DOI:
10.1039/D2EN00605G
(Critical Review)
Environ. Sci.: Nano, 2023,
10, 41-61
Nanobiopesticides in sustainable agriculture: developments, challenges, and perspectives
Received
23rd June 2022
, Accepted 13th November 2022
First published on 14th November 2022
Abstract
Environment-friendly nanomaterials with biodegradable, responsive, and biocompatible properties have attracted considerable interest for exploring safe and efficient pesticides, and applying nanotechnology to develop new nanopesticide formulations would avoid the hazards of chemical pesticides and improve the efficacy of biopesticides. It can also be conducive to the development of less toxic biopesticides with increased stability of active ingredients, favorable biological safety, and enhanced activity on target pests. At present, there are comprehensive research papers, reviews, and books available on nanopesticides, but there are few reviews on nanobiopesticides. This review summarizes the main applications of nanotechnology in biopesticide formulation (such as nanoinsecticides, nanofungicides, nanobactericides, nanobioherbicides, and RNA pesticides), explores the possible uptake and transport mechanisms of nanobiopesticides in plants, analyzes its possible fate and impact on environment, and describes the challenges and possible risk of nanobiopesticides. Overall, this review provides a promising and comprehensive environmental perspective on the latest nanotechnology in the biopesticides field.
Environmental significance
Nanotechnology offers great opportunity to achieve more rational use of pesticides, and nanopesticides are considered to be one of the most important chemical pesticides innovations, which would largely enhance the bioactivity, achieve controlled release, increase the solubility, and prolong the duration. But the environmental behaviors of these novel agrochemicals are not fully characterized, and the lack of information of their effects on human and animal health have limited their rapid development. This review highlights the applications of nanotechnology in the agricultural fields and the application field of nanomaterials on biopesticides, summarizes the main progress of nanobiopesticides, and discusses the challenges of nanopesticides and restrictions in an actual environment. Further, this review is expected to provide a more comprehensive understanding of nanobiopesticides and promote its development, which is novel and interesting for readers in the environmental field.
|
1. Introduction
Nanotechnology, as “the next industrial revolution”, has been widely explored to improve agriculture. It is a promising and fascinating technology, which opens a new door for the next revolution in agriculture and agri-technology fields. Nanotechnology has shown promise for more sustainable and efficient agricultural systems to promote food security.1,2 In recent times, more intensive research and practices of nanotechnology in agriculture have been developed, where the applications can be categorized into nanopesticides, agricultural diagnostics, nanofertilizers, nanobiosensors, pollution management, sustainable agriculture, etc., (illustrated in Fig. 1a). IUPAC selected the “Ten Chemical Innovations That Will Change Our World” in 2019, and the nanopesticide ranks first.3 Applying nanotechnology to develop new nanopesticide formulations plays an important and effective role in plant pests and disease control to avoid the hazards of chemical pesticides and improve the efficacy of biopesticides.
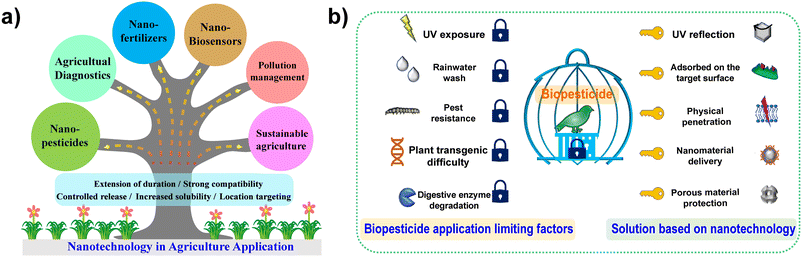 |
| Fig. 1 a) Applications of nanotechnology in agricultural sciences; it could achieve the controlled release of the active ingredient, increase the solubility, and exhibit the extension of duration, location targeting, and strong compatibility. b) The application limiting factors of biopesticides and nanobiopesticides based on nanotechnology. | |
Pesticides are chemicals that are applied to control pests and prevent plant diseases. They can be divided into insecticides, fungicides, bactericides, herbicides, and rodenticides according to their structure,4,5 but it is toxic to both humans and pests. Biopesticide formulations, which are ecofriendly pesticides, are alternatives to agrochemicals. Biopesticides can be obtained from different organisms, such as plants, bacteria, fungi, and nematodes.6,7
Biopesticides exhibit less toxicity, quick decomposition, low exposition characteristics, and can overcome the adverse effects (such as persistence, bioaccumulation, and toxicity) of chemical pesticides in agricultural activity. However, the low efficacy, short duration, inconsistent field performance, and high cost have generally limited the development of biopesticides, and biopesticides still only account for a small percentage of the global crop-protection market (around 5% market share with a value of about US$ 3 billion worldwide).8,9 The poor performance of pesticides/biopesticides has compelled the scientific community to discover new and cost-effective alternative strategies.10,11 Therefore, the introduction of nanomaterials in pesticides can effectively avoid the negative aspects of traditional agrochemicals or biologically-originated pesticides.10 Nanotechnology is conducive to the development of less toxic biopesticides with increased stability of the active ingredients, favorable biological safety, and enhanced activity on target pests (as illustrated in Fig. 1b).12–15
Currently, there are relatively comprehensive research papers, reviews, and books available on nanopesticides (194 results containing the word nanopesticide on WOS for the period from 1900 to October 2022), but there are few reviews on nanobiopesticides (with hardly 7 results containing the word nanobiopesticide in WOS). The present review summarizes the types and research progress of nanobiopesticides (mainly focusing on the application of nanotechnology in insecticides, fungicides, bactericides, herbicides, and novel RNA pesticides), explores the possible uptake and transport mechanisms of nanobiopesticides in plants, evaluates their possible impacts on environmental fate, discusses their future perspectives and challenges, and provides an in-depth understanding of the interactions among NPs, the environment (microorganisms, soils, plants), and humans that are still needed to develop a practical and effective strategy in nanobiopesticide applications.
2. The main categories of nanobiopesticides and their research progress
2.1 The common nanocarriers in biopesticides
Nanoparticles (NPs), which possess a small size and high specific surface area, show unusual and fascinating physicochemical properties in comparison to their bulk materials, such as specific chemical properties, physical strength, optical–electrical properties, thermal properties, magnetism, and biological activity.10,16,17 These novel properties of NPs impart enhanced beneficial characteristics and allow them to be converted from insoluble, poorly absorbed, and unstable biologically active substances into convenient deliverable substances.18,19 The main categories of nanomaterials are pure carbon nanostructures, inorganic nanomaterials, organic nanomaterials, and organic–inorganic hybrids according to their composition,20 and the most widely used NPs in agriculture are silver, copper, titanium, zinc, silica, gold, aluminum, iron, chitin NPs, chitosan, nanoclay, graphene NPs, multiwalled carbon nanotubes, and biopolymers.21–28
Nanobiopesticides are associated with optimistic innovative technologies of producing and utilizing biopesticides that fall in the nanoscale (1–100 nm).29 However, the nanocarriers used for medical, cosmetic, food, or agricultural applications generally have outer diameters much larger than 100 nm, although the internal functional features may be smaller than 100 nm.30 Therefore, nanopesticides may or may not fall within the definition of nanomaterials. Nanopesticides are used to describe any pesticide formulation that (i) intentionally include entities in the nm size range (up to 1000 nm), (ii) are designated with a “nano” prefix (e.g., nanohybrid, nanocomposite), and/or (iii) are claimed to exhibit novel properties associated with the small size of their components.30,31 Nanopesticide delivery has been classified into nanospheres, nanocapsules, nanocontainers, nanoemulsions, nanogels, liposomes, inorganic nanocarriers, etc. according to their forms,29 where the nanocapsules, nanogels, and micelles are the most popular shapes of NPs used in controlled-release biopesticide delivery.32 In general, the polymer- and clay-based nanomaterials are considered as biocompatible, cost-effective, and stimulus-responsive nanocarriers to encapsulate the active ingredients, and the active ingredients are mainly conventional pesticides, such as avermectin, atrazine, and glyphosate.2 Chitosan, cellulose, and polylactide are the most common natural polymers for developing nanocapsules, nanospheres, nano(hydro)gels, and nanomicelles for the active ingredients. Besides, mesoporous silica and montmorillonite are typical clay-based nanomaterials with high active ingredient encapsulation capacity, and some nanocomposites and two-dimensional (2D) nanomaterials with high specific surface areas have also been reported to facilitate the loading of active ingredients.2 Some of the reported NPs in biopesticide application are represented in Table 1 and Fig. 2. Overall, the advantages of nanopesticides are increased stability and bioavailability, enhanced adhesion to surfaces, controlled release of active ingredients, high targeting properties, and enhanced environmental safety.33–37
Table 1 Different types of NPs and their application in biopesticides
Nanoparticle |
Size (nm) |
Biopesticides |
Bioactivity effect against |
Ref. |
Nano-Mg(OH)2 |
50–100 |
Bacillus thuringiensis
|
Culex quinquefasciatus
|
57
|
Mg(OH)2 cross-nanosheets |
712 |
Bacillus thuringiensis
|
Helicoverpa armigera
|
59
|
Mesoporous silicon |
5000/400 |
Bacillus thuringiensis
|
Caenorhabditis elegans/Ancylostoma ceylanicum |
60
|
Silica NPs |
90.2 |
Bacillus thuringiensis
|
Caenorhabditis elegans
|
64
|
Silver NPs |
100–300 |
Bacillus thuringiensis
|
Aedes aegypti
|
65
|
Mesoporous silica NPs |
40 |
Bacillus thuringiensis
|
Tuta absoluta
|
61
|
TiO2 |
33–44 |
Bacillus thuringiensis
|
Ephestia kuehniella Zeller
|
66
|
Zinc oxide NPs |
20 |
Bacillus thuringiensis
|
Callosobruchus maculatus
|
67
|
Fe3O4 NPs |
168 |
Bacillus thuringiensis
|
Helicoverpa armigera
|
68
|
Amino acid-functionalized fluorescent nanocarriers |
49 |
Bacillus thuringiensis
|
Agrotis ypsilon
|
69
|
Graphene oxide |
0.85 |
Bacillus thuringiensis
|
Ephestia kuehniella Zeller
|
70
|
Lignin nanospheres |
166–210 |
Avermectin |
— |
71
|
Polylactic acid |
245.7 |
Avermectin |
Grapholitha molesta Busck |
72
|
Poly-γ-glutamic acid and chitosan |
56–61 |
Avermectin |
Pine wood nematodes |
38
|
Carboxymethyl cellulose and rosin |
167 |
Avermectin |
Plutella xylostella
|
56
|
MSNs-ss-starch nanoparticles |
80.3 |
Avermectin |
Plutella xylostella larvae |
62
|
Star polyamine (SPc) |
108.1 |
Avermectin |
Green peach aphids |
73
|
Layered double hydroxides (LDHs) |
60–130 |
Avermectin |
— |
48
|
Poly(glycidyl methacrylate-co-acrylic acid) grafted hollow mesoporous silica |
190.1 |
Abamectin |
Cnaphalocrocis medinalis larvae |
63
|
Plant virus nanoparticle (PVN) |
37.6 |
Abamectin |
Meloidogyne hapla root knot nematodes |
55
|
Nanochitin |
102–119.2 |
Abamectin |
Helicoverpa armigera
|
74
|
Mythimna separata
|
Zein |
198 |
Neem oil |
Acanthoscelides obtectus, Bemisia tabaci, and Tetranychus urtica |
75
|
Star polymer (SPc) |
17.4–144.6 |
Eugenol |
Potato late blight |
76
|
Silver nanoparticles |
9.54–49.0 |
H. coronarium rhizomes extract |
Aedes aegypti
|
77
|
Carboxymethyl chitosan with ricinoleic acid (R–CM-chitosan) |
200–500 |
Azadirachtin (Aza) |
— |
46
|
Starch nanoparticles (SNPs) |
93–113 |
Essential oils (EOs): menthone |
Escherichia coli
|
78
|
Staphylococcus aureus
|
Cashew gum (CG) |
27.7–432.7 |
Eucalyptus staigeriana essential oil (ESO) |
Listeria monocytogenes
|
79
|
Salmonella enteritidis
|
Agar or alginate or carrageenan |
359–634 |
Zataria essential oil |
— |
80
|
Zein with sodium caseinate (SC)–chitosan hydrochloride (CHC) double layers |
176.9–741.6 |
Thymol |
Staphylococcus aureus
|
39
|
Chitosan |
82–165 |
Achillea millefolium essential oil (AEO) |
Tetranychus urticae
|
40
|
Poly-γ-glutamic acid (γ-PGA) and chitosan (CS) |
56–61 |
Avermectin |
Caenorhabditis elegans
|
81
|
Chitosan derivate (CMC-g-RA) |
215.2–960.1 |
Botanical insecticide capsaicin (Cap) |
— |
41
|
Chitosan nanoparticles |
10–28 |
Metarhizium anisopliae
|
Plutella xylostella
|
42
|
Spodoptera litura
|
Chitosan nanoparticles |
20–60 |
Green tea oil |
Staphylococcus aureus
|
43
|
Escherichia coli
|
Chitosan |
100–200 |
Chitin synthase2 gene |
Anopheles gambiae
|
44
|
dsRNA |
Chitosan crosslinked to sodium tripolyphosphate |
<200 |
dsRNA |
Aedes aegypti
|
45
|
Star polycation (SPc) |
146.1 |
Hemocytin (hem) dsRNA and botanical pesticide atrine |
Green peach aphids |
82
|
Branched amphiphilic peptide capsules (BAPCs) |
70–300 |
dsRNA |
Tribolium castaneum
|
83
|
Functionalized perylene diimides nanocarriers |
<200 |
dsHem |
Aphis glycines
|
84
|
Layered double hydroxide (LDH) |
15–120 |
dsRNA |
Pepper mild mottle virus (PMMoV) and cucumber mosaic virus (CMV) |
85
|
Nanoliposome |
110 |
Bacterial crude extract |
Fusarium oxysporum
|
86
|
TiO2 and ZnO |
50 and 67 |
Bio-agent Bacillus subtilus |
Podosphaera xanthii
|
87
|
ZnO synthesized by aqueous plant leaf extract of Terminalia bellerica |
20–30 |
— |
Alternaria brassicae
|
88
|
Herbicidal metabolites extracted from Fusarium oxysporum fungi |
60–90 |
— |
Ninidam theenjan
|
89
|
Nanoemulsion containing palm oil derivatives and Parthenium hysterophorus crude extract |
140.1 |
— |
Diodia ocimifolia
|
90
|
Mesoporous silica nanoparticles |
80–180 |
Citridiol |
— |
91
|
Cellulose nanocrystals and silicon dioxide NPs |
82.5 |
Emamectin benzoate |
Phenacoccus solenopsis
|
92
|
Porous hollow silica |
80.0 |
Validamycin |
— |
93
|
Nanosized calcium carbonate |
50–200 |
Validamycin |
Rhizoctonia solani
|
94
|
Amino-modified silica |
52.5–315.4 |
Kasugamycin |
Escherichia coli
|
95
|
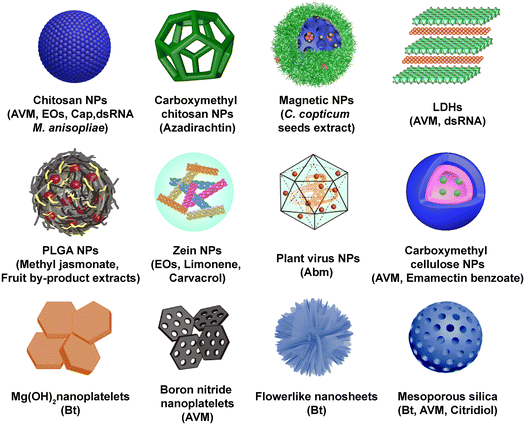 |
| Fig. 2 Common nanocarrier types in biopesticide application, including chitosan NPs,38–45 carboxymethyl chitosan NPs,46 magnetic NPs,47 layered double hydroxides (LDHs),48 poly(lactic-co-glycolic acid) (PLGA) NPs,49,50 zein nanoparticles,51–54 plant virus NPs,55 carboxymethyl cellulose NPs,56 Mg(OH)2 nanoplatelets,57 boron nitride nanoplatelets,58 flowerlike nanosheets,59 and mesoporous silica.60–63 | |
2.2 The application of nanotechnology to insecticides
Bacillus thuringiensis-based nanoinsecticides.
Bacillus thuringiensis (Bt) is a microbial pesticide with a wide range of applications that has insecticidal activity against lepidopteran and coleopteran pests and has no toxicity to non-target organisms. However, Bt is easily affected by environmental factors, such as ultraviolet (UV) light, rainwater, and digestive enzymes, which lead to a decrease in its insecticidal duration and cannot meet biological pest control work. The application of nanotechnology in the preparation of Bt biopesticides can provide more efficient, stable, and environment-friendly Bt formulations.
Nanomaterials can improve the reflection and scattering of Bt preparations with respect to UV radiation and improve the environmental stability of Bt-active ingredients. For example, nano-Mg(OH)2 can efficiently adsorb the Bt mosquito-killing protein Cry11Aa and improve the UV stability of the protein, while improving the enzymatic hydrolysis of the protein in the midgut of the target pest process, promoting the destruction of midgut cells, and ultimately increasing the insecticidal activity of the protein on the pests.57,96 Bt chitinase was immobilized onto the surface-modified spherical nanosilica to prepare a silica–chitinase complex by electrostatic adsorption and covalent binding, and the obtained product exhibits high resistance to UV radiation.64 Bt mixed with different crystal forms of nano-TiO2 shows different anti-UV activity,66 which indicates that the UV resistance properties may be closely related to its morphology and crystal forms. Moreover, graphene oxide combined with olive oil on the Bt cell mixture showed synergistic UV protection effects,97 and UV reflective nanomaterials, nano-ZnO, nano-SiO2, and nano-TiO2, combined with Bt to form a microcapsule formulation with relatively long-lasting UV resistance.98
Rao et al. synthesized nano-Mg(OH)2 to absorb Cry1Ac protein and control the loss of Cry1Ac protein that can be induced by rainwater flushing, where the nano-Mg(OH)2–Cry1Ac mixture can be loaded on the groove of the cotton leaf, which could enhance the anti-rainwater washing ability and insecticidal activity compared to those of the pure protein.59 Wu et al. combined mesoporous silica with Bt Cry5B protein and the mesoporous silica loading not only protected the Cry5B protein from hydrolysis by mammalian pepsin but also improved the protein transmission in the worm and increased the control efficiency of the protein on the nematode.60 In addition, studies have shown that GM plants can secrete Bt Cry protein in their roots and other tissues, and the secreted protein can bind to nanoparticles in soil to form complexes with long-term activity. For instance, nano-SiO2 and Cry1Ab protein can be combined by electrostatic adsorption, where the electrostatic adsorption is based on the non-uniformity of the surface charge distribution of the Cry1Ab protein, while the conformation and insecticidal activity of Cry1Ab protein did not significantly change during the adsorption process.99,100 Montmorillonite and kaolin can prevent Cry1Aa protein from oligoaggregation, maintain protein conformational stability, and exhibit higher insecticidal activity.101,102 Therefore, the tight binding of soil nanoparticles is the key to the transgenic protein possessing a persistent insecticidal effect in the soil environment.
Moreover, nanomaterials can be used as carriers to prepare GM crops due to their efficient gene-delivering properties. Modified magnetic nano-Fe3O4 particles were used to adsorb Bt cry1Ac genes, and the prepared gene delivery tool could provide a fast and efficient solution for the preparation of GM cotton.68 Nanomesoporous silica was used as the cry1Ab gene carrier and was introduced into the tomato via injection, and the related gene could be highly expressed in the progeny tomato seeds.103 Meanwhile, the cry1Ab gene carried by mesoporous silica can also be expressed in tomato progeny by injecting the plant leaves.61 The resistance of Lepidoptera and other pests to Bt Cry protein is also an important concern. Amino acid-functionalized fluorescent nanocarriers can improve the penetration ability of the Bt Cry1Ab protein into the midgut cells of Agrotis ypsilon, which is independent of midgut receptors; thus, it can bypass the changes caused by the receptor changes.69 This strategy can improve the control effect of Bt-resistant pests, reduce the frequency of use of alternative chemical pesticides, and has a good promoting effect on the sustainable development of agriculture and environmental protection. In addition, the green synthesis of NPs based on Bt cell culture is an alternative to chemical and physical methods, where it not only reduces the content of toxic chemical raw materials required in the preparation process but also increases the insecticidal activity of the obtained products. The prepared nanosilver using the supernatant of the Bt culture has a synergistic effect on the control of Aedes aegypti.65 Nano-ZnO was synthesized by a Bt cell culture medium as the matrix for the control of storage pests, and the results showed that the insecticidal activity of the complex was significantly higher than that of the pure nano-ZnO and Bt preparations.67,104
Nanotechnology in avermectin insecticides.
Avermectin (AVM) plays a very important function as a bactericidal and an antiparasitic agent against nematodes and arthropods, and has been widely used for decades. However, the low solubility, high sensitivity, easy decomposition, and short efficacy duration of AVM limit its application. It has been proved that nanosilica could be a super nanocarrier to load and control the release of AVM. Liang et al. established a novel redox-α-amylase double-stimulus-responsive pesticide release system (AVM@MSNs-starch NPs, Fig. 3a), and the constructed compound could protect AVM from photodegradation and achieve the controlled release of the active ingredient with longer effectiveness.62 An efficient AVM nanodelivery system based on a star polyamine (SPc) was constructed by Yang et al., which could enhance the contact and stomach toxicity of AVM against green peach aphids, which reveals the wide applications in the agricultural field.73 Gao et al. designed a pH-responsive organic–inorganic hybrid nanomaterial by modifying HMSNs with high adhesion and wettability on rice leaves,63 which could significantly improve the photostability of AVM and exhibit the high release rate to achieve the high activity of Lepidoptera pests.
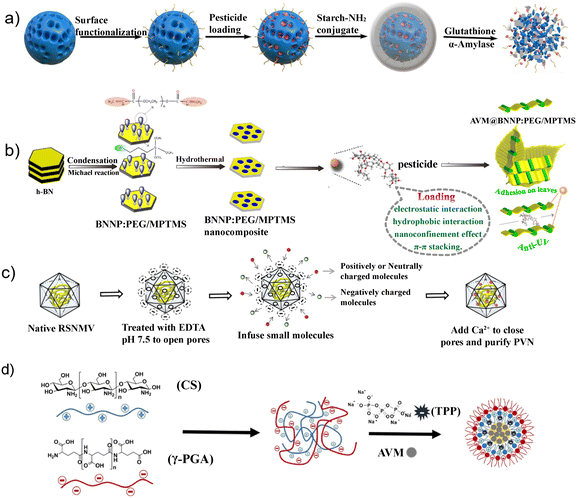 |
| Fig. 3 a) Mesoporous organosilica nanovehicles used as nanocarriers of avermectin, adapted with permission from ref. 62, copyright 2020, Elsevier B.V. b) Boron nitride nanoplatelets for pH-responsive release and enhanced UV stability of avermectin, adapted with permission from ref. 58, copyright 2020, Elsevier B.V. c) Avermectin-loaded plant virus NPs, adapted with permission from ref. 55, copyright 2015, American Chemical Society. d) Preparation of AVM–chitosan/γ-PGA nanoparticles. Adapted with permission from ref. 110, copyright 2018, Elsevier B.V. | |
Moreover, scientists have prepared boron nitride interface-modified nanocarriers with 18.19% loading of AVM (Fig. 3b), where the presence of a PEG modification layer improves the dispersion of drug-carrying NPs in water and imparts an AVM pH-sensitive release signature, which could improve the AVM's adhesion performance and photostability on cucumber leaves.58 Zhao et al. used sodium dodecyl sulfate to modify AVM-layered double hydroxide nanohybrids, which achieved the well-controlled release of AVM, and it is expected to become a candidate material for water-dispersible controlled-release formulations.48 Song et al. constructed a pH-responsive release AVM nanopesticide based on 2D MXene (Ti3C2) nanomaterials with a maximum pesticide load of 81.44%, where the nanopesticide delivers high water solubility and good photostability, which could reduce pesticide dosage and spraying time.105
Lignin is a renewable resource of aromatic compounds in nature and is a widely found biopolymers around the world.106 The hydrophobic modification of lignin was carried out by the electrostatic self-assembly method to prepare microspheres with an AVM loading rate of 62.58 ± 0.06%, where the obtained microspheres also show good performance on the release control of AVM and resistance to photolysis.107 Liang et al. synthesized biomimetic mussel AVM NPs with a diameter of about 120 nm and an >50% adsorption rate of AVM (w/w), which showed good adhesion to crop leaves, excellent storage and light stability, and sustained-release ability.108 In addition, Guan et al. used raw materials to prepare AVM nanoemulsions that can be efficiently improve the photostability of AVM and provide strong target crop leaf adhesion and low residue.109 As a non-inhalant insecticide, AVM is difficult to be absorbed and transmitted by plants because it only has a small amount of osmotic absorption at the application site. Wang et al. prepared AVM/glycine methyl ester-modified polysuccinimide NPs (AVM@PGA) by self-assembly, where the release of AVM has obvious pH sensitivity, and the anti-UV photolysis ability of AVM is greatly improved.34 In addition, AVM@PGA can also regulate the transmission and distribution of AVM in plants, which has a good effect on the prevention of plant pests.
In addition to poor UV light stability and low water solubility, AVM's poor fluidity in soil can also affect its nematode performance due to the limited protected areas around the plant growth roots. Cao et al. encapsulated AVM in red trilobite necrosis virus to manipulate the physicochemical properties of the soil of AVM (Fig. 3c), and it was found that PVNAVM expanded the treatment range of root-knot nematodes in the soil and improved its insect resistance activity against nematodes compared to the free AVM molecular treatment.55 A simple electrostatic interaction method was reported to encapsulate AVM within NPs composed of poly-γ-glutamic acid (γ-PGA) and chitosan (CS), which achieved high water dispersibility, anti-photolysis, high nematicidal activity, and controlled-release multifunctional nanocarrier of pesticides (Fig. 3d).81 All this research on different types of nanoencapsulation technologies of AVM provides references for the preparation of more efficient, stable, and environment-friendly AVM formulations.
Nanobotanical insecticide.
Botanical pesticides are a branch of the biopesticide family, and have advantages of low residues and toxicity, no bioaccumulation, and are environment friendly.111 The secondary metabolites produced by plants, such as alkaloids, flavonoids, terpenoids, and volatile oils,112 are used in the development and utilization of botanical pesticides.113,114 However, there are problems such as difficulty in formulation, poor stability, and easy decomposition due to their special sources and complex components, which affect the efficacy of active ingredients.113 The application of nanotechnology has helped to solve these problems of botanical pesticides in agricultural application to a large extent (Fig. 4).115 Nanoemulsions have been developed to improve the chemical stability, hydrophilicity, and environmental durability of botanical pesticides.116 Nanoemulsions based on plant essential oils have broad applications in insecticides and have good insecticidal activity against various agricultural pests.117–120 For example, Iqbal et al. developed a stable neem oil-based nanoemulsion (NE) formulation with bio-inspired adjuvant of Cymbopogon citratus and Prosopis juliflora with long-term storage stability that showed significant insecticidal activity against Bemisia tabaci in eggplant (91.24%) by reducing the surface tension and contact angle.121 Wuryantini et al. compared the efficacy of tobacco plant-derived insecticide nanoemulsion and crude extract and found that the nanoemulsion had better control effect on Citrus aphids than the crude extract,122 indicating that the use of nanoagents has broad prospects in improving the utilization of active ingredients of plant-derived pesticides.
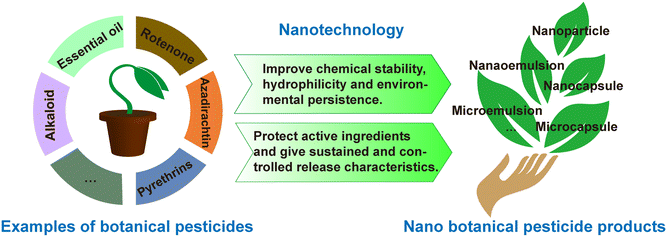 |
| Fig. 4 Application of nanotechnology for botanical pesticides. The nanobotanical pesticide products mainly contain nanoparticles, nanoemulsions, nanocapsules, microcapsules, and microemulsions. Also, the performances of traditionally botanical pesticides could be effectively improved by nanotechnology. | |
Incorporating polymers into botanical pesticide formulations to form nanospheres or nanocapsules can reduce the loss of active ingredients and achieve controlled release.123 The microencapsulation of essential oils can improve the oxidative stability, thermal stability, shelf life, and biological activity of oils,86,124 and it can also control the volatility and release properties of essential oils.125 Feng et al. synthesized an amphiphilic carboxymethyl chitosan with ricinoleic acid (R–CM-chitosan) to be used as a carrier for the botanical pesticide azadirachtin (Aza), where the formed Aza/R–CM-chitosan water dispersion on the nanoscale level had a better controlled release activity.46 González et al. found that the incorporation of geranium or bergamot essential oil into a solid controlled-release nanoformulation could prevent its rapid evaporation and degradation and enhance its stability and insecticidal activities through contact and ingestion.126
Moreover, plant polyphenols are embedded in or adsorbed on the surface of nanoparticles to produce polyphenol particles, which have stronger activity in terms of solubility, antioxidant stability, and wider application range. Three plant compounds (geraniol, eugenol, and cinnamaldehyde) were encapsulated in zein NPs,127 where the nanoencapsulation provided protection against the degradation of the compounds during storage and resulted in reduced toxicity to non-target organisms, while also showing superiority to the emulsifying compound in terms of repellent and insecticidal activity. In all, the application of nanotechnology in botanical insecticides can improve their chemical stability, hydrophilicity, environmental durability, and insecticidal activity.
2.3 Nanotechnology in fungicides
Plant pathogens can infect the plant tissue at different stages of crop growth,128 and phytopathogenic fungi are a type of plant pathogen that can cause great economic losses worldwide.129 Nanotechnology can offer ecofriendly and green alternatives for plant disease control, and the use of nanotechnology to develop safe antifungal agents is of great significance.129–134 Nanobiofungicides are fungicides formulated at the nanometer size with novel properties and can be designated as nanohybrids or nanocomposites.135,136 It has been reported that microorganisms (including bacteria, fungi, actinomyces, yeast, and algae) and plants are able to synthesize nanofungicides,137–139 and the biosynthesis of nanofungicides has been recognized as an efficient and green method.140
Silver NPs can be synthesized by a biological method using Bacillus amyloliquefaciens as a new source, and in vitro studies have revealed that biosynthesized AgNPs exhibit good antifungal effects on Fusarium solani and Fusarium oxysporium, which indicates that the low-cost and ecofriendly nanobiofungicide can be a promising alternative to traditional chemical fungicides.141 A novel nanobiofungicide encapsulated in nanoscale liposome particles with high antifungal activity against Fusarium spp. was reported, which was synthesized by ethanol crude extract from plant growth-promoting bacteria.142 The co-effect of bioagent Bacillus subtilus, -TiO2, and nano-ZnO was investigated against powdery mildew disease caused by Podosphaera xanthii, and the result indicated that the combination of B. subtilus and nano-ZnO treatment could significantly reduce the symptoms and severity of P. xanthii by reducing electrolyte leakage and elevating the ROS levels.87 Phytofabricated ZnO NPs were synthesized using the aqueous plant leaf extract of Terminalia bellerica, and it has shown significant antifungal potential against blight disease/leaf spot disease.88 Moreover, ZnO NPs and ZnO-based nanobiohybrids have been synthesized by a chemical route and green synthesis from garlic extract, respectively, and it was found that the nanobiohybrids exhibited a fungal inhibition of 72.4%, while the inhibition of the chemically-synthesized ZnO NPs was 87.1%. In addition, ZnO-based and phytofabricated ZnO NPs have also been reported as nanofungicides to manage plant pathogens.88,143 All these studies suggest that the biosynthesis of nanobiofungicides is cheaper and more environmentally sustainable and stable than using chemical synthesis methods.
Chitin/chitosan-based stimulus responsive nanopreparations can release active nanopesticides more accurately and effectively and adopt targeted delivery or controlled-release mechanism. Nanochitin has good ability to inhibit mycelial growth, a strong ability to inhibit conidial formation, and increase disease control efficiency when applied as a seed-coating agent and/or when mixed with chemical fungicide.38 Zhou et al. reported that nanochitin can help with seed germination, plant growth promotion, and fungicide efficacy in inhibiting tobacco root rot.144 Saharan et al. synthesized chitosan-copper nanocomposites and showed excellent antifungal activity in tomato, where it has also been proved to be an important growth promoter in different crops.145 Dananjaya et al. studied the antifungal activity of chitosan–silver nanocomposites on Fusarium oxysporum species complexes, and the results showed that chitosan–silver nanocomposites caused obvious damage to the surface of mycelium and increased membrane permeability and, sometimes, cell disintegration.146 Oleoyl–chitosan nanocomposites were tested on pathogenic fungi (Verticillium dahlia), which showed that the oleoyl–chitosan nanoparticles dramatically decreased the mycelium growth.147 All these findings show that chitin/chitosan could be used as excellent nanocarriers.
Moreover, porous hollow silica NPs were prepared as the controlled delivery system of validamycin to enhance the activity and reduce the toxicity compared to free validamycin, and it was shown that the release rate of validamycin is mainly related to the pH and temperature of the dissolution medium.93 Nanosized calcium carbonate was used as a carrier to load with the biopesticide validamycin, where the composite exhibited high loading efficiency, sustained-release performance, and good environmental compatibility, and it displayed better germicidal efficacy against Rhizoctonia solani compared to conventional validamycin.94 Similarly, the dual-functionalized pesticide nanocapsules (loaded with validamycin and thifluzamide) exhibited good foliar spread to reduce the loss of pesticide on leaves, and it had a clear synergistic effect between the two active ingredients.148 A nanoconjugate derived from kasugamycin with amino-modified silica was developed by Ding et al., and it was found that the kasuga-silica can effectively protect kasugamycin against photodegradation and the release rate of kasugamycin was dependent on the temperature, pH, and particle size.95 Overall, the application of nanotechnology in the fungicide could effectively improve the performance of biopesticides to a large extent.115
2.4 Nanobactericides
At present, there are also many explorations of nanotechnology in the bactericides field. Liang et al. prepared ZnO@ZIF-8 nanospheres with the core–shell structure by the in situ growth method and used it to load berberine with efficient synergistic bactericidal effect, which could induce reactive oxygen species (ROS) generation, cause bacterial DNA damage, cytoplasm leakage and, membrane permeability changes (Fig. 5a).149 Peppermint oil and green tea oil were encapsulated in chitosan NPs by emulsification–ionic gelation technique; it was found that nanoencapsulation maintained the stability of the total phenolic contents in both EOs, and the antioxidant and antibacterial activity (against Staphylococcus aureus and Escherichia coli) was significantly improved for peppermint oil and green tea oil, respectively (Fig. 5b).43 Leimann et al. found that microencapsulation of lemongrass oil with polyvinyl alcohol (PVA) could protect the oil from spoilage and maintain its antibacterial activity.150 Du et al. compounded Zn2+ particles into chitosan solution, which showed a significant improvement of the antibacterial activity of the composites with increasing Zn2+ concentration.151 The fabricated CuNP composite nanogel showed high controllability of Pseudomonas syringae on tobacco and safety compared to the commercial bactericide thiodiazole copper.152 All these studies indicated that nanotechnology can improve the antibacterial activity of traditional biopesticides.
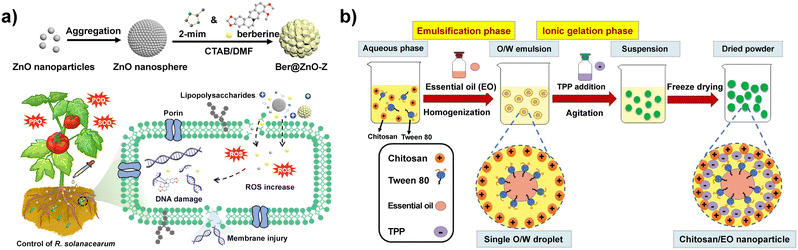 |
| Fig. 5 a) Illustration of the synthesis of Ber@ZnO-Z nanospheres and their applications in the synergistic control of bacterial wilt disease of tomato. Adapted with permission from ref. 149. Copyright 2022, American Chemical Society. b) Nanoencapsulation of essential oils by the emulsification–ionic gelation method. Adapted with permission from ref. 43. Copyright 2019, Elsevier B.V. | |
Moreover, pure nanomaterials also show high antibacterial activity, and the use of nanomaterials as active ingredients for crop protection is also a very important field of research. Silver, zinc, and copper nanoparticles are most reported in agricultural practices as the antibacterial agent.153 Silver chloride NPs was also biosynthesized by Rhizospheric bacteria and exhibited high antibacterial activity against Ralstonia solanacearum;154 the green synthesized Ag NPs exhibited long-term antimicrobial properties and attenuated toxicity to plants compared with chemically synthesized Ag NPs,155 which suggested that the green synthesized Ag NPs could be used as potential nanopesticide or nanoscale growth regulator in agriculture. ZnO nanomaterials also showed high antibacterial activity (against Xanthomonas citri) without phototoxicity.156 It was found that the shape of the NP significantly influences the antibacterial activity of spherical CuO NPs and nanosheets of CuO, and both NPs could damage the bacterial membrane and DNA.157 Moreover, the biosynthesized magnesium oxide NPs could be used as effective antibacterial agents against rice pathogen Acidovorax oryzae, indicating that MgO NPs could be used to formulate a potent nanopesticide.158
2.5 Bioherbicides and nanotechnology
Agricultural weed is a kind of plant adapted to the agricultural environment, which directly and indirectly interferes with crop production and causes great economic losses worldwide.159 The control of weeds mainly depends on herbicides and includes chemical herbicides and biological herbicides (industrially produced by microorganisms, plants, and animals or their tissues and metabolites).160–162 However, in practical application, the herbicide utilization rate is low due to man-made or environmental factors, which also causes a series of problems to the environment, increasingly serious damage to the ecological environment, thus significantly affecting the sustainable development of agriculture.163 The application of nanomaterials and technology in the field of pesticides can change the physical and chemical properties of pesticides, fully improve the utilization rate of pesticides, reduce pesticide residues, and reduce environmental pollution.164–166
Bioherbicides are natural products that can be used for weed control,167 which show a short residual period in the environment and less pollution and adverse effects on soil, water, and non-target organisms.161,168 However, the relatively short half-life reduces the weed control effect in the field scale, and the complicated and expensive preparations restrict the development of biological herbicides.169 Therefore, it is necessary to develop suitable protective bioherbicide active substances to optimize their deployment and effect in the field.169 However, few reports have focused on the combination of biological herbicides and nanotechnology, where research on biological herbicides has great potential for innovation and development. At present, the nanoformulation technology that has attracted considerable attention is the nanocapsule. Taban et al. studied the herbicidal activity of different concentrations of nanocapsule mint essential oil, and it was found that the nanocapsule herbicide had a considerable herbicidal activity on weeds and a mild effect on non-target crops.86 Some NPs (i.e., alginate, chitosan, nanoclay, and lipids) with special chemical properties can be used as bioherbicide carriers to control the release of active components, thus reducing the loss of active components and improving the utilization rate of bioherbicides.115,163 The herbicide metabolites were extracted from the free supernatant of Fusarium oxysporum isolated from soil and coated with chitosan NPs, and it was found that chitosan NPs could be used as the carrier of F. oxysporum herbicide, which showed good herbicidal activity against weeds.89 In addition, the nanoemulsion system can also be used as another way of effectively delivering biological herbicides, where the NPs are evenly dispersed on the surface of leaves, enhance the permeability of the active ingredients in weed leaves, and thus show better control effects on weeds.90 It is believed that nanobiological herbicides that combine biological herbicides with green, inexpensive nanomaterials will play an important role in reducing environmental pollution and the sustainable and healthy development of agriculture (Fig. 6), but it is necessary to consider the compatibility of NPs with other biological agents and their specificity to plant hosts under field conditions to limit or avoid further damage to the environment and human health.168,170
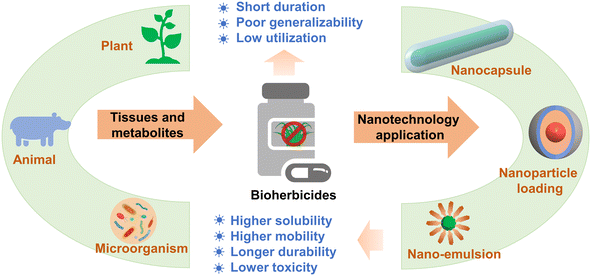 |
| Fig. 6 Nanotechnology application in bioherbicides that could potentially enhance the solubility, mobility, and durability, and reduce the toxicity. | |
2.6 Nanotechnology application in other types of biopesticides
RNA biopesticides use RNA interference (RNAi) to inhibit important gene expression in target organisms, leading to the developmental retardation or death of harmful organisms, thus achieving the purpose of pest control. The traditional delivery system for RNA pesticides is usually influenced by various external factors that can lead to low efficiency and hinder its application in modern agriculture. In recent years, nanodelivery system-based RNA pesticides have drawn increasing attention in the development of green and sustainable agriculture (Fig. 7). Compared to traditional delivery strategies, the nanocarrier-mediated RNAi delivery system has several advantages such as high efficiency, low dose, and slow release. RNAi is considered a safe pest control strategy due to its high sequence specificity.171
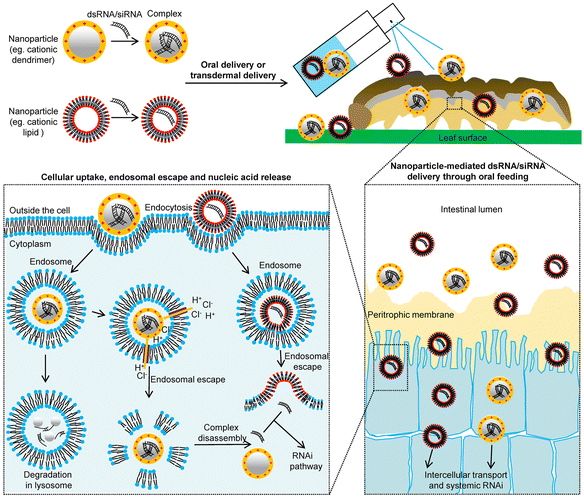 |
| Fig. 7 Schematic representation of a nanoparticle-mediated double-stranded RNA/small interfering RNA (dsRNA/siRNA) delivery system. Adapted with permission from ref. 171. Copyright 2020, John Wiley & Sons Australia, Ltd on behalf of Institute of Zoology, Chinese Academy of Sciences. | |
In general, the siRNA, miRNA, and piRNA pathways would be the RNAi pathways in insects,172 but double-stranded RNA (dsRNA) may be restricted or limited to the xylem vessels and plant vascular system. At present, a novel delivery strategy based on nanocarriers can overcome the cell wall barriers of plants and accurately deliver DNA or RNA into the plant, leading to transient or stable transformation.173 A perylene imide nanocarriers-mediated dsRNA delivery system was developed on Aphis glycines, where the interference efficiency of the nanocomplex on the target gene reached 95.4% and the population inhibition effect was 80.5%.84 Moreover, a star polycation-based gene and drug co-delivery system was constructed to deliver hemocytin (hem) dsRNA and botanical pesticide matrine against green peach aphids, and it was found that the matrine/star polycation/dshem complex could overcome the short life disadvantage of dshem an slow-acting properties of matrine simultaneously.82 Guanidine-containing polymers were developed to protect dsRNA against nucleolytic degradation in alkaline intestinal environments, where they can effectively interfere with the essential gene chitin synthase B, and the mortality rate of Spodoptera exigua increased from 16% to 53% by the polymer-protected dsRNA treatment.174 dsRNA loaded on layered double hydroxide (LDH) clay nanosheets (formation of BioClay) can provide sustained-release performance, where dsRNA can be detected on sprayed leaves even 30 days after application, and the BioClay could achieve a 76% antiviral efficiency on being sprayed on Arabidopsis thaliana leaves.85 The smaller and cheaper soybean phosphatidylcholine (SPc) was selected as the nanocarrier to effectively penetrate the body wall of the soybean aphid through topical application and spraying, with a high mortality up to 81.67% and 78.50%, respectively.172
Although RNA pesticides have been a breakthrough in the fields of pest control and plant protection, the instability, high cost, and short life of dsRNA seriously constrain their commercial application.82 Meanwhile, the dsRNA delivery efficiency is often low, and it is difficult to produce sufficient amounts of stable dsRNA in traditional transgenesis because of the plant RNAi machinery.171 Hence, more in-depth studies on nanodelivery system-based RNA pesticides must be conducted before the broad usage of nanodelivery system-based RNA pesticides.171 For instance, cheap raw materials have been used in the synthesis process of NPs to overcome the high production cost of nanoparticle-based RNA pesticides, where the common commercial chemical pentaerythritol is used as the star initiator to reduce the production costs.175 Meanwhile, the potential risk and biocompatibility of nanodelivery system-based RNA pesticides should be assessed before their application,176,177 and the non-target effects and insect resistance to RNA pesticides should be considered.178
3. The uptake and transport of nanobiopesticides in plants
In recent years, many studies have focused on the construction of smart controlled-release nanopesticide formulations and biological activity evaluation.179 The uptake, transport, and distribution of nanopesticides in plants are not fully understood, and the phytotoxicity and genotoxicity caused by NPs are rarely considered.180–183 Indeed, understanding the interaction behavior between nanopesticides and plants is helpful for designing new nanocarrier systems for desired applications,184 and it is also conducive to revealing their biocumulative effects and biological safety to provide guidance for the safe use of nanopesticides.185–187
The efficient deposition and strong adhesion of pesticides on the foliage surface are key factors in minimizing pesticide loss and improving the utilization efficiency.179 NPs are easily adsorbed or aggregated on plant epidermis by electrostatic adsorption, mechanical adhesion, and hydrophobic affinity due to their large specific surface area and high surface reactivity.188 The interaction between nanopesticides and plants mainly includes three steps: (ref. 20 and 189) (1) NPs are deposited or adsorbed on the surface of plants (roots, stems, and leaves); (2) NPs permeate into the cuticle and epidermis, and then migrate to the vascular tissues by means of symplasts or exoplasms; (3) NPs are transported to other parts of the plant through the vascular tissue (illustrated in Fig. 8). Although similar features exist in roots (an epidermis with stomata, mesophyll, and vascular tissue) and leaves (trichomes, cuticular folds, and wax crystals), the different morphological characteristics of roots leaves would lead to different barriers for NPs entry.20 Meanwhile, the accumulation and transport of NPs also depend on nanoparticle shape, application method, and nature of plant tissues, and the translocation of NPs from leaf to root was achieved by the phloem transport mechanism.190
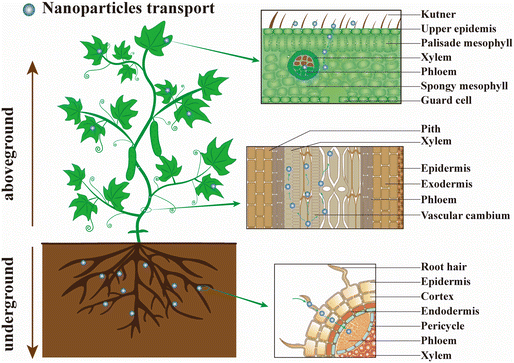 |
| Fig. 8 Schematic diagrams of the uptake and transport process of NPs in plants. The NPs could deposit or adsorb on the surface of plants (roots, stems and leaves); or permeate into cuticle and epidermis, and then migrate to vascular tissues by means of symplasts or exoplasms; or transported to other parts of the plant through the vascular tissue. | |
More details on the uptake, transport, and fate of NPs in plants could be seen in Su et al.'s20 and Schwab et al.'s reviews,191 and most reports focus on the delivery of the nanopesticide. Currently, the transport of avermectin-based nanobiopesticides has attracted some attention. Yu et al. have developed three types of functionalized abamectin poly(lactic acid) (Abam-PLA) NPs with different adhesion abilities to cucumber foliage, where the adhesion of Abam-PLA NPs to the foliage surface mainly depends on the surface functional groups of NPs and was easily regulated by varying the functional groups.179 Liang et al. have developed avermectin NPs with a particle size of 120 nm using a copolymer (styrene and methacrylic acid) as the avermectin carrier and polycatechol as the adhesive group on the surface. The interaction force between NPs and the surface of cucumber and broccoli foliages is mainly derived from the hydrogen bond, which highly enhanced the adhesive performance on the foliage surface.81 Moreover, multifunctional avermectin/polysuccinimide with glycine methyl ester nanoparticles (AVM–PGA) were prepared,34 where AVM could be detected in stems and all leaves after treatment with AVM–PGA, whereas only few AVM molecules could be detected in treated rice leaves for free-form AVM. The PGA nanocarriers could improve the uptake and transportation of AVM in rice, and could also interact with the plant amino acid transporter to enhance their cellular uptake and phloem loading.192 The above reports implied that nanocarriers can improve the uptake and transport characteristics of nonsystemic AVM biopesticide. In addition, novel Au NPs conjugated with D-glucose (Glc) and rotenone (R) nanopesticide were designed, and it was found that R–Au NPs–Glc could be actively transported into tobacco BY-2 cells by hexose transporters dependent on an energy-dependent process. Glc was found to be a promising ligand for the uptake and delivery of nanopesticides.193
In the future, exploring the interactions between nanopesticides and plants should not be limited to the migration path mechanism but also the dynamic digestion, residual behavior, and toxicology of nanopesticides in plants. To understand the differences in the morphological and physiological characteristics of plants before and after the NP treatment, identifying the key factors in determining the penetration process of NPs into plants, and selecting suitable nanosizes and carriers to reduce the residue and improve the utilization rate of pesticide would be helpful for the development of green and efficient nanopesticides.
4. Future perspectives and challenges of nanobiopesticides
Nanotechnology and new nanomaterials are gaining popularity at the forefront of agricultural industry,194 where numerous publications are focused on the development of nanoformulated pesticides and more commercial products are available in global markets. Although several agrochemical companies (such as Syngenta, Bayer, Monsanto, BASF, and Dow AgroScience) have developed a large number of patents on nanopesticides,195,196 there are still very few types of nanopesticides that have reached the market, except nanoemulsions. The new nanoformulations must compete with existing conventional chemical pesticides with excellent control performance and economic feasibility; some pesticides with high efficacy and a safe environmental profile are already available in the market. For example, plant EOs and neem seed extracts (i.e., azadirachtin) botanical insecticides have been commercialized,197 and NSPW-L30SS, CLARIVA®, SIVANTO® prime, and Nimbecidine EC (0.03% azadirachtin) were developed by agrochemical companies.153
Previous studies have indicated that nanomaterials such as carbon nanotubes and nanosilver can produce acute toxicity in fish, even affect their reproduction, and cause negative effects on algae and other aquatic organisms.198,199 Therefore, the selection of non-toxic, biocompatible, and easily-degradable nanomaterials as the carriers is very important to for the development of nanoformulations,200,201 and it is necessary to intensify the application of nanotechnology in agriculture by developing more stable and safer nanoformulations of biopesticide products. The “green” synthesis of stable nanoformulations of biopesticides with prolonged insecticidal and antimicrobial effects have a promising future, and the biosynthesis of nanoscale materials from a variety of nanoencapsulated biocidal essential oils, enzymes, plant extracts, or biological extract could be a smart alternative.194 The environmental fate of nanobiopesticide formulations should also raise researchers' concerns despite their positive benefits compared to conventional biopesticides, and the potential toxicity of NPs in different crops and the effect on the plant's metabolic activity should also be considered.32,202
Nanobiopesticides can be absorbed, accumulated, and transmitted by the roots and leaves of plants, which is beneficial to the entry of pesticides into the plant and effectively controls of sucking pests and plant diseases. Root absorption is the main pathway for NPs to enter the plants, and the absorbed NPs may be transported upward to the aboveground part of the plants by transpiration,203,204 which makes it possible to control aboveground pests by applying pesticides underground. However, the uptake, bioavailability, and toxicity of nanobiopesticides mainly depend on the particle number, particle stability, and particle size distribution,181 and understanding whether the transport characteristic of nanobiopesticides leads to residues in plants should attract enough attention.204 More studies should be conducted on leaf-targeted bioaccumulation and dose biotransformation mechanisms of nanodelivery to develop specific risk assessment guidelines for nanobiopesticides in the future.
In addition, most reported nanobiopesticides are synthesized at the laboratory level currently, and it is difficult to synthesize high stability nanobiopesticides in large quantities in the preparation process as the preparation process is too complex and sophisticated for industrialization.205 As a result, there are no obvious advantages of equipment investment and production cost of nanobiopesticides when compared with conventional pesticide formulations. Therefore, the development of large-scale standardized preparation of nanobiopesticides is an important research issue, and it is urgent for researchers to develop simple and renewable methods to fabricate nanomaterials to be applied in agriculture fields.4
As for the controlled release of active ingredients nanoformulation, its efficacy and other performance tests must be evaluated over a longer period of time.206,207 As a result, standards for nanoscale preparations and environmental risk assessment criteria should be established to provide basis for new nanopesticide registration, and more field validation trials are needed to evaluate the potential hazards, non-target effects, and resistance of nanobiopesticides by humans and impacts on environmental health.176,208 Moreover, the acceptance of nanopesticides by consumers is low; thus, it seems that nanoparticle-based biopesticides still need more time for an in-depth study to evaluate their safety and increase their acceptance by consumers, and experts and educators should establish more research plans and developmental initiatives to increase the possible interests of customers.
5. Conclusion
Nanotechnology promises a breakthrough in improving the efficacy and safety through the nanoformulation of traditional pesticides/biopesticides, and it is one of the most promising solutions to the real-time challenges in agricultural activity. The development of nanoformulations would promote nanobiopesticide use in agriculture over the next decade. In this review, the potential uses of NPs are briefly illustrated, and the application strategies of nanotechnology in various biopesticides with current and future scenarios are discussed. The uptake and transport of nanobiopesticides in plants are evaluated, and the field evaluations, regulations, and risks involved in nanobiopesticide use are discussed. We believe more governments, universities, industries, and the public should be focused on the nanobiopesticide, and nanotechnology applications in agriculture, which can be successful under greater scientific and sophisticated design.
Author contributions
All the authors contributed to this review. Xiaohong Pan proposed the conceptualization, modified the structures of this review and made subsequent modifications. Xueping Guo wrote the original draft of botanical pesticide, and participated in specifically visualization presentation. Tianyun Zhai wrote the original draft of avermectin and nano chitin/chitosan. Dingyang Zhang wrote the original draft of nanobioherbicides, and participated in references proofreading. Wenhua Rao and Fang Cao wrote the original draft of Bt nanobiopesticide and nanobiofungicides. Xiong Guan acquired the funding and supervised the writing.
Conflicts of interest
There are no conflicts to declare.
Acknowledgements
This work was supported by the Natural Science Foundation of Fujian Province, China (2020 J01522), Fujian Agriculture and Forestry University Construction Project for Technological Innovation and Service System of Tea Industry Chain (K1520005A03), and the Special Fund for Scientific and Technological Innovation of Fujian Agriculture and Forestry University (CXZX2019005S and CXZX2020024A).
References
- G. V. Lowry, A. Avellan and L. M. Gilbertson, Opportunities and challenges for nanotechnology in the agri-tech revolution, Nat. Nanotechnol., 2019, 14, 517 CrossRef CAS PubMed
.
- D. Wang, N. B. Saleh, A. Byro, R. Zepp, E. Sahle-Demessie, T. P. Luxton, K. T. Ho, R. M. Burgess, M. Flury, J. C. White and C. Su, Nano-enabled pesticides for sustainable agriculture and global food security, Nat. Nanotechnol., 2022, 17, 347–360 CrossRef CAS
.
- F. Gomollón-Bel, Ten chemical innovations that will change our world: IUPAC identifies emerging technologies in chemistry with potential to make our planet more sustainable, Chem. Int., 2019, 41, 12–17 Search PubMed
.
- D. Abdollahdokht, Y. Gao, S. Faramarz, A. Poustforoosh, M. Abbasi, G. Asadikaram and M. H. Nematollahi, Conventional agrochemicals towards nano-biopesticides: an overview on recent advances, Chem. Biol. Technol. Agric., 2022, 9, 13 CrossRef
.
-
Y. Abubakar, H. Tijjani, C. Egbuna, C. O. Adetunji, S. Kala, T. L. Kryeziu, J. C. Ifemeje and K. C. Patrick-Iwuanyanwu, in Natural Remedies for Pest, Disease and Weed Control, eds. C. Egbuna and B. Sawicka, Academic Press, 2020, pp. 29–42, DOI:10.1016/B978-0-12-819304-4.00003-8
.
- V. Ghormade, M. V. Deshpande and K. M. Paknikar, Perspectives for nano-biotechnology enabled protection and nutrition of plants, Biotechnol. Adv., 2011, 29, 792–803 CrossRef CAS
.
- T. Glare, J. Caradus, W. Gelernter, T. Jackson, N. Keyhani, J. Köhl, P. Marrone, L. Morin and A. Stewart, Have biopesticides come of age?, Trends Biotechnol., 2012, 30, 250–258 CrossRef CAS
.
- S. Arthurs and S. K. Dara, Microbial biopesticides for invertebrate pests and their markets in the United States, J. Invertebr. Pathol., 2019, 165, 13–21 CrossRef
.
- F. Cappa, D. Baracchi and R. Cervo, Biopesticides and insect pollinators: Detrimental effects, outdated guidelines, and future directions, Sci. Total Environ., 2022, 837, 155714 CrossRef CAS
.
- S. Baker, T. Volova, S. V. Prudnikova, S. Satish and P. Nagendra, Nanoagroparticles emerging trends and future prospect in modern agriculture system, Environ. Toxicol. Pharmacol., 2017, 53, 10–17 CrossRef CAS
.
- J. Popp, K. Pető and J. Nagy, Pesticide productivity and food security. A review, Agron. Sustainable Dev., 2013, 33, 243–255 CrossRef
.
- P. Ram, K. Vivek and S. P. Kumar, Nanotechnology in sustainable agriculture: Present concerns and future aspects, Afr. J. Biotechnol., 2014, 13, 705–713 CrossRef
.
- L. R. Khot, S. Sankaran, J. M. Maja, R. Ehsani and E. W. Schuster, Applications of nanomaterials in agricultural production and crop protection: A review, Crop Prot., 2012, 35, 64–70 CrossRef CAS
.
- R. K. Patel, Nanotechnology pros and cons to agriculture: A review, Int. J. Curr. Microbiol. Appl. Sci., 2014, 3, 43–55 Search PubMed
.
-
O. Koul, in Nano-biopesticides today and future perspectives, ed. O. Koul, Academic Press, 2019, pp. 1–15, DOI:10.1016/B978-0-12-815829-6.00001-2
.
-
S. R. Djiwanti and S. Kaushik, in Plant nanobionics: Approaches in nanoparticles, biosynthesis, and toxicity, ed. R. Prasad, 2019, pp. 255–298, DOI:10.1007/978-3-030-16379-2_10
.
- C. An, C. Sun, N. Li, B. Huang, J. Jiang, Y. Shen, C. Wang, X. Zhao, B. Cui, C. Wang, X. Li, S. Zhan, F. Gao, Z. Zeng, H. Cui and Y. Wang, Nanomaterials and nanotechnology for the delivery of agrochemicals: strategies towards sustainable agriculture, J. Nanobiotechnol., 2022, 20, 11 CrossRef CAS PubMed
.
-
A. Rasool, W. H. Shah, I. Tahir and R. U. Rehman, in Plant panobionics: Volume 2, Approaches in nanoparticles, biosynthesis, and toxicity, ed. R. Prasad, Springer International Publishing, Cham, 2019, pp. 235–253, DOI:10.1007/978-3-030-16379-2_9
.
- Z. Xu, T. Tang, Q. Lin, J. Yu, C. Zhang, X. Zhao, M. Kah and L. Li, Environmental risks and the potential benefits of nanopesticides: a review, Environ. Chem. Lett., 2022, 20, 2097–2108 CrossRef CAS
.
- Y. Su, V. Ashworth, C. Kim, A. S. Adeleye, P. Rolshausen, C. Roper, J. White and D. Jassby, Delivery, uptake, fate, and transport of engineered nanoparticles in plants: a critical review and data analysis, Environ. Sci.: Nano, 2019, 2311–2331 RSC
.
- M. L. Ermini and V. Voliani, Antimicrobial nano-agents: The copper age, ACS Nano, 2021, 15, 6008–6029 CrossRef CAS
.
- M. A. Farooq, F. Hannan, F. Islam, A. Ayyaz, N. Zhang, W. Chen, K. Zhang, Q. Huang, L. Xu and W. Zhou, The potential of nanomaterials for sustainable modern agriculture: present findings and future perspectives, Environ. Sci.: Nano, 2022, 9, 1926–1951 RSC
.
- T. S. Santos, T. M. Silva, J. C. Cardoso, R. L. C. de Albuquerque, A. Zielinska, E. B. Souto, P. Severino and M. D. Mendonca, Biosynthesis of silver nanoparticles mediated by entomopathogenic fungi: Antimicrobial resistance, nanopesticides, and toxicity, Antibiotics, 2021, 10, 852 CrossRef CAS
.
- S. Kumar, M. Nehra, N. Dilbaghi, G. Marrazza, A. A. Hassan and K. H. Kim, Nano-based smart pesticide formulations: Emerging opportunities for agriculture, J. Controlled Release, 2019, 294, 131–153 CrossRef CAS
.
- T. Ahmed, M. Noman, H. Jiang, M. Shahid, C. Ma, Z. Wu, M. M. Nazir, M. A. Ali, J. C. White, J. Chen and B. Li, Bioengineered chitosan-iron nanocomposite controls bacterial leaf blight disease by modulating plant defense response and nutritional status of rice (Oryza sativa L.), Nano Today, 2022, 45, 101547 CrossRef CAS
.
- W. Yang, B. Wang, G. Lei, G. Chen and D. Liu, Advances in nanocarriers to improve the stability of dsRNA in the environment, Front Bioeng Biotechnol., 2022, 10, 974646 CrossRef
.
- Q. Zheng, D. Qin, R. Wang, W. Yan, W. Zhao, S. Shen, S. Huang, D. Cheng, C. Zhao and Z. Zhang, Novel application of biodegradable chitosan in agriculture: Using green nanopesticides to control Solenopsis invicta, Int. J. Biol. Macromol., 2022, 220, 193–203 CrossRef CAS
.
- M. Granetto, L. Serpella, S. Fogliatto, L. Re, C. Bianco, F. Vidotto and T. Tosco, Natural clay and biopolymer-based nanopesticides to control the environmental spread of a soluble herbicide, Sci. Total Environ., 2022, 806(Part 3), 151199 CrossRef CAS PubMed
.
-
P. Ganguli, in Nano-Biopesticides Today and Future Perspectives, ed. O. Koul, Academic Press, 2019, pp. 367–395, DOI:10.1016/B978-0-12-815829-6.00017-6
.
- M. Kah, S. Beulke, K. Tiede and T. Hofmann, Nanopesticides: State of knowledge, environmental fate, and exposure modeling, Crit. Rev. Environ. Sci. Technol., 2013, 43, 1823–1867 CrossRef CAS
.
- M. Kah and T. Hofmann, Nanopesticide research: Current trends and future priorities, Environ. Int., 2014, 63, 224–235 CrossRef CAS PubMed
.
-
B. D. Lade, D. P. Gogle, D. B. Lade, G. M. Moon, S. B. Nandeshwar and S. D. Kumbhare, in Nano-Biopesticides
Today and Future Perspectives, ed. O. Koul, Academic Press, 2019, pp. 179–206, DOI:10.1016/B978-0-12-815829-6.00007-3
.
- M. Kah, R. S. Kookana, A. Gogos and T. D. Bucheli, A critical evaluation of nanopesticides and nanofertilizers against their conventional analogues, Nat. Nanotechnol., 2018, 13, 677–684 CrossRef CAS PubMed
.
- G. Wang, Y. Xiao, H. Xu, P. Hu, W. Liang, L. Xie and J. Jia, Development of multifunctional avermectin poly(succinimide) nanoparticles to improve bioactivity and transportation in rice, J. Agric. Food Chem., 2018, 66, 11244–11253 CrossRef CAS PubMed
.
- G. W. Walker, R. S. Kookana, N. E. Smith, M. Kah, C. L. Doolette, P. T. Reeves, W. Lovell, D. J. Anderson, T. W. Turney and D. A. Navarro, Ecological risk assessment of nano-enabled pesticides: A perspective on problem formulation, J. Agric. Food Chem., 2018, 66, 6480–6486 CrossRef CAS PubMed
.
- X. Lv, M. Yuan, Y. Pei, C. Liu, X. Wang, L. Wu, D. Cheng, X. Ma and X. Sun, The enhancement of antiviral activity of chloroinconazide by aglinate-based nanogel and its plant growth promotion effect, J. Agric. Food Chem., 2021, 69, 4992–5002 CrossRef CAS PubMed
.
- J. Yang, J. Feng, K. He, Z. Chen, W. Chen, H. Cao and S. Yuan, Preparation of thermosensitive buprofezin-loaded mesoporous silica nanoparticles by the sol-gel method and their application in pest control, Pest Manage. Sci., 2021, 77, 4627–4637 CrossRef CAS PubMed
.
- L. Rui, L. Xueyun, Y. Wanling, J. Songyao, H. Shuaitao, W. Min and W. Hezhong, Antifungal activity of nanochitin whisker against crown rot diseases of wheat, J. Agric. Food Chem., 2018, 66, 9907–9913 CrossRef PubMed
.
- Y. Zhang, Y. Niu, Y. Luo, M. Ge, T. Yang, L. Yu and Q. Wang, Fabrication, characterization and antimicrobial activities of thymol-loaded zein nanoparticles stabilized by sodium caseinate–chitosan hydrochloride double layers, Food Chem., 2014, 142, 269–275 CrossRef CAS PubMed
.
- Z. Ahmadi, M. Saber, M. Bagheri and G. R. Mahdavinia, Achillea millefolium essential oil and chitosan nanocapsules with enhanced activity against Tetranychus urticae, J. Pest Sci., 2018, 91, 837–848 CrossRef
.
- B. H. Feng and Z. Y. Zhang, Carboxymethy chitosan grafted ricinoleic acid group for nanopesticide carriers, Adv. Mater. Res., 2011, 236–238, 1783–1788 CAS
.
- J. Wu, C. Du, J. Zhang, B. Yang, A. G. S. Cuthbertson and S. Ali, Synthesis of Metarhizium anisopliae–chitosan nanoparticles and their pathogenicity against Plutella xylostella (Linnaeus), Microorganisms, 2021, 10, 1 CrossRef
.
- A. Shetta, J. Kegere and W. Mamdouh, Comparative study of encapsulated peppermint and green tea essential oils in chitosan nanoparticles: Encapsulation, thermal stability, in-vitro release, antioxidant and antibacterial activities, Int. J. Biol. Macromol., 2019, 126, 731–742 CrossRef CAS
.
- X. Zhang, K. Mysore, E. Flannery, K. Michel, D. W. Severson, K. Y. Zhu and M. Duman-Scheel, Chitosan/Interfering RNA nanoparticle mediated gene silencing in disease vector mosquito larvae, J. Visualized Exp., 2016, 97, e52523 Search PubMed
.
- R. K. Dhandapani, D. Gurusamy, J. L. Howell and S. R. Palli, Development of CS-TPP-dsRNA nanoparticles to enhance RNAi efficiency in the yellow fever mosquito, Aedes aegypti, Sci. Rep., 2019, 9, 8775 CrossRef PubMed
.
- B. H. Feng and L. F. Peng, Synthesis and characterization of carboxymethyl chitosan carrying ricinoleic functions as an emulsifier for azadirachtin, Carbohydr. Polym., 2012, 88, 576–582 CrossRef CAS
.
- A. Esmaeili and S. Ghobadianpour, Antibacterial activity of Carum copticum extract loaded MnFe2O4 nanoparticles coated with PEGylated chitosan, Ind. Crops Prod., 2016, 91, 44–48 CrossRef CAS
.
- J. Zhao, X. Fu, S. Zhang and W. Hou, Water dispersible avermectin-layered double hydroxide nanocomposites modified with sodium dodecyl sulfate, Appl. Clay Sci., 2011, 51, 460–466 CrossRef CAS
.
- L. M. Silva, L. E. Hill, E. Figueiredo and C. L. Gomes, Delivery of phytochemicals of tropical fruit by-products using poly (DL-lactide-co-glycolide) (PLGA) nanoparticles: Synthesis, characterization, and antimicrobial activity, Food Chem., 2014, 165, 362–370 CrossRef CAS PubMed
.
- L. Chronopoulou, L. Donati, M. Bramosanti, R. Rosciani, C. Palocci, G. Pasqua and A. Valletta, Microfluidic synthesis of methyl jasmonate-loaded PLGA nanocarriers as a new strategy to improve natural defenses in Vitis vinifera, Sci. Rep., 2019, 9, 18322 CrossRef CAS PubMed
.
- M. Pascoli, F. P. de Albuquerque, A. K. Calzavara, B. Tinoco-Nunes, W. H. Cruz Oliveira, K. C. Goncalves, R. A. Polanczyk, J. F. Della Vechia, S. T. Soares de Matoss, D. J. de Andrade, H. C. Oliveira, J. A. Souza-Neto, R. de Lima and L. F. Fraceto, The potential of nanobiopesticide based on zein nanoparticles and neem oil for enhanced control of agricultural pests, J. Pest Sci., 2020, 93, 793–806 CrossRef
.
- J. L. de Oliveira, E. V. R. Campos, A. E. S. Pereira, T. Pasquoto, R. Lima, R. Grillo, D. J. de Andrade, F. A. dos Santos and L. F. Fraceto, Zein nanoparticles as eco-friendly carrier systems for botanical repellents aiming sustainable agriculture, J. Agric. Food Chem., 2018, 66, 1330–1340 CrossRef CAS PubMed
.
- J. L. de Oliveira, E. V. R. Campos, M. C. Camara, J. F. Della Vechia, S. T. Soares de Matos, D. J. de Andrade, K. C. Goncalves, J. Do Nascimento, R. A. Polanczyk, D. R. de Araujo and L. F. Fraceto, Hydrogels containing botanical repellents encapsulated in zein nanoparticles for crop protection, ACS Appl. Nano Mater., 2020, 3, 207–217 CrossRef CAS
.
- M. Pascoli, M. T. Jacques, D. A. Agarrayua, D. S. Avila, R. Lima and L. F. Fraceto, Neem oil based nanopesticide as an environmentally-friendly formulation for applications in sustainable agriculture: An ecotoxicological perspective, Sci. Total Environ., 2019, 677, 57–67 CrossRef CAS PubMed
.
- J. Cao, R. R. H. Guenthe, T. L. Sit, S. A. Lommel, C. H. Opperman and J. A. Willoughby, Development of abamectin loaded plant virus nanoparticles for efficacious plant parasitic nematode control, ACS Appl. Mater. Interfaces, 2015, 7, 9546–9553 CrossRef CAS
.
- P. Zhao, L. Cao, D. Ma, Z. Zhou, Q. Huang and C. Pan, Translocation, distribution and degradation of prochloraz-loaded mesoporous silica nanoparticles in cucumber plants, Nanoscale, 2018, 10, 1798–1806 RSC
.
- X. Pan, Z. Xu, L. Li, E. Shao, S. Chen, T. Huang, Z. Chen, W. Rao, T. Huang, L. Zhang, S. Wu and X. Guan, Adsorption of insecticidal crystal protein Cry11Aa onto nano-Mg(OH)2: effects on bioactivity and anti-ultraviolet ability, J. Agric. Food Chem., 2017, 65, 9428–9434 CrossRef CAS PubMed
.
- L. Hao, L. Gong, L. Chen, M. Guan, H. Zhou, S. Qiu, H. Wen, H. Chen, X. Zhou and M. Akbulut, Composite pesticide nanocarriers involving functionalized boron nitride nanoplatelets for pH-responsive release and enhanced UV stability, Chem. Eng. J., 2020, 396, 125233 CrossRef CAS
.
- W. Rao, Y. Zhan, S. Chen, Z. Xu, T. Huang, X. Hong, Y. Zheng, X. Pan and X. Guan, Flowerlike Mg(OH)2 cross-nanosheets for controlling Cry1AC protein loss: Evaluation of insecticidal activity and biosecurity, J. Agric. Food Chem., 2018, 66, 3651–3657 CrossRef CAS
.
- C.-C. Wu, Y. Hu, M. Miller, R. V. Aroian and M. J. Sailor, Protection and delivery of anthelmintic protein Cry5B to nematodes using mesoporous silicon particles, ACS Nano, 2015, 9, 6158–6167 CrossRef CAS PubMed
.
- Z. Hajiahmadi, R. Shirzadian-Khorramabad, M. Kazemzad and M. M. Sohani, Enhancement of tomato resistance to Tuta absoluta using a new efficient mesoporous silica nanoparticle-mediated plant transient gene expression approach, Sci. Hortic., 2019, 243, 367–375 CrossRef CAS
.
- Y. Liang, Y. Gao, W. Wang, H. Dong, R. Tang, J. Yang, J. Niu, Z. Zhou, N. Jiang and Y. Cao, Fabrication of smart stimuli-responsive mesoporous organosilica nano-vehicles for targeted pesticide delivery, J. Hazard. Mater., 2020, 389, 122075 CrossRef CAS
.
- Y. Gao, Y. Zhang, S. He, Y. Xiao, X. Qin, Y. Zhang, D. Li, H. Ma, H. You and J. Li, Fabrication of a hollow mesoporous silica hybrid to improve the targeting of a pesticide, Chem. Eng. J., 2019, 364, 361–369 CrossRef CAS
.
- X. Qin, X. Xiang, X. Sun, H. Ni and L. Li, Preparation of nanoscale Bacillus thuringiensis chitinases using silica nanoparticles for nematicide delivery, Int. J. Biol. Macromol., 2016, 82, 13–21 CrossRef CAS PubMed
.
- A. Thammasittirong, K. Prigyai and S. N. Thammasittirong, Mosquitocidal potential of silver nanoparticles synthesized using local isolates of Bacillus thuringiensis subsp. israelensis and their synergistic effect with a commercial strain of B. thuringiensis subsp. israelensis, Acta Trop., 2017, 176, 91–97 CrossRef CAS PubMed
.
- E. Jalali, S. Maghsoudi and E. Noroozian, A novel method for biosynthesis of different polymorphs of TiO2 nanoparticles as a protector for Bacillus thuringiensis from Ultra Violet, Sci. Rep., 2020, 10, 426 CrossRef CAS PubMed
.
- B. Malaikozhundan, B. Vaseeharan, S. Vijayakumar and M. P. Thangaraj,
Bacillus thuringiensis coated zinc oxide nanoparticle and its biopesticidal effects on the pulse beetle, Callosobruchus maculatus, J. Photochem. Photobiol., B, 2017, 174, 306–314 CrossRef CAS
.
- X. Zhao, Z. Meng, Y. Wang, W. Chen, C. Sun, B. Cui, J. Cui, M. Yu, Z. Zeng, S. Guo, D. Luo, J. Q. Cheng, R. Zhang and H. Cui, Pollen magnetofection for genetic modification with magnetic nanoparticles as gene carriers, Nat. Plants, 2017, 3, 956–964 CrossRef CAS PubMed
.
- Y. Zheng, S. You, C. Ji, M. Yin, W. Yang and J. Shen, Development of an amino acid-functionalized fluorescent nanocarrier to deliver a toxin to kill insect pests, Adv. Mater., 2016, 28, 1375–1380 CrossRef CAS
.
- S. Maghsoudi and E. Jalali, Noble UV protective agent for Bacillus thuringiensis based on a combination of graphene oxide and olive oil, Sci. Rep., 2017, 7, 11019 CrossRef PubMed
.
- Y. Jiang, Y. Chen, D. Tian, F. Shen, X. Wan, L. Xu, Y. Chen, H. Zhang, J. Hu and F. Shen, Fabrication and characterization of lignin-xylan hybrid nanospheres as pesticide carriers with enzyme-mediated release property, Soft Matter, 2020, 16, 9083–9093 RSC
.
- C. An, J. Cui, Q. Yu, B. Huang, N. Li, J. Jiang, Y. Shen, W. Chong, S. Zhan, X. Zhao, X. Li, C. Sun, C. Bo, W. Chunxin, G. Fei, Z. Zeng, H. Cui, R. Zhang and Y. Wang, Polylactic acid nanoparticles for co-delivery of dinotefuran and avermectin against pear tree pests with improved effective period and enhanced bioactivity, Int. J. Biol. Macromol., 2022, 206, 633–641 CrossRef CAS PubMed
.
- Y. Yang, Q. Jiang, M. Peng, Z. Zhou, X. Du, M. Yin, J. Shen and S. Yan, A star polyamine-based nanocarrier delivery system for enhanced avermectin contact and stomach toxicity against green peach aphids, Nanomaterials, 2022, 12, 1445 CrossRef CAS PubMed
.
- Z. Li, L. Su, H. Wang, S. An and X. Yin, Physicochemical and biological properties of nanochitin—abamectin conjugate for Noctuidae insect pest control, J. Nanopart. Res., 2020, 22, 286 CrossRef CAS
.
- P. Mônica, P. D. A. Felícia, K. C. Anderson, T.-N. Bruno, H. C. O. Wanderson, C. G. Kelly, A. P. Ricardo, F. D. V. Jaqueline, T. S. D. M. Sidnéia, J. D. A. Daniel, C. O. Halley, A. S.-N. Jayme, D. L. Renata and F. F. Leonardo, The potential of nanobiopesticide based on zein nanoparticles and neem oil for enhanced control of agricultural pests, J. Pest Sci., 2020, 93, 793–806 CrossRef
.
- X. Wang, K. Zheng, W. Cheng, J. Li and S. Yan, Field application of star polymer-delivered chitosan to amplify plant defense against potato late blight, Chem. Eng. J., 2021, 417, 129327 CrossRef CAS
.
- K. Kalimuthu, C. Panneerselvam, C. Chou, L.-C. Tseng, K. Murugan, K.-H. Tsai, A. A. Alarfaj, A. Higuchi, A. Canale, J.-S. Hwang and G. Benelli, Control of dengue and Zika virus vector Aedes aegypti using the predatory copepod Megacyclops formosanus: Synergy with Hedychium coronarium-synthesized silver nanoparticles and related histological changes in targeted mosquitoes, Process Saf. Environ. Prot., 2017, 109, 82–96 CrossRef CAS
.
- C. Qiu, R. Chang, J. Yang, S. Ge, L. Xiong, M. Zhao, M. Li and Q. Sun, Preparation and characterization of essential oil-loaded starch nanoparticles formed by short glucan chains, Food Chem., 2017, 221, 1426–1433 CrossRef CAS PubMed
.
- E. D. Herculano, H. C. B. de Paula, E. A. T. de Figueiredo, F. G. B. Dias and V. D. A. Pereira, Physicochemical and antimicrobial properties of nanoencapsulated Eucalyptus staigeriana essential oil, LWT–Food Sci. Technol., 2015, 61, 484–491 CrossRef CAS
.
- G. Kavoosi, M. Derakhshan, M. Salehi and L. Rahmati, Microencapsulation of zataria essential oil in agar, alginate and carrageenan, Innovative Food Sci. Emerging Technol., 2018, 45, 418–425 CrossRef CAS
.
- W. Liang, A. Yu, G. Wang, F. Zheng, J. Jia and H. Xu, Chitosan-based nanoparticles of avermectin to control pine wood nematodes, Int. J. Biol. Macromol., 2018, 112, 258–263 CrossRef CAS PubMed
.
- M. Li, Z. Ma, M. Peng, L. Li, M. Yin, S. Yan and J. Shen, A gene and drug co-delivery application helps to solve the short life disadvantage of RNA drug, Nano Today, 2022, 43, 101452 CrossRef CAS
.
- L. A. Avila, R. Chandrasekar, K. E. Wilkinson, J. Balthazor, M. Heerman, J. Bechard, S. Brown, Y. Park, S. Dhar and G. R. Reeck, Delivery of lethal dsRNAs in insect diets by branched amphiphilic peptide capsules, J. Controlled Release, 2018, 273, 139–146 CrossRef CAS
.
- Y. Zheng, Y. Hu, S. Yan, H. Zhou, D. Song, M. Yin and J. Shen, A polymer/detergent formulation improves dsRNA penetration through the body wall and RNAi-induced mortality in the soybean aphid Aphis glycines, Pest Manage. Sci., 2019, 75, 1993–1999 CrossRef CAS PubMed
.
- N. Mitter, E. A. Worrall, K. E. Robinson, P. Li, R. G. Jain, C. Taochy, S. J. Fletcher, B. J. Carroll, G. Q. Lu and Z. P. Xu, Clay nanosheets for topical delivery of RNAi for
sustained protection against plant viruses, Nat. Plants, 2017, 3, 16207 CrossRef CAS PubMed
.
- A. Taban, M. J. Saharkhiz and M. Khorram, Formulation and assessment of nano-encapsulated bioherbicides based on biopolymers and essential oil, Ind. Crops Prod., 2020, 149, 112348–112348 CrossRef CAS
.
- Y. M. Hafez, K. A. Attia, S. Kamel, S. F. Alamery and K. Abdelaal,
Bacillus subtilis as a bio-agent combined with nano molecules can control powdery mildew disease through histochemical and physiobiochemical changes in cucumber plants, Physiol. Mol. Plant Pathol., 2020, 111, 101489 CrossRef CAS
.
- S. Dhiman, S. Singh, A. Varma and A. Goel, Phytofabricated zinc oxide nanoparticles as a nanofungicide for management of Alternaria blight of Brassica, BioMetals, 2021, 34, 1275–1293 CrossRef CAS PubMed
.
- S. K. R. Namasivayam, B. Tony, R. S. A. Bharani and F. R. Raj, Herbicidal activity of soil isolate of Fusarium oxysporum free and chitosan nanoparticles coated metabolites against economic important weedninidam theenjan, Asian J. Microbiol., Biotechnol. Environ. Sci., 2015, 17, 1015–1020 Search PubMed
.
- N. J. Zainuddin, S. E. Ashari, N. Salim, N. Asib, D. Omar and G. E. C. Lian, Optimization and characterization of palm oil-based nanoemulsion loaded with Parthenium hysterophorus crude extract for natural herbicide formulation, J. Oleo Sci., 2019, 68, 747–757 CrossRef CAS PubMed
.
- P. Olivija, G. Sašo and F. Z. Lidija, Mesoporous silica nanoparticles modified with N-rich polymer as a potentially environmentally-friendly delivery system for pesticides, Microporous Mesoporous Mater., 2021, 310, 110663 CrossRef
.
- A. Elabasy, A. Shoaib, M. Waqas, M. Jiang and Z. Shi, Synthesis, characterization, and pesticidal activity of emamectin benzoate nanoformulations against Phenacoccus solenopsis tinsley (hemiptera: Pseudococcidae), Molecules, 2019, 24, 2801 CrossRef PubMed
.
- F. Liu, L. X. Wen, Z. Z. Li, W. Yu, H. Y. Sun and J. F. Chen, Porous hollow silica nanoparticles as controlled delivery system for water-soluble pesticide, Mater. Res. Bull., 2006, 41, 2268–2275 CrossRef CAS
.
- K. Qian, T. Shi, T. Tang, S. Zhang, X. Liu and Y. Cao, Preparation and characterization of nano-sized calcium carbonate as controlled release pesticide carrier for validamycin against Rhizoctonia solani, Microchim. Acta, 2011, 173, 51–57 CrossRef CAS
.
- G. Ding, D. Li, Y. Liu, M. Guo, Y. Duan, J. Li and Y. Cao, Preparation and characterization of kasuga-silica-conjugated nanospheres for sustained antimicrobial activity, J. Nanopart. Res., 2014, 16, 2671 CrossRef
.
- X. Pan, Z. Xu, Y. Zheng, T. Huang, L. Li, Z. Chen, W. Rao, S. Chen, X. Hong and X. Guan, The adsorption features between insecticidal crystal protein and nano-Mg(OH)2, R. Soc. Open Sci., 2017, 4, 170883 CrossRef PubMed
.
- M. Shahab and J. Elham, Noble UV protective agent for Bacillus thuringiensis based on a combination of graphene oxide and olive oil, Sci. Rep., 2017, 7, 11019 CrossRef
.
- Y. Zhang, A. Zhang, M. Li, K. He and S. Guo, Nanoparticle-loaded microcapsules providing effective UV protection for Cry1Ac, J. Microencapsulation, 2021, 38, 522–532 CrossRef CAS PubMed
.
- M. Madliger, C. A. Gasser, R. P. Schwarzenbach and M. Sander, Adsorption of transgenic insecticidal Cry1Ab protein to silica particles. Effects on transport and bioactivity, Environ. Sci. Technol., 2011, 45, 4377–4384 CrossRef CAS PubMed
.
- S. Michael, M. Michael and R. P. Schwarzenbach, Adsorption of transgenic insecticidal Cry1Ab protein to SiO2. 1. Forces driving adsorption, Environ. Sci. Technol., 2010, 44, 8870–8876 CrossRef PubMed
.
- N. Helassa, H. Quiquampoix, S. Noinville, W. Szponarski and S. Staunton, Adsorption and desorption of monomeric Bt (Bacillus thuringiensis) Cry1Aa toxin on montmorillonite and kaolinite, Soil Biol. Biochem., 2009, 41, 498–504 CrossRef CAS
.
- N. Helassa, M. Revault, H. Quiquampoix, P. Déjardin, S. Staunton and S. Noinville, Adsorption on montmorillonite prevents oligomerization of Bt Cry1Aa toxin, J. Colloid Interface Sci., 2011, 356, 718–725 CrossRef CAS PubMed
.
- Z. Hajiahmadi, R. Shirzadian-Khorramabad, M. Kazemzad, M. M. Sohani and J. Khajehali, A novel, simple, and stable mesoporous silica nanoparticle-based gene transformation approach in Solanum lycopersicum, 3 Biotech, 2020, 10, 370 CrossRef PubMed
.
- H. Iqbal, A. Fatima and H. A. A. Khan, ZnO nanoparticles produced in the culture supernatant of Bacillus thuringiensis ser. israelensis affect the demographic parameters of Musca domestica using the age-stage, two-sex life table, Pest Manage. Sci., 2022, 78, 1640–1648 CrossRef CAS PubMed
.
- S. Song, X. Jiang, H. Shen, W. Wu, Q. Shi, M. Wan, J. Zhang, H. Mo and J. Shen, MXene (Ti3C2) based pesticide delivery system for sustained release and enhanced pest control, ACS Appl. Bio Mater., 2021, 4, 6912–6923 CrossRef CAS PubMed
.
- J. Luo, D. X. Zhang, T. F. Jing, G. Liu, H. C. Cao, B. X. Li, Y. M. Hou and F. Liu, Pyraclostrobin loaded lignin-modified nanocapsules: Delivery efficiency enhancement in soil improved control efficacy on tomato Fusarium crown and root rot, Chem. Eng. J., 2020, 394, 124854 CrossRef CAS
.
- Y. Li, M. Zhou, Y. Pang and X. Qiu, Lignin-based microsphere: preparation and performance on encapsulating the pesticide avermectin, ACS Sustainable Chem. Eng., 2017, 5, 3321–3328 CrossRef CAS
.
- Y. Liang, C. Fan, H. Dong, W. Zhang, G. Tang, J. Yang, N. Jiang and Y. Cao, Preparation of MSNs-Chitosan@Prochloraz nanoparticles for reducing toxicity and improving release properties of prochloraz, ACS Sustainable Chem. Eng., 2018, 6, 10211–10220 CrossRef CAS
.
- W. Guan, W. Zhang, L. Tang, Y. Wang and H. Cui, Fabrication of novel avermectin nanoemulsion using a polyurethane emulsifier with cleavable disulfide bonds, J. Agric. Food Chem., 2017, 66, 6569–6577 CrossRef PubMed
.
- W. Liang, A. Yu, G. Wang, F. Zheng, J. Jia and H. Xu, Chitosan-based nanoparticles of avermectin to control pine wood nematodes, Int. J. Biol. Macromol., 2018, 112, 258–263 CrossRef CAS PubMed
.
-
S. Guleria and A. K. Tiku, in Integrated Pest Management: Innovation-Development Process, ed. R. Peshin and A. K. Dhawan, Springer Netherlands, Dordrecht, 2009, pp. 317–329, DOI:10.1007/978-1-4020-8992-3_12
.
- K. Jorgensen, A. V. Rasmussen, M. Morant, A. H. Nielsen, N. Bjarnholt, M. Zagrobelny, S. Bak and B. L. Moller, Metabolon formation and metabolic channeling in the biosynthesis of plant natural products, Curr. Opin. Plant Biol., 2005, 8, 280–291 CrossRef CAS PubMed
.
- J. L. de Oliveira, E. V. R. Campos and L. F. Fraceto, Recent developments and challenges for nanoscale formulation of botanical pesticides for use in sustainable agriculture, J. Agric. Food Chem., 2018, 66, 8898–8913 CrossRef PubMed
.
- Y. Wu, D. Ren, C. Gao, J. Li, B. Du, Z. Wang and S. Qian, Recent advances for alkaloids as botanical pesticides for use in organic agriculture, Int. J. Pest Manage., 2021, 1–11, DOI:10.1080/09670874.2021.1917723
.
- J. L. de Oliveira, E. V. R. Campos, M. Bakshi, P. C. Abhilash and L. F. Fraceto, Application of nanotechnology for the encapsulation of botanical insecticides for sustainable agriculture: Prospects and promises, Biotechnol. Adv., 2014, 32, 1550–1561 CrossRef CAS PubMed
.
- O. Campolo, G. Giunti, M. Laigle, T. Michel and V. Palmeri, Essential oil-based nano-emulsions: effect of different surfactants, sonication and plant species on physicochemical characteristics, Ind. Crops Prod., 2020, 157, 8 CrossRef
.
- L. Pavoni, R. Pavela, M. Cespi, G. Bonacucina, F. Maggi, V. Zeni, A. Canale, A. Lucchi, F. Bruschi and G. Benelli, Green micro- and nanoemulsions for managing parasites, vectors and pests, Nanomaterials, 2019, 9, 1285 CrossRef CAS PubMed
.
- M. J. Pascual-Villalobos, M. Canto-Tejero, R. Vallejo, P. Guirao, S. Rodriguez-Rojo and M. J. Cocero, Use of nanoemulsions of plant essential oils as aphid repellents, Ind. Crops Prod., 2017, 110, 45–57 CrossRef CAS
.
- S. M. M. Mohafrash, S. A. Fallatah, S. M. Farag and A. T. H. Mossa,
Mentha spicata essential oil nanoformulation and its larvicidal application against Culex pipiens and Musca domestica, Ind. Crops Prod., 2020, 157, 112944 CrossRef CAS
.
- M. Pant, S. Dubey, P. K. Patanjali, S. N. Naik and S. Sharma, Insecticidal activity of eucalyptus oil nanoemulsion with karanja and jatropha aqueous filtrates, Int. Biodeterior. Biodegrad., 2014, 91, 119–127 CrossRef CAS
.
- N. Iqbal, D. K. Hazra, A. Purkait, A. Agrawal and J. Kumar, Bioengineering of neem nano-formulation with adjuvant for better adhesion over applied surface to give long term insect control, Colloids Surf., B, 2022, 209, 112176 CrossRef CAS PubMed
.
-
S. Wuryantini and Harwanto, The prospect of nanoinsecticide in controlling citrus aphids Aphis gossypii, International Conference and the 10th Congress of the Entomological Society of Indonesia (ICCESI 2019), 2020 Search PubMed.
- P. L. Soujanya, J. C. Sekhar, P. Kumar, N. Sunil, C. V. Prasad and U. V. Mallavadhani, Potentiality of botanical agents for the management of post harvest insects of maize: a review, J. Food. Sci. Technol., 2016, 53, 2169–2184 CrossRef CAS PubMed
.
- S. Kala, N. Sogan, S. N. Naik, A. Agarwal and J. Kumar, Impregnation of pectin-cedarwood essential oil nanocapsules onto mini cotton bag improves larvicidal performances, Sci. Rep., 2020, 10, 14107 CrossRef CAS PubMed
.
- A. M. Bakry, S. Abbas, B. Ali, H. Majeed, M. Y. Abouelwafa, A. Mousa and L. Liang, Microencapsulation of oils: A comprehensive review of benefits, techniques, and applications, Compr. Rev. Food Sci. Food Saf., 2016, 15, 143–182 CrossRef CAS PubMed
.
- J. O. Werdin González, M. M. Gutiérrez, A. A. Ferrero and B. Fernández Band, Essential oils nanoformulations for stored-product pest control – Characterization and biological properties, Chemosphere, 2014, 100, 130–138 CrossRef PubMed
.
- J. L. de Oliveira, E. V. R. Campos, T. Germano-Costa, R. Lima, J. F. Della Vechia, S. T. Soares, D. J. de Andrade, K. C. Goncalves, J. Do Nascimento, R. A. Polanczyk and L. F. Fraceto, Association of zein nanoparticles with botanical compounds for effective pest control systems, Pest Manage. Sci., 2019, 75, 1855–1865 CrossRef CAS PubMed
.
- F. J. Francisco, C. Maria, G. Carlos, V. Inmaculada and C. M. Jesus, Proteomic advances in phytopathogenic fungi, Curr. Proteomics, 2007, 4, 79–88 CrossRef
.
- M. A. Alghuthaymia, H. Almoammarb, M. Raic, E. Said-Galievd and K. A. Abd-Elsalame, Myconanoparticles: synthesis and their role in phytopathogens management, Biotechnol. Biotechnol. Equip., 2015, 29, 221–236 CrossRef PubMed
.
- J. Feng, Z. Chen, W. Chen, L. Sun, J. Yang, K. He, S. Dong and S. Yuan, Facile pathway to construct mesoporous silica nanoparticles loaded with pyraclostrobin: Physicochemical properties, antifungal activity, and biosafety, Pest Manage. Sci., 2022, 78, 2332–2341 CrossRef CAS PubMed
.
- X. Zhang, X. Tang, C. Zhao, Z. Yuan, D. Zhang, H. Zhao, N. Yang, K. Guo, Y. He, Y. He, J. Hu, L. He, L. He and K. Qian, A pH-responsive MOF for site-specific delivery of fungicide to control citrus disease of Botrytis cinerea, Chem. Eng. J., 2022, 431, 133351 CrossRef CAS
.
-
A. Bhattacharyya, P. Duraisamy, M. Govindarajan, A. A. Buhroo and R. Prasad, Nano-Biofungicides: Emerging Trend in Insect Pest Control, Advances and Applications Through Fungal Nanobiotechnology, 2016, pp. 307–319 Search PubMed
.
- F. Peng, X. Wang, W. Zhang, X. Shi, C. Cheng, W. Hou, X. Lin, X. Xiao and J. Li, Nanopesticide formulation from pyraclostrobin and graphene oxide as a nanocarrier and application in controlling plant fungal pathogens, Nanomaterials, 2022, 12, 1112 CrossRef CAS PubMed
.
- Y. P. Shan, L. D. Cao, B. Muhammad, B. Xu, P. Y. Zhao, C. Cao and Q. L. Huang, Iron-based porous metal-organic frameworks with crop nutritional function as carriers for controlled fungicide rel ase, J. Colloid Interface Sci., 2020, 566, 383–393 CrossRef CAS PubMed
.
- K. A. Abd-Elsalam and M. A. Alghuthaymi, Nanobiofungicides: Are they the next-generation of fungicides?, J. Nanotechnol. Mater. Sci., 2015, 2, 1–3 Search PubMed
.
-
K. A. Abd-Elsalam, F. A. Al-Dhabaan, M. Alghuthaymi, P. B. Njobeh and H. Almoammar, in Nano-Biopesticides today and future perspectives, ed. O. Koul, Academic Press, 2019, pp. 315–351, DOI:10.1016/B978-0-12-815829-6.00014-0
.
- A. Singh, N. B. Singh, I. Hussain, H. Singh and S. C. Singh, Plant-nanoparticle interaction: An approach to improve agricultural practices and plant productivity, Int. J. Pharm. Sci. Invent., 2015, 4, 25–40 CAS
.
- S. S. Salem and A. Fouda, Green synthesis of metallic nanoparticles and their prospective biotechnological applications: an overview, Biol. Trace Elem. Res., 2020, 199, 1–27 Search PubMed
.
- H. Bahrulolum, S. Nooraei, N. Javanshir, H. Tarrahimofrad and G. Ahmadian, Green synthesis of metal nanoparticles using microorganisms and their application in the agrifood sector, J. Nanobiotechnol., 2021, 19, 86 CrossRef PubMed
.
- P. Singh, Y.-J. Kim, D. Zhang and D.-C. Yang, Biological synthesis of nanoparticles from plants and microorganisms, Trends Biotechnol., 2016, 34, 588–599 CrossRef CAS PubMed
.
- A. A. E. A. Abeer and M. Z. Moustafa, Green-synthesis and optimization of an eco-friendly nanobiofungicide from Bacillus amyloliquefaciens MH046937 with antimicrobial potential against phytopathogens, Environ. Nanotechnol., Monit. Manage., 2020, 14, 100309 Search PubMed
.
- M. Atienza, M. Magpantay, K. Santos, N. B. Mora, R. P. Balaraman, M. E. Gemeinhardt, F. Cueva, E. S. Paterno, L. M. Fernando and P. Kohli, Encapsulation of plant growth-promoting bacterial crude extract in nanoliposome and its antifungal property against Fusarium oxysporum, ACS Agric. Sci. Technol., 2021, 1, 691–701 CrossRef CAS
.
- P. A. Arciniegas-Grijalba, M. C. Patino-Portela, L. P. Mosquera-Sanchez, B. G. Sierra, J. E. Munoz-Florez, L. A. Erazo-Castillo and J. E. Rodriguez-Paez, ZnO-based nanofungicides: synthesis, characterization and their effect on the coffee fungi Mycena citricolor and Colletotrichum sp, Mater. Sci. Eng., C, 2019, 98, 808–825 CrossRef CAS PubMed
.
- Y. Zhou, S. Jiang, Y. Jiao and H. Wang, Synergistic effects of nanochitin on inhibition of tobacco root rot disease, Int. J. Biol. Macromol., 2017, 99, 205–212 CrossRef CAS PubMed
.
- V. Saharan, R. V. Kumaraswamy, R. C. Choudhary, S. Kumari, A. Pal, R. Raliya and P. Biswas, Cu-chitosan nanoparticle mediated sustainable approach to enhance seedling growth in maize by mobilizing reserved food, J. Agric. Food Chem., 2016, 64, 6148–6155 CrossRef CAS PubMed
.
- S. H. S. Dananjaya, W. K. C. U. Erandani, C.-H. Kim, C. Nikapitiya, J. Lee and M. D. Zoysa, Comparative study on antifungal activities of chitosan nanoparticles and chitosan silver nano composites against Fusarium oxysporum species complex, Int. J. Biol. Macromol., 2017, 105, 478–488 CrossRef CAS PubMed
.
- K. Xing, Y. Liu, X. Shen, X. Zhu, X. Li, X. Miao, Z. Feng, X. Peng and S. Qin, Effect of o-chitosan nanoparticles on the development and membrane permeability of Verticillium dahliae, Carbohydr. Polym., 2017, 165, 334–343 CrossRef CAS PubMed
.
- J. Cui, C. Sun, A. Wang, Y. Wang, H. Zhu, Y. Shen, N. Li, X. Zhao, B. Cui, C. Wang, F. Gao, Z. Zeng and H. Cui, Dual-functionalized pesticide nanocapsule delivery system with improved spreading behavior and enhanced bioactivity, Nanomaterials, 2020, 10, 220 CrossRef CAS PubMed
.
- W. Liang, J. Cheng, J. Zhang, Q. Xiong, M. Jin and J. Zhao, pH-Responsive on-demand alkaloids release from core-shell ZnO@ZIF-8 nanosphere for synergistic control of bacterial wilt disease, ACS Nano, 2022, 16, 2762–2773 CrossRef CAS PubMed
.
- F. V. Leimann, O. H. Goncalves, R. A. F. Machado and A. Bolzan, Antimicrobial activity of microencapsulated lemongrass essential oil and the effect of experimental parameters on microcapsules size and morphology, Mater. Sci. Eng., C, 2009, 29, 430–436 CrossRef CAS
.
- W. Du, S. Niu, Y. Xu, Z. Xu and C. Fan, Antibacterial activity of chitosan tripolyphosphate nanoparticles loaded with various metal ions, Carbohydr. Polym., 2009, 75, 385–389 CrossRef CAS
.
- X. Ma, X. Zhu, S. Qu, L. Cai, G. Ma, G. Fan and X. Sun, Fabrication of copper nanoparticle composite nanogel for high-efficiency management of Pseudomonas syringae pv. tabaci on tobacco, Pest Manage. Sci., 2022, 78, 2074–2085 CrossRef CAS PubMed
.
- S. Kumar, M. Nehra, N. Dilbaghi, G. Marrazza, A. A. Hassan and K.-H. Kim, Nano-based smart pesticide formulations: Emerging opportunities for agriculture, J. Controlled Release, 2019, 294, 131–153 CrossRef CAS PubMed
.
- I. S. Abd Alamer, A. A. Tomah, T. Ahmed, B. Li and J. Zhang, Biosynthesis of silver chloride nanoparticles by rhizospheric bacteria and their antibacterial activity against phytopathogenic bacterium Ralstonia solanacearum, Molecules, 2022, 27, 224 CrossRef CAS PubMed
.
- H. Zhang, S. Chen, X. Jia, Y. Huang, R. Ji and L. Zhao, Comparation of the phytotoxicity between chemically and green synthesized silver nanoparticles, Sci. Total Environ., 2021, 752, 142264 CrossRef CAS PubMed
.
- J. H. Graham, E. G. Johnson, M. E. Myers, M. Young, P. Rajasekaran, S. Das and S. Santra, Potential of nano-formulated zinc oxide for control of Citrus canker on grapefruit trees, Plant Dis., 2016, 100, 2442–2447 CrossRef CAS PubMed
.
- D. Laha, A. Pramanik, A. Laskar, M. Jana, P. Pramanik and P. Karmakar, Shape-dependent bactericidal activity of copper oxide nanoparticle mediated by DNA and membrane damage, Mater. Res. Bull., 2014, 59, 185–191 CrossRef CAS
.
- T. Ahmed, M. Noman, M. Shahid, M. S. Shahid and B. Li, Antibacterial potential of green magnesium oxide nanoparticles against rice pathogen Acidovorax oryzae, Mater. Lett., 2021, 282, 128839 CrossRef CAS
.
- A. Presotto, F. Hernández, M. Díaz, I. Fernández-Moroni, C. Pandolfo, J. Basualdo, S. Cuppari, M. Cantamutto and M. Poverene, Crop-wild sunflower hybridization can mediate weediness throughout growth-stress tolerance trade-offs, Agric., Ecosyst. Environ., 2017, 249, 12–21 CrossRef
.
- R. Radhakrishnan, A. A. Alqarawi and E. F. Abd_Allah, Bioherbicides: Current knowledge on weed control mechanism, Ecotoxicol. Environ. Saf., 2018, 158, 131–138 CrossRef CAS PubMed
.
- M. Hasan, M. S. AhmadHamdani, M. Rosli Adam and H. Hamdan, Bioherbicides: an eco-friendly tool for sustainable weed management, Plants, 2021, 10, 1212 CrossRef CAS PubMed
.
- T. Raza, Y. K. Muhammad, M. N. Sajid, I. Shakeel, N. Q. Kashif, N. M. Muhammad, S. Muhammad, S. Achim and S. E. Neal, Biological management of selected weeds of wheat through co-application of allelopathic rhizobacteria and sorghum extract, Biol. Control, 2021, 164, 104775 CrossRef CAS
.
- S. R. Pratap, H. Rahul and M. Geetanjali, Nanoparticles in sustainable agriculture: an emerging opportunity, J. Controlled Release, 2020, 329, 1234–1248 Search PubMed
.
- C. F. OkeyOnyesolu, M. Hassanisaadi, M. Bilal, M. Barani, A. Rahdar, J. Iqbal and G. Z. Kyzas, Nanomaterials as nanofertilizers and nanopesticides: an overview, ChemistrySelect, 2021, 6, 8645–8663 CrossRef CAS
.
- Y. Xiang, G. Zhang, Y. Chi, D. Cai and Z. Wu, Fabrication of a controllable nanopesticide system with magnetic collectability, Chem. Eng. J., 2017, 328, 320–330 CrossRef CAS
.
- Y. Chi, G. Zhang, Y. Xiang, D. Cai and Z. Wu, Fabrication of a temperature-controlled-release herbicide using a nanocomposite, ACS Sustainable Chem. Eng., 2017, 5, 4969–4975 CrossRef CAS
.
-
K. L. Bailey, in Integrated Pest Management, ed. D. P. Abrol, Academic Press, San Diego, 2014, pp. 245–266, DOI:10.1016/B978-0-12-398529-3.00014-2
.
- D. Karl-Josef, M. Ron and N. Graham, Recent progress in understanding the role of reactive oxygen species in plant cell signaling, Plant Physiol., 2016, 171, 1535–1539 CrossRef PubMed
.
- J. M. Matthew, I. E. Popova and R. A. Boydston, Bioherbicidal activity of Sinapis alba seed meal extracts, Ind. Crops Prod., 2018, 115, 174–181 CrossRef
.
- U. Aqeela, T. Aftab, M. M. A. Khan, M. Naeem and M. N. Khan, A comprehensive review of impacts of diverse nanoparticles on growth, development and physiological adjustments in plants under changing environment, Chemosphere, 2021, 291, 132672 CrossRef PubMed
.
- S. Yan, B. Ren and J. Shen, Nanoparticle-mediated double-stranded RNA delivery system: a promising approach for sustainable pest management, Insect Sci., 2020, 28, 21–34 CrossRef PubMed
.
- S. Yan, B. Ren, B. Zeng and J. Shen, Improving RNAi efficiency for pest control in crop species, BioTechniques, 2020, 68, 283–290 CrossRef CAS PubMed
.
- J. W. Wang, E. G. Grandio, G. M. Newkirk, G. S. Demirer, S. Butrus, J. P. Giraldo and M. P. Landry, Nanoparticle-mediated genetic engineering of plants, Mol. Plant, 2019, 12, 1037–1040 CrossRef CAS PubMed
.
- C. Olivier, M. G. Tardajos, R. Z. L. Martinez, D. Mamoni, D. Peter and S. Guy, Increased RNAi efficacy in Spodoptera exigua via the formulation of dsRNA with guanylated polymers, Front. Physiol., 2018, 9, 316 Search PubMed
.
- J. Li, J. Qian, Y. Xu, S. Yan, J. Shen and M. Yin, A facile-synthesized star polycation constructed as a highly efficient gene vector in pest management, ACS Sustainable Chem. Eng., 2019, 7, 6316–6322 CrossRef CAS
.
- M. Kah, Nanopesticides and nanofertilizers: emerging contaminants or opportunities for risk mitigation?, Front. Chem., 2015, 3, 64 Search PubMed
.
- Z. Xu, B. He, W. Wei, K. Liu, M. Yin, W. Yang and J. Shen, Highly water-soluble perylenediimide-cored poly(amido amine) vector for efficient gene transfection, J. Mater. Chem. B, 2014, 2, 3079–3086 RSC
.
- N. Kunte, E. McGraw, S. Bell, D. Held and L.-A. Avila, Prospects, challenges and current status of RNAi through insect feeding, Pest Manage. Sci., 2020, 76, 26–41 CrossRef CAS PubMed
.
- M. Yu, J. Yao, J. Liang, Z. Zeng, B. Cui, X. Zhao, C. Sun, Y. Wang, G. Liu and H. Cui, Development of functionalized abamectin poly(lactic acid) nanoparticles with regulatable adhesion to enhance foliar retention, RSC Adv., 2017, 7, 11271–11280 RSC
.
- S. Huang, L. Wang, L. Liu, Y. Hou and L. Li, Nanotechnology in agriculture, livestock, and aquaculture in China. A review, Agron. Sustainable Dev., 2015, 35, 369–400 CrossRef
.
- D.-Y. Kim, A. Kadam, S. Shinde, R. G. Saratale, J. Patra and G. Ghodake, Recent developments in nanotechnology transforming the agricultural sector: a transition replete with opportunities, J. Sci. Food Agric., 2018, 98, 849–864 CrossRef CAS PubMed
.
- L. F. Fraceto, R. E. Grillo, G. A. D. Medeiros, V. E. Scognamiglio, G. E. Rea and C. E. Bartolucci, Nanotechnology in agriculture: which innovation potential does it have?, Front. Environ. Sci., 2016, 4, 20 Search PubMed
.
- L. Cai, L. Cai, H. Jia, C. Liu, D. Wang and X. Sun, Foliar exposure of Fe3O4 nanoparticles on Nicotiana benthamiana: Evidence for nanoparticles uptake, plant growth promoter and defense response elicitor against plant virus, J. Hazard. Mater., 2020, 393, 122415 CrossRef CAS PubMed
.
- B. Aline, P. A. E. Santo, L. M. Garibotti, O. E. M. De, D. O. J. Luiz, C. E. V. Ramos, D. J. M. Bispo, O. H. Caixeta, F. L. Fernandes and M. J. L. Sampaio, A mechanistic view of interactions of a nanoherbicide with target organism, J. Agric. Food Chem., 2019, 67, 4453–4462 CrossRef PubMed
.
- L. Zhao, Q. Hu, Y. Huang, A. N. Fulton, B. C. Hannah, A. S. Adeleye and R. A. A. Kelle, Activation of antioxidant and detoxification gene expression in cucumber plants exposed to a Cu(OH)2 nanopesticide, Environ. Sci.: Nano, 2017, 4, 1750–1760 RSC
.
- A. Valletta, L. Chronopoulou, C. Palocci, B. Baldan and G. Pasqua, Poly(lactic-co-glycolic) acid nanoparticles uptake by Vitis vinifera and grapevine-pathogenic fungi, J. Nanopart. Res., 2014, 16, 1–14 CrossRef CAS
.
- M. D. Stamm, T. M. Heng-Moss, F. P. Baxendale, B. D. Siegfried, E. E. Blankenship and R. Nauen, Uptake and translocation of imidacloprid, clothianidin and flupyradifurone in seed-treated soybeans, Pest Manage. Sci., 2016, 72, 1099–1109 CrossRef CAS PubMed
.
- L. Zhao, J. R. Peralta-Videa, M. Ren, A. Varela-Ramirez, C. Li, J. A. Hernandez-Viezcas, R. J. Aguilera and J. L. Gardea-Torresdey, Transport of Zn in a sandy loam soil treated with ZnO NPs and uptake by corn plants: Electron microprobe and confocal microscopy studies, Chem. Eng. J., 2012, 184, 1–8 CrossRef CAS
.
- J. R. Lead, G. E. Batley, P. J. J. Alvarez, M.-N. Croteau, R. D. Handy, M. J. McLaughlin, J. D. Judy and K. Schirmer, Nanomaterials in the environment: Behavior, fate, bioavailability, and effects-an updated review, Environ. Toxicol. Chem., 2018, 37, 2029–2063 CrossRef CAS PubMed
.
- R. Raliya, C. Franke, C. Franke, S. Chavalmane, R. Nair, N. Reed and P. Biswas, Quantitative understanding of nanoparticle uptake in watermelon plants, Front. Plant Sci., 2016, 7, 1288 Search PubMed
.
- F. Schwab, G. S. Zhai, M. Kern, A. Turner, J. L. Schnoor and M. R. Wiesner, Barriers, pathways and processes for uptake, translocation and accumulation of nanomaterials in plants--Critical
review, Nanotoxicology, 2016, 10, 257–278 CrossRef CAS PubMed
.
- H. Wu, P. Hu, Y. Xu, C. Xiao, Z. Chen, X. Liu, J. Jia and H. Xu, Phloem delivery of fludioxonil by plant amino acid transporter-mediated polysuccinimide nanocarriers for controlling fusarium wilt in banana, J. Agric. Food Chem., 2021, 69, 2668–2678 CrossRef CAS PubMed
.
- J. J. Liang, J. X. Yong, Z. Li, Z. Z. Xiang, L. W. Long, W. G. Dong, Z. Feng, W. X. Zhou and X. H. Hong, Enhanced intracellular uptake in vitro by glucose-functionalized nanopesticides, New J. Chem., 2017, 41, 11398–11404 RSC
.
-
J. Jampílek and K. Kráľová, in Nano-Biopesticides Today and Future Perspectives, ed. O. Koul, Academic Press, 2019, pp. 397–447, DOI:10.1016/B978-0-12-815829-6.00018-8
.
- M. Kah, N. Tufenkji and J. C. White, Nano-enabled strategies to enhance crop nutrition and protection, Nat. Nanotechnol., 2019, 14, 532–540 CrossRef CAS PubMed
.
- R. J. B. Peters, H. Bouwmeester, S. Gottardo, V. Amenta, M. Arena, P. Brandhoff, H. J. P. Marvin, A. Mech, F. B. Moniz, L. Q. Pesudo, H. Rauscher, R. Schoonjans, A. K. Undas, M. V. Vettori, S. Weigel and K. Aschberger, Nanomaterials for products and application in agriculture, feed and food, Trends Food Sci. Technol., 2016, 54, 155–164 CrossRef CAS
.
- M. B. Isman, S. Miresmailli and C. Machial, Commercial opportunities for pesticides based on plant essential oils in agriculture, industry and consumer products, Phytochem. Rev., 2011, 10, 197–204 CrossRef CAS
.
- M. H. Jang, W. K. Kim, S. K. Lee, T. B. Henry and J. W. Park, Uptake, tissue distribution, and depuration of total silver in common carp (Cyprinus carpio) after aqueous exposure to silver nanoparticles, Environ. Sci. Technol., 2014, 48, 11568–11574 CrossRef CAS PubMed
.
- C. J. Smith, B. J. Shaw and R. D. Handy, Toxicity of single walled carbon nanotubes to rainbow trout, (Oncorhynchus mykiss): respiratory toxicity, organ pathologies, and other physiological effects, Aquat. Toxicol., 2007, 82, 94–109 CrossRef CAS PubMed
.
- J. Feng, Y. Ma, Z. Chen, Q. Liu, J. Yang, Y. Gao, W. Chen, K. Qian and W. Yang, Development and characterization of pyriproxyfen-loaded nanoemulsion for housefly control: Improving activity, reducing toxicity, and protecting ecological environment, ACS Sustainable Chem. Eng., 2021, 9, 4988–4999 CrossRef CAS
.
- D. X. Zhang, J. Du, R. Wang, J. Luo, T. F. Jing, B. X. Li, W. Mu, F. Liu and Y. M. Hou, Core/shell dual-responsive nanocarriers via iron-mineralized electrostatic self-assembly for precise pesticide delivery, Adv. Funct. Mater., 2021, 31, 21020271 Search PubMed
.
-
P. Ganguli, in Nano-Biopesticides Today and Future Perspectives, 2019, pp. 367–395 Search PubMed
.
- R. Nair, S. H. Varghese, B. G. Nair, T. Maekawa and D. S. Kumar, Nanoparticulate material delivery to plants, Plant Sci., 2010, 179, 154–163 CrossRef CAS
.
- S. Lin, J. Reppert, H. Qian, J. Hudson and C. K. Pu, Uptake, translocation, and transmission of carbon nanomaterials in rice plants, Small, 2009, 5, 1128–1132 CrossRef CAS PubMed
.
- A. A. Haytham, K. Mohamed, E. Salaheldeen, A. R. Farouk and F. A.-H. Hanan, Pesticidal activity of nanostructured metal oxides for generation of alternative pesticide formulations, J. Agric. Food Chem., 2018, 66, 5491–5498 CrossRef PubMed
.
- D. Wibowo, C. X. Zhao, B. C. Peters and A. P. J. Middelberg, Sustained release of fipronil insecticide in vitro and in vivo from biocompatible silica nanocapsules, J. Agric. Food Chem., 2014, 62, 12504–12511 CrossRef CAS PubMed
.
- M. Ao, Y. Zhu, S. He, D. Li, P. Li, J. Li and Y. Cao, Preparation and characterization of 1-naphthylacetic acid-silica conjugated nanospheres for enhancement of controlled-release performance, Nanotechnology, 2013, 24, 035601 CrossRef PubMed
.
- J. A. Baum, T. Bogaert, W. Clinton, G. R. Heck, P. Feldmann, O. Ilagan, S. Johnson, G. Plaetinck, T. Munyikwa and M. Pleau, Control of coleopteran insect pests through RNA interference, Nat. Biotechnol., 2007, 25, 1322–1326 CrossRef CAS PubMed
.
|
This journal is © The Royal Society of Chemistry 2023 |
Click here to see how this site uses Cookies. View our privacy policy here.