DOI:
10.1039/D4EN00954A
(Perspective)
Environ. Sci.: Nano, 2025,
12, 41-47
Nanotechnology for oil spill response and cleanup in coastal regions
Received
10th October 2024
, Accepted 13th November 2024
First published on 18th November 2024
Abstract
Oil spills frequently cause devastating impacts on coastal ecosystems and communities. Spill response methods for coastal regions, such as spill-treating agents, sorbents, and bioremediation, may face constraints due to environmental concerns, limited absorption capacity, and low effectiveness. Fortunately, the emergence of nanomaterials with unique properties has introduced promising solutions for coastal oil spill remediation. These nanomaterials have shown great potential in oil removal, recovery, and degradation through different mechanisms. Nanoparticles or nanocomposites can interact with spilled oil by breaking it into small droplets and forming stable Pickering emulsions. They can also remove oil from water by absorption, adsorption, or in combination with both due to their large surface area and numerous sorption sites. Furthermore, some nanomaterials possess catalytic activity to speed up the degradation of petroleum hydrocarbons into less harmful compounds. Moreover, the introduction of nanomaterials can be beneficial for bacteria proliferation, nutrient supply, and maintenance of favorable conditions, thereby accelerating the oil biodegradation process by microorganisms. In this perspective, we discussed the interactions between nanomaterials and oil, as well as their applications in various coastal oil spill response methods.
Environmental significance
Coastal regions are particularly vulnerable to oil spills, which can trigger a cascade of adverse environmental consequences. In response to this pressing challenge, the integration of nanomaterials into oil spill response in coastal regions presents a promising and sustainable solution to improve the effectiveness and efficiency of cleanup efforts. Nanomaterials possess unique properties that allow them to interact with spilled oil in different manners, enabling their application across a diverse range of response methods, such as surface washing agents, dispersants, sorbents, and bioremediation. As research in this field continues to advance, the application of nanomaterials is poised to lead to the development of more advanced and robust spill response techniques, ensuring that more spilled oil will be removed thoroughly and quickly. This perspective presents a focused overview of nanomaterial–oil interactions and possible applications in coastal oil spill response.
|
1. Introduction
Spilled oil poses a grave threat when it reaches coastal areas, inflicting long-term adverse effects on fragile coastal ecosystems and communities.1–3 Efficient and effective oil spill response measures are essential for removing and recovering spilled oil, thereby minimizing environmental consequences to coastal regions. These measures include deploying sorbents, booms, and skimmers, using spill-treating agents (e.g., surface washing agents and dispersants), and conducting bioremediation.4 However, these conventional response methods often face limitations, such as low oil absorption capacity, potential toxicity to aquatic life, and slow remediation process.5 Clearly, there is a pressing need to develop more advanced spill response techniques to cope with the challenges of oil spills in coastal areas.
Fortunately, the use of nanomaterials has emerged as a promising solution for coastal oil spill response, owing to their inherent benefits of nanoscale size effects, versatility, and environmental compatibility.6–11 Numerous studies have reported that nanomaterials, with or without modifications, can more effectively interact with oil to enhance oil removal and recovery through mechanisms such as changing surface wetting properties, stabilizing oil/water emulsion, and decreasing interfacial tension. Moreover, some catalytic nanoparticles or nanocomposites are capable of accelerating the breakdown of petroleum hydrocarbons, converting them into less harmful substances. Additionally, the oil biodegradation process by marine microorganisms can be expedited with the help of nanotechnology, as nanomaterials can disperse spilled oil into small droplets with a large surface area for interaction, provide nutrients to oil-degrading bacteria, and create or maintain favorable conditions for microbial attachment and growth.12 These remarkable features suggest that the application of nanomaterials holds great potential for oil removal and biodegradation. Therefore, the incorporation of nanotechnology into various response methods in coastal regions (Fig. 1), such as surface washing agents, dispersants, sorbents, and biodegradation, is discussed in this article.
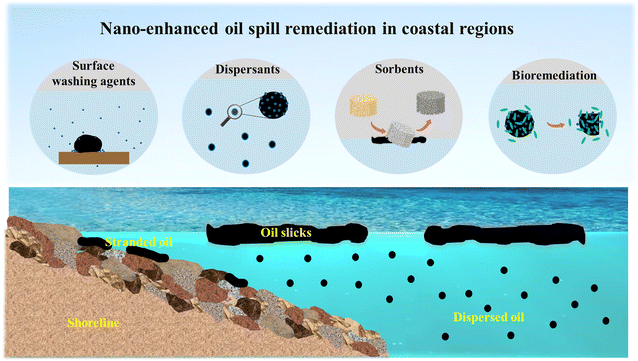 |
| Fig. 1 Schematic diagram demonstrating the application of nanomaterials in coastal oil spill response and cleanup. | |
2. Interactions of nanomaterials with oil
2.1 Adsorption
Adsorption is a surface-based exothermic process in which oil from the water column or solid substrates adheres to the surface of nanoparticles. This process can be categorized into two main types: physical adsorption (e.g., Van der Waals forces and electrostatic interactions) and chemical adsorption (e.g., covalent bonds), based on interactions between oil and nanoparticles.13 Nanomaterial-based adsorbents exhibit excellent performance in oil removal from coastal environments due to their large surface area, easy functionalization, numerous sorption sites, tunable pore size, etc. Bio-based nanomaterials and nanoclay, with numerous advantages such as environmental friendliness, easy availability, and cost-effectiveness, have become preferred choices for oil spill response methods in coastal regions, although some of them may have low sorption capacity and poor oil wettability. For example, cellulose nanofibers are hydrophilic due to the presence of hydroxyl groups on their surfaces and lack of water and oil selectivity, restricting their application in oil removal. However, their structures can be modified or functionalized through substituting hydrophobic/oleophilic moieties to increase oil adsorption and water repellency.14 The adsorption efficiency is also greatly affected by the shape, morphology, and porosity (porous materials) of nanomaterials.
2.2 Interface interactions
The surface and interfacial properties of nanoparticles enable them to accumulate at the oil/water or oil/water/solid interface, profoundly affecting the behavior of spilled oil in coastal environments. These nanoparticle-driven interactions can manifest in various ways, such as interfacial tension reduction, wettability alteration, and emulsion stabilization or destabilization.15 The presence of nanoparticles often leads to lower interfacial tension between oil and water, promoting the breakup of oil slicks into smaller droplets to improve oil removal efficiency and facilitate oil dispersion and biodegradation in coastal regions. Furthermore, hydrophilic nanoparticles have the ability to alter the wettability of solid substrates from an oil-wet to a water-wet status, facilitating the mobilization of stranded oil, and their concentrations, size, surface properties, as well as base fluid characteristics are critical to determine the wettability alteration degree. For example, nanoparticles with smaller sizes and higher charge density will experience a high degree of electrostatic repulsion among themselves, which is beneficial for fluid stability and their spreading on substrate surfaces. When confined to the wedge in the three-phase contact region, they also exert high disjoining pressure that forces nanoparticles move forward, separating oil from solid surfaces.16 Moreover, nanoparticles can act as Pickering emulsifiers, adsorbing irreversibly at immiscible biphasic interfaces and preventing droplet coalescence. The stability of formed Pickering emulsion is largely determined by the wettability of nanoparticles. Other nanoparticle parameters, such as morphology, can also affect the emulsion stability, with cylindrical shapes reported to have the potential to generate more stable emulsions.17 Compared to surfactant-stabilized emulsions, Pickering emulsions exhibit superior stability, environmental friendliness, and broader applicability, showing great potential for oil spill recovery.
2.3 Catalytic activity
Photocatalytic nanomaterials, such as ZnO and TiO2 nanoparticles, possess the remarkable ability to degrade petroleum hydrocarbons when exposed to solar energy, which can greatly enhance oil degradation and decrease the environmental consequences. For example, when TiO2 is exposed to light, the electrons in the valence band (VB) are excited by photons to the conduction band (CB), generating electron (e−)–hole (h+) pairs, which will interact with water and dissolved oxygen to form reactive oxygen species (ROS). The ROS generated at the active sites of the photocatalytic nanomaterials can then degrade hydrocarbon molecules through successive radical reactions, ultimately converting them into inorganic and nontoxic substances, such as carbon dioxide and water.18 Piezocatalysis of nanoparticles is able to convert ceaseless mechanical energy into chemical energy to initiate redox reactions. The piezoelectric nanomaterials have the ability to generate electric charges when subjected to mechanical stress, such as the motion of water. These generated electric charges can then interact with water and dissolved oxygen to produce ROS for oil degradation.19
2.4 Biodegradation
The incorporation of nanoparticles can significantly enhance the oil biodegradation by microorganisms in different ways during oil spill response in coastal areas. As an environmentally friendly alternative to chemical surfactants, some nanoparticles, such as nanoclay, can assemble at the oil/water interface and act as Pickering emulsifiers to stabilize oil droplets, which is beneficial for oil-consuming bacterial proliferation. The biodegradation rate will also be improved because of the large surface area of oil and increased oil bioavailability. These nanoparticles often have buffering ability and the capacity to adsorb protons generated during hydrocarbon breakdown, being crucial for maintaining suitable pH levels and sustaining bacterial metabolism and growth. Meanwhile, nutrient adsorption will be facilitated with the generation of C–O–Na–Si complexes on bacterial cell walls to enhance bacterial activity.12,20 Furthermore, nanoparticles can be engineered for the target release of essential nutrients to oil phase to promote the bacterial degradation of spilled oil.21 Moreover, the cell walls of oil-degrading bacteria can be encapsulated and functionalized with nanoparticles to impart magnetic responsiveness, which can accelerate the oil biodegradation process.
3. Nanotechnology-based response methods in coastal regions
3.1 Surface washing agents
Surface washing agents are used to remove the stranded oil from shoreline substrates in coastal regions. Nanomaterials have emerged as a versatile tool in the formulation of these surface washing fluids, offering several advantages in oil removal through various mechanisms. The primary mechanisms by which nanomaterials enhance the performance of surface washing fluids include wettability alteration, interfacial tension reduction, and the enhancement of disjoining pressure between oil and solid surfaces (Fig. 2a).22 Bio-based nanoparticles or nanocomposites, such as cellulose nanocrystal and its functionalized composite, modified nanoclay, and chitosan/rhamnolipid complexes, have been utilized as green and responsive washing fluids to treat oiled beach sand. These nanomaterial-based washing fluids have demonstrated high oil removal capacity, reduced oil biotoxicity to algae, and efficient ionic strength-responsive separation of washing effluents, making them promising candidates for shoreline cleanup applications. In addition, magnetic-mediated surface washing fluids containing iron-based nanomaterials have also been developed to remove oil from the shoreline. For instance, a washing fluid containing magnetite nanoparticles functionalized with carboxyl groups achieved over 70% of engine oil removal from oiled sand under conditions of 1 g L−1, 300 rpm, and 20 °C. The performance of these magnetic-based washing fluids can be influenced by various factors, including salinity, temperature, mineral concentration, and magnetite concentration. The underlying mechanisms involve the synergistic effects of interfacial tension reduction, wetting behavior alternation, the transport behavior of magnetite, and changes in solid–fluid contact.23 The magnetic responsiveness of these nanomaterial-based washing fluids enables the treatment of the magnetic fields to recover the oil and magnetite, thereby reducing the potential for secondary pollution. Furthermore, surface washing fluids containing piezoelectric materials, such as MoS2, could not only physically remove oil from sand through interfacial tension reduction but also chemically degrade high-molecular-weight hydrocarbons into low-molecular-weight hydrocarbons through the triggering piezocatalysis by water motion.24 Despite the promise of nano-based washing fluids in terms of environmental sustainability, further improvements in their oil removal performance and broadening application range are crucial to fit diverse cleanup scenarios in coastal regions, such as Arctic and subarctic climatic conditions. Meanwhile, molecular simulations can be conducted to investigate how nanoparticles interact with oil molecules at the molecular level, providing a deeper understanding in oil detachment mechanisms and processes, which will help develop more efficient and adaptable formulations.
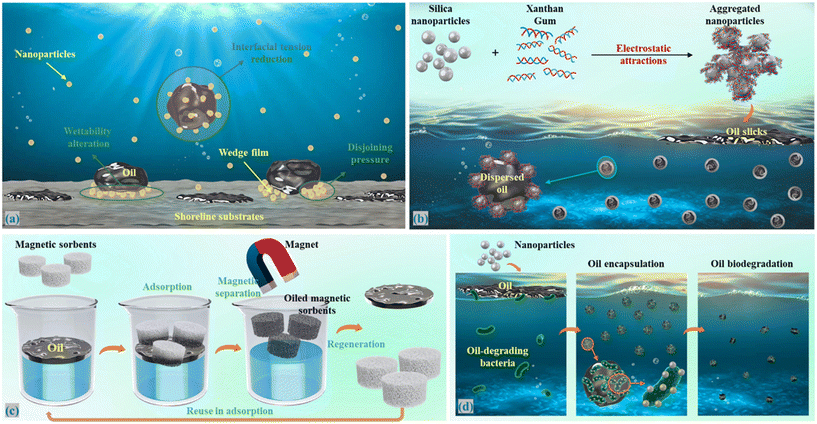 |
| Fig. 2 (a) Oil removal mechanisms of nanoparticles used as surface washing fluids. (b) A nanoparticle-based dispersant example using the synergy of xanthan gum and silica nanoparticles. (c) Using and recycling of nano-based magnetic sponge for oil sorption and regeneration. (d) Accelerating natural breakdown process of spilled oil through the addition of nanoparticles. | |
3.2 Dispersants
Chemical dispersants, typically formulated with surfactants and organic solvents, are commonly used to reduce the oil/water interfacial tension and disperse oil slicks into small oil droplets.25,26 However, these dispersants may pose toxicity risks to aquatic organisms, especially in more sensitive coastal environments. As an environmentally friendly alternative to chemical dispersants, nanoparticles can adsorb on the oil/water interface and stabilize emulsions, forming nanoparticle-based dispersants with high effectiveness and less toxicity.27 The nanoparticle-stabilized oil droplets function in a manner similar to naturally occurring oil particle aggregates (OPAs) found in near seashore regions that are composed of suspended sediments and oil droplets. The large surface area created by nanoparticles enhances the interaction between the spilled oil and indigenous bacteria, thereby accelerating the biodegradation of the oil in coastal areas.28 Various types of nanoparticles such as naturally occurring clay mineral, silica, carbon black, and nickel hydroxide have been used as dispersants to form stable emulsions in simulated oil spills, and their surfaces are often precisely engineered by other additives to change hydrophilic–lipophilic balance, optimize wetting characteristics, and further reduce interfacial tension to generate more stable emulsions with much smaller oil droplets, improving the dispersion ability. In addition to chemical modification, physical adsorption of oppositely charged polymer on nanoparticles was also applied in oil dispersion. For example, a proposed dispersant that mixes xanthan gum with silica nanoparticles demonstrates a synergistic emulsification effect, where the electrostatic interactions between the two components lead to greater stability and reduced coalescence of the oil-in-water emulsion (Fig. 2b).29 The size of oil droplets generated by nanoparticle-based dispersants was significantly influenced by factors such as nanoparticle type and properties, oil characteristics, and environmental conditions. Like chemical dispersants, nano-based dispersants cannot be recovered or reused once applied to disperse spilled oil in coastal regions. Some nanoparticles used in formulating nano-dispersants, such as silicon dioxide and nickel hydroxide, may not degrade easily, potentially accumulating and disrupting marine ecosystems. Focusing on biodegradable or natural-origin nanoparticles and conducting long-term environmental impact studies of nano-dispersants is essential to ensure the sustainable use of nanotechnology in coastal oil spill response.
3.3 Sorbents
During oil spill response, sorbents (insoluble products) are commonly deployed to remove spilled oil from water through absorption, adsorption, or a combination of both. Nanotechnology-based sorbents have emerged as a promising solution, offering high oil sorption capacity, low cost, and excellent oil selectivity; these sorbents can take various forms including aerogels, foams, sponges, nanoparticles, fibers, and so on. Bio-based nanomaterials are considered potential candidates for sorbents, as they offer advantages such as biodegradability and biocompatibility. However, most of these bio-based sorbents require modifications to achieve optimal performance, such as changing wetting properties, increasing surface area, reducing density, and optimizing microstructures. Cellulose-based sorbents have been well-studied for their application in oil spill cleanup and have demonstrated promising results.30 Sorbent derived from inorganic nanocomposites (e.g., carbon nanotubes, graphene, mineral clays, and silica) also exhibited excellent performance with chemical stability, great sorption ability, and excellent recyclability. For instance, a sponge coated with reduced graphene oxide has shown absorption capacities of over 80 g g−1 for all types of organic liquids with a maximum value of 160 g g−1 for chloroform and did not show deterioration even after 50 reuse cycles.31 Furthermore, magnetic sorbents functionalized with nanoparticles have been developed to improve stability, increase wetting ability and sorption ability, and make them recyclable (Fig. 2c). The collected oil can be easily removed through the application of magnetic fields. Challenges for the application of nano-sorbents include synthetic methods that are difficult to implement on a large scale, the involvement of harmful chemicals, nanoparticle flaking during use and reuse, and the lack of cost estimation. A green synthesis approach should be developed to ensure cost-effective production of nano-sorbents. Meanwhile, ensuring stronger adhesion of nanoparticles to sorbent matrices is crucial and can be achieved through advanced surface modification techniques. There is also a need for a detailed cost–benefit analysis covering production, use, recycling, and disposal of nano-sorbents during oil spill response in coastal areas.
3.4 Nano-enhanced bioremediation
Incorporating nanomaterials into bioremediation strategies has been studied extensively to enhance the breakdown process of spilled oil, particularly in the context of polluted seawater water (Fig. 2d). Sustainable Pickering emulsifiers, such as the addition of clay minerals like kaolinite, montmorillonite, and nontronite, have been found to be beneficial for oil degradation by microorganisms, even in high oil concentrations (i.e., ∼150 g l−1 of heavy oil).32 These clay minerals, with their large specific surface areas and high cation exchange capacities, have demonstrated a more significant level of oil biodegradation.33 A nanoscale reaction model has been proposed, in which bivalent cations (i.e., Ca2+ and Mg2+ ions) at interlayer and hydrated surfaces can locally bridge clay minerals and bacterial cells or biofilms, promoting the nutrient supply to the bacteria from clay surface.20 In addition to using unmodified clay minerals, nanoparticles can also be functionalized to further accelerate the oil biodegradation process, although this approach can sometimes result in inhibition. For example, modified halloysite nanotubes with amphiphilic polypeptoid have been shown to form more stable oil emulsions as stabilizers with much smaller droplet sizes. They were also found to be noncytotoxic toward an alkane degrading bacterium, thereby promoting greater bacteria proliferation.34 Similarly, functionalized mesoporous silica nanoparticles with a gated system of hydrophobicity-responsive features have enabled the release of nutrients at the oil/water interface, stimulating bacteria to degrade the oil.21 It is worth noting that the possibility of modifying the bacteria itself to enhance the biodegradation of spilled oil has also been explored, although the relevant studies in this area are limited.35 Current research on nano-enhanced bioremediation is largely confined to lab-scale studies under well controlled conditions, making it difficult to predict its effectiveness in highly dynamic coastal environments, such as those with varying nutrient availability and salinity. Another concern is associated with unknown environmental impacts of introducing nanomaterials into marine environments, particularly synthetic nanomaterials (e.g., magnetic nanoparticles). Future research is needed to evaluate the performance of nano-enhanced bioremediation under more complex scenarios, such as low temperature, high salinity, and varying nutrient levels, and to further explore long-term biological impacts of released nanoparticles. Additionally, studies should prioritize the use of more sustainable and eco-friendly nanomaterials, such as mineral- and bio-based nanoparticles, to minimize potential environmental risks.
4. Conclusions and future outlook
The use of nanomaterials has shown great promise in addressing oil spill response, particularly in coastal areas that are vulnerable to the adverse effects of spilled oil. Nanomaterials possess unique characteristics that allow them to interact with the oil from water column or shoreline substrates due to their great adsorption capacity, interfacial properties, catalytic activity, and ability to enhance biodegradation, significantly accelerating oil removal and degradation. Based on these promising characteristics, the application of nanomaterials has been studied and investigated in a series of oil spill response methods in coastal regions, including surface washing agents, dispersants, sorbents, and biodegradation. The results of these studies have been encouraging and have demonstrated the potential of nanomaterials to improve the effectiveness of oil spill cleanup efforts in coastal areas. It is important to note that nano-based solutions in this area are still being developed and are mainly based on laboratory-scale experiments, which may not fully reflect the complexities and challenges of oil spill response and cleanup in coastal regions. However, the findings of these studies can provide critical insights into the design and implementation of field tests and on-site applications.
There is a need to scale up these tests to mesocosm or field experiments to validate the feasibility and efficacy of nanomaterials under more real-world conditions in coastal oil spill response. Since nano-enhanced response methods such as dispersants, bioremediation, and in situ treatment with washing fluids may release nanoparticles into coastal environments during oil spill response, future research should emphasize the use of safer nanomaterials with better environmental compatibility and less toxicity. For nano-based sorbents, nanoparticles should be encapsulated in biodegradable carriers or in that are easier to collect and recover to minimize environmental impacts. However, environmental risks associated with using nanotechnology in spill response still need to be thoroughly investigated, as nanoparticles can interact with sediments, vegetation, microorganisms, and marine animals (e.g., invertebrates and fish) in coastal regions and may also accumulate through the food chain.
Data availability
No primary research results, software or code have been included and no new data were generated or analysed as part of this perspective article.
Conflicts of interest
There are no conflicts to declare.
Acknowledgements
This research was supported by the Multi-partner Research Initiative of Natural Resources of Canada, Le Fonds de recherche du Québec – Nature et technologies (FRQNT), and the Natural Sciences and Engineering Research Council of Canada. Huifang Bi gratefully acknowledges the support of the Vanier Canada Graduate Scholarship program. The authors are thankful to the comments and suggestions from the editor and the anonymous reviewers.
References
- L. Rabinovitch, G. Saturos, P. McCallum, H. Kwok, J. Yan, T. Filewood, R. Cody, P. Brunswick and D. Shang, Rapid analysis of spilled petroleum oils by direct analysis in real time time-of-flight mass spectrometry with hydrophobic paper sample collection, Environ. Syst. Res., 2024, 13, 1–16 CrossRef.
- D. Liu, M. Liu, G. Sun, Z. Zhou, D. Wang, F. He, J. Li, J. Xie, R. Gettler and E. Brunson, Assessing environmental oil spill based on fluorescence images of water samples and deep learning, J. Environ. Inform., 2023, 42, 1–12 CAS.
- Z. Asif, Z. Chen, C. An and J. Dong, Environmental impacts and challenges associated with oil spills on shorelines, J. Mar. Sci. Eng., 2022, 10, 762 CrossRef.
-
ECCC, A field guide to oil spill response on marine shorelines, Environment and Climate Change Canada, Ottawa, ON, 2016 Search PubMed.
- H. Bi, C. An, C. N. Mulligan, Z. Chen, K. Lee, J. Wen, Z. Qu and X. Chen, Application of phase-selective organogelators (PSOGs) for marine oil spill remediation, J. Mar. Sci. Eng., 2022, 10, 1111 CrossRef.
- S. Iftekhar, A. Deb, G. Heidari, M. Sillanpää, V.-P. Lehto, B. Doshi, M. Hosseinzadeh and E. N. Zare, A review on the effectiveness of nanocomposites for the treatment and recovery of oil spill, Environ. Sci. Pollut. Res., 2023, 30, 16947–16983 CrossRef CAS PubMed.
- Y. Gao, L. Zhou, S. Ouyang, J. Sun and Q. Zhou, Environmental applications and risks of engineered nanomaterials in removing petroleum oil in soil, Sci. Total Environ., 2024, 174165 CrossRef CAS.
- H. Bi, C. An, C. N. Mulligan, K. Zhang, K. Lee and R. Yue, Treatment of oiled beach sand using a green and responsive washing fluid with nonionic surfactant-modified nanoclay, J. Cleaner Prod., 2022, 333, 130122 CrossRef CAS.
- Z. Chen, C. An, J. Yin, E. Owens, K. Lee, K. Zhang and X. Tian, Exploring the use of cellulose nanocrystal as surface-washing agent for oiled shoreline cleanup, J. Hazard. Mater., 2021, 402, 123464 CrossRef CAS.
- H. Zhao, X. Hu, H. Kang, D. Yang, M. Bao and Y. Li, Synergistic dispersion and biodegradation of oil in seawater based on Janus nanosheets and oil-degrading bacteria, Environ. Sci.: Nano, 2024, 11, 123–135 RSC.
- Y. Shen, B. Li, X. Chen, S. Wang, Y. Guan and Y. Li, Phase-selective cellulose nanofibril-based oil gelling agent for oil spill recovery, Environ. Sci.: Nano, 2022, 9, 489–498 RSC.
- A. J. Pete, B. Bharti and M. G. Benton, Nano-enhanced bioremediation for oil spills: a review, ACS ES&T Eng., 2021, 1, 928–946 Search PubMed.
-
R. Kecili and C. M. Hussain, in Nanomaterials in Chromatography, Elsevier, 2018, pp. 89–115 Search PubMed.
- S. b. Hammouda, Z. Chen, C. An and K. Lee, Recent advances in developing cellulosic sorbent materials for oil spill cleanup: A state-of-the-art review, J. Cleaner Prod., 2021, 127630 CrossRef CAS.
- J. Foroozesh and S. Kumar, Nanoparticles behaviors in porous media: Application to enhanced oil recovery, J. Mol. Liq., 2020, 316, 113876 CrossRef CAS.
- S. Li, O. Torsæter, H. C. Lau, N. J. Hadia and L. P. Stubbs, The impact of nanoparticle adsorption on transport and wettability alteration in water-wet Berea sandstone: An experimental study, Front. Phys., 2019, 7, 74 CrossRef.
- A. Pandey, M. Derakhshandeh, S. A. Kedzior, B. Pilapil, N. Shomrat, T. Segal-Peretz, S. L. Bryant and M. Trifkovic, Role of interparticle interactions on microstructural and rheological properties of cellulose nanocrystal stabilized emulsions, J. Colloid Interface Sci., 2018, 532, 808–818 CrossRef CAS.
- I. Ani, U. Akpan, M. Olutoye and B. Hameed, Photocatalytic degradation of pollutants in petroleum refinery wastewater by TiO2-and ZnO-based photocatalysts: recent development, J. Cleaner Prod., 2018, 205, 930–954 CrossRef CAS.
- S. Li, Z. Zhao, D. Yu, J.-Z. Zhao, Y. Su, Y. Liu, Y. Lin, W. Liu, H. Xu and Z. Zhang, Few-layer transition metal dichalcogenides (MoS2, WS2, and WSe2) for water splitting and degradation of organic pollutants: Understanding the piezocatalytic effect, Nano Energy, 2019, 66, 104083 CrossRef CAS.
- L. N. Warr, J. N. Perdrial, M.-C. Lett, A. Heinrich-Salmeron and M. Khodja, Clay mineral-enhanced bioremediation of marine oil pollution, Appl. Clay Sci., 2009, 46, 337–345 CrossRef CAS.
- N. Corvini, M. El Idrissi, E. Dimitriadou, P. F.-X. Corvini and P. Shahgaldian, Hydrophobicity-responsive engineered mesoporous silica nanoparticles: Application in the delivery of essential nutrients to bacteria combating oil spills, Chem. Commun., 2019, 55, 7478–7481 RSC.
- H. Rezvani, M. Riazi, M. Tabaei, Y. Kazemzadeh and M. Sharifi, Experimental investigation of interfacial properties in the EOR mechanisms by the novel synthesized Fe3O4@ Chitosan nanocomposites, Colloids Surf., A, 2018, 544, 15–27 CrossRef CAS.
- R. Yue, C. An, Z. Ye, X. Chen, K. Lee, K. Zhang, S. Wan and Z. Qu, Exploring the characteristics, performance, and mechanisms of a magnetic-mediated washing fluid for the cleanup of oiled beach sand, J. Hazard. Mater., 2022, 438, 129447 CrossRef CAS PubMed.
- R. Yue, Z. Ye, B. Zhang, C. An, Z. Qu and S. Wan, Two birds with one stone: A dual-functional washing fluid for shoreline oil spill response, ACS ES&T Eng., 2023, 4, 445–454 Search PubMed.
- X. Song, B. Zhang, Y. Cao, B. Liu and B. Chen, Shrimp-waste based dispersant as oil spill treating agent: Biodegradation of dispersant and dispersed oil, J. Hazard. Mater., 2022, 439, 129617 CrossRef CAS.
- J. Wei, G. Huang, C. An and H. Yu, Investigation on the solubilization of polycyclic aromatic hydrocarbons in the presence of single and mixed Gemini surfactants, J. Hazard. Mater., 2011, 190, 840–847 CrossRef CAS.
- A. L. Rodd, M. A. Creighton, C. A. Vaslet, J. R. Rangel-Mendez, R. H. Hurt and A. B. Kane, Effects of surface-engineered nanoparticle-based dispersants for marine oil spills on the model organism Artemia franciscana, Environ. Sci. Technol., 2014, 48, 6419–6427 CrossRef CAS.
- W. Ji, C. Abou-Khalil, M. Parameswarappa Jayalakshmamma, M. Boufadel and K. Lee, Post-formation of oil particle aggregates: Breakup and biodegradation, Environ. Sci. Technol., 2023, 57, 2341–2350 CrossRef CAS PubMed.
- G. Pi, Y. Li, M. Bao, L. Mao, H. Gong and Z. Wang, Novel and environmentally friendly oil spill dispersant based on the synergy of biopolymer xanthan gum and silica nanoparticles, ACS Sustainable Chem. Eng., 2016, 4, 3095–3102 CrossRef CAS.
- S. ben Hammouda, Z. Chen, C. An and K. Lee, Recent advances in developing cellulosic sorbent materials for oil spill cleanup: A state-of-the-art review, J. Cleaner Prod., 2021, 311, 127630 CrossRef.
- Y. Liu, J. Ma, T. Wu, X. Wang, G. Huang, Y. Liu, H. Qiu, Y. Li, W. Wang and J. Gao, Cost-effective reduced graphene oxide-coated polyurethane sponge as a highly efficient and reusable oil-absorbent, ACS Appl. Mater. Interfaces, 2013, 5, 10018–10026 CrossRef CAS.
- S. K. Chaerun, K. Tazaki, R. Asada and K. Kogure, Interaction between clay minerals and hydrocarbon-utilizing indigenous microorganisms in high concentrations of heavy oil: implications for bioremediation, Clay Miner., 2005, 40, 105–114 CrossRef CAS.
- U. C. Ugochukwu, M. D. Jones, I. M. Head, D. A. Manning and C. I. Fialips, Biodegradation and adsorption of crude oil hydrocarbons supported on “homoionic” montmorillonite clay minerals, Appl. Clay Sci., 2014, 87, 81–86 CrossRef CAS.
- T. Yu, L. T. Swientoniewski, M. Omarova, M.-C. Li, I. I. Negulescu, N. Jiang, O. A. Darvish, A. Panchal, D. A. Blake and Q. Wu, Investigation of amphiphilic polypeptoid-functionalized halloysite nanotubes as emulsion stabilizer for oil spill remediation, ACS Appl. Mater. Interfaces, 2019, 11, 27944–27953 CrossRef CAS.
- H. Cheng, Z. Li, Y. Li, Z. Shi, M. Bao, C. Han and Z. Wang, Multi-functional magnetic bacteria as efficient and economical Pickering emulsifiers for encapsulation and removal of oil from water, J. Colloid Interface Sci., 2020, 560, 349–358 CrossRef CAS.
|
This journal is © The Royal Society of Chemistry 2025 |
Click here to see how this site uses Cookies. View our privacy policy here.