalent organic frameworks, and metal–organic frameworks, automated synthesis and characterization, and their applications in electronics, catalysis and energy devices. |
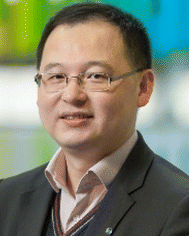 Xinliang Feng | Xinliang Feng is the Director of the Department of Synthetic Materials and Functional Devices at the Max Planck Institute of Microstructure Physics and the head of the Chair of Molecular Functional Materials at Technische Universität Dresden. His current scientific interests include synthetic methodology for new-type of polymers, organic and polymer synthesis, interfacial chemistry, supramolecular chemistry of π-conjugated system, bottom-up synthesis of carbon nanostructures and graphene nanoribbons, 2D polymers, and supramolecular polymers, 2D carbon-rich conjugated polymers for optoelectronics and spintronics, electrochemical exfoliation of 2D crystals, graphene and 2D materials for energy storage and conversion, new energy devices and technologies. |
10th anniversary statement
It is a great honor to contribute to the 10th-anniversary collection of Organic Chemistry Frontiers. Over the past decade, the journal has established itself as a highly visible platform in the fields of organic chemistry and organic materials, fostering innovation and collaboration in the global chemistry community. Our experiences with the journal have been exceptional, marked by rigorous peer review and impactful publication processes. Coincidentally, 2024 also marks the 10th anniversary of our research group at TU Dresden, we have been dedicated to advancing polymer synthesis, supramolecular chemistry, synthetic 2D materials, electronic and energy technologies. Looking ahead, we share the journal's mission to push the boundaries of chemical research and drive transformative discoveries in organic chemistry and advanced organic materials. As the journal celebrates this milestone, we would like to express our wholehearted support for its commitment to advancing chemistry in China, Asia, and beyond, and to inspiring researchers to address pressing scientific challenges. We are confident that the journal will continue to grow and flourish in the years to come.
|
1. Introduction
Conjugated polymers (CPs) are a class of organic semiconductors that feature tunable energy levels and delocalized π-electron clouds along their conjugated chains.1 Traditional conjugated polymers usually comprise one-dimensional (1D) backbones decorated with flexible side chains that can be easily integrated into electronic devices through solution-processing methods.2,3 Nonetheless, the charge transport performance achieved so far in 1D CPs are still inadequate for high-performance organic electronics: the charge carriers are delocalized only along the linear polymer backbone, and the hopping between adjacent polymer chains limits the charge transport in space. By extending the dimensionality, multiple strands of charge transport can be, in principle, established to bypass defects, narrow the band gaps, and suppress vibration to facilitate band-like transport in the resulting two-dimensional (2D) CPs.4
2D CPs are generally represented by 2D conjugated covalent organic frameworks (2D c-COFs),5 which possess in-plane extended π-conjugation and out-of-plane electronic coupling.6 2D CPs are assembled from aromatic building blocks linked together by conjugated covalent linkages that can be formed through various 2D polycondensation/polymerization reactions. Highly planar and conjugated building blocks can facilitate the crystallization process and improve in-plane π-delocalization as well as out-of-plane π-orbital overlapping. Linkage chemistry determines the reversibility of the error-correction crystallization and influences the stability and electronic communication between different building blocks. The 2D lattice and the interlayer stacking mode define band structure features, such as Dirac points and topological flat bands.7 Researchers should consider the above four important aspects before developing 2D CPs, i.e., suitable building blocks, linkages, lattice, and interlayer stacking modes. In particular, linkage chemistry is essential for the controllable synthesis of crystalline 2D CPs and strongly impacts the underlying physicochemical properties. In addition, the layer numbers (i.e., thickness in the axial direction), the rational aspect ratios, and the periodic alignments are also important merits for 2D CPs.
Unlike 1D CPs that can be prepared efficiently through irreversible transition metal-catalyzed cross-coupling reactions, such as Suzuki/Stille/Sonagashira/Yamamoto polymerization, direct arylation polycondensation (DArP), and Kumada catalyst transfer polycondensation (KCTP),8–11 etc., the synthesis of 2D CPs relies on the reversible or quasi-reversible linkage formation and/or the subsequent error-correction.12 Currently, only very limited synthetic methodologies are accessible for the synthesis of crystalline 2D CPs. Among them, 2D polyimines (2D PIs, or imine-linked 2D c-COFs) synthesized through reversible Schiff-base chemistry are the most prevalent. However, the imine-linked 2D c-COFs suffer from low chemical stability and poor π-electron delocalization, making them less attractive for future electronic and spintronic device integrations.13,14 The first imine-linked COF was reported in 2009,13 while the first 2D PI was developed in 2011.15 Subsequently, researchers have made significant efforts to the development of 2D PI derivatives, employing post-synthetic modification of 2D PIs and multiple-component one-pot synthesis (to create e.g., thiazole/pyridine rings) through imine-linked intermediates. These strategies have been employed to enhance the chemical stability and/or conjugation of the resulting materials. Using a similar Schiff-base reaction of α-diketones with α-diamines, ladder-type 2D polypyrazines (2D PPZs, or pyrazine/phenazene-linked 2D c-COFs) can be developed with enhanced framework planarity and π-conjugation.
As early as 2009, aryl–aryl coupling on flat metal surfaces overcame the irreversibility of the C–C coupling reaction by using halide-functionalized monomers, thereby enabling the synthesis of 2D polyarylenes (2D PAs).16 For example, a 2D polyphenylene was successfully synthesized on the Ag (111) surface.17 However, the obtained thin layer showed limited lateral size (ca. 50 nm) and strong binding with the Ag(111) surface, which presents a significant challenge in terms of transferring the thin layer for device integration. In 2016, 2D poly(arylene vinylene)s (2D PAVs, or vinylene/sp2-C-linked 2D c-COFs) were synthesized by the Knoevenagel polycondensation in solution, showing much strengthened 2D conjugation and chemical stability compared to 2D PIs.18 In comparison to the single-double bond alternation in the above 2D CPs, ladder-type 2D poly(benzimidazobenzophenanthroline)s (2D BBLs or BBL-type 2D c-COFs) represent unique fused molecular geometry to further increase the effective π-conjugation length by strengthened parallel p-orbital interactions. A phthalocyanine-based 2D BBL has exhibited intrinsic charge carrier mobility as high as ca. 1000 cm2 V−1 s−1,4 showing the potential of 2D CPs in high-performance (opto)electronic and electrochemical applications.
Over the past decade, the growing interest in 2D CPs has prompted significant research efforts to advance their design, synthesis, and potential applications. It is beyond question that the synthesis methodologies provide the foundation and material library for subsequent investigations. To date, no representative review article has provided the progress on the synthesis of 2D CPs. Given our expertise in the chemistry and physics of 2D CPs, we anticipate presenting an overview of the latest developments in this field and offering insights into its prospects. This review aims to provide a comprehensive overview of the strategies employed to synthesize crystalline 2D CPs, including 2D PIs and derivatives, 2D PAs, 2D PAVs, and 2D BBLs. By examining the various synthetic approaches and the intricate relationship between structure and properties, we aim to present a comprehensive guideline for readers with a general background in synthetic chemistry and those engaged in electronic device research.
2. Synthetic strategy for 2D CPs
Various approaches, including solution synthesis, on-surface synthesis, and interfacial synthesis, have been explored to synthesize 2D CPs in bulk powders and thin films, with a few exceptions being single crystals. In consideration of the various polymerization and crystallization mechanisms – that is to say, thermodynamic and/or kinetic control – employed in different synthetic strategies, the present review does not seek to provide a comparison of these mechanisms. Solid-state synthesis, combined with exfoliation techniques, has been a method of choice for creating a range of 2D CPs.19,20 Nonetheless, this technique requires the meticulous pre-assembly of monomers into single crystals before polymerization, which limits its broad applicability in the synthesis of 2D CPs. In contrast, solution-based synthesis methods, such as hydrothermal or solvothermal processes, stand out as the most efficient and straightforward way of producing 2D CPs in polycrystalline bulk forms. Furthermore, on-surface synthesis and interfacial polymerization are adept at generating thin films of these materials.21
2.1 Solution synthesis
Solution synthesis, including hydro- and solvothermal synthesis, provides a high degree of adaptability in reaction parameters, encompassing a spectrum of variables like solvent, catalyst, temperature, volume, pressure, etc.22–24 This adaptable approach relies on the general reversibility or thermodynamic control of reactions, offering benefits such as high selectivity, precise control over stereochemistry, and high yields. It has been commonly used to produce bulk polycrystalline 2D CPs and, in some instances, small-sized single crystals with domain size of ca. 1 μm. For the fabrication of thin films, solution processing of exfoliated 2D CPs is essential to maximize their potential across a variety of applications and to explore their layer-dependent physical characteristics.
2.2 On-surface synthesis
On-surface synthesis under vacuum conditions is appealing for the meticulous crafting of monolayer 2D CP with tunable lateral size.25,26 In this respect, the metal substrates provide confining surfaces and catalytic sites. So far, this method has been limited to 2D PAs synthesized via Ullmann-type coupling. The chemical vapor deposition (CVD) technique has proven effective in producing thin films with smooth surfaces and adjustable thicknesses.27–29 However, the interaction between molecules and the substrate, along with the high sublimation temperatures of monomers, can restrict their surface mobility, leading to limited crystallinity with domain sizes typically below 100 nm. An additional significant challenge is the tricky transfer process of these thin layers from the initial solid surface to alternative substrates for further characterization and integration into devices.
2.3 Interfacial synthesis
The interface between two immiscible liquids, such as chloroform/water or dichloromethane/water, forms a nanoscale-thick boundary that is inherently uneven due to the minor solubility of one liquid in the other.30 This characteristic makes liquid/liquid interfacial synthesis an effective technique for creating multilayer or relatively thick films spanning from a few nanometers to micrometers.31 Techniques like on-water surface synthesis, such as surfactant-monolayer-assisted interfacial synthesis (SMAIS), provide a smooth and well-defined surface with a root mean square roughness of approximately 3 Å,32 which is ideal for the large-scale fabrication of monolayer to few-layer 2D CPs.21,33–35 These approaches also simplify the transfer of the synthesized films from the water surface onto various substrates for subsequent characterization and device fabrication. Recent studies utilizing these methods have produced highly crystalline 2D PIs,36,37 2D poly(pyridinium salt),38 2D PAVs,39 etc., with adjustable thicknesses from ∼1 to ∼200 nm.
3. Linkage chemistry towards crystalline 2D CPs
The choice of linkage is a pivotal factor for the construction of 2D CPs, as they affect the stability and the degree of conjugation, which in turn dictates the physicochemical properties of the 2D CPs.12 Typical conjugated linkages discussed in this Review include imine (formed by Schiff-base condensation between aromatic aldehyde and amine, Table 1, Fig. 2 and 3), pyrazine (prepared by two consecutive Schiff-base reactions of α-diketone with α-diamine), vinylene (furnished by the different condensation reaction of aromatic aldehyde and active methyl, i.e. Knoevenagel/Aldol-type/Horner–Wadsworth–Emmons (HWE)/Wittig reaction), C–C (formed mainly by Ullmann-type coupling), and BBL-imidazole (prepared by imide bond formation between α-diamine and naphthalene anhydride followed by intramolecular dehydration).4,40 Imine linkages are commonly utilized because of their facile formation of highly crystalline 2D CPs. Yet, they are characterized by moderate π-electron delocalization due to the inherent polarity of the imine (C
N) bonds. The ring structure of pyrazine linkage enhances conjugation and chemical stability and facilitates charge carrier transport. Compared to 2D PIs and PPZs, 2D PAVs, 2D PAs, and 2D BBLs are difficult to synthesize due to the challenges associated with low reversibility of C
C bond/BBL-imidazole formation or irreversibility of C–C bond formation. From the perspective of enhancing the in-plane conjugation, we will discuss the 2D PIs, 2D PPZs, 2D PAVs, 2D PAs, and 2D BBLs in sequence.
Table 1 Post-modification of 2D PIs to construct conjugated linkages with better chemical stability and/or enhanced conjugation
Multicomponent one-pot synthesis through imine-linked intermediates |
Ref. |
Thiazole (C–H functionalization and oxidative annulation)  |
61 |
Benzoxazole (imine formation, cyclization, and oxidation)  |
64 |
Non-substituted quinoline  |
65 |
Substituted quinoline (Povarov reaction) 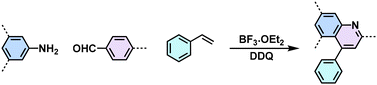 |
66 |
Pyrimidazole (Groebke–Blackburn–Bienaymé reaction)  |
67 |
3.1 2D polyimines (2D PIs) by Schiff-base reaction
Since the pioneering work of 2D PIs (COF-366, named as 2D PI-1 in this Review) between terephthalaldehyde–4,4′,4′′,4′′′-(porphyrin-5,10,15,20-tetrayl)tetraaniline (M-NH2-1, the monomers are also renamed in this review: M means monomer followed by its functional groups such as –NH2, –CHO, –CH3, –CH2CN, etc.) and terephthalaldehyde (M-CHO-1) by Yaghi and colleagues in 2011 (Scheme 1),15 many efforts, from both synthetic and application aspects, have been made to achieve high-crystalline 2D PIs and the corresponding electronic device integrations.12,41–43 Structural modulation according to the experimental powder X-ray diffraction (pXRD) pattern reveals that 2D PI-1 adopts a fully eclipsed structure with an AA stacking sequence, exhibiting an interlayer distance of 5.64 Å.15 The formation of imine linkage includes two steps. First, the amine nitrogen acts as a nucleophile, attacking the electrophilic carbonyl carbon of aldehydes. Then, the nitrogen is deprotonated, and the electrons from this N–H bond push the oxygen off the carbon, leaving a compound with a C
N double bond and releasing a water molecule (Table 1). Because of the highly reversible reaction merit, crystalline 2D PIs are experimentally the most feasible. During the past decade, various 2D PIs have been developed with robust topologies like honeycomb, rhombic, square, Kagome, hexagonal, etc. Both polycrystalline bulk powders, single crystals, and wafer-size thin films have been synthesized.44–50 For comprehensive discussions about the imine-linked 2D PIs, we recommend readers turn to recent comprehensive reviews.12,41,51
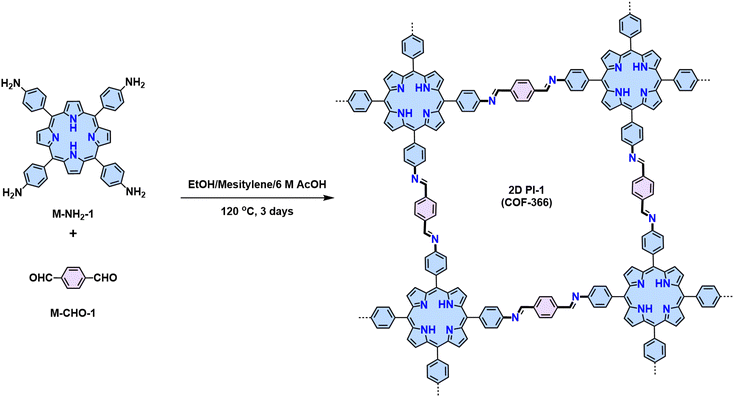 |
| Scheme 1 Synthesis of the first 2D polyimines of 2D PI-1 (COF-366). | |
The growth of single crystals of 2D CPs can facilitate a deeper understanding of the 2D polymer crystallization and growth process at the atomic level. This will provide more accurate information about the structure–property relationship, thus guiding the design of the new generation of electronic materials.52 The high reversibility of imine-bond formation and deformation allows for achieving 2D single crystals by delicately tuning the nucleation and growth dynamics. Zhao et al. disclosed that building blocks with a large π-conjugated planar structure, e.g., 4,4′,4′′,4′′′-(pyrene-1,3,6,8-tetrayl)tetraaniline (M-NH2-3), can facilitate the self-assembly and pre-arrangement of monomers via enhanced intermolecular interactions, which is beneficial to the growth of single crystals (Fig. 1a).45 Dichtel et al. synthesized two single crystals of 2D PI-2 and 2D PI-3 using benzoic acid as the catalyst and aniline as a monofunctional modulator in benzonitrile (Fig. 1b).49 Instead of crystallizing in a typical organic solvent, Wei and colleagues used supercritical CO2 (sc-CO2) as the solvent medium to accelerate single-crystal polymerization by 10
000
000 folds and produce 2D PI-2 single crystals with sizes up to 0.2 mm within 2–5 min (Fig. 1c).44 Cortés and colleagues used optical interferometric scattering microscopy for the operando studies of COF polymerization and framework formation.52 It is noteworthy that the size and quality of 2D PI crystals achieved so far are still not comparable to those of 3D PI crystals, due to strong π-π stacking of planar building blocks and thus low solubility of crystal seeds in solvent. A structure resolution by single-crystal X-ray diffraction remains a dream for 2D PIs.47 A more profound comprehension of growth mechanisms is imperative to direct the synthesis of high-quality 2D PI single crystals.
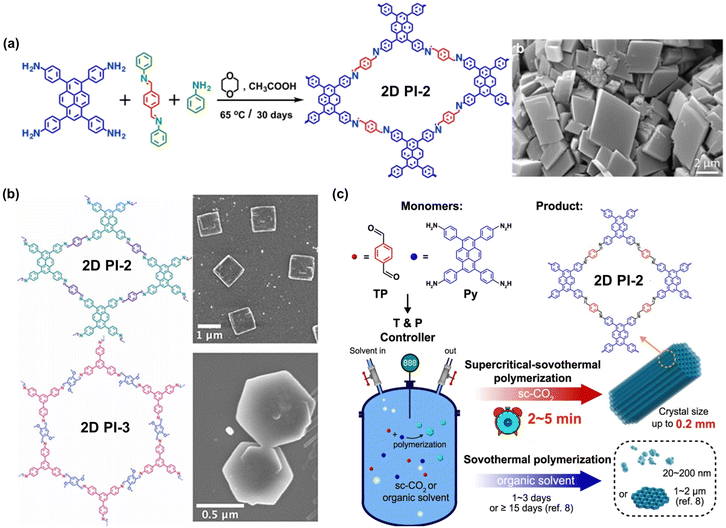 |
| Fig. 1 Representative 2D CP single crystals. (a) Planar building block gives easy single crystal synthesis of 2D PI-2 (ref. 45). (b) The synthesis of 2D PI-2 and 2D PI-3 single crystals at ambient pressure and short reaction times (ref. 49). Adapted with permission American Chemical Society. (c) Schematic of the 2D PI-2 single crystals in supercritical CO2 (ref. 44). | |
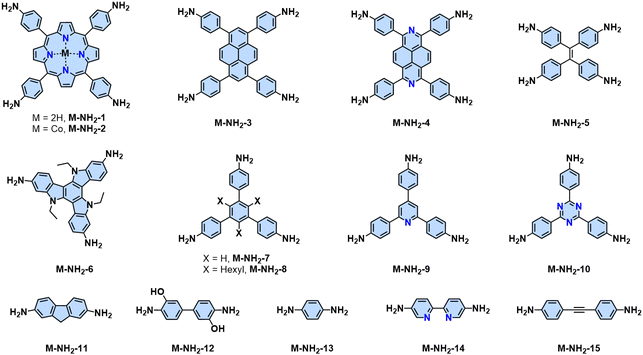 |
| Fig. 2 Representative monomers contain –NH2 groups for constructing 2D PIs. | |
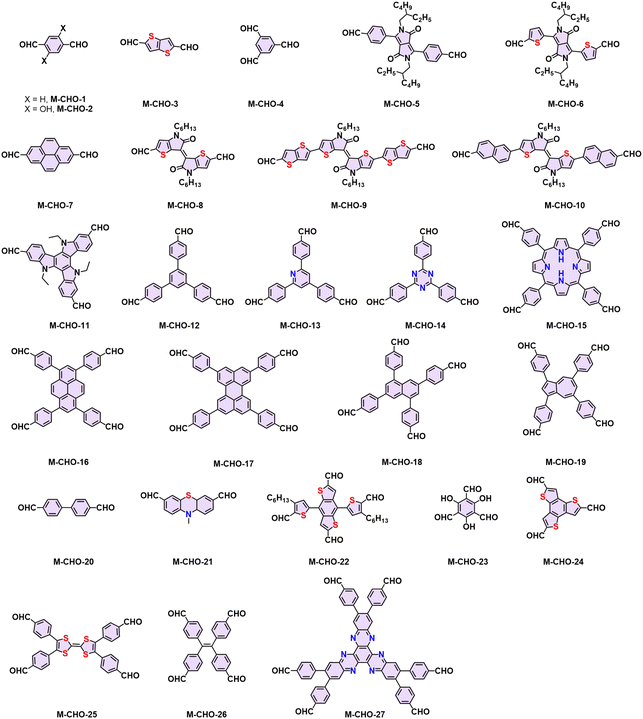 |
| Fig. 3 Representative aromatic aldehydes for constructing 2D PIs and 2D PAVs. | |
2D PI films have been synthesized using on-surface and interfacial synthesis methods. Our group prepared porphyrin-containing monolayer and multilayer 2D CPs from M-NH2-1/2 and 2,5-dihydroxyterephthalaldehyde (M-CHO-2) at an air/water and liquid/liquid interface, respectively.53 Both the monolayer and multilayer 2D CPs have crystalline structures as indicated by selected area electron diffraction. The monolayer 2D CP has a thickness of ∼0.7 nm with a lateral size of 4-inch wafer and a Young's modulus of 267 ± 30 GPa. The field-effect transistor fabricated from this 2D CP shows a charge mobility of 1.3 × 10−6 cm2 V−1 s−1, which can be increased over 100 times after doping with iodine. Later, various highly crystalline and large-area 2D PIs were developed via the SMAIS method, showing tunable thicknesses (1 to 100 nm) and large crystalline domain sizes (up to 120 μm2).50,54–57
3.2 2D PI derivatives by post-modification or one-pot synthesis
The high reversibility of imine linkages would conversely endow poor π-electron delocalization due to the polarized C
N bonds and poor stability against strong acids/bases. Consequently, different post-synthetic methods have been developed to enhance the stability and the conjugation of 2D PIs or imine-linked intermediates (Table 1). For example, Liu and colleagues reported a facile and general strategy to transform imine-linked 2D PIs into a series of quinoline-linked 2D CPs utilizing an aza-Diels–Alder cycloaddition reaction, reacting with various aryl alkynes.58 Yaghi and coworkers converted imine-linked 2D PIs based on 1,4-phenylenediamine (M-NH2-13) and 1,3,6,8-tetrakis(4-formylphenyl)pyrene (M-CHO-16) through consecutive linker substitution and oxidative cyclization to two isostructural 2D CPs, having thiazole and oxazole linkages.59 Zhao and colleagues next reported an approach for constructing thieno[3,2-c]pyridine-linked 2D CPs, featuring a combination of imine formation and a post-oxidative cyclization.60 These resultant 2D CPs generally exhibit enhanced chemical stability and have enabled them to visualize the periodic structural characteristics as honeycomb-like porous structures by high-resolution TEM (HR-TEM) after transformation. However, the corresponding 2D PI counterparts are extremely unstable under the electron beam, and HR-TEM images could not be obtained so far.
Despite the enhanced conjugation, the post-modification approach frequently results in framework collapse and reduced crystallinity during solid-state transformations, which ultimately compromises the physicochemical properties of 2D CPs. As an alternative approach, multicomponent one-pot or de novo synthesis via imine intermediates has the potential to yield crystalline 2D CPs from the outset, thereby circumventing the inefficiencies associated with solid-state post-modification. This method can also create 2D CPs that are otherwise unattainable through post-modification.61–63 Cooper and his coworkers developed a simple and efficient three-component assembly reaction between readily available aldehydes, amines, and elemental sulfur via a C–H functionalization and oxidative annulation under transition-metal-free conditions.61 Wang proposed cascade reactions to construct stable and crystalline benzoxazole-linked 2D CPs.64 Xiang and coworkers prepared non-substituted quinoline-linked 2D CPs through post-synthetic modification strategies and one-pot synthesis methods.65 The mechanism for the construction of non-substituted quinoline linkages starts with the formation of an imine through the condensation of aldehyde and amine, followed by Rh-catalyzed [4 + 2] annulation between the in situ generated imine and vinylene carbonate. In addition, Dong and coworkers successfully synthesized phenyl-substituted quinoline-linked 2D CPs through one-pot aza-Diels–Alder cycloaddition (Povarov) reactions under solvothermal conditions in high yields.66 Wang and coworkers utilized the representative Groebke–Blackburn–Bienaymé (GBB) reaction based on isocyanide chemistry to construct a series of pyrimidazole-based COFs in one step from isocyanide, aminopyridine, and aldehyde monomers.67 The formation of fused imidazole rings within the frameworks is ubiquitous, ensuring excellent chemical stability (Table 1).
3.3 2D polypyrazines (2D PPZs) by Schiff-base reaction
The Schiff-base reaction mechanism is also employed for the synthesis of 2D PPZs, utilizing monomers with multiple amine groups and ketone (–CO) groups at the -ortho position.68,69 In 2013, Jiang reported a triphenylene-based 2D PPZs (CS-COF, designated as 2D PPZ-1 in this review) that exhibits a fully fused backbone and semiconducting character (Scheme 2a).68 Later, Baek and coworkers reported the synthesis of 2D PPZ-2 (C2N-h2D) crystals through the condensation of benzene-1,2,3,4,5,6-hexaamine (M-NH2-19) and cyclohexane-1,2,3,4,5,6-hexaone (M-CO-1) (Scheme 2b).69 Other amino monomers including triphenylene-2,3,6,7,10,11-hexaamine (M-NH2-19), 2,3,9,10,16,17,23,24-octaaminophthalocyaninato Zn/Cu(II) (M-NH2-20/21), and ketone monomers including 2,7-di-tert-butylpyrene-4,5,9,10-tetraone (M-CO-3) and 2,7,12,17,22,27-hexa-tert-butylphenanthro[4,5-abc]phenanthro[4′,5′:5,6,7,8]quinoxalino[2,3-h]phenanthro[4′,5′:5,6,7,8]-quinoxalino[2,3-j]phenazine-9,10,19,20,29,30-hexaone (M-CO-5), were also developed for the synthesis of 2D PPZs (Fig. 4).
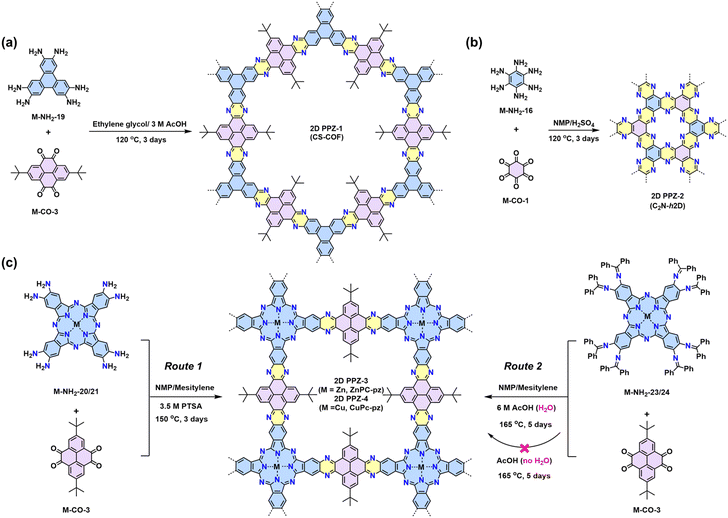 |
| Scheme 2 (a) Synthesis of the first crystalline pyrazine-bridged 2D polypyrazine of 2D PPZ-1 (CS-COF). (b) Synthesis of 2D PPZ-2 crystals. (c) Two routes to synthesize 2D PPZ-3 (ZnPc-pz) and 2D PPZ-4 (CuPc-pz) with cubic lattice. | |
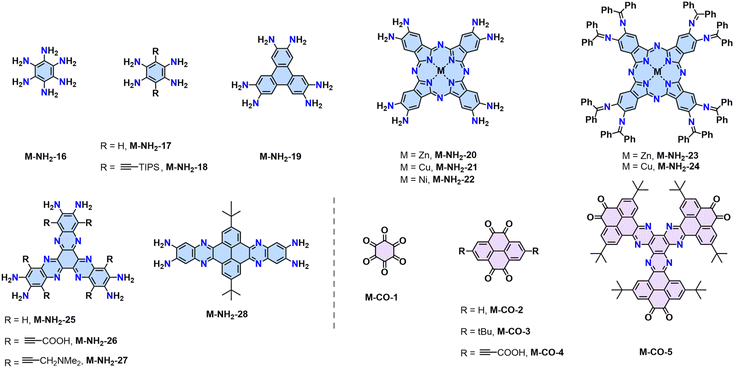 |
| Fig. 4 Representative monomers contain multiple –NH2 (blue) and –C O (pink) groups at the -ortho positions to construct 2D PPZs. | |
In 2019, our group demonstrated two metal-phthalocyanine-based 2D PPZs, namely ZnPc-pz COF and CuPc-pz COF (2D PPZ-3 and 2D PPZ-4), via the direct Schiff-base condensation between M-NH2-20/21 and M-CO-3. pXRD analysis reveals the crystalline nature of both 2D PPZs with a = b = 22.2 Å and an interplane distance of ca. 3.30 Å for a serrated AA stacking mode.70 2D PPZ-3 and 2D PPZ-4 are p-type semiconductors with an optical band gap of ∼1.2 eV and charge mobility of ∼5 cm2 V−1 s−1.70–72 Reversible p-doping of the materials with iodine can enhance not only the electrical conductivity but also the charge carrier scattering time and mobility of 2D PPZs.73 Additionally, they can also be synthesized through a multistep Schiff-base reaction from the –N
CPh2 protected amine monomers (precursors of M-NH2-20/21 with enhanced solubility), which undergo the hydrolysis reaction of Schiff-base (–N
CPh2) to amine (–NH2) prior to following Schiff-base condensation between –NH2 and ketone (Scheme 2c). Moreover, Mateo-Alonso and colleagues developed two 2D PPZs with semiconducting properties through polycondensation in the combination of M-NH2-16/M-CO-3 and M-NH2-28/M-CO-5, respectively.73 Besides, the enriched sp2-N makes 2D PPZs suitable cathodes in aqueous Zn-ion batteries, as illustrated in Zhu's work.74
3.4 2D poly(arylene vinylene)s (2D PAVs) by various polycondensation reactions
In comparison to 2D PIs and 2D PPZs, 2D PAVs with robust polymeric skeletons have demonstrated inherently improved chemical stability and 2D π-conjugation, which have attracted significant interest over the last decade. Nevertheless, the synthesis of highly crystalline 2D PAVs remains challenging, given that the formation of vinylene linkages is semi-reversible, in contrast to the well-established Schiff-base chemistry with high reversibility. The current focus of 2D PAV development is to establish methodologies and design novel building blocks that facilitate the formation of highly crystalline structures. To date, a number of synthetic methodologies such as Knoevenagel,18,75–77 Aldol-type,78–82 HWE,83 and Wittig84 polycondensations have been developed for this class of materials (Scheme 3).
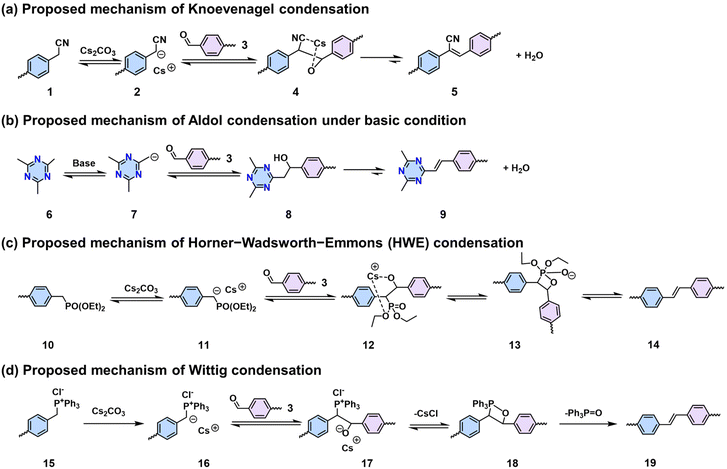 |
| Scheme 3 Methodologies and proposed mechanisms of (a) Knoevenagel, (b) Aldol-type, (c) HWE, and (d) Wittig condensations that can construct 2D PAVs. | |
3.4.1 Knoevenagel 2D polycondensation. Knoevenagel condensation is a powerful tool for the construction of 2D PAVs. In a Knoevenagel reaction, the α-carbon atom of the aromatic methylene nitrile (1) can be easily converted to a reactive intermediate carbanion (2) under basic conditions and further stabilized through the conjugation effect. This carbanion will then nucleophilically attack the carbonyl group of (3) to form a C–C bond-linked intermediate (4). Finally, the elimination reaction releases a water molecule to establish the C
C bond (5) simultaneously (Scheme 3a). In 2016, our group reported the first highly crystalline cyano-vinylene-linked 2D poly(phenylene vinylene) (2D PPV, named as 2D PAV-1 in this Review) based on p-phenylenediacetonitrile (M-CH2CN-1) and 1,3,5-tris(4-formylphenyl)benzene (M-CHO-12) (Scheme 4a).18 The formation of cyano-vinylene linkages was identified by solid-state 13C-NMR and infrared spectroscopy. The exact structure of 2D PAV-1 is proposed to adopt a serrated stacking mode with neighboring layers slipped by 1/4 of the unit cell distance on average, and a distance of 0.35 nm separates the layers. The catalyst plays an important role in determining the reversibility of the vinylene linkages formation, and only the Cs+ ion can stabilize the carbanion intermediate (4), endowing quasi-reversible C–C bond formation and thus promoting the formation of a highly crystalline structure through a quasi-reversible self-healing process (Scheme 3a).85 At the same time, Jiang and colleagues reported 2D PAV-2 (sp2-c-COF) synthesized by Knoevenagel polycondensation between M-CH2CN-1 and 4,4′,4′′,4′′′-(pyrene-1,3,6,8-tetrayl)tetrabenzaldehydepyrene (M-CHO-16) (Scheme 4b).75 The lattice parameters for 2D PAV-2 are a = 34.4632 Å, b = 35.4951 Å, c = 3.7199 Å, α = γ = 90°, and β = 104.0277°, with a layer spacing of 3.58 Å in the z-direction. Doping with iodine, high-density radicals can be generated in 2D PAV-2, leading to a largely improved electrical conductivity from 6.1 × 10−14 S m−1 to 7.1 × 10−2 S m−1.
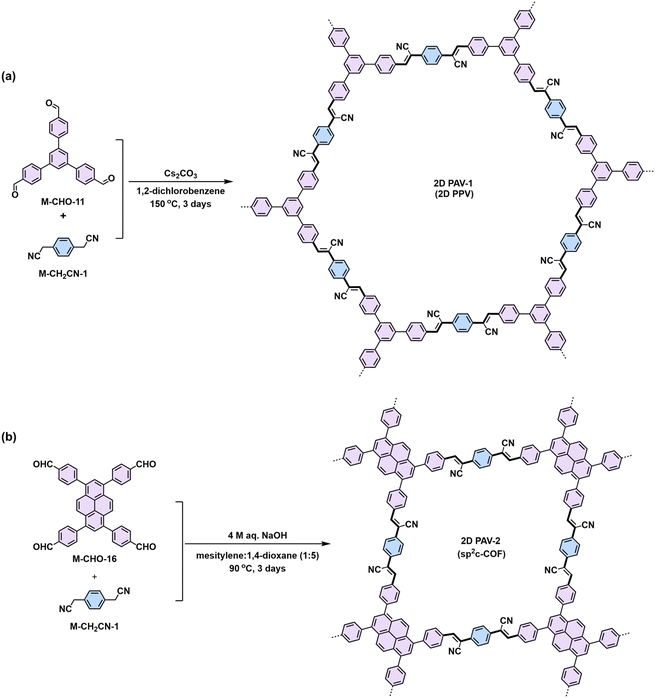 |
| Scheme 4 Synthesis of (a) 2D PAV-1 (2D PPV) with hexagonal lattice and (b) 2D PAV-2 (sp2c-COF) with 2D square lattice. | |
In the last several years, several aromatic aldehyde monomers and acetonitrile with different geometries have been successfully implemented in the synthesis of crystalline cyano-substituted 2D PAVs (Fig. 3 and 5), such as C2-symmetrical 4,8-bis(5-formyl-4-hexylthiophen-2-yl)benzo[1,2-b:4,5-b′]dithiophene-2,6-dicarbaldehyde (M-CHO-22),86 C3-symmetrical 4,4′,4′′-(1,3,5-triazine-2,4,6-triyl)tribenzaldehyde (M-CHO-14),87 benzo[1,2-b:3,4-b′:5,6-b′′]trithiophene-2,5,8-tricarbaldehyde (M-CHO-24),88 and 2,3,8,9,14,15-hexa(4-formylphenyl)diquinoxalino[2,3-a:2′,3′-c]phenazine (M-CHO-27),89 C4-symmetrical 4,4′,4′′,4′′′-(porphyrin-5,10,15,20-tetrayl)tetrabenzaldehyde (M-CHO-15),76 and 4,4′,4′′,4′′′-(perylene-2,5,8,11-tetrayl)tetrabenzaldehyde (M-CHO-17),90 as well as C2-symmetrical 2,2′-bipyridine-based 5,5-bis(cyanomethyl)-2,2-bipyridine (M-CH2CN-3)91 and 2,2′([2,2′-bithiophene]-5,5′-diyl)diacetonitrile (M-CH2CN-6),86 and 2,2′-(benzo[1,2-d:4,5-d′]bis(thiazole)-2,6-diyl)diacetonitrile (M-CH2CN-9).77
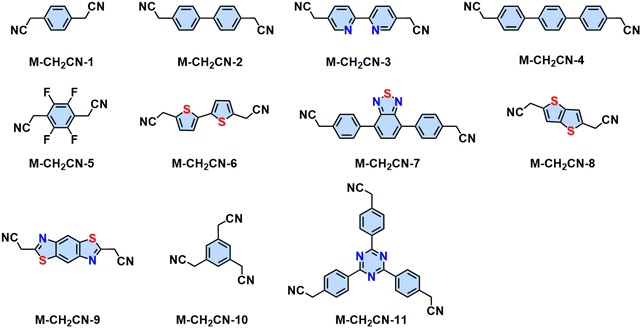 |
| Fig. 5 Representative aromatic methylene nitrile for the synthesis of 2D PAVs utilizing Knoevenagel polycondensation. | |
Highly crystalline cyano-substituted 2D PAVs can be synthesized by combining Knoevenagel polycondensation and water-assisted Michael addition–elimination.92 Through in situ high-temperature NMR measurements of model reactions and intermediate, we disclosed that Michael-addition–elimination is an efficient dynamic covalent chemistry for the direct C
C bond exchange, which endows the self-correction property to synthesize complex structures. The deep understanding of the reaction mechanism further guides the synthesis of four highly crystalline 2D PAVs with crystalline domain sizes ranging from 20 to 100 nm. Jiang proposed synthesizing crystalline 2D PAVs using a topology-templated synthesis strategy.93 In this strategy, the corresponding imine-linked 2D PIs were used as templates to facilitate and confine the growth of the cyano-substituted 2D PAVs to the x–y plane. Afterward, crystalline cyano-substituted 2D PAVs were obtained after hydrolyzing the corresponding 2D PI template. This template-assisted strategy can also synthesize crystalline 2D PAVs that are inaccessible through direct polymerization. Moreover, Hecht and colleagues demonstrated the on-surface synthesis of a single-layer 2D PAV through dynamic Knoevenagel polycondensation between 2,2′,2′′-((1,3,5-triazine-2,4,6-triyl)tris(benzene-4,1-diyl))triacetonitrile (M-CH2CN-11) and terephthalaldehyde. High-resolution STM characterizations reveal a covalently connected honeycomb lattice with domains up to 70 nm.94
Unlike the other 2D CPs constructed by the dynamic Knoevenagel polycondensation, Gu et al. reported the synthesis of crystalline 2D CPs (GS-COF-1 and GS-COF-2) by connecting hydroxybenzene-aldehyde and acetonitrile building blocks to form cyano-substituted benzofuran linkages.95 In the initial process, the Knoevenagel condensation reversibly generates the frameworks, whereas consecutive cyanide migration, ring-closure, and oxidation reactions yield an irreversible heteroaromatic cyano-substituted benzofuran linkage (Scheme 5).
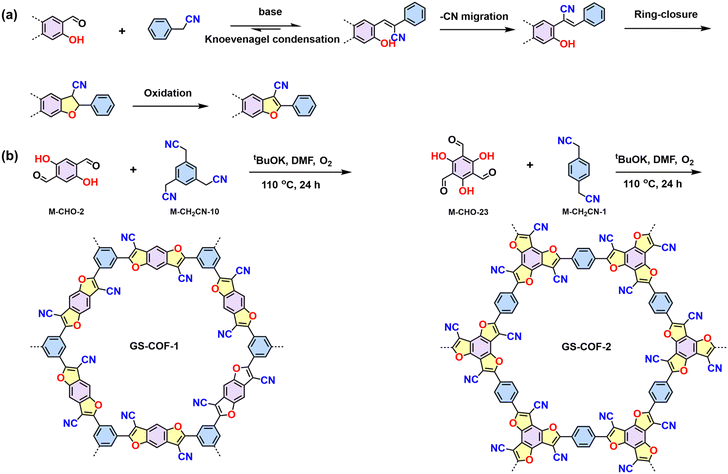 |
| Scheme 5 (a) Proposed mechanism of benzodifuran linkage formation. (b) Synthesis of GS-COF-1 and GS-COF-2. | |
Combining dynamic Knoevenagel condensation and Claisen–Schmidt reaction in one pot can build cyano-substituted buta-1,3-diene linked 2D PAVs, as illustrated by Gu's work.96 They initially synthesized a model compound with cyano-equipped buta-1,3-diene-linkage (Scheme 6a), which is almost coplanar with a slight torsion angle of 15.09° between one benzene ring and the buta-1,3-diene. The near-planar structure of buta-1,3-diene promotes the formation of layer-stacked 2D PAV-3 (Scheme 6b) condensed from consecutive Claisen–Schmidt reaction and Knoevenagel condensation.
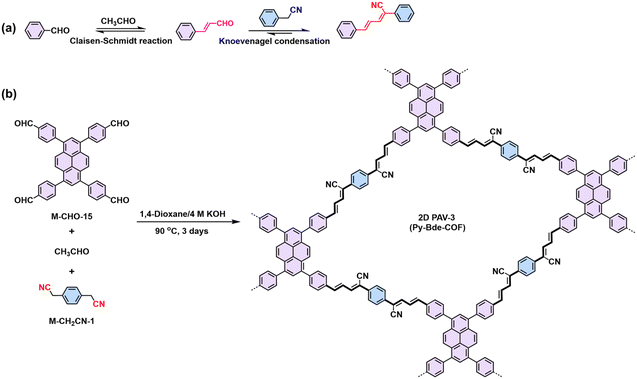 |
| Scheme 6 (a) Synthesis of the model compound using Knoevenagel condensation and Claisen–Schmidt reaction in one pot. (b) Synthesis of CN-equipped buta-1,3-diene-linked 2D PAV-3 (Py-Bde-COF). | |
Regarding electronic applications, 2D PAVs with excellent charge transporting properties are highly desirable. Considering the thiophene-enriched building blocks feature highly planar backbone and strengthened p-orbital interactions, our group demonstrated the first example of fully thiophene (thienyl-benzodithiophene, M-CHO-22)-based 2D PAVs of 2D PAV-4 (2DPAV-BDT-BT, BT = bithiophene) and 2D PAV-5 (2DPAV-BDT-BP, BP = biphenyl) via Knoevenagel polycondensation (Scheme 7).86 Compared to the biphenyl-bridged 2D PAV-5, the fully thiophene-based 2D PAV-4 exhibits enhanced planarity, π-delocalization with a reduced band gap (1.62 eV), and significant electronic band dispersion, as revealed by the optical absorption and density functional theory calculations of electronic band structures. Remarkably, temperature-dependent terahertz spectroscopy discloses a unique band-like transport and excellent room-temperature charge carrier mobility of 65 cm2 V−1 s−1 for the fully thiophene-based 2D PAV, exceeding the reported 2D CPs in powder form. Moreover, some acceptor units, including benzothiadiazole,97 benzobisthiazole,98 benzothiadiazole,99 2,2′-bipyridine,91 1,2,4-thiadiazole,99 and triazine100 have been incorporated into 2D PAVs for improved charge separation and reduced band gap.
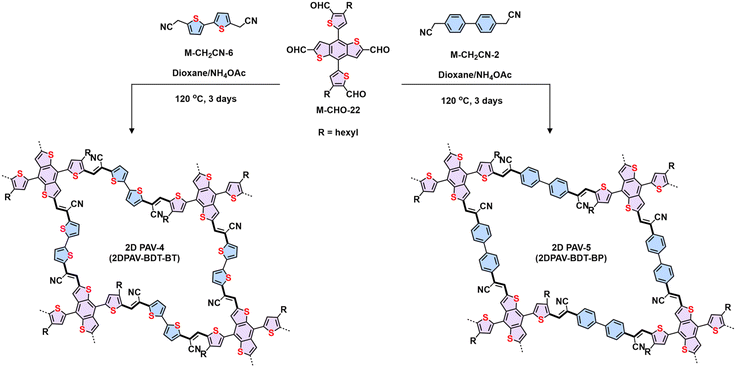 |
| Scheme 7 Synthesis of 2D PAV-4 (2DPAV-BDT-BT) and 2D PAV-5 (2DPAV-BDT-BP). | |
The majority of 2D PAVs are obtained as polycrystalline powders by solvothermal synthesis, which exhibit poor processability, hindering their integration into devices. Our group prepared 2D PAV films using the SMAIS method via Knoevenagel polycondensation conducted under a three-step methodology: (i) formation of surfactant monolayer on the water surface; (ii) injection and adsorption of the aldehyde monomer under the surfactant monolayer; (iii) injection and adsorption of aromatic methylene nitrile monomer; (iv) subsequent 2D polymerization. The synthesized films have a large lateral size (∼28 cm2), tunable thickness (∼2–52 nm), and high chemical stability. They can be used as an anion-selective electrode coating for reversible and durable zinc-based dual-ion batteries.39 DFT calculations demonstrated that, in comparison to the synthesis in an aqueous solution, the on-water surface synthesis exhibits lower energy barriers for intermediates, indicating the considerable influence of the 2D confinement provided by the air/water interface, which effectively constrains the out-of-plane molecule movement.
3.4.2 Aldol-type 2D polycondensation. In contrast to synthesizing cyano-substituted 2D PAVs using Knoevenagel 2D polycondensation, other Aldol-type (or extended Knoevenagel) polycondensation reactions enable the synthesis of unsubstituted-vinylene-linked 2D PAVs. As shown in Scheme 3b, a symmetric and electron-deficient methyl monomer (e.g., 4,6-trimethyl-1,3,5-triazine, the compound 6) is essential for Aldol-type condensation, which can be readily deprotonated into a reactive carbanion species (7) by various bases (e.g., NaOH, piperidine, dimethylamine). Then the aldehyde group of compound (3) is attacked by this nucleophilic intermediate, initiating a reaction that culminates in forming a C–C bond, yielding compound (8). Subsequent elimination generates water and unsubstituted vinylene-linked aromatic frameworks (9).In 2019, Yaghi,80 Thomas,78 Perepichka,82 and Zhang101 reported the Aldol-type polycondensation method for the synthesis of 2D PAVs independently. For example, 2D PAV-6 (COF-701), was achieved by trifluoroacetic acid (TfOH)-catalyzed condensation between 2,4,6-trimethyl-1,3,5-triazine (M-CH3-1) and 4,4′-biphenyldicarbaldehyde (M-CHO-20) (Scheme 8a). Thomas and colleagues obtained two unsubstituted-vinylene linked 2D PAVs using base-catalyzed Aldol-type polycondensation between M-CH3-1 and terepthaldehyde (M-CHO-1)/1,3,5-tris(4-formylphenyl)benzene (M-CHO-11).78 Then, they combined cyclotrimerization of nitrile and Aldol-type condensation in one pot (Scheme 8b) and achieved crystalline 2D PAV-6 with a BET surface area of 736 m2 g−1.80 Other electron-deficient monomers, such as 2,4,6-trimethylpyridine derivatives (M-CH3-3 and M-CH3-4),101–104 2,4,6-trimethylbenzene-1,3,5-tricarbonitrile (M-CH3-9),105 benzodifurandione (M-CH3-11),106 2,6-dimethylbenzo[1,2-d:4,5-d′]bis(oxazole) (M-CH3-12),97 and 2,6-dimethylbenzo[1,2-d:4,5-d′]bis(thiazole) (M-CH3-13),98 and s-indacene-1,3,5,7(2H,6H)-tetraone (M-CH3-14)107 have also been successfully used for the construction of crystalline 2D PAVs (Fig. 6).
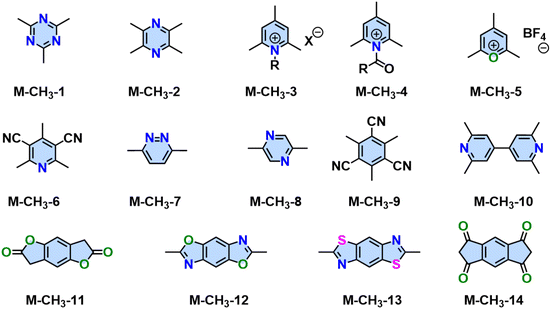 |
| Fig. 6 Representative aromatic monomers with active methyl groups for synthesizing 2D PAVs utilizing Aldol-type 2D polycondensation. | |
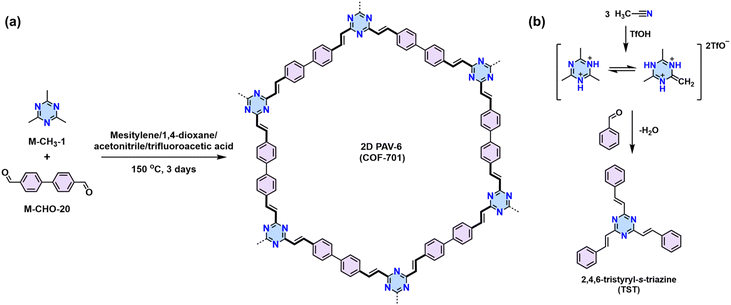 |
| Scheme 8 (a) Synthesis of the first un-substituted vinylene-linked 2D PAV-5 (COF-701). (b) Combination of cyclotrimerization and Aldol-type condensation to synthesize the model compound of 2,4,6-tristyryl-s-triazine (TST). | |
Aldol-type polycondensation also represents a powerful strategy to synthesize 2D PAVs on a large scale or directly as a thin film on substrates. In 2021, Zhang and colleagues developed a solvent-free method to synthesize 2D PAVs using benzoic anhydride as a catalyst.79 Later, Zhang et al. developed a simple and robust interfacial methodology for fabricating 2D PAV films on various solid substrates (e.g., fluorine-doped tin oxide, aluminum sheet, polyacrylonitrile membrane).108 The resultant 2D PAV films show lateral sizes up to 120 cm2 and tunable thickness from tens of nanometers to a few micrometers.
3.4.3 HWE and Wittig 2D polycondensation. Another methodology for synthesizing 2D PAVs is the HWE polycondensation as demonstrated by our group.109 The HWE-based 2D polymerization between aromatic phosphonate and aldehyde represents a more robust way to synthesize unsubstituted 2D PAVs with different topologies. The mechanism of the HWE reaction is proposed to involve three steps and a stabilized six-membered cyclic transition state with Cs+ (12), which includes an exclusive trans-vinylene bond (14) after the elimination step (Scheme 3c). Moreover, DFT simulations suggest that C–C single-bond formation is reversible (from 11 and 3 to 13), which is crucial to the formation of crystalline 2D PAVs (2D PAV-7 and 2D PAV-8, Scheme 9). Compared to cyano-vinylene-linked 2D PAV-11 developed by Knoevenagel polycondensation, 2D PAV-7 exhibits a narrower band gap (2.20 eV vs. 2.39 eV) and a bathochromic shift in photoluminescence of ca. 65 nm, indicative of an enhanced 2D conjugation in the latter. Besides the formation of crystalline 2D PAVs mentioned above, an in-depth exploration of the mechanism is needed to expand the monomer scope suitable for HWE polycondensation.
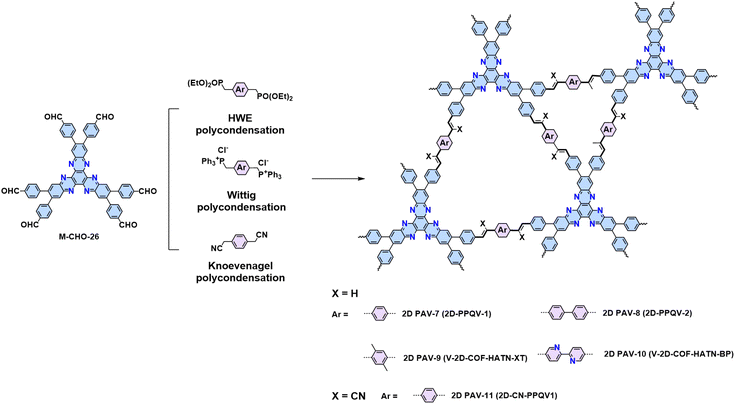 |
| Scheme 9 Synthesis of 2D PAV-7 (2D-PPQV-1), 2D PAV-8 (2D-PPQV-2), 2D PAV-9 (V-2D-COF-HATN-XT), and 2D PAV-10 (V-2D-COF-HATN-BP) through HWE or Wittig polymerization, together with 2D PAV-11 synthesized by Knoevenagel polycondensation. | |
Similarly, the Wittig reaction from phosphonium ylides and aryl aldehydes has proved to be a useful strategy for constructing crystalline 2D PAVs (Scheme 3d).84 The (trans/cis-) E/Z selectivity of aryl–ylide-based Wittig reactions is generally poor. Our group tailored the high E selectivity through (I) elevating temperature as an additional energy source to provide the thermodynamic driving force; (II) screening proper catalyst to obtain more stable intermediates with preferred geometry and associated facial selectivity during C
C bond formation.84 Four different 2D PAVs are successfully synthesized by combining 2,3,8,9,14,15-hexa(4-formylphenyl)diquinoxalino[2,3-a:2′,3′-c]phenazine (M-CHO-26) and four ylides (Scheme 9). The resultant 2D PAVs (2D PAV-7, 2D PAV-8, 2D PAV-9, and 2D PAV-10) present crystalline dual-pore structures and robust unsubstituted vinylene linkages with high chemical stability. THz spectroscopy reveals a charge carrier mobility of 10.3 cm2 V−1 s−1 for 2D PAV-9 at room temperature.
3.5 2D polyarylenes (2D PAs) by aryl–aryl coupling
Considering the different linking chemistries developed so far, the direct connection of aromatic moieties is advantageous to establish π-conjugation for efficient charge transfer. The synthesis of crystalline 2D CPs linked by C–C bonds has been considered one of the holy grails of synthetic chemistry.16,110,111 Both Au(111) and Ag(111) surfaces can promote aryl–aryl coupling. In 2009, our group and cooperation partner specifically designed a hexaiodo-substituted macrocycle cyclo-hexa-m-phenylene (CHP), which adopts an essentially planar conformation on the Ag(111) surface.17 Annealing to temperatures above 570 K initiates the coupling reaction. Six-fold aryl–aryl coupling of the CHP macrocycles results in a monolayer 2D polyphenylene (2D PA-1) with a 2D honeycomb network with a domain size up to 50 nm (Fig. 7a). The irreversible C–C bond formation prevents self-correction, which leads to many defects, i.e., 1D chains will be introduced into the expected 2D networks.
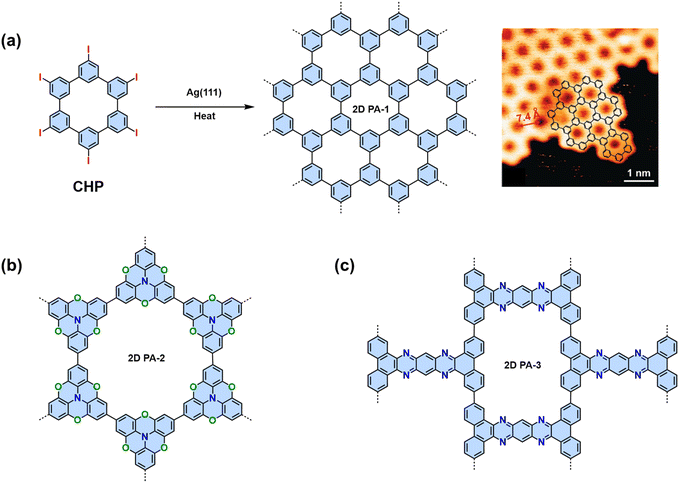 |
| Fig. 7 (a) On-surface synthesis of 2D polyphenylene (2D PA-1) and the corresponding STM image. (b) Chemical structure of 2D PA-2 synthesized on Au(111) surface. (c) Chemical structure of 2D PA-3 synthesized by solid-state synthesis. | |
In order to address the high defect density issue, Contini and coworkers realized mesoscale ordered 2D PAs by dosing rigid achiral heterotriangulene molecules (tribromotrioxaazatriangulene, TBTANG) on hot (210 °C) Au(111) surfaces (Fig. 7b). This process minimizes the formation of network defects and favors the growth of highly extended (>100 nm) hexagonal structures.112 The ordered and defect-less mesoscale structure facilitated the use of angle-resolved photoelectron spectroscopy for analyzing the 2D polymer. This technique disclosed the presence of both a Dirac cone and flat bands within their kagome lattices, rendering this 2D PA a fascinating arena for exploring phenomena such as the anomalous Hall effect, surface superconductivity, and superfluid transport.
In addition the on-surface synthesis on a metal substrate, in 2017, Loh and coworkers reported the solid-state synthesis of another 2D PA based on 2,7,13,18-tetrabromodibenzo[a,c]dibenzo[5,6:7,8]quinoxalino-[2,3-i]phenazine (2-TBQP) (Fig. 7c). Firstly, 2-TBQP was sublimated to generate needle-like single crystals of monomers with a zigzag and tightly packed lamellar structure through a displaced face-to-face π–π interaction at a distance of 3.269 Å.113 After holding the temperature at 520 °C for six hours, the monomer single crystals transformed into a shiny grey needle-shaped polymer. Scanning electron microscopy studies revealed that the bulk precursor 2-TBQP crystal underwent a transformation into polymers with apparent lamellar features. The embedded polymeric sheets are aligned parallel to the c-axis of the original precursor 2-TBQP crystal. The use of Scotch tape facilitates the exfoliation of these crystals into micrometer-sized, nanometer-thick sheets.
It should be noted that aryl–aryl coupling reactions are not limited to the Ullmann-type coupling. For example, monomers with terminal alkenyl or alkynyl groups, e.g., 2,4,6-tris(4-vinylphenyl)-1,3,5-triazine (TVTP),114 and 1,3,5-tris-(4-ethynylphenyl)benzene (TEPB),114 can trigger a cyclotrimerization towards the synthesis of 2D PAs on the gold surface. In addition, a 1,3,5-tris(4hydroxyphenyl)benzene (THPB)115 monomer can undergo an oxidative C–C homocoupling into 2D PA followed by a reductive cleavage of the hydroxyl groups on both the Au(111) and Ag(111) surfaces (Fig. 8).
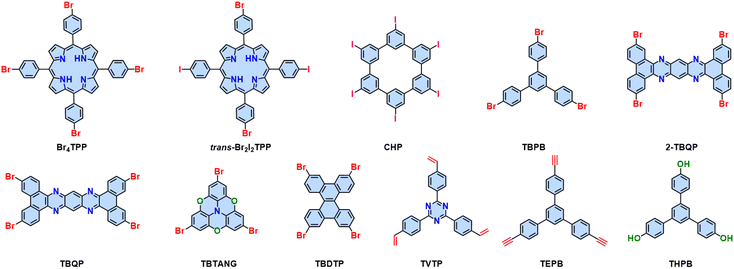 |
| Fig. 8 Building blocks investigated for the formation of 2D CPs on metal surfaces. | |
3.6 2D poly(benzimidazobenzophenanthroline)s (2D BBLs)
Ladder-type CPs, which consists of linearly fused aromatic subunits, are highly desirable due to the rigidity of the polymer backbone and the enhanced π-orbital overlapping. Charge delocalization is significantly facilitated because of the reduced conformational and energetic disorder endowed by the rigid “lock-in” backbone conformation.116 Theoretical calculations on various phthalocyanine-based model compounds and 1D CPs suggest a superior conjugation degree (i.e., an inferior highest occupied molecular orbital–lowest unoccupied molecular orbital gap) for the BBL-ladder-type structure to those model compounds/polymers linked by imines, pyrazines, vinylenes, etc. The formation of BBL-imidazole linkage involves two consecutive steps. The first step is the quasi-reversible formation of imide-intermediate, followed by irreversible intramolecular dehydration (Scheme 10a). However, due to the harsh conditions required for the imidazole ring formation from o-diamine and anhydride (e.g., temperatures more than 200 °C), the synthesis of crystalline 2D BBL has been a great challenge.116
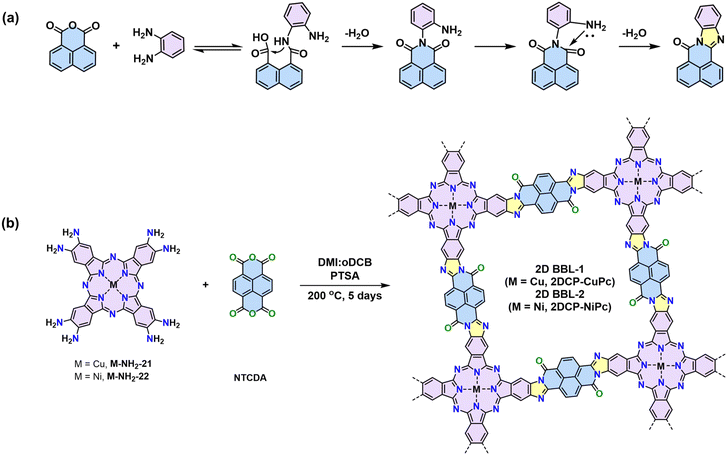 |
| Scheme 10 (a) Proposed reaction mechanism of imidazole linkages. (b) Synthesis of the two phthalocyanine-based BBL-ladder-type crystalline 2D CPs. | |
Our group reported the first crystalline 2D BBLs based on phthalocyanine building block.4 pXRD analysis reveals the crystalline nature with distinct diffractions from different crystallographic planes, corresponding to a slipped-AA stacking geometry. We disclosed that p-toluenesulfonic acid can improve the reactivity due to its unique protonation–deprotonation ability. Crystalline 2D BBL-1 and 2D BBL-2 were synthesized by polycondensation with PTSA as the catalyst (Scheme 10b). The achieved 2D BBL-1 and 2D BBL-2 show highly delocalized π-electrons and strongly dispersive electronic bands. They present narrow optical bandgaps of 1.3 eV and a unique band transport feature with high charge mobility of ∼970 cm2 V−1 s−1 at room temperature and ∼1390 cm2 V−1 s−1 at 78 K, demonstrating the great potential of 2D CPs in high-performance organic electronics. This condition can be generalized to the synthesis of other 2D BBLs, e.g., polycondensation between triphenylene-based M-NH2-19 and naphthalene tetracarboxylic dianhydride leads to another crystalline 2D BBL, which can be used for ultrafast electrochemical proton storage.40
4 Conclusions and outlook
Over the last decade, we have witnessed the rapid development of 2D CPs, not only as a new class of porous materials with long-range ordered structures but also as a playground for integrating pre-designed electronic and quantum functionalities. This review highlights the importance of synthetic methodologies to fully unlock the potential of 2D CPs through various linkage chemistry and the structural/topological designs. We commence with a summary of the most popular 2D PIs, outlining the efforts of the synthetic community in relation to Schiff-base chemistry. These efforts are directed towards enhancing conjugation and chemical stability, i.e., 2D PI derivatives by post-modification or multicomponent one-pot synthesis, and ladder-type 2D PPZs by consecutive Schiff-base reactions.
More importantly, 2D PAVs have experienced substantial advancement in the last few years, with the development of synthetic chemistry and the investigation of their physical properties. A variety of synthetic methodologies, including Knoevenagel, Aldol-type, HWE, and Wittig 2D polycondensation reactions, have been established for 2D PAVs with enlarged crystal domains. The replacement of C
N linkages in 2D PIs with C
C linkages in 2D PAVs not only enhances the stability by protecting it from nucleophile attack but also improves the conjugation and delocalization of the electron cloud by reducing the polarity of the linkages. To date, the synthesis of 2D PAs has predominantly involved the formation of C–C bonds on the metal surface. This unique surface chemistry can overcome the irreversibility of C–C coupling in traditional wet chemistry. However, the obtained thin layers usually show limited domain size and are unsuitable for further device integration. It is evident that new strategies, such as new monomer design, are still required to overcome the limitations associated with the polymer domain size. Finally, we illustrate the development of ladder-type 2D BBLs with unique band-like transport and exceptionally high charge carrier mobility, a “booster dose” to this field. Notwithstanding the considerable advances that have been made, a number of challenges remain to be addressed.
(1) As illustrated, the library of 2D CPs has considerably expanded with the development of novel building blocks and synthetic methodologies. Nonetheless, the design of 2D CPs to achieve charge mobility higher than 10 cm2 V−1 s−1 at the device level remains to be demonstrated. Molecular design strategies to facilitate π-delocalization, narrow band gap, and improve charge mobility are highly demanding. Novel planar conjugated building blocks, new conjugated linkages, and unique 2D lattice and stack modes are always welcome. Moreover, the donor–acceptor molecular design in 1D CPs can be adapted to develop the electron-deficient n-type 2D CPs.
(2) While narrow optical band gap and high charge carrier mobility are at reach by rationally tuning the structures of 2D PAVs and 2D BBLs, the role of intrinsic and extrinsic (e.g., boundaries and defects) contributions remains to be elucidated. It is imperative that the development of highly crystalline or single-crystalline 2D PAVs/BBLs be pursued before any attempt is made to establish structure–property relationships that are currently ambiguous. A more profound comprehension of the underlying reaction mechanisms is of paramount importance in order to regulate the reactivity and reversibility of monomers, which, in turn, is fundamental to the process of crystal growth. Before this objective can be achieved, it is essential to ascertain whether the crystallization of 2D CPs is thermodynamically or dynamically controlled. The deployment of in situ and ex situ characterization techniques, encompassing both spectroscopic and crystallographic analyses, will prove invaluable in elucidating the underlying processes occurring during crystallization.
(3) Solvothermal synthesis is a predominant approach for producing 2D CPs, yet they offer limited control over crystal growth, and the bulk samples obtained present challenges in terms of processing and integration into devices. The quality of exfoliated bulk crystals into nanosheets is still unsatisfactory, which relies on the development of 2D CPs with large crystal sizes and weak interlayer coupling, thus facilitating the exfoliation of 2D CP layers. Developing large-area, free-standing 2D CP films through wet interface-assisted strategies is beneficial for easy device integration. However, these interfacial strategies have been limited thus far, with successful syntheses heavily dependent on the suitable solubility of certain building blocks in water or organic solvents. In light of these considerations, the synthesis of processable 2D CPs and their integration into functional devices, e.g., field-effect transistors and bioelectronics, is urgently needed to drive future advancements in the field.
Data availability
No primary research results, software or code have been included and no new data were generated or analysed as part of this review.
Conflicts of interest
There are no conflicts to declare.
Acknowledgements
This work was financially supported by DFG project (CRC 1415, no. 417590517) and ERC grant (T2DCP, no. 819698).
References
- T. M. Swager, 50th Anniversary Perspective: Conducting/Semiconducting Conjugated Polymers. A Personal Perspective on the Past and the Future, Macromolecules, 2017, 50, 4867–4886 CrossRef CAS.
- L. Ye, S. Zhang, L. Huo, M. Zhang and J. Hou, Molecular design toward highly efficient photovoltaic polymers based on two-dimensional conjugated benzodithiophene, Acc. Chem. Res., 2014, 47, 1595–1603 CrossRef CAS PubMed.
- X. Guo, S. R. Puniredd, M. Baumgarten, W. Pisula and K. Mullen, Rational design of benzotrithiophene-diketopyrrolopyrrole-containing donor-acceptor polymers for improved charge carrier transport, Adv. Mater., 2013, 25, 5467–5472 CrossRef CAS PubMed.
- M. Wang, S. Fu, P. Petkov, Y. Fu, Z. Zhang, Y. Liu, J. Ma, G. Chen, S. M. Gali, L. Gao, Y. Lu, S. Paasch, H. Zhong, H. P. Steinruck, E. Canovas, E. Brunner, D. Beljonne, M. Bonn, H. I. Wang, R. Dong and X. Feng, Exceptionally high charge mobility in phthalocyanine-based poly(benzimidazobenzophenanthroline)-ladder-type two-dimensional conjugated polymers, Nat. Mater., 2023, 22, 880–887 CrossRef CAS PubMed.
- R. B. Rashid, A. M. Evans, L. A. Hall, R. R. Dasari, E. K. Roesner, S. R. Marder, D. M. D'Allesandro, W. R. Dichtel and J. Rivnay, A Semiconducting Two-Dimensional Polymer as an Organic Electrochemical Transistor Active Layer, Adv. Mater., 2022, 34, e2110703 CrossRef PubMed.
- Z. Wang, M. Wang, T. Heine and X. Feng, Electronic and quantum properties of organic two-dimensional crystals, Nat. Rev. Mater., 2024 DOI:10.1038/s41578-024-00740-8.
- M. A. Springer, T. J. Liu, A. Kuc and T. Heine, Topological two-dimensional polymers, Chem. Soc. Rev., 2020, 49, 2007–2019 RSC.
- S. Cheng, R. Zhao and D. S. Seferos, Precision Synthesis of Conjugated Polymers Using the Kumada Methodology, Acc. Chem. Res., 2021, 54, 4203–4214 CrossRef CAS PubMed.
- R. Zhao, J. Liu and L. Wang, Polymer Acceptors Containing B←N Units for Organic Photovoltaics, Acc. Chem. Res., 2020, 53, 1557–1567 CrossRef CAS PubMed.
- Y. Gao, X. Zhang, H. Tian, J. Zhang, D. Yan, Y. Geng and F. Wang, High Mobility Ambipolar Diketopyrrolopyrrole-Based Conjugated Polymer Synthesized Via Direct Arylation Polycondensation, Adv. Mater., 2015, 27, 6753–6759 CrossRef CAS PubMed.
- H. Xu, S. Ye, R. Zhao and D. S. Seferos, Homogeneous Synthesis of Monodisperse Sequence-Defined Conjugated Oligomers by Temperature Cycling, Angew. Chem., Int. Ed., 2022, 61, e202210340 CrossRef CAS PubMed.
- C. Qian, L. Feng, W. L. Teo, J. Liu, W. Zhou, D. Wang and Y. Zhao, Imine and imine-derived linkages in two-dimensional covalent organic frameworks, Nat. Rev. Chem., 2022, 6, 881–898 CrossRef CAS PubMed.
- J. R. H. Fernando, J. Uribe-Romo, H. Furukawa, C. Kloeck, M. O'Keeffe and O. M. Yaghi, A Crystalline Imine-Linked 3-D Porous Covalent Organic Framework, J. Am. Chem. Soc., 2009, 131, 4570–4571 CrossRef PubMed.
- Y. Li, W. Chen, G. Xing, D. Jiang and L. Chen, New synthetic strategies toward covalent organic frameworks, Chem. Soc. Rev., 2020, 49, 2852–2868 RSC.
- S. Wan, F. Gándara, A. Asano, H. Furukawa, A. Saeki, S. K. Dey, L. Liao, M. W. Ambrogio, Y. Y. Botros, X. Duan, S. Seki, J. F. Stoddart and O. M. Yaghi, Covalent Organic Frameworks
with High Charge Carrier Mobility, Chem. Mater., 2011, 23, 4094–4097 CrossRef CAS.
- W. Liu and K. P. Loh, Two-Dimensional Conjugated Polymers Based on C-C Coupling, Acc. Chem. Res., 2017, 50, 522–526 CrossRef CAS PubMed.
- M. Bieri, M. Treier, J. Cai, K. Ait-Mansour, P. Ruffieux, O. Groning, P. Groning, M. Kastler, R. Rieger, X. Feng, K. Mullen and R. Fasel, Porous graphenes: two-dimensional polymer synthesis with atomic precision, Chem. Commun., 2009, 6919–6921 RSC.
- X. D. Zhuang, W. X. Zhao, F. Zhang, Y. Cao, F. Liu, S. Bia and X. L. Feng, A two-dimensional conjugated polymer framework with fully sp2-bonded carbon skeleton, Polym. Chem., 2016, 7, 4176–4181 RSC.
- P. Kissel, D. J. Murray, W. J. Wulftange, V. J. Catalano and B. T. King, A nanoporous two-dimensional polymer by single-crystal-to-single-crystal photopolymerization, Nat. Chem., 2014, 6, 774–778 CrossRef CAS PubMed.
- M. J. Kory, M. Wörle, T. Weber, P. Payamyar, S. W. van de Poll, J. Dshemuchadse, N. Trapp and A. D. Schlüter, Gram-scale synthesis of two-dimensional polymer crystals and their structure analysis by X-ray diffraction, Nat. Chem., 2014, 6, 779–784 CrossRef CAS PubMed.
- R. Dong, T. Zhang and X. Feng, Interface-assisted synthesis of 2D materials: trend and challenges, Chem. Rev., 2018, 118, 6189–6235 CrossRef CAS PubMed.
- T. Ma, E. A. Kapustin, S. X. Yin, L. Liang, Z. Zhou, J. Niu, L.-H. Li, Y. Wang, J. Su and J. Li, Single-crystal X-ray diffraction structures of covalent organic frameworks, Science, 2018, 361, 48–52 CrossRef CAS PubMed.
- K. T. Tan, S. Ghosh, Z. Wang, F. Wen, D. Rodríguez-San-Miguel, J. Feng, N. Huang, W. Wang, F. Zamora and X. Feng, Covalent organic frameworks, Nat. Rev. Methods Primers, 2023, 3, 1 CrossRef CAS.
- J. Mahmood, E. K. Lee, M. Jung, D. Shin, I.-Y. Jeon, S.-M. Jung, H.-J. Choi, J.-M. Seo, S.-Y. Bae, S.-D. Sohn, N. Park, J. H. Oh, H.-J. Shin and J.-B. Baek, Nitrogenated holey two-dimensional structures, Nat. Commun., 2015, 6, 6486 CrossRef CAS PubMed.
- L. Lafferentz, V. Eberhardt, C. Dri, C. Africh, G. Comelli, F. Esch, S. Hecht and L. Grill, Controlling on-surface polymerization by hierarchical and substrate-directed growth, Nat. Chem., 2012, 4, 215–220 CrossRef CAS PubMed.
- G. Galeotti, F. De Marchi, E. Hamzehpoor, O. MacLean, M. Rajeswara Rao, Y. Chen, L. V. Besteiro, D. Dettmann, L. Ferrari, F. Frezza, P. M. Sheverdyaeva, R. Liu, A. K. Kundu, P. Moras, M. Ebrahimi, M. C. Gallagher, F. Rosei, D. F. Perepichka and G. Contini, Synthesis of mesoscale ordered two-dimensional π-conjugated polymers with semiconducting properties, Nat. Mater., 2020, 19, 874–880 CrossRef CAS PubMed.
- M. Liu, Y. Liu, J. Dong, Y. Bai, W. Gao, S. Shang, X. Wang, J. Kuang, C. Du, Y. Zou, J. Chen and Y. Liu, Two-dimensional covalent organic framework films prepared on various substrates through vapor induced
conversion, Nat. Commun., 2022, 13, 1411 CrossRef CAS PubMed.
- M. Choe, J. Y. Koo, I. Park, H. Ohtsu, J. H. Shim, H. C. Choi and S. S. Park, Chemical vapor deposition of edge-on oriented 2D conductive metal–organic framework thin films, J. Am. Chem. Soc., 2022, 144, 16726–16731 CrossRef CAS PubMed.
- J. P. Daum, A. Ajnsztajn, S. A. Iyengar, J. Lowenstein, S. Roy, G.-h. Gao, E. H. R. Tsai, P. M. Ajayan and R. Verduzco, Solutions are the problem: ordered two-dimensional covalent organic framework films by chemical vapor deposition, ACS Nano, 2023, 17, 21411–21419 CrossRef PubMed.
- C. N. R. Rao and K. P. Kalyanikutty, The liquid–liquid interface as a medium to generate nanocrystalline films of inorganic materials, Acc. Chem. Res., 2008, 41, 489–499 CrossRef CAS PubMed.
- R. Yang, S. Liu, Q. Sun, Q. Liao, K. Xi and B. Su, Potential difference-modulated synthesis of self-standing covalent organic framework membranes at liquid/liquid interfaces, J. Am. Chem. Soc., 2022, 144, 11778–11787 CrossRef CAS PubMed.
- Y. Zhong, B. Cheng, C. Park, A. Ray, S. Brown, F. Mujid, J.-U. Lee, H. Zhou, J. Suh, K.-H. Lee, A. J. Mannix, K. Kang, S. J. Sibener, D. A. Muller and J. Park, Wafer-scale synthesis of monolayer two-dimensional porphyrin polymers for hybrid superlattices, Science, 2019, 366, 1379–1384 CrossRef CAS PubMed.
- R. Dong, M. Pfeffermann, H. Liang, Z. Zheng, X. Zhu, J. Zhang and X. Feng, Large-area, free-standing, two-dimensional supramolecular polymer single-layer sheets for highly efficient electrocatalytic hydrogen evolution, Angew. Chem., Int. Ed., 2015, 54, 12058–12063 CrossRef CAS PubMed.
- H. Sahabudeen, H. Qi, B. A. Glatz, D. Tranca, R. Dong, Y. Hou, T. Zhang, C. Kuttner, T. Lehnert and G. Seifert, Wafer-sized multifunctional polyimine-based two-dimensional conjugated polymers with high mechanical stiffness, Nat. Commun., 2016, 7, 13461 CrossRef CAS PubMed.
- K. Liu, H. Qi, R. Dong, R. Shivhare, M. Addicoat, T. Zhang, H. Sahabudeen, T. Heine, S. Mannsfeld, U. Kaiser, Z. Zheng and X. Feng, On-water surface synthesis of crystalline, few-layer two-dimensional polymers assisted by surfactant monolayers, Nat. Chem., 2019, 11, 994–1000 CrossRef CAS PubMed.
- H. Qi, H. Sahabudeen, B. Liang, M. Položij, M. A. Addicoat, T. E. Gorelik, M. Hambsch, M. Mundszinger, S. Park, B. V. Lotsch, S. C. B. Mannsfeld, Z. Zheng, R. Dong, T. Heine, X. Feng and U. Kaiser, Near–atomic-scale observation of grain boundaries in a layer-stacked two-dimensional polymer, Sci. Adv., 2020, 6, eabb5976 CrossRef CAS PubMed.
- H. Sahabudeen, H. Qi, M. Ballabio, M. Položij, S. Olthof, R. Shivhare, Y. Jing, S. Park, K. Liu, T. Zhang, J. Ma, B. Rellinghaus, S. Mannsfeld, T. Heine, M. Bonn, E. Cánovas, Z. Zheng, U. Kaiser, R. Dong and X. Feng, Highly crystalline and semiconducting imine-based two-dimensional polymers enabled by interfacial synthesis, Angew. Chem., Int. Ed., 2020, 59, 6028–6036 CrossRef CAS PubMed.
- Z. Wang, Z. Zhang, H. Qi, A. Ortega-Guerrero, L. Wang, K. Xu, M. Wang, S. Park, F. Hennersdorf, A. Dianat, A. Croy, H. Komber, G. Cuniberti, J. J. Weigand, U. Kaiser, R. Dong and X. Feng, On-water surface synthesis of charged two-dimensional polymer single crystals via the irreversible Katritzky reaction, Nat. Synth., 2022, 1, 69–76 CrossRef.
- Y. Yang, D. Sabaghi, C. Liu, A. Dianat, D. Mucke, H. Qi, Y. Liu, M. Hambsch, Z. K. Xu, M. Yu, G. Cuniberti, S. C. B. Mannsfeld, U. Kaiser, R. Dong, Z. Wang and X. Feng, On-Water Surface Synthesis of Vinylene-Linked Cationic Two-Dimensional Polymer Films as the Anion-Selective Electrode Coating, Angew. Chem., Int. Ed., 2024, 63, e202316299 CrossRef CAS PubMed.
- M. Wang, G. Wang, C. Naisa, Y. Fu, S. M. Gali, S. Paasch, M. Wang, H. Wittkaemper, C. Papp, E. Brunner, S. Zhou, D. Beljonne, H. P. Steinruck, R. Dong and X. Feng, Poly(benzimidazobenzophenanthroline)-Ladder-Type Two-Dimensional Conjugated Covalent Organic Framework for Fast Proton Storage, Angew. Chem., Int. Ed., 2023, 62, e202310937 CrossRef CAS PubMed.
- N. Keller and T. Bein, Optoelectronic processes in covalent organic frameworks, Chem. Soc. Rev., 2021, 50, 1813–1845 RSC.
- X. Liu, H. Li, W. Zhang, Z. Yang, D. Li, M. Liu, K. Jin, L. Wang and G. Yu, Magnetoresistance in Organic Spin Valves Based on Acid-Exfoliated 2D Covalent Organic Frameworks Thin Films, Angew. Chem., Int. Ed., 2023, 62, e202308921 CrossRef CAS PubMed.
- Y. Li, Q. Chen, T. Xu, Z. Xie, J. Liu, X. Yu, S. Ma, T. Qin and L. Chen, De Novo Design and Facile Synthesis of 2D Covalent Organic Frameworks: A Two-in-One Strategy, J. Am. Chem. Soc., 2019, 141, 13822–13828 CrossRef CAS PubMed.
- L. Peng, Q. Guo, C. Song, S. Ghosh, H. Xu, L. Wang, D. Hu, L. Shi, L. Zhao, Q. Li, T. Sakurai, H. Yan, S. Seki, Y. Liu and D. Wei, Ultra-fast single-crystal polymerization of large-sized covalent organic frameworks, Nat. Commun., 2021, 12, 5077 CrossRef CAS PubMed.
- C. Kang, K. Yang, Z. Zhang, A. K. Usadi, D. C. Calabro, L. S. Baugh, Y. Wang, J. Jiang, X. Zou, Z. Huang and D. Zhao, Growing single crystals of two-dimensional covalent organic frameworks enabled by intermediate tracing study, Nat. Commun., 2022, 13, 1370 CrossRef CAS PubMed.
- B. Yu, R. B. Lin, G. Xu, Z. H. Fu, H. Wu, W. Zhou, S. Lu, Q. W. Li, Y. Jin, J. H. Li, Z. Zhang, H. Wang, Z. Yan, X. Liu, K. Wang, B. Chen and J. Jiang, Linkage conversions in single-crystalline covalent organic frameworks, Nat. Chem., 2024, 16, 114–121 CrossRef CAS PubMed.
- J. F. Jing Han, J. Kang, J.-M. Chen, X.-Yu Du, S.-Y. Ding, L. Liang and W. Wang, Fast growth of single-crystal covalent organic frameworks for laboratory X-ray diffraction, Science, 2024, 383, 1014–1019 CrossRef PubMed.
- L. Liang, Y. Qiu, W. D. Wang, J. Han, Y. Luo, W. Yu, G. L. Yin, Z. P. Wang, L. Zhang, J. Ni, J. Niu, J. Sun, T. Ma and W. Wang, Non-Interpenetrated Single-Crystal Covalent Organic Frameworks, Angew. Chem., Int. Ed., 2020, 59, 17991–17995 CrossRef CAS PubMed.
- A. Natraj, W. Ji, J. Xin, I. Castano, D. W. Burke, A. M. Evans, M. J. Strauss, M. Ateia, L. S. Hamachi, N. C. Gianneschi, A. L. Za, J. Sun, K. Yusuf and W. R. Dichtel, Single-Crystalline Imine-Linked Two-Dimensional Covalent Organic Frameworks Separate Benzene and Cyclohexane Efficiently, J. Am. Chem. Soc., 2022, 144, 19813–19824 CrossRef CAS PubMed.
- Y. Yang, B. Liang, J. Kreie, M. Hambsch, Z. Liang, C. Wang, S. Huang, X. Dong, L. Gong, C. Liang, D. Lou, Z. Zhou, J. Lu, Y. Yang, X. Zhuang, H. Qi, U. Kaiser, S. C. B. Mannsfeld, W. Liu, A. Golzhauser and Z. Zheng, Elastic films of single-crystal two-dimensional covalent organic frameworks, Nature, 2024, 630, 878–883 CrossRef CAS PubMed.
- R. Liu, K. T. Tan, Y. Gong, Y. Chen, Z. Li, S. Xie, T. He, Z. Lu, H. Yang and D. Jiang, Covalent organic frameworks: an ideal platform for designing ordered materials and advanced applications, Chem. Soc. Rev., 2021, 50, 120–242 RSC.
- C. G. Gruber, L. Frey, R. Guntermann, D. D. Medina and E. Cortés, Early stages of covalent organic framework formation imaged in operando, Nature, 2024, 630, 872–877 CrossRef CAS PubMed.
- H. Sahabudeen, H. Qi, B. A. Glatz, D. Tranca, R. Dong, Y. Hou, T. Zhang, C. Kuttner, T. Lehnert, G. Seifert, U. Kaiser, A. Fery, Z. Zheng and X. Feng, Wafer-sized multifunctional polyimine-based two-dimensional conjugated polymers with high mechanical stiffness, Nat. Commun., 2016, 7, 13461 CrossRef CAS PubMed.
- F. Ni, Z. Wang and X. Feng, On-Water Surface Synthesis of Two-Dimensional Polymer Membranes for Sustainable Energy Devices, Acc. Chem. Res., 2024, 57, 2414–2427 CrossRef CAS PubMed.
- K. Liu, L. Wang and R. Dong, Two-dimensional conjugated polymer films via liquid-interface-assisted synthesis toward organic electronic devices, J. Mater. Chem. C, 2020, 8, 10696–10718 RSC.
- Z. Zhou, L. Zhang, Y. Yang, I. J. Vitorica-Yrezabal, H. Wang, F. Tan, L. Gong, Y. Li, P. Chen, X. Dong, Z. Liang, J. Yang, C. Wang, Y. Hong, Y. Qiu, A. Golzhauser, X. Chen, H. Qi, S. Yang, W. Liu, J. Sun and Z. Zheng, Growth of single-crystal imine-linked covalent organic frameworks using amphiphilic amino-acid derivatives in water, Nat. Chem., 2023, 15, 841–847 CrossRef CAS PubMed.
- Q. Guo, W. Li, X. Li, J. Zhang, D. Sabaghi, J. Zhang, B. Zhang, D. Li, J. Du, X. Chu, S. Chung, K. Cho, N. N. Nguyen, Z. Liao, Z. Zhang, X. Zhang, G. F. Schneider, T. Heine, M. Yu and X. Feng, Proton-selective coating enables fast-kinetics high-mass-loading cathodes for sustainable zinc batteries, Nat. Commun., 2024, 15, 2139 CrossRef CAS PubMed.
- X. Li, C. Zhang, S. Cai, X. Lei, V. Altoe, F. Hong, J. J. Urban, J. Ciston, E. M. Chan and Y. Liu, Facile transformation of imine covalent organic frameworks into ultrastable crystalline porous aromatic frameworks, Nat. Commun., 2018, 9, 2998 CrossRef PubMed.
- P. J. Waller, Y. S. AlFaraj, C. S. Diercks, N. N. Jarenwattananon and O. M. Yaghi, Conversion of Imine to Oxazole and Thiazole Linkages in Covalent Organic Frameworks, J. Am. Chem. Soc., 2018, 140, 9099–9103 CrossRef CAS PubMed.
- Y. Wang, H. Liu, Q. Pan, C. Wu, W. Hao, J. Xu, R. Chen, J. Liu, Z. Li and Y. Zhao, Construction of Fully Conjugated Covalent Organic Frameworks via Facile Linkage Conversion for Efficient Photoenzymatic Catalysis, J. Am. Chem. Soc., 2020, 142, 5958–5963 CrossRef CAS PubMed.
- K. Wang, Z. Jia, Y. Bai, X. Wang, S. E. Hodgkiss, L. Chen, S. Y. Chong, X. Wang, H. Yang, Y. Xu, F. Feng, J. W. Ward and A. I. Cooper, Synthesis of Stable Thiazole-Linked Covalent Organic Frameworks via a Multicomponent Reaction, J. Am. Chem. Soc., 2020, 142, 11131–11138 CrossRef CAS PubMed.
- P. L. Wang, S. Y. Ding, Z. C. Zhang, Z. P. Wang and W. Wang, Constructing Robust Covalent Organic Frameworks via Multicomponent Reactions, J. Am. Chem. Soc., 2019, 141, 18004–18008 CrossRef CAS PubMed.
- X. R. Ren, B. Bai, Q. Zhang, Q. Hao, Y. Guo, L. J. Wan and D. Wang, Constructing Stable Chromenoquinoline-Based Covalent Organic Frameworks via Intramolecular Povarov Reaction, J. Am. Chem. Soc., 2022, 144, 2488–2494 CrossRef CAS PubMed.
- P. F. Wei, M. Z. Qi, Z. P. Wang, S. Y. Ding, W. Yu, Q. Liu, L. K. Wang, H. Z. Wang, W. K. An and W. Wang, Benzoxazole-Linked Ultrastable Covalent Organic Frameworks for Photocatalysis, J. Am. Chem. Soc., 2018, 140, 4623–4631 CrossRef CAS PubMed.
- H. Pang, D. Huang, Y. Zhu, X. Zhao and Y. Xiang, One-pot cascade construction of nonsubstituted quinoline-bridged covalent organic frameworks, Chem. Sci., 2023, 14, 1543–1550 RSC.
- X. T. Li, J. Zou, T. H. Wang, H. C. Ma, G. J. Chen and Y. B. Dong, Construction of Covalent Organic Frameworks via Three-Component One-Pot Strecker and Povarov Reactions, J. Am. Chem. Soc., 2020, 142, 6521–6526 CrossRef CAS PubMed.
- J. Liu, T. Yang, Z. P. Wang, P. L. Wang, J. Feng, S. Y. Ding and W. Wang, Pyrimidazole-Based Covalent Organic Frameworks: Integrating Functionality and Ultrastability via Isocyanide Chemistry, J. Am. Chem. Soc., 2020, 142, 20956–20961 CrossRef CAS PubMed.
- J. Guo, Y. Xu, S. Jin, L. Chen, T. Kaji, Y. Honsho, M. A. Addicoat, J. Kim, A. Saeki, H. Ihee, S. Seki, S. Irle, M. Hiramoto, J. Gao and D. Jiang, Conjugated organic framework with three-dimensionally ordered stable structure and delocalized pi clouds, Nat. Commun., 2013, 4, 2736 CrossRef PubMed.
- J. Mahmood, E. K. Lee, M. Jung, D. Shin, I. Y. Jeon, S. M. Jung, H. J. Choi, J. M. Seo, S. Y. Bae, S. D. Sohn, N. Park, J. H. Oh, H. J. Shin and J. B. Baek, Nitrogenated holey two-dimensional structures, Nat. Commun., 2015, 6, 6486 CrossRef CAS PubMed.
- M. Wang, M. Ballabio, M. Wang, H.-H. Lin, B. P. Biswal, X. Han, S. Paasch, E. Brunner, P. Liu, M. Chen, M. Bonn, T. Heine, S. Zhou, E. Cánovas, R. Dong and X. Feng, Unveiling Electronic Properties in Metal–Phthalocyanine-Based Pyrazine-Linked Conjugated Two-Dimensional Covalent Organic Frameworks, J. Am. Chem. Soc., 2019, 141, 16810–16816 CrossRef CAS PubMed.
- H. Zhong, M. Wang, M. Ghorbani-Asl, J. Zhang, K. H. Ly, Z. Liao, G. Chen, Y. Wei, B. P. Biswal, E. Zschech, I. M. Weidinger, A. V. Krasheninnikov, R. Dong and X. Feng, Boosting the Electrocatalytic Conversion of Nitrogen to Ammonia on Metal-Phthalocyanine-Based Two-Dimensional Conjugated Covalent Organic Frameworks, J. Am. Chem. Soc., 2021, 143, 19992–20000 CrossRef CAS PubMed.
- M. Wang, M. Wang, H. H. Lin, M. Ballabio, H. Zhong, M. Bonn, S. Zhou, T. Heine, E. Canovas, R. Dong and X. Feng, High-Mobility Semiconducting Two-Dimensional Conjugated Covalent Organic Frameworks with p-Type Doping, J. Am. Chem. Soc., 2020, 142, 21622–21627 CrossRef CAS PubMed.
- A. Riano, K. Strutynski, M. Liu, C. T. Stoppiello, B. Lerma-Berlanga, A. Saeki, C. Marti-Gastaldo, A. N. Khlobystov, G. Valenti, F. Paolucci, M. Melle-Franco and A. Mateo-Alonso, An Expanded 2D Fused Aromatic Network with 90-Ring Hexagons, Angew. Chem., Int. Ed., 2022, 61, e202113657 CrossRef CAS PubMed.
- J. Wang, X. Zhang, Z. Liu, J. Yu, H. G. Wang, X. L. Wu, F. Cui and G. Zhu, Tuning Electron Delocalization of Redox-Active Porous Aromatic Framework for Low-Temperature Aqueous Zn-K Hybrid Batteries with Air Self-Chargeability, Angew. Chem., Int. Ed., 2024, 63, e202401559 CrossRef CAS PubMed.
- E. Jin, M. Asada, Q. Xu, S. Dalapati, M. A. Addicoat, M. A. Brady, H. Xu, T. Nakamura, T. Heine, Q. Chen and D. Jiang, Two-dimensional sp2 carbon–conjugated covalent organic frameworks, Science, 2017, 357, 673–676 CrossRef CAS PubMed.
- R. Chen, J. L. Shi, Y. Ma, G. Lin, X. Lang and C. Wang, Designed Synthesis of a 2D Porphyrin-Based sp(2) Carbon-Conjugated Covalent Organic Framework for Heterogeneous Photocatalysis, Angew. Chem., Int. Ed., 2019, 58, 6430–6434 CrossRef CAS PubMed.
- Y. Wang, W. Hao, H. Liu, R. Chen, Q. Pan, Z. Li and Y. Zhao, Facile construction of fully sp(2)-carbon conjugated two-dimensional covalent organic frameworks containing benzobisthiazole units, Nat. Commun., 2022, 13, 100 CrossRef CAS PubMed.
- A. Acharjya, P. Pachfule, J. Roeser, F. J. Schmitt and A. Thomas, Vinylene-Linked Covalent Organic Frameworks by Base-Catalyzed Aldol Condensation, Angew. Chem., Int. Ed., 2019, 58, 14865–14870 CrossRef CAS PubMed.
- Z. Wang, Y. Yang, Z. Zhao, P. Zhang, Y. Zhang, J. Liu, S. Ma, P. Cheng, Y. Chen and Z. Zhang, Green synthesis of olefin-linked covalent organic frameworks for hydrogen fuel cell applications, Nat. Commun., 2021, 12, 1982 CrossRef CAS PubMed.
- H. Lyu, C. S. Diercks, C. Zhu and O. M. Yaghi, Porous Crystalline Olefin-Linked Covalent Organic Frameworks, J. Am. Chem. Soc., 2019, 141, 6848–6852 CrossRef CAS PubMed.
- A. Acharjya, P. Pachfule, J. Roeser, F.-J. Schmitt and A. Thomas, Vinylene-Linked Covalent Organic Frameworks by Base-Catalyzed Aldol Condensation, Angew. Chem., Int. Ed., 2019, 58, 14865–14870 CrossRef CAS PubMed.
- T. Jadhav, Y. Fang, W. Patterson, C. H. Liu, E. Hamzehpoor and D. F. Perepichka, 2D Poly(arylene vinylene) Covalent Organic Frameworks via Aldol Condensation of Trimethyltriazine, Angew. Chem., Int. Ed., 2019, 58, 13753–13757 CrossRef CAS PubMed.
- D. L. Pastoetter, S. Xu, M. Borrelli, M. Addicoat, B. P. Biswal, S. Paasch, A. Dianat, H. Thomas, R. Berger, S. Reineke, E. Brunner, G. Cuniberti, M. Richter and X. Feng, Synthesis of Vinylene-Linked Two-Dimensional Conjugated Polymers via the Horner–Wadsworth–Emmons Reaction, Angew. Chem., Int. Ed., 2020, 59, 23620–23625 CrossRef CAS PubMed.
- Y. Liu, S. Fu, D. L. Pastoetter, A. H. Khan, Y. Zhang, A. Dianat, S. Xu, Z. Liao, M. Richter, M. Yu, M. Polozij, E. Brunner, G. Cuniberti, T. Heine, M. Bonn, H. I. Wang and X. Feng, Vinylene-Linked 2D Conjugated Covalent Organic Frameworks by Wittig Reactions, Angew. Chem., Int. Ed., 2022, 61, e202209762 CrossRef CAS PubMed.
- D. Becker, B. P. Biswal, P. Kalenczuk, N. Chandrasekhar, L. Giebeler, M. Addicoat, S. Paasch, E. Brunner, K. Leo, A. Dianat, G. Cuniberti, R. Berger and X. Feng, Fully sp(2)-Carbon-Linked Crystalline Two-Dimensional Conjugated Polymers: Insight into 2D Poly(phenylenecyanovinylene) Formation and its Optoelectronic Properties, Chem. – Eur. J., 2019, 25, 6562–6568 CrossRef CAS PubMed.
- Y. Liu, H. Zhang, H. Yu, Z. Liao, S. Paasch, S. Xu, R. Zhao, E. Brunner, M. Bonn, H. I. Wang, T. Heine, M. Wang, Y. Mai and X. Feng, A Thiophene Backbone Enables Two-Dimensional Poly(arylene vinylene)s with High Charge Carrier Mobility, Angew. Chem., Int. Ed., 2023, 62, e202305978 CrossRef CAS PubMed.
- H. Yan, Z. Kou, S. Li and T. Zhang, Synthesis of sp(2) Carbon-Conjugated Covalent Organic Framework Thin-Films via Copper-Surface-Mediated Knoevenagel Polycondensation, Small, 2023, 19, e2207972 CrossRef PubMed.
- H. Wei, J. Ning, X. Cao, X. Li and L. Hao, Benzotrithiophene-Based Covalent Organic Frameworks: Construction and Structure Transformation under Ionothermal Condition, J. Am. Chem. Soc., 2018, 140, 11618–11622 CrossRef CAS PubMed.
- S. Xu, G. Wang, B. P. Biswal, M. Addicoat, S. Paasch, W. Sheng, X. Zhuang, E. Brunner, T. Heine, R. Berger and X. Feng, A Nitrogen-Rich 2D sp(2)-Carbon-Linked Conjugated Polymer Framework as a High-Performance Cathode for Lithium-Ion Batteries, Angew. Chem., Int. Ed., 2019, 58, 849–853 CrossRef CAS PubMed.
- L. Ascherl, E. W. Evans, J. Gorman, S. Orsborne, D. Bessinger, T. Bein, R. H. Friend and F. Auras, Perylene-Based Covalent Organic Frameworks for Acid Vapor Sensing, J. Am. Chem. Soc., 2019, 141, 15693–15699 CrossRef CAS PubMed.
- L. Dai, A. Dong, X. Meng, H. Liu, Y. Li, P. Li and B. Wang, Enhancement of Visible-Light-Driven Hydrogen Evolution Activity of 2D pi-Conjugated Bipyridine-Based Covalent Organic Frameworks via Post-Protonation, Angew. Chem., Int. Ed., 2023, 62, e202300224 CrossRef CAS PubMed.
- S. Xu, Z. Liao, A. Dianat, S. W. Park, M. A. Addicoat, Y. Fu, D. L. Pastoetter, F. G. Fabozzi, Y. Liu, G. Cuniberti, M. Richter, S. Hecht and X. Feng, Combination of Knoevenagel Polycondensation and Water-Assisted Dynamic Michael-Addition-Elimination for the Synthesis of Vinylene-Linked 2D Covalent Organic Frameworks, Angew. Chem., Int. Ed., 2022, 61, e202202492 CrossRef CAS PubMed.
- E. Jin, K. Geng, K. H. Lee, W. Jiang, J. Li, Q. Jiang, S. Irle and D. Jiang, Topology-Templated Synthesis of Crystalline Porous Covalent Organic Frameworks, Angew. Chem., Int. Ed., 2020, 59, 12162–12169 CrossRef CAS PubMed.
- F. G. Fabozzi, N. Severin, J. P. Rabe and S. Hecht, Room Temperature On-Surface Synthesis of a Vinylene-Linked Single Layer Covalent Organic Framework, J. Am. Chem. Soc., 2023, 145, 18205–18209 CrossRef CAS PubMed.
- Y. Su, Y. Wan, H. Xu, K. I. Otake, X. Tang, L. Huang, S. Kitagawa and C. Gu, Crystalline and Stable Benzofuran-Linked Covalent Organic Frameworks from Irreversible Cascade Reactions, J. Am. Chem. Soc., 2020, 142, 13316–13321 CrossRef CAS PubMed.
- Y. Su, B. Li, H. Xu, C. Lu, S. Wang, B. Chen, Z. Wang, W. Wang, K. I. Otake, S. Kitagawa, L. Huang and C. Gu, Multi-Component Synthesis of a Buta-1,3-diene-Linked Covalent Organic Framework, J. Am. Chem. Soc., 2022, 144, 18218–18222 CrossRef CAS PubMed.
- S. Li, R. Ma, S. Xu, T. Zheng, G. Fu, Y. Wu, Z. Liao, Y. Kuang, Y. Hou, D. Wang, P. S. Petkov, K. Simeonova, X. Feng, L. Z. Wu, X. B. Li and T. Zhang, Direct Construction of Isomeric Benzobisoxazole-Vinylene-Linked Covalent Organic Frameworks with Distinct Photocatalytic Properties, J. Am. Chem. Soc., 2022, 144, 13953–13960 CrossRef CAS PubMed.
- S. Li, R. Ma, S. Xu, T. Zheng, H. Wang, G. Fu, H. Yang, Y. Hou, Z. Liao, B. Wu, X. Feng, L.-Z. Wu, X.-B. Li and T. Zhang, Two-Dimensional Benzobisthiazole-Vinylene-Linked Covalent Organic Frameworks Outperform One-Dimensional Counterparts in Photocatalysis, ACS Catal., 2023, 13, 1089–1096 CrossRef CAS.
- S. Li, L. Li, Y. Li, L. Dai, C. Liu, Y. Liu, J. Li, J. Lv, P. Li and B. Wang, Fully Conjugated Donor–Acceptor Covalent Organic Frameworks for Photocatalytic Oxidative Amine Coupling and Thioamide Cyclization, ACS Catal., 2020, 10, 8717–8726 CrossRef CAS.
- S. Wei, F. Zhang, W. Zhang, P. Qiang, K. Yu, X. Fu, D. Wu, S. Bi and F. Zhang, Semiconducting 2D Triazine-Cored Covalent Organic Frameworks with Unsubstituted Olefin Linkages, J. Am. Chem. Soc., 2019, 141, 14272–14279 CrossRef CAS PubMed.
- J. Xu, Y. He, S. Bi, M. Wang, P. Yang, D. Wu, J. Wang and F. Zhang, An Olefin-Linked Covalent Organic Framework as a Flexible Thin-Film Electrode for a High-Performance Micro-Supercapacitor, Angew. Chem., Int. Ed., 2019, 58, 12065–12069 CrossRef CAS PubMed.
- F. Meng, S. Bi, Z. Sun, B. Jiang, D. Wu, J. S. Chen and F. Zhang, Synthesis of Ionic Vinylene-Linked Covalent Organic Frameworks through Quaternization-Activated Knoevenagel Condensation, Angew. Chem., Int. Ed., 2021, 60, 13614–13620 CrossRef CAS PubMed.
- F. Meng, S. Bi, Z. Sun, D. Wu and F. Zhang, 2,4,6-Trimethylpyridine-Derived Vinylene-Linked Covalent Organic Frameworks for Confined Catalytic Esterification, Angew. Chem., Int. Ed., 2022, 61, e202210447 CrossRef CAS PubMed.
- S. Bi, C. Yang, W. Zhang, J. Xu, L. Liu, D. Wu, X. Wang, Y. Han, Q. Liang and F. Zhang, Two-dimensional semiconducting covalent organic frameworks via condensation at arylmethyl carbon atoms, Nat. Commun., 2019, 10, 2467 CrossRef PubMed.
- S. Bi, Z. A. Lan, S. Paasch, W. Zhang, Y. He, C. Zhang, F. Liu, D. Wu, X. Zhuang, E. Brunner, X. Wang and F. Zhang, Substantial Cyano–Substituted Fully sp2-Carbon–Linked Framework: Metal–Free Approach and Visible–Light–Driven Hydrogen Evolution, Adv. Funct. Mater., 2017, 27, 1703146 CrossRef.
- N. Yang, W. Yan, Z. J. Zhou, C. Tian, P. Zhang, H. Liu, X. P. Wu, C. Xia, S. Dai and X. Zhu, Synthetic Leaves Based on Crystalline Olefin-Linked Covalent Organic Frameworks for Efficient CO2 Photoreduction with Water, Nano Lett., 2024, 24, 5444–5452 CrossRef CAS PubMed.
- X. Xu, S. Zhang, K. Xu, H. Chen, X. Fan and N. Huang, Janus Dione-Based Conjugated Covalent Organic Frameworks with High Conductivity as Superior Cathode Materials, J. Am. Chem. Soc., 2023, 145, 1022–1030 CrossRef CAS PubMed.
- K. Wang, H. Yang, Z. Liao, S. Li, M. Hambsch, G. Fu, S. C. B. Mannsfeld, Q. Sun and T. Zhang, Monolayer-Assisted Surface-Initiated Schiff-Base-Mediated Aldol Polycondensation for the Synthesis of Crystalline sp(2) Carbon-Conjugated Covalent Organic Framework Thin Films, J. Am. Chem. Soc., 2023, 145, 5203–5210 CrossRef CAS PubMed.
- D. L. Pastoetter, S. Xu, M. Borrelli, M. Addicoat, B. P. Biswal, S. Paasch, A. Dianat, H. Thomas, R. Berger, S. Reineke, E. Brunner, G. Cuniberti, M. Richter and X. Feng, Synthesis of Vinylene-Linked Two-Dimensional Conjugated Polymers via the Horner-Wadsworth-Emmons Reaction, Angew. Chem., Int. Ed., 2020, 59, 23620–23625 CrossRef CAS PubMed.
- T. Niu, C. Hua and M. Zhou, On-Surface Synthesis toward Two-Dimensional Polymers, J. Phys. Chem. Lett., 2022, 13, 8062–8077 CrossRef CAS PubMed.
- L. Grill, M. Dyer, L. Lafferentz, M. Persson, M. V. Peters and S. Hecht, Nano-architectures by covalent assembly of molecular building blocks, Nat. Nanotechnol., 2007, 2, 687–691 CrossRef CAS PubMed.
- G. Galeotti, F. De Marchi, E. Hamzehpoor, O. MacLean, M. Rajeswara Rao, Y. Chen, L. V. Besteiro, D. Dettmann, L. Ferrari, F. Frezza, P. M. Sheverdyaeva, R. Liu, A. K. Kundu, P. Moras, M. Ebrahimi, M. C. Gallagher, F. Rosei, D. F. Perepichka and G. Contini, Synthesis of mesoscale ordered two-dimensional pi-conjugated polymers with semiconducting properties, Nat. Mater., 2020, 19, 874–880 CrossRef CAS PubMed.
- W. Liu, X. Luo, Y. Bao, Y. P. Liu, G. H. Ning, I. Abdelwahab, L. Li, C. T. Nai, Z. G. Hu, D. Zhao, B. Liu, S. Y. Quek and K. P. Loh, A two-dimensional conjugated aromatic polymer via C-C coupling reaction, Nat. Chem., 2017, 9, 563–570 CrossRef CAS PubMed.
- J. Liu, P. Ruffieux, X. Feng, K. Müllen and R. Fasel, Cyclotrimerization of arylalkynes on Au(111), Chem. Commun., 2014, 50, 11200–11203 RSC.
- Q. Li, B. Yang, H. Lin, N. Aghdassi, K. Miao, J. Zhang, H. Zhang, Y. Li, S. Duhm, J. Fan and L. Chi, Surface-Controlled Mono/Diselective ortho C-H Bond Activation, J. Am. Chem. Soc., 2016, 138, 2809–2814 CrossRef CAS PubMed.
- H. J. Noh, Y. K. Im, S. Y. Yu, J. M. Seo, J. Mahmood, T. Yildirim and J. B. Baek, Vertical two-dimensional layered fused aromatic ladder structure, Nat. Commun., 2020, 11, 2021 CrossRef CAS PubMed.
|
This journal is © the Partner Organisations 2025 |
Click here to see how this site uses Cookies. View our privacy policy here.