Ecotoxicity risk assessment of amines used in ‘switchable water’ and CO2-capturing processes†
Received
29th October 2024
, Accepted 24th February 2025
First published on 25th February 2025
Abstract
Utilizing amines and alkanolamines as CO2-capturing agents and water-soluble ionogens in ‘switchable water’ systems is an intensively explored research area. However, the potential risks of such amine derivatives to the environment have been poorly evaluated. In this work, we report on the ecotoxicological effect of relevant amines and alkanolamines in an aqueous environment on various classes of organisms such as bacteria (Aliivibrio fischeri), vascular plants (Spirodela polyrhiza), and invertebrates (Daphnia magna). The measured half maximal effective concentration (EC50) data indicate that all tested alkanolamines and most amines have EC50 values over 100 mg L−1 and can be classified as practically harmless or harmless. On the other hand, tetramethyl-1,3-propane diamine afforded EC50 values between 61 and 73 mg L−1, indicating moderate toxicity towards invertebrates and vascular plants. Moreover, we observed a good agreement between the experimental results and the ECOSAR predictive model. Thus, our work indicates that hydrophilic amines and alkanolamines utilized in emerging CO2-mediated processes can generally be considered harmless or practically harmless in an aqueous environment towards bacteria, vascular plants, and invertebrates, except more lipophilic diamines, which may need careful consideration.
Environmental significance
Many CO2-capturing and ‘switchable water’ processes rely on amines' ability to absorb CO2 gas. These processes are an essential part of the more sustainable chemical and energy sector, but the involvement of large amounts of amines may pose risks to aquatic and terrestrial ecosystems. The work provides data on the ecotoxic effects on various classes of organisms, such as bacteria (Aliivibrio fischeri), vascular plants (Spirodela polyrhiza), and invertebrates (Daphnia magna). Our risk assessment enables the design of safer chemicals and processes, minimizes long-term environmental harm, and supports regulatory compliance, ensuring that amine-mediated emerging technologies contribute to both emission control and ecosystem protection.
|
Introduction
The need for green transition has sparked wide interest in utilizing amines as CO2-capturing (CC) agents and, more recently, also as water-soluble ionogens in switchable water (SW) systems. The aim of these technologies is to capture gaseous CO2 and, in the case of the SW process, to reduce the energy requirements associated with the separation of organic products from water. Therefore, they are crucial in moving towards more sustainable chemical processes.
In both processes, a key intermediate is a soluble bicarbonate salt, which forms due to an acid–base reaction between hydrated CO2 and an amine (Fig. 1).1
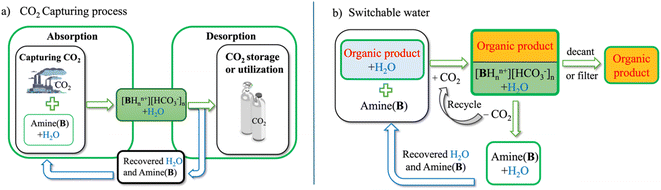 |
| Fig. 1 Schematic illustration of using amines in the (a) CO2 capture and (b) switchable water (SW) processes. | |
In the case of amine-mediated CO2 capturing technology (Fig. 1a), gaseous CO2 is first absorbed into an aqueous amine solution, where CO2 reacts with the amines to form carbamates or bicarbonates, depending on the type of amine (primary, secondary, or tertiary). The CO2 can be released upon heating, and the water and amines can be subsequently recovered and reused in the process.2–5 It is a well-established process that offers operational convenience, rapid and high-capacity CO2 absorption, and recyclability.
On the other hand, the SW process facilitates the removal of water-soluble organic compounds (e.g., EtOH, etc.) from the water. This is particularly relevant in emerging chemical production processes from biomass, where the conventional separation techniques, such as distillation, have high energy requirements and can thus negate the environmental benefits of biomass conversion.6 In this process, an amine is added to the mixture of water and an organic product.7–13 Upon subsequent introduction of CO2 gas into the system, the amine reacts to form its bicarbonate salt (Fig. 1b), inducing the precipitation of the organic product if it is a solid or facilitating its separation in the form of an “organic-rich” liquid phase if it is a liquid. After separating the organic component, the aqueous phase can be decarbonated, and the removed CO2 can be re-used. Decarbonation reverses the reaction, converting the amine back to its neutral state. Finally, the amine can be recovered from the decarbonated water via reverse osmosis or by filtration and reused for another cycle.
The development of any new technology requires a critical assessment of its potential environmental impact. Hence, the environmental assessment of amines, particularly those used in emerging technologies, is imperative due to their potential environmental exposure and biological effects. Although the amines used in these processes are recycled, the unintended leaks into the environment cannot be excluded. Moreover, such an assessment during the early stages of development identifies the hotspots directly and guides the process development towards lower risks.
Studies on the ecotoxicity of amines have primarily focused only on a few alkanolamines, such as monoethanolamine (MEA), diethanolamine (DEA), and triethanolamine (TEA; for structures, see Table 1).14–19 Only limited and divergent information is available, mainly for some decomposer, producer, and first-level consumer organisms. These amines have been evaluated previously toward single-celled organisms (Entosiphon sulcatum and Chilomonas paramecium), bacterium (Vibrio fischeri), invertebrate (Daphnia magna) and alga (Skeletonema costatum).17,19,20 Generally, the findings from these studies suggest a low toxicity level of alkanolamines towards most tested species. On the other hand, amines developed more recently, especially for the SW process, have not been evaluated. Moreover, the potentially harmful effects of amine emissions, such as the formation of nitrosamines and nitramines via photooxidation in the atmosphere, which could harm human health and the environment, have been observed.21 Hence, there is a gap in the knowledge about the toxicity data of amines employed more recently in SW or CC processes. It is also generally known that even minor modifications in chemical structure can lead to substantial changes in biological activity and environmental fate.22 The lack of comprehensive data on the environmental toxicity of many of these compounds motivates us to carry out wider ecotoxicological testing.
Table 1 List of compounds evaluated in this study
Entry |
Name/amine type |
Formula |
CAS no. |
MW (g mol−1) |
log Kowa |
Structure |
Data taken from QSAR prediction analysis (ECOSAR). |
1 |
Monoethanolamine (MEA)/primary |
C2H7NO |
141-43-5 |
61.08 |
−1.6 |
 |
2 |
Diethanolamine (DEA)/secondary |
C4H11NO2 |
111-42-2 |
105.14 |
−1.7 |
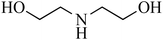 |
3 |
Triethanolamine (TEA)/tertiary |
C6H15NO3 |
102-71-6 |
149.19 |
−2.5 |
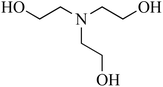 |
4 |
Dimethylethanolamine (DMEA)/tertiary |
C4H11NO |
108-01-0 |
89.14 |
−0.9 |
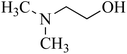 |
5 |
Amino-2-methyl-1-propanol (AMP)/hindered |
C4H11NO |
124-68-5 |
89.14 |
−0.7 |
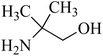 |
6 |
Tetramethylethylenediamine (TMEDA)/diamine |
C6H16N2 |
110-18-9 |
116.24 |
−0.3 |
 |
7 |
Tetramethyl-1,3-propanediamine (TMPDA)/diamine |
C7H18N2 |
110-95-2 |
130.23 |
0.2 |
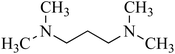 |
In this work, we report on the ecotoxicity of structurally diverse amines utilized in emerging SW and CO2 capture technologies. The list includes primary monoethanolamine (MEA), secondary diethanolamine (DEA), tertiary triethanol – (TEA) and dimethylethanolamine (DMEA), structurally hindered amino-2-methyl-1-propanol (AMP), and two diamines – tetramethylethylenediamine (TMEDA) and tetramethyl-1,3-propanediamine (TMPDA). Such amines are often used for SW and CC processes due to their ability to absorb gases efficiently.23 MEA, DEA, TEA, AMP, and DMEA are alkanolamines used in both processes.
The ecotoxicity was assessed on aquatic organisms with varying biological complexity, i.e., bacteria, vascular plants, and invertebrates.
Experimental
Test compounds
The tested compounds are listed in Table 1: monoethanolamine (MEA) (Alfa Aesar, purity 98%), diethanolamine (DEA) (Lach-Ner, purity 99%), triethanolamine (TEA) (Alfa Aesar, purity 98%), dimethylethanolamine (DMEA, reagent grade), 2-amino-2-methyl-1-propanol (AMP, reagent grade), tetramethylethylenediamine (TMEDA) (Sigma Aldrich, purity 99%), tetramethyl-1,3-propanediamine (TMPDA) (Sigma Aldrich, purity 99%). All tested compounds were liquids and miscible with water.
Ecotoxicity testing
The toxicity assessment was performed using three standardized tests. The level of toxicity of compounds was determined by establishing the half-maximal effective concentration, EC50, the concentration of substances in the environment that will affect 50% of the organisms in the test population under specified conditions. For bacterial tests, the range of concentration of each sample was 1–5000 mg L−1, and for vascular plants and invertebrates, the range was 1–1000 mg L−1. A fresh stock solution with the highest tested concentration was prepared for each test, thus ensuring concentration accuracy throughout the studies. Serial dilutions of the stock solution were then performed to obtain the lower-concentration solutions. All samples were tested in triplicate for each assay to ensure test reproducibility. The stability of TMPDA and TMEDA was checked by incubating the compounds under tested conditions without organisms and the results were confirmed by 1H and 13C NMR (Fig. S1–S12†).
WaterTOX™ STD: bacterial luminescence inhibition test using Aliivibrio fischeri. Toxicity of samples towards the bioluminescent marine bacterium A. fischeri was measured by comparing initial and final light emission after 15 min according to the ISO standard 11348-3:2007.24 The toxic effect caused by a decrease in cellular metabolism is expressed as a decrease of the luminescence intensity. Tests were carried out at 15 °C in the kit's standard diluent. Potassium dichromate [12.5–100 mg L−1] was used as a reference positive control. A series of dilutions were prepared for each sample according to the manufacturer's instructions.
Growth inhibition test with vascular plants. Spirodela polyrhiza is based on the measurement of growth retardation of the germinated dormant vegetative buds (turions) after 3 days of exposure to samples according to the ISP standard.25 The tests were carried out on a 48-well plate containing a dilution series of tested samples at 25 °C in the plant growth chamber, using an illumination system enabling at least 6000 lux. A digital image of the multiwell plate was taken at the start of the test and after the incubation to measure the growth inhibition by Image Analysis (Image J, National Institute of Mental Health, Bethesda, Maryland, USA, software for image processing and analysis) to determine the size of the vegetative buds (turions) before and after incubation. Next, by comparing the data obtained from the test plate, the growth of the duckweeds was calculated by subtracting the mean of the “initial” size of the first frond from the mean “final” size, in the control and at various concentrations of diluted samples. The 72 h EC50 concentration of the compound was obtained from the percentage of growth inhibition of the duckweed.
Crustacean toxicity screening test for freshwater using Daphnia magna. This test determines the lethal effects of toxicants on the D. magna after 48 h exposure. The 48 h immobilization test was performed in a multi-well test plate using neonate D. magna hatched from ephippia based on the ISO standard.26 Ephippia hatching was initiated before the start of the toxicity test in a Petri dish with standard freshwater medium at 25 °C for 72 h, under continuous illumination (at least 6000 lux). Two hours before collecting the neonates for the test, they were pre-fed with Spirulina powder. The test incubation was carried out on a multiwell plate containing a dilution series of the tested samples in darkness for 48 hours. The number of immobilized (dead) organisms was counted after 48 h under a microscope (magnification 10–12×). The obtained data was used to determine the EC50 values.
Prediction of EC50 for amines. Modelling was done using the Ecological Structure Activity Relationships (ECOSAR) predictive model (Tracy Wright, U.S. EPA Existing Chemicals Risk Assessment Division), software for estimating a chemical's acute (short-term) toxicity and chronic (long-term or delayed) toxicity to aquatic organisms.
Statistical analysis. A comparison of the tested substrates was analysed using one-way ANOVA, and a post hoc pairwise comparison via a Tukey test in R (version 3.6.0) using aov( ) and TukeyHSD( ) on EC50 data (Table S2†). The linear trends of log(1/EC50) against log
Kow for each tested organism were found based on the performed tests.
Results and discussion
The results of the toxicity measurements of the tested compounds are visualized in Fig. 2. The tertiary amine TEA showed the highest EC50 values (i.e., the lowest toxicity) across all tested organisms and can be rated as non-toxic (EC50 > 1000 mg L−1, Fig. 2a–c). Compared to TEA, the secondary DEA exhibited lower EC50 values, followed by the primary MEA but both can still be categorized as practically harmless towards bacteria [EC50(DEA) = 468 (95% CI: 280; 656) mg L−1; EC50(MEA) = 227 (95% CI: 150; 304) mg L−1], vascular plants [ EC50(DEA) = 549 (95% CI: 479; 619) mg L−1; EC50(MEA) = 358 (95% CI: 262; 454) mg L−1] and invertebrates [EC50(DEA) = 367 (95% CI: 288; 447) mg L−1; EC50(MEA) = 260 (95% CI: 203; 317) mg L−1]. Thus, the toxicity of these aminoalcohols increases in the following order: TEA < DEA < MEA. These results align with the previous ecotoxicity studies of MEA, DEA, and TEA in seawater environments, where the toxicity also decreased when alkyl substituents were added to the nitrogen atom.20,27 Similarly, Finlay and Callow have investigated the impact of alkyl chain length and branching on aquatic organisms.14 They found that alkylamines with shorter and less-branched alkyl chains exhibited higher toxicity than those with longer and more highly branched chains.
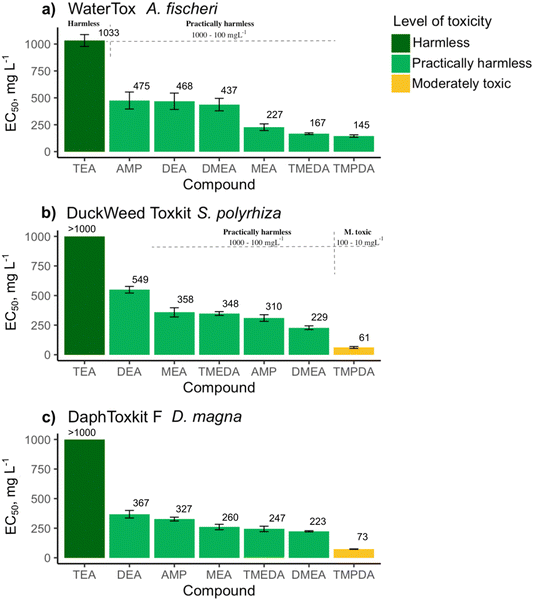 |
| Fig. 2 Values of the tested compounds' mean effective concentrations (EC50, mg L−1) towards (a) A. fischeri, (b) S. polyrhiza, (c) D. magna. The compounds are ordered according to the level of toxicity: dark green represents the harmless (EC50 values > 1000 mg L−1) compounds; light green represents the practically harmless (EC50 values 100–1000 mg L−1) compounds; yellow denotes the moderately toxic (EC50 values of 10–100 mg L−1) compounds. For numerical values, see (Table S1†). | |
Compared to TEA, another tertiary alkanolamine, DMEA, which has two ethanol groups replaced by methyl substituents, exhibited somewhat lower EC50 values towards all three groups of organisms. DMEA showed a practically harmless level of toxicity towards S. polyrhiza [EC50 = 229 (95% CI: 188; 269) mg L−1], D. magna [EC50 = 223 (95% CI: 213; 234) mg L−1] and A. fischeri [EC50 = 437 (95% CI: 293; 581) mg L−1].
Primary aminoalcohol AMP is a structural isomer of DMEA with different arrangements of substituents. The toxicity levels of these two isomers were also relatively similar, although the EC50 values for AMP were slightly higher towards the tested organisms, i.e., EC50 = 475 (95% CI: 279; 671) mg L−1 towards A. fischeri, EC50 = 310 (95% CI: 240; 380) mg L−1 towards S. polyrhiza and EC50 = 327 (95% CI: 287; 367) mg L−1 towards D. magna.
Finally, we evaluated two very similar structures, TMEDA and TMPDA, which differ only by the length of the carbon spacer between the two nitrogen atoms. While diamine TMEDA with an ethylene spacer showed a practically harmless effect toward A. fischeri [EC50 = 167 (95% CI: 149; 186) mg L−1], S. polyrhiza [EC50 = 348 (95% CI: 308; 388) mg L−1] and D. magna [EC50 = 247 (95% CI: 192; 302) mg L−1], in contrast, TMPDA with a propylene spacer was moderately toxic to S. polyrhiza [EC50 = 61 (95% CI: 41; 81) mg L−1] and to D. magna [EC50 = 73 (95% CI: 69; 77) mg L−1]. Moreover, TMPDA was the only tested compound with EC50 values in the moderate toxicity range, although towards A. fischeri it can still be categorized as practically harmless [EC50 = 145 (95% CI: 117; 172) mg L−1].
We correlated our results with the octanol–water partition coefficient (log
Kow; for calculated values, see Table 1). This coefficient measures a compound's hydrophobicity and shows the distribution between a hydrophobic (octanol) and a hydrophilic (water) phase.28 This parameter is often used to estimate the potential environmental risk of compounds to the aquatic environment. Chemicals with high log
Kow values are less soluble in water, which increases bioaccumulation and potential toxicity levels.29 In our study, tertiary alkanolamine TEA is the only compound that showed a harmless level of toxicity towards all tested organisms. The log
Kow for TEA is −2.5, which is the lowest value compared to other alkanolamines that have log
Kow values in the range from −0.7 to −1.7 and a practically harmless level of toxicity. The diamine TMPDA, which has the highest log
Kow value among all tested compounds (log
Kow = 0.2), showed moderate toxicity toward S. polyrhiza and D. magna. Such correlation, where more hydrophilic compounds (TEA, DEA, MEA, DMEA, AMP, TMEDA) exhibit lower toxicity compared to the more hydrophobic compounds (TMPDA), also aligns with our previous ecotoxicology study with isosorbide-based compounds.30
There could be several potential mechanisms responsible for amine toxicity. One of the reported mechanisms is the induction of oxidative stress by the generation of reactive oxygen species, which leads to lipid peroxidation and cytotoxicity.31,32 Additionally, reactive amine-derived species, such as nitrosamines or imines, can cause DNA damage.33
We also evaluated the aquatic toxicity of the same test compounds towards Daphnia magna and green algae using the Ecological Structure Activity Relationships (ECOSAR) predictive model (Table 2). Compared to our experimental results, the ECOSAR predicts similar trends, and in many cases, even the individual EC50 values are comparable. For example, TEA and TMPDA are the least and most toxic compounds, respectively, by both methods, and their EC50 values fall into the same range. Additionally, mono-, di-, and triethanolamines exhibit the same order of toxicity and similar EC50 values. Hence, for such types of amines and alkanolamines, the ECOSAR can be considered a valuable tool for initial screening.
Table 2 ECOSAR prediction results for amines
Short name |
D. magna 48 h |
Green algae 48 h |
EC50 mg L−1 (ECOSAR) |
EC50 mg L−1 (ECOSAR) |
TEA |
1771 |
4092 |
DEA |
430 |
834 |
MEA |
217 |
411 |
DMEA |
123 |
199 |
AMP |
94 |
145 |
TMEDA |
63 |
87 |
TMPDA |
35 |
44 |
Additionally, we carried out liner regression analysis between log
Kow and toxicity for amines to each tested organism log(1/EC50) (Fig. S13†). The resultant regressions are: log(1/EC50) = 0.32
log
Kow − 2.1 (R2 = 0.76, p-value = 3.03 × 10−7) for D. magna; log(1/EC50) = 0.35
log
Kow − 2.1 (R2 = 0.74, p-value = 5.33 × 10−7) for S. polyrhiza; and log(1/EC50) = 0.33
log
Kow − 2.6 (R2 = 0.62, p-value = 2.01 × 10−5) for V. fischeri. After analyzing the obtained equations, we conclude that there is no statistical difference between the tested organisms (Fig. S14†). Compared to the reported linear regression between log
Kow and log(1/EC50) for non-amine compounds,34 our results indicate that the amine compounds showed a similar or slightly lower level of ecotoxicity (Fig. S15†).
Conclusion
The toxicity measurements of various amines reveal a clear trend related to their structural and hydrophilicity properties. Tertiary amine TEA exhibited the highest EC50 values, indicating the lowest toxicity among the tested compounds, and is categorized as harmless. Secondary amine DEA and primary amine MEA, while slightly more toxic, are still considered practically harmless to the tested organisms. Tertiary DMEA, despite being somewhat more toxic than TEA, also falls into the almost harmless category. The structurally hindered AMP showed similar toxicity levels to DMEA.
The ecotoxicity results of diamines TMEDA and TMPDA demonstrated that even small structural changes, such as the length of the carbon spacer between nitrogen atoms, can impact toxicity. TMEDA remained practically harmless, while TMPDA showed moderate toxicity towards S. polyrhiza and D. magna organisms, likely due to its lower hydrophilicity. Overall, the results suggest that increased hydrophilicity in these amines correlates with reduced toxicological impact, probably due to enhanced excretion and decreased bioaccumulation.
Data availability
Data supporting this article have been included as a part of the ESI.†
Author contributions
Alina Ismagilova: investigation, data curation, formal analysis, validation, visualization, writing – original draft. Veljo Kisand: supervision, methodology, writing – review & editing. Lauri Vares: conceptualization, funding acquisition, supervision, methodology, writing – review & editing.
Conflicts of interest
The authors declare no conflict of interest.
Acknowledgements
This work was supported by the EEA and Norway Grants through the Baltic Research Programme (grant EMP426), by the Estonian Ministry of Education and Research through the Centre of Excellence in Circular Economy for Strategic Mineral and Carbon Resources (01.01.2024–31.12.2030, TK228), and by the Estonian Research Council (PRG2665). We thank Rauno Sedrik for critically reviewing the manuscript.
References
- R. Ben Said, J. M. Kolle, K. Essalah, B. Tangour and A. Sayari, A Unified Approach to CO2-Amine Reaction Mechanisms, ACS Omega, 2020, 5, 26125–26133 CrossRef PubMed.
- K. Maneeintr, R. O. Idem, P. Tontiwachwuthikul and A. G. H. Wee, Synthesis, solubilities, and cyclic capacities of amino alcohols for CO2 capture from flue gas streams, Energy Procedia, 2009, 1, 1327–1334 CrossRef CAS.
- F. Meng, Y. Meng, T. Ju, S. Han, L. Lin and J. Jiang, Research progress of aqueous amine solution for CO2 capture: a review, Renewable Sustainable Energy Rev., 2022, 168, 112902 CrossRef CAS.
- A. Gautam and M. K. Mondal, Review of recent trends and various techniques for CO2 capture: special emphasis on biphasic amine solvents, Fuel, 2023, 334, 126616 CrossRef CAS.
- S. Anwer, I. I. I. Alkhatib, H. A. Salih, L. F. Vega and I. AlNashef, Investigating the role of water on CO2 capture by amine-based deep eutectic solvents through a combined experimental-molecular modeling approach, Sep. Purif. Technol., 2024, 330, 125350 CrossRef CAS.
- F. Ausfelder, A. Bazzanella, H. VanBracki, R. Wilde, C. Beckmann, R. Mills, E. Rightor, C. Tam, N. Trudeau and P. Botschek, Technology Roadmap – Energy and GHG Reductions in the Chemical Industry via Catalytic Processes, 2013 Search PubMed.
- P. Pollet, C. A. Eckertabc and C. L. Liotta, Switchable solvents, Chem. Sci., 2011, 2, 609–614 RSC.
- S. M. Mercer, T. Robert, D. V. Dixon and P. G. Jessop, Recycling of a homogeneous catalyst using switchable water, Catal. Sci. Technol., 2012, 2, 1315–1318 RSC.
- X. Li, H. Lu, D. Liu, G. Yan, S. Dai and Z. Huang, Cleaner and sustainable approach of washing oil sands using CO2-responsive composite switchable water (CSW) with lower volatility, J. Cleaner Prod., 2019, 236, 117554 CrossRef CAS.
- S. M. Mercer, T. Robert, D. V. Dixon, C. S. Chen, Z. Ghoshouni, J. R. Harjani, S. Jahangiri, G. H. Peslherbe and P. G. Jessop, Design, synthesis, and solution behaviour of small polyamines as switchable water additives, Green Chem., 2012, 14, 832–839 RSC.
- M. Sanger, D. Barker and P. G. Jessop, Simultaneous switching of two different CO2-switchable amines in the same solution, Phys. Chem. Chem. Phys., 2024, 11406–11413 RSC.
- I. T. Cunha, H. Yang and P. G. Jessop, High pressure switchable water: an alternative method for separating organic products from water, Green Chem., 2021, 23, 3996–4007 RSC.
- V. S. Liberato, T. F. Ferreira, A. R. MacDonald, B. Dias Ribeiro, M. A. Zarur Coelho and P. G. Jessop, A CO2-responsive method for separating hydrophilic organic molecules from aqueous solutions: solvent-assisted switchable water, Green Chem., 2023, 25, 4705–4712 RSC.
- J. A. Finlay and M. E. Callow, The potential of alkyl amines as antifouling biocides I: toxicity and structure activity relationships, Biofouling, 1996, 9, 257–268 CrossRef CAS.
- L. Garnick, C. Bates, A. Massarsky, P. Spencer, P. Sura, A. D. Monnot and A. Maier, Developmental and reproductive toxicity hazard characterization of 2-amino-2-methyl-1-propanol (AMP), J. Appl. Toxicol., 2024, 44, 316–332 CrossRef CAS PubMed.
- B. Peric, J. Sierra, E. Martí, R. Cruañas, M. A. Garau, J. Arning, U. Bottin-Weber and S. Stolte, (Eco)toxicity and biodegradability of selected protic and aprotic ionic liquids, J. Hazard. Mater., 2013, 261, 99–105 CrossRef CAS PubMed.
- I. Eide-Haugmo, O. G. Brakstad, K. A. Hoff, K. R. Sørheim, E. F. da Silva and H. F. Svendsen, Environmental impact of amines, Energy Procedia, 2009, 1, 1297–1304 CrossRef CAS.
- G. Libralato, A. V. Ghirardini and F. Avezzù, Evaporation and air-stripping to assess and reduce ethanolamines toxicity in oily wastewater, J. Hazard. Mater., 2008, 153, 928–936 CrossRef CAS PubMed.
- A. E. Poste, M. Grung and R. F. Wright, Amines and amine-related compounds in surface waters: a review of sources, concentrations and aquatic toxicity, Sci. Total Environ., 2014, 481, 274–279 CrossRef CAS PubMed.
- J. W. Davis and C. L. Carpenter, Environmental assessment of the alkanolamines, Rev. Environ. Contam. Toxicol., 1997, 149, 87–137 CAS.
- F. Vega, A. Sanna, B. Navarrete, M. M. Maroto-Valer and V. J. Cortés, Degradation of amine-based solvents in CO2 capture process by chemical absorption, Greenhouse Gases:Sci. Technol., 2014, 4, 707–733 CrossRef CAS.
- J. M. van Rossum, The Relation Between Chemical Structure and Biological Activity, J. Pharm. Pharmacol., 1963, 15, 285–316 CrossRef CAS PubMed.
- P. C. Rooney, M. S. DuPart and T. R. Bacon, Oxygen's role in alkanolamine degradation, Hydrocarbon Process., 1998, 77, 109–113 CAS.
- European Committee for Standardization, ISO 11348-3:2007 – Water Quality – Determination of the Inhibitory Effect of Water Samples on the Light Emission of Vibrio fischeri (Luminescent Bacteria Test), 2007 Search PubMed.
- European Committee for Standardization, ISO 20079:2005 – Water Quality – Determination of Toxic Effect of Water Constituents and Waste Water to Duckweed (Lemna minor) – Duckweed Growth Inhibition Test, 2003 Search PubMed.
- European Committee for Standardization, ISO 6341:2012 – Water Quality – Determination of the Inhibition of the Mobility of Daphnia magna Straus (Cladocera, Crustacea) – Acute Toxicity Test, 2012 Search PubMed.
- G. Libralato, A. Volpi Ghirardini and F. Avezzù, Seawater ecotoxicity of monoethanolamine, diethanolamine and triethanolamine, J. Hazard. Mater., 2010, 176, 535–539 CrossRef CAS PubMed.
- S. Amézqueta, X. Subirats, E. Fuguet, M. Roses and C. Rafols, Octanol-water partition constant, Liquid-Phase Extraction, 2019, pp. 183–208 Search PubMed.
- E. Popek, Environmental Chemical Pollutants, Sampling and Analysis of Environmental Chemical Pollutants, 2018, pp. 13–69 Search PubMed.
- A. Ismagilova, L. Matt, P. Jannasch, V. Kisand and L. Vares, Ecotoxicity of isosorbide acrylate and methacrylate monomers and corresponding polymers, Green Chem., 2023, 25, 1626–1634 RSC.
- S. Awasthi and P. J. Boor, Lipid peroxidation and oxidative stress during acute allylamine-induced cardiovascular toxicity, J. Vasc. Res., 1994, 31, 33–41 CrossRef CAS PubMed.
- Y. Feng, S. Chen, Y. Zhao, D. Wu and G. Li, Heterocyclic aromatic amines induce Neuro-2a cells cytotoxicity through oxidative stress-mediated mitochondria-dependent apoptotic signals, Food Chem. Toxicol., 2022, 168 DOI:10.1016/j.fct.2022.113376.
- H. Bartsch and R. Montesano, Relevance of nitrosamines to human cancer, Carcinogenesis, 1984, 5, 1381–1393 CrossRef CAS PubMed.
- X. Zhang, W. Qin, J. He, Y. Wen, L. Su, L. Sheng and Y. Zhao, Discrimination of excess toxicity from narcotic effect: comparison of toxicity of class-based organic chemicals to Daphnia magna and Tetrahymena pyriformis, Chemosphere, 2013, 93, 397–407 CrossRef CAS PubMed.
|
This journal is © The Royal Society of Chemistry 2025 |
Click here to see how this site uses Cookies. View our privacy policy here.