DOI:
10.1039/C8RA00161H
(Paper)
RSC Adv., 2018,
8, 6306-6314
Design, synthesis, insecticidal activity and 3D-QSR study for novel trifluoromethyl pyridine derivatives containing an 1,3,4-oxadiazole moiety†
Received
6th January 2018
, Accepted 22nd January 2018
First published on 7th February 2018
Abstract
A series of trifluoromethyl pyridine derivatives containing 1,3,4-oxadiazole moiety was designed, synthesized and bio-assayed for their insecticidal activity. The result of bio-assays indicated the synthesized compounds exhibited good insecticidal activity against Mythimna separata and Plutella xylostella, most of the title compounds show 100% insecticidal activity at 500 mg L−1 and >80% activity at 250 mg L−1 against the two pests. Compounds E18 and E27 showed LC50 values of 38.5 and 30.8 mg L−1 against Mythimna separata, respectively, which were close to that of avermectin (29.6 mg L−1); compounds E5, E6, E9, E10, E15, E25, E26, and E27 showed 100% activity at 250 mg L−1, which were better than chlorpyrifos (87%). CoMFA and CoMSIA models with good predictability were proposed, which revealed the electron-withdrawing groups with an appropriate bulk at 2- and 4-positions of benzene ring could enhance insecticidal activity.
Introduction
Harmful insect pest in agriculture has brought about actual losses in productivity of crops all over the world annually.1 Take Mythimna separata Walker and Plutella xylostella as examples, they cause enormous economic losses in agricultural production worldwide.2,3 Currently, because of the generation of resistance and cross resistance, the insecticidal effect of phthaldiamides (such as fluobendiamide) and traditional organophosphorus pesticides was tapered.4–8 Thus, the development of insecticidal agent with novel structure is increasingly required.
Pesticide containing the fluorine is the hot topics in creating novel pesticides.9 As an important class of fluorinated heterocycle, the skeleton of trifluoromethyl pyridine showed a vital role in the discovery of pesticide molecules; so far, there are about 27 commercial pesticides with trifluoromethyl pyridine were commercialized, and five of them were used as insecticidal agent (Fig. 1).9 In recent years, studies for development of novel potential insecticidal molecules with the trifluoromethyl pyridine are very popular, and large number of potential compounds with this substructure were reported.10–16 Such as sulfoxaflor, a representative commercial insecticide, and was industrialized by Dow Agroscience.16
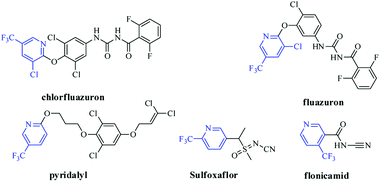 |
| Fig. 1 The commercial insecticide containing trifluoromethyl pyridine. | |
1,3,4-Oxadiazole derivative is a highly active pharmacophore and widely was used in pesticide molecules,17 many active substance containing such a scaffold have been reported as insecticides,18–24 fungicides,25–31 and herbicides,32–34 some of them were treated as a pesticide candidate for further industrialization.25 Recently, Liu and co-workers reported the 1,3,4-oxadiazole derivative with an anthranilic diamide moiety possessed excellent insecticidal activity against P. xylostella,20 as well as some insecticidal sarisan analogues with an 1,3,4-oxadiazole scaffold were developed by Guo et al.23,35 And in our recent work, a series of insecticidal 1,3,4-oxadiazole molecules bearing a 3-chloropyridin-2-yl-1H-pyrazole was proposed.36
Inspired by the description above, herein we attempted to replace 3-chloropyridin-2-yl-1H-pyrazole using a trifluorom-ethyl pyridine based on our previous work (Fig. 2),36 then structural variation at the other side of the 1,3,4-oxadiazole ring by the introduction of different substituted phenyl, resulting in series of trifluoromethyl pyridine derivatives containing an 1,3,4-oxadiazole moiety. Their insecticidal activity was evaluated, as well as the quantitative structure–activity relationship was analyzed based on CoMFA and CoMSIA. To the best of our knowledge, this is the first report on the insecticidal activity of 2-(trifluoromethyl pyridin-2-yl)-1,3,4-oxadiazole derivatives.
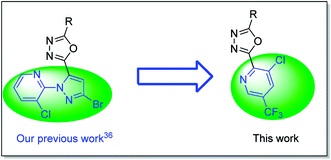 |
| Fig. 2 The design of title compound. | |
Results and discussion
Chemistry
The synthetic route for the title compounds is depicted in Scheme 1. The key intermediate ethyl substituted benzoate (B) was easily obtained in good yield via reactions of substituted benzoic acid with ethanol in the present of concentrated sulfuric acid, which further reacted with hydrazine hydrate (80%) to yield substituted benzohydrazide C in >90% yields.25,37 Subsequently, treatment of C with 3-chloro-5-(trifluoromethyl)picolinic acid (D) in the presence of phosphorus oxychloride at refluxing temperature afforded the title compounda (E) with excellent yield by employed known protocol.36
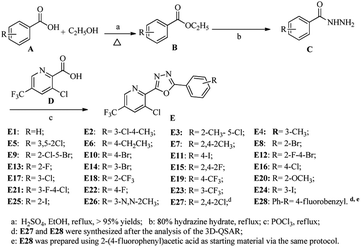 |
| Scheme 1 The synthetic routine of the title compounds E1–E28. | |
Structures of the compounds E1 to E28 were established on basis of 1H NMR, 19F NMR, 13C NMR and HR-MS data. In the 1H NMR spectra, the H proton near the “N” of pyridine appeared as a singlet near δ 8.95 ppm. As well as the proton at ortho-position of chlorine atom at pyridine appeared as a singlet near δ 8.20. In its 13C NMR spectra, the carbons near the group of “–CF3” were split into a quartet due to the coupling coefficients from “F”, take compound E1 as example, the carbon in “–CF3” group is near δC 122.21 ppm and the coupling constant (1JF−C) was 273.5 Hz, the carbon that is linked to group of “–CF3” resonance frequency is near δC 128.75 ppm as a quartet and with the coupling constant (2JC−F) is 34.1 Hz; and the carbons at ortho-position of “–CF3” were also split into very weak quartets with coupling constant (3JC−F) ranged from 3.6 to 3.8 Hz.
Insecticidal activity and 3D-QSAR study
The insecticidal activity of the synthesized compound against Mythimna separata (Walker) was carried out using reference method.38 The result listed in Table 1 revealed most of the synthesized compounds showed 100% activity against M. separata (Walker) at the 500 mg L−1. And the most of them displayed >80% activity at 250 mg L−1. The median lethal concentrations (LC50) values for all of the synthesized compounds were further investigated and also shown in Table 1. For demonstrating the effectiveness of evaluation, the LC50 value of commercial avermectin (a commonly used insecticide) was also evaluated as positive control. The results indicated the LC50 of synthesized compound was ranged from 38.0 mg L−1 to 284.6 mg L−1, in particularly, the LC50 values of compounds E18 and E27 were 38.5 and 30.8 mg L−1, respectively. And the LC50 value of E27 was the similar as that of avermectin (29.6 mg L−1). A preliminary SAR study indicated that the insecticidal activity can be impacted by the different type of groups on benzene ring, it could be concluded that the activity could be enhanced by introduction of the electron-withdrawing groups, and decreased by electron-donating group. For example, the activity of compounds with a methyl (E2, E3, E4, E7) and ethyl (E6) showed much lower activity than and the compounds with an electron-withdrawing group (such as E8, E18, E27), and the introduction of electronegative group could increase the insecticidal activity.
Table 1 Insecticidal activity of title compounds against Mythimna separata (Walker)
Compounds |
Concentrations/mg L−1 |
LC50/mg L−1 |
Y = a + bx |
r |
500 |
250 |
The compound was synthesized after the analysis of the 3D-QSAR. |
E1 |
90 |
30 |
284.6 |
Y = −5.68355 + 4.35314x |
0.99 |
E2 |
90 |
40 |
257.8 |
Y = −3.48605 + 3.519296x |
0.97 |
E3 |
100 |
87 |
87.1 |
Y = 0.9845 + 2.0696x |
0.98 |
E4 |
90 |
45 |
195 |
Y = −0.79654 + 2.5306x |
0.97 |
E5 |
100 |
100 |
49.9 |
Y = 0.50982 + 2.64453x |
0.99 |
E6 |
100 |
90 |
94.4 |
Y = 0.2253 + 2.41787x |
0.96 |
E7 |
100 |
86 |
85.6 |
Y = 0.58869 + 2.282861x |
0.99 |
E8 |
100 |
87 |
77.5 |
Y = 1.680326 + 1.756857x |
0.99 |
E9 |
100 |
93 |
66.2 |
Y = 0.9206 + 2.24037x |
0.98 |
E10 |
100 |
80 |
70.2 |
Y = −0.012067 + 2.71443x |
0.98 |
E11 |
100 |
97 |
100.5 |
Y = 0.6878 + 2.153806x |
0.99 |
E12 |
100 |
93 |
61.4 |
Y = 0.9228 + 2.27983x |
0.97 |
E13 |
100 |
100 |
42.7 |
Y = 1.59716 + 2.08747x |
0.99 |
E14 |
100 |
93 |
65.6 |
Y = 0.887681 + 2.263608x |
0.99 |
E15 |
100 |
95 |
53.9 |
Y = 0.515867 + 2.589976x |
0.99 |
E16 |
100 |
95 |
61.3 |
Y = 1.460812 + 1.9793x |
0.95 |
E17 |
100 |
90 |
61.6 |
Y = 1.531383 + 1.938511x |
0.99 |
E18 |
100 |
93 |
38.5 |
Y = 1.890028 + 1.962144x |
0.99 |
E19 |
100 |
80 |
58.9 |
Y = 2.567415 + 1.373985x |
0.99 |
E20 |
100 |
90 |
73.7 |
Y = 0.940985 + 2.173735x |
0.98 |
E21 |
100 |
88 |
55.1 |
Y = 1.624982 + 1.938583x |
0.99 |
E22 |
100 |
87 |
79.6 |
Y = 0.850476 + 2.183056x |
0.98 |
E23 |
100 |
87 |
71.2 |
Y = 1.34511 + 1.97326x |
0.98 |
E24 |
100 |
90 |
98.9 |
Y = −1.01199 + 3.01345x |
0.99 |
E25 |
100 |
90 |
83.7 |
Y = −0.09434 + 2.6496x |
0.99 |
E26 |
100 |
93 |
57.6 |
Y = 1.332377 + 2.082927x |
0.99 |
E27a |
100 |
100 |
30.8 |
Y = 2.419324 + 1.699131x |
0.99 |
E28a |
90 |
65 |
128.9 |
Y = 0.82303 + 1.97925x |
0.99 |
Avermectin |
100 |
100 |
29.6 |
Y = 1.86578 + 2.130825x |
0.96 |
In order to give more information of structure–activity relationship, 3D-QSAR models (CoMFA and CoMSIA) were further built based on the active data of M. separata (Walker). Six compounds (E7, E8, E9, E16, E22 and E26) were randomly chosen as a test set. The training set consisted of 20 compounds. The superimposition of training set compounds was done by using compounds E20 as a template and had shown in ESI (Fig. 1S†).
The results of the PLS statistics tested on bioactivity against M. separata are presented in Table S1 (see ESI†). In result of CoMFA, the cross-validated q2 value was 0.742 with five components, and the non-crossvalidated conventional r2 value was 0.975 with a standard error of estimation (SEE) of 0.044 and F = 92.362. The relative contributions between steric and electrostatic fields for the CoMFA model were 0.586 and 0.414, respectively. As for CoMSIA, as well as the q2 value and optimum number of components were 0.614 and 6, respectively. And the r2 value, SEE, and F value were 0.92, 0.084, and 32.793, respectively. And the CoMSIA result revealed the contributions of steric, electrostatic hydrophobic, hydrogen-bond donor and acceptor fields were 0.511, 0.489, 0, 0, and 0, respectively. These results indicated that both CoMFA and CoMSIA models showed good predictability. As well as the two models revealed that both the steric and electrostatic field made an important contribution to bioactivity, and steric field show a little priority than electrostatic field.
The PLC50 values of the training set compounds and test set compounds were predicted by the two models (more details can be found in ESI, Table S2†). And the correlations between the predicted PLC50 and experimental PLC50 are represented in Fig. 3. The result indicated the proposed model predicted the activity successfully.
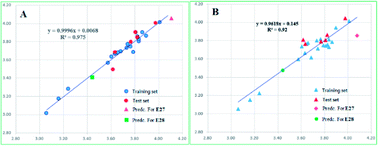 |
| Fig. 3 Scatter plots between experimental activities and predicted activities for CoMFA (A) and CoMSIA (B). | |
The steric contour map of CoMFA is shown in Fig. 4(A and B). The green contours near the 2- and 4-positions of benzene ring revealed a large substituent is necessary for contributing to the anti-insecticidal activity, however, the yellow contours near the two positions indicated a bulky substituent could be decreased the activity, for instance, the activity of compound E6 (with ethyl at 4-position of benzene and LC50 = 94.4 mg L−1), and E20 (with ethyl at 2-position of benzene and LC50 = 73.7 mg L−1) was much low than E22 (LC50 = 79.6 mg L−1) and E8 (LC50 = 42.7 mg L−1), thus an appropriate size of substituent should be considered near the two positions, from the LC50 values of the compounds, we speculated a chlorine at 4-position of benzene (E16, LC50 = 61.3 mg L−1) is much more suitable than other halogen atoms. Moreover, in electrostatic contour map (Fig. 4C and D), the blue contour indicated that negative charge near the benzene ring (2–5 positions) is favored by the activity. Hence, the electron withdrawing group is in favor of improving the insecticidal activity, the activity for the compounds with an electron-donating group is much lower than these compounds containing an electron withdrawing group (e.g.: E4 < E23, E24 et al.; E6 < E22, E7 < E12).
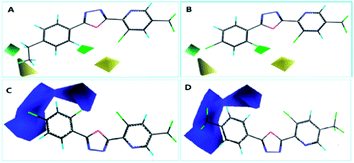 |
| Fig. 4 The contour map of CoMFA. (A) Steric contour map with compound E6, (B) steric contour map with compound E12; (C) electrostatic contour map with compound E12, (D) electrostatic contour map with compound E19. | |
The CoMSIA contour map was shown in Fig. 5, the steric and electrostatic contour map (Fig. 5A and B) revealed the similar regularity as CoMFA shown. And furthermore, the electrostatic contour map (Fig. 5C and D) give a necessary supplement for the electrostatic contour map of CoMFA, from the result of CoMSIA, we can see that the electronegative groups around the benzene is necessary, but an appropriate group at 3-position of benzene should be taken into account, or else the activity was be decreased (e.g.: the LC50 value of compound E2 is 257.8 mg L−1). Moreover, the predictability of the 3D-QSAR models were investigated by the new structures with two chlorine atoms (E27) and a 4-fluorobenzyl (E28), which were synthesized and tested for their insecticidal activities, the results showed in Table S2† indicated the models showed good predictability.
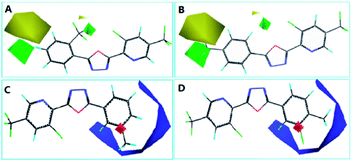 |
| Fig. 5 The contour map of CoMSIA. (A) Steric contour map with compound E18, (B) steric contour map with compound E6; (C) electrostatic contour map with compound E4, (D) electrostatic contour map with compound E2. | |
The insecticidal activity of the synthesized compounds against Plutella xylostella, Nilaparvata lugens and Aphis craccivora Koch were carried out using reference method.39,40 The results listed in Table 2 indicated that some of the synthesized compounds showed certain insecticidal activity against Nilaparvata lugens and Aphis craccivora Koch at 500 mg L−1, the activity of compounds E14, E15 and E18 was medium. However, the synthesized compounds showed good insecticidal against Plutella xylostella. Most of them showed 100% activity at 500 mg L−1 and >80% at 250 mg L−1, and in particularly, compounds E5, E6, E9, E10, E15, E25, E26, and E27 showed 100% activity, which were better than chlorpyrifos (87%). The preliminary SAR analysis show similar trends as that of M. separata.
Table 2 Insecticidal activity of title compounds against P. xylostella, N. lugens, and A. craccivor
Compounds |
P. xylostella |
N. lugens |
A. craccivora |
500 mg L−1 |
250 mg L−1 |
500 mg L−1 |
500 mg L−1 |
E1 |
100 |
63 |
20 |
20 |
E2 |
100 |
60 |
50 |
10 |
E3 |
100 |
60 |
30 |
50 |
E4 |
100 |
87 |
40 |
10 |
E5 |
100 |
100 |
20 |
40 |
E6 |
100 |
100 |
20 |
10 |
E7 |
100 |
80 |
50 |
40 |
E8 |
100 |
90 |
45 |
44 |
E9 |
100 |
100 |
63 |
55 |
E10 |
100 |
100 |
20 |
20 |
E11 |
100 |
93 |
30 |
30 |
E12 |
100 |
97 |
32 |
42 |
E13 |
100 |
90 |
10 |
60 |
E14 |
100 |
93 |
50 |
50 |
E15 |
100 |
100 |
60 |
65 |
E16 |
100 |
67 |
10 |
40 |
E17 |
100 |
87 |
14 |
44 |
E18 |
100 |
93 |
54 |
54 |
E19 |
100 |
90 |
23 |
0 |
E20 |
100 |
85 |
15 |
0 |
E21 |
96 |
70 |
13 |
15 |
E22 |
87 |
90 |
29 |
35 |
E23 |
100 |
97 |
24 |
40 |
E24 |
100 |
93 |
30 |
40 |
E25 |
100 |
100 |
30 |
23 |
E26 |
100 |
100 |
15 |
41 |
E27 |
100 |
100 |
23 |
43 |
E28 |
100 |
93 |
23 |
34 |
Chlorpyrifos |
100 |
87 |
— |
— |
Imidacloprid |
— |
— |
100 |
100 |
Conclusions
In current work, a new series of 1,3,4-oxadiazole derivatives containing trifluoromethyl pyridine moiety was designed and synthesized. The structures of these compounds were characterized and confirmed by 1H NMR, 19F NMR, 13C NMR, HR-MS. Bioassays for insecticidal activity of the synthesized compounds against M. separata, P. xylostella, N. lugens, and A. craccivora were conducted. The results indicated some of the synthesized compounds exhibited good insecticidal activity against M. separata (with LC50 values ranged from 30.8 mg L−1 to 284.6 mg L−1) and P. xylostella (most of them showed 100% activity at 500 mg L−1 and >80% at 250 mg L−1). In particularly, the LC50 value of compounds E18 and E27 was 38.5 and 30.8 mg L−1, respectively. CoMFA and CoMSIA models were built, the models exhibited good correlation, predictability, and revealed the activity could be enhanced by introduction of the electron-withdrawing groups with proper bulk at 2- and 4-positions of benzene ring. The proposed models could predict the activity with reasonable accuracy and will be useful in the near future for finding potent insecticidal molecules in our group.
Experimental section
Materials and methods
All aromatic acids were purchased from Accela Chem-Bio Co., Ltd (Shanghai, China) and Inno-chem Co., Ltd (Beijing, China). Melting points of the synthesized compounds were measured using a XT-4 binocular microscope (Beijing Tech Instrument Co., China). 1H, 19F and 13C NMR spectra were recorded on a JEOL ECX 500 NMR (JEOL Ltd., Japan) or AVANCE III HD 400M NMR (Bruker Corporation, Switzerland) spectrometer operating at room temperature using CDCl3 or DMSO as solvents. HR-MS was recorded on an Orbitrap LC-MS instrument (Q-Exative, Thermo Scientific™, and American). The course of the reactions was monitored by TLC.
Synthetic procedures
Synthesis of substituted benzoyl hydrazine (C). Substituted benzoyl hydrazine (C) was prepared by following the known procedure.25 Substituted benzoic acid or para-fluorophenylacetic acid (for preparation of compound E28) as starting materials, followed by esterification and hydrazinolysis to yield substituted 2-phenoxyacetohydrazide in excellent yields.37
Synthesis of title compounds (E1 to E28)36. The mixture of substituted 2-phenoxyacetohydrazide C (0.5 mmol), 3-chloro-5-(trifluoromethyl)picolinic acid (0.5 mmol) and phosphorus oxychloride (3 mL) was stirred for 8 h under refluxing temperature, the resulted mixture was the poured to crushed ice and neutralized with 5% NaOH, the precipitate was then filtered and purification using silica gel (200–300 mesh) column chromatography with using ethyl acetate/petroleum ether (1
:
5). The melting point, yield, IR, 1H NMR, 13C NMR and HR-MS data for compound E1 are listed below, and those for compounds E2–E28 can be found in the ESI.†
2-(3-Chloro-5-(trifluoromethyl)pyridin-2-yl)-5-phenyl-1,3,4-oxadiazole (E1). Yield 58.2%; light yellow solid; mp 104.2–105.4 °C; 1H NMR (500 MHz, chloroform-D) δ 8.96 (d, J = 1.0 Hz, 1H, pyridine-H), 8.23–8.21 (m, 1H, pyridine-H), 8.20 (dt, J = 3.6, 1.9 Hz, 2H, Ph-H), 7.61–7.53 (m, 3H, Ph-H); 19F NMR (471 MHz, chloroform-D) δ −62.41; 13C NMR (125 MHz, chloroform-D) δ 165.84, 161.23, 144.66 (q, J = 3.8 Hz), 144.09, 136.76 (q, J = 3.6 Hz), 132.57, 132.18, 129.30, 128.75 (q, J = 34.1 Hz), 127.60, 123.25, 122.21 (q, J = 273.5 Hz); HR-MS (ESI): calculated for C14H7ClF3N3O [M + H]+: 326.03025, found: 326.02969.
2-(3-Chloro-4-methylphenyl)-5-(3-chloro-5-(trifluoromethyl)pyridin-2-yl)-1,3,4-oxadiazole (E2). Yield 48.3%; light yellow solid; mp 94.0–96.2 °C; 1H NMR (500 MHz, chloroform-D) δ 8.96 (s, 1H, pyridine-H), 8.19 (d, J = 9.1 Hz, 2H, pyridine-H), 8.00 (d, J = 7.9 Hz, 1H, Ph-H), 7.41 (d, J = 7.9 Hz, 1H, Ph-H), 2.47 (s, 3H, –CH3); 19F NMR (471 MHz, chloroform-D) δ −62.43; 13C NMR (125 MHz, chloroform-D) δ 164.80, 161.22, 144.63 (d, J = 3.5 Hz), 143.93, 141.21, 136.76 (d, J = 3.3 Hz), 135.49, 132.23, 131.81, 128.80 (q, J = 34.2 Hz), 127.88, 125.65, 122.18 (q, J = 273.5 Hz), 122.31, 20.49; HR-MS (ESI): calculated for C15H8Cl2F3N3O [M + H]+: 374.00693, found: 374.00598.
2-(5-Chloro-2-methylphenyl)-5-(3-chloro-5-(trifluoromethyl)pyridin-2-yl)-1,3,4-oxadiazole (E3). Yield 29.0%; light yellow solid; mp 128.4–131.5 °C; 1H NMR (500 MHz, chloroform-D) δ 8.97 (s, 1H, pyridine-H), 8.20 (s, 1H, pyridine-H), 8.09 (s, 1H, Ph-H), 7.43 (d, J = 8.3 Hz, 1H, Ph-H), 7.33 (d, J = 8.1 Hz, 1H, Ph-H), 2.77 (s, 3H, –CH3); 19F NMR (471 MHz, chloroform-D) δ −62.43; 13C NMR (125 MHz, chloroform-D) δ 164.94, 160.99, 144.72 (d, J = 3.5 Hz), 143.98, 137.62, 136.75 (d, J = 3.3 Hz), 133.40, 132.31, 132.23, 131.88, 129.11, 128.90 (q, J = 34.2 Hz), 123.74, 122.19 (q, J = 273.8 Hz), 21.84; HR-MS (ESI): calculated for C15H8Cl2F3N3O [M + H]+: 374.00693, found: 374.00607.
2-(3-Chloro-5-(trifluoromethyl)pyridin-2-yl)-5-(m-tolyl)-1,3,4-oxadiazole (E4). Yield 61.1%; light red solid; mp 75.4–76.6 °C; 1H NMR (500 MHz, chloroform-D) δ 8.96 (dd, J = 1.7, 0.7 Hz, 1H, pyridine-H), 8.19 (dd, J = 1.8, 0.6 Hz, 1H, pyridine-H), 8.01 (ddd, J = 8.7, 4.4, 0.9 Hz, 2H, Ph-H), 7.41 (dt, J = 15.2, 4.0 Hz, 2H, Ph-H), 2.46 (s, 3H–CH3); 19F NMR (471 MHz, chloroform-D) δ −62.42; 13C NMR (125 MHz, chloroform-D) δ 166.00, 161.15, 144.64 (d, J = 3.5 Hz), 144.14, 139.24, 136.74 (q, J = 2.9 Hz), 133.39, 132.15, 129.19, 128.71 (q, J = 34.1 Hz), 128.03, 124.78, 123.11, 122.22 (q, J = 273.6 Hz), 21.43; HR-MS (ESI): calculated for C15H9ClF3N3O [M + H]+: 340.04590, found: 340.04696.
2-(3-Chloro-5-(trifluoromethyl)pyridin-2-yl)-5-(3,5-dichlorophenyl)-1,3,4-oxadiazole (E5). Yield 32.0%; light gray solid; mp 115.9–117.1 °C; 1H NMR (500 MHz, chloroform-D) δ 8.97 (s, 1H, pyridine-H), 8.21 (d, J = 1.1 Hz, 1H, pyridine-H), 8.10 (d, J = 2.0 Hz, 2H, Ph-H), 7.58 (t, J = 1.9 Hz, 1H, Ph-H); 19F NMR (471 MHz, chloroform-D) δ −62.46; 13C NMR (125 MHz, chloroform-D) δ 163.64, 161.66, 144.70 (d, J = 3.7 Hz), 143.66, 136.83 (d, J = 3.3 Hz), 136.31, 132.47, 132.41, 129.09 (q, J = 34.5 Hz), 125.89, 125.75, 122.13 (q, J = 273.6 Hz); HR-MS (ESI): calculated for C14H5Cl3F3N3O [M + H]+: 393.95231, found: 393.95306.
2-(3-Chloro-5-(trifluoromethyl)pyridin-2-yl)-5-(4-ethylphenyl)-1,3,4-oxadiazole (E6). Yield 66.9%; yellow solid; mp 88.0–89.2 °C; 1H NMR (500 MHz, chloroform-D) δ 8.98–8.89 (m, 1H, pyridine-H), 8.17 (dd, J = 1.3, 0.6 Hz, 1H, pyridine-H), 8.10 (d, J = 8.2 Hz, 2H, Ph-H), 7.36 (d, J = 8.1 Hz, 2H, Ph-H), 2.73 (q, J = 7.6 Hz, 2H, –CH2–), 1.27 (t, J = 7.6 Hz, 3H, –CH3); 19F NMR (471 MHz, chloroform-D) δ −62.44; 13C NMR (125 MHz, chloroform-D) δ 165.97, 160.99, 149.42, 144.59 (d, J = 3.6 Hz), 144.19, 136.67 (d, J = 3.3 Hz), 132.08, 128.78, 128.63 (q, J = 34.1 Hz), 127.64, 122.23 (q, J = 273.4 Hz), 120.67, 29.08, 15.23; HR-MS (ESI): calculated for C16H11ClF3N3O [M + H]+: 354.06155, found: 354.06137.
2-(3-Chloro-5-(trifluoromethyl)pyridin-2-yl)-5-(2,4-dimethyl phenyl)-1,3,4-oxadiazole (E7). Yield 63.8%; white solid; mp 86.5–87.7 °C; 1H NMR (500 MHz, chloroform-D) δ 8.95 (dd, J = 1.9, 0.7 Hz, 1H, pyridine-H), 8.19–8.16 (m, 1H, pyridine-H), 7.99 (d, J = 7.9 Hz, 1H, Ph-H), 7.16 (dd, J = 13.6, 5.5 Hz, 3H-phenyl), 2.75 (s, 3H, –CH3), 2.40 (s, 3H, –CH3); 19F NMR (471 MHz, chloroform-D) δ −62.43; 13C NMR (125 MHz, chloroform-D) δ 166.25, 160.60, 144.63 (d, J = 3.9 Hz), 144.30, 142.53, 139.03, 136.64 (d, J = 3.4 Hz), 132.76, 132.07, 129.56, 128.59 (q, J = 34.3 Hz), 127.18, 122.25 (q, J = 273.5 Hz), 119.57, 22.21, 21.55; HR-MS (ESI): calculated for C16H11ClF3N3O [M + H]+: 354.06155, found: 354.06128.
2-(2-Bromophenyl)-5-(3-chloro-5-(trifluoromethyl)pyridin-2-yl)-1,3,4-oxadiazole (E8). Yield 46.8%; light yellow solid; mp 75.7–77.0 °C; 1H NMR (500 MHz, chloroform-D) δ 8.96 (s, 1H, pyridine-H), 8.20 (s, 1H, pyridine-H), 8.05 (dd, J = 7.8, 1.3 Hz, 1H, Ph-H), 7.79 (d, J = 8.0 Hz, 1H, Ph-H), 7.50 (dd, J = 11.8, 4.4 Hz, 1H, Ph-H), 7.43 (td, J = 7.8, 1.4 Hz, 1H, Ph-H); 19F NMR (471 MHz, chloroform-D) δ −62.44; 13C NMR (125 MHz, chloroform-D) δ 164.74, 161.66, 144.75 (d, J = 3.6 Hz), 144.01, 136.75 (d, J = 3.3 Hz), 134.78, 133.14, 132.35, 132.12, 128.92 (q, J = 34.3 Hz), 127.79, 124.76, 122.19 (q, J = 273.5 Hz), 122.12; HR-MS (ESI): calculated for C14H6ClF4N3O [M + H]+: 344.02083, found: 344.02029.
2-(5-Bromo-2-chlorophenyl)-5-(3-chloro-5-(trifluoromethyl)pyridin-2-yl)-1,3,4-oxadiazole (E9). Yield 55.5%; yellow solid; mp 99.4–100.6 °C; 1H NMR (500 MHz, chloroform-D) δ 8.96 (d, J = 1.0 Hz, 1H, pyridine-H), 8.26 (d, J = 2.3 Hz, 1H, pyridine-H), 8.20 (d, J = 1.6 Hz, 1H, Ph-H), 7.62 (dd, J = 8.6, 2.5 Hz, 1H, Ph-H), 7.45 (d, J = 8.6 Hz, 1H, Ph-H); 19F NMR (471 MHz, chloroform-D) δ −62.44; 13C NMR (125 MHz, chloroform-D) δ 162.99, 161.77, 144.78 (d, J = 3.4 Hz), 143.79, 136.80 (d, J = 2.9 Hz), 135.97, 134.05, 132.88, 132.64, 132.47, 129.07 (q, J = 34.1 Hz), 124.16, 122.15 (q, J = 273.7 Hz), 120.86; HR-MS (ESI): calculated for C14H5BrCl2F3N3O [M + H]+: 437.90179, found: 437.90149.
2-(3-Chloro-5-(trifluoromethyl)pyridin-2-yl)-5-(4-iodophenyl)-1,3,4-oxadiazole (E10). Yield 48.0%; yellow solid; mp 153.0–153.7 °C; 1H NMR (500 MHz, chloroform-D) δ 8.95 (s, 1H, pyridine-H), 8.20 (s, 1H, pyridine-H), 7.92 (d, J = 0.5 Hz, 4H, Ph-H); 19F NMR (471 MHz, chloroform-D) δ −62.44; 13C NMR (125 MHz, chloroform-D) δ 165.29, 161.33, 144.66 (d, J = 3.5 Hz), 143.89, 138.62, 136.80 (d, J = 3.3 Hz), 132.28, 128.87 (q, J = 34.3 Hz), 128.83, 122.67, 122.17 (q, J = 273.5 Hz), 99.76; HR-MS (ESI): calculated for C14H6ClF3IN3O [M + H]+: 451.92689, found: 451.92648.
2-(4-Bromophenyl)-5-(3-chloro-5-(trifluoromethyl)pyridin-2-yl)-1,3,4-oxadiazole (E11). Yield 37.9%; light yellow solid; mp 151.2–154.9 °C; 1H NMR (500 MHz, chloroform-D) δ 8.95 (d, J = 0.6 Hz, 1H, pyridine-H), 8.21–8.18 (m, 1H, pyridine-H), 8.08 (dd, J = 8.3, 0.5 Hz, 2H, Ph-H), 7.70 (dd, J = 7.1, 1.2 Hz, 2H, Ph-H); 19F NMR (471 MHz, chloroform-D) δ −62.45; 13C NMR (125 MHz, chloroform-D) δ 165.08, 161.31, 144.63 (d, J = 3.5 Hz), 143.91, 136.75 (d, J = 3.3 Hz), 132.66, 132.26, 128.92, 128.81 (q, J = 27.1 Hz), 127.40, 122.18 (q, J = 273.7 Hz), 122.17; HR-MS (ESI): calculated for C14H6BrClF3N3O [M + H]+: 403.94076, found: 403.94077.
2-(4-Bromo-2-fluorophenyl)-5-(3-chloro-5-(trifluoromethyl)pyridin-2-yl)-1,3,4-oxadiazole (E12). Yield 10.9%; light yellow solid; mp 105.9–107.1 °C; 1H NMR (500 MHz, chloroform-D) δ 8.97 (t, J = 5.9 Hz, 1H, pyridine-H), 8.21 (t, J = 5.7 Hz, 1H, pyridine-H), 8.09 (t, J = 7.8 Hz, 1H, Ph-H), 7.51 (d, J = 8.6 Hz, 2H, Ph-H); 19F NMR (471 MHz, chloroform-D) δ −62.47, −106.35; 13C NMR (125 MHz, chloroform-D) δ 162.04 (d, J = 4.8 Hz), 161.57, 159.97 (d, J = 264.2 Hz), 144.75 (d, J = 3.7 Hz), 143.86, 136.75 (d, J = 3.2 Hz), 132.41, 130.99, 128.99 (d, J = 34.1 Hz), 128.55 (d, J = 3.5 Hz), 127.75 (d, J = 9.3 Hz), 124.34 (d, J = 274.4 Hz), 121.01 (d, J = 23.8 Hz), 113.43, 111.02 (d, J = 11.4 Hz); HR-MS (ESI): calculated for C14H5BrClF4N3O [M + H]+: 421.93134, found: 421.93088.
2-(3-Chloro-5-(trifluoromethyl)pyridin-2-yl)-5-(2-fluorophenyl)-1,3,4-oxadiazole (E13). Yield 34.1%; light red solid; mp 83.6–84.8 °C; 1H NMR (500 MHz, chloroform-D) δ 8.96 (d, J = 1.0 Hz, 1H, pyridine-H), 8.21 (dd, J = 7.5, 1.5 Hz, 1H, pyridine-H), 8.19 (d, J = 1.8 Hz, 1H, Ph-H), 7.63–7.55 (m, 1H, Ph-H), 7.37–7.27 (m, 2H, Ph-H); 19F NMR (471 MHz, chloroform-D) δ −62.47, −108.87; 13C NMR (125 MHz, chloroform-D) δ 162.66 (d, J = 4.8 Hz), 161.44, 160.42 (d, J = 262.9 Hz), 144.72 (d, J = 3.6 Hz), 144.03, 136.70 (d, J = 3.3 Hz), 134.34 (d, J = 8.5 Hz), 132.32, 130.27, 128.87 (q, J = 34.0 Hz), 124.89 (d, J = 3.5 Hz), 122.19 (q, J = 273.5 Hz), 117.21 (d, J = 20.7 Hz), 111.88 (d, J = 11.3 Hz); HR-MS (ESI): calculated for C14H6ClF4N3O [M + H]+: 344.02083, found: 344.02042.
2-(3-Bromophenyl)-5-(3-chloro-5-(trifluoromethyl)pyridin-2-yl)-1,3,4-oxadiazole (E14). Yield 42.4%; light gray solid; mp 107.8–108.9 °C; 1H NMR (500 MHz, chloroform-D) δ 8.96 (d, J = 1.1 Hz, 1H, pyridine-H), 8.35 (t, J = 1.7 Hz, 1H, pyridine-H), 8.22–8.19 (m, 1H, Ph-H), 8.17–8.14 (m, 1H, Ph-H), 7.73 (ddd, J = 8.0, 1.8, 1.0 Hz, 1H, Ph-H), 7.44 (t, J = 7.9 Hz, 1H, Ph-H); 19F NMR (471 MHz, chloroform-D) δ −62.44; 13C NMR (125 MHz, chloroform-D) δ 164.50, 161.44, 144.67 (d, J = 3.8 Hz), 143.86, 136.78 (d, J = 3.4 Hz), 135.51, 132.33, 130.84, 130.32, 128.92 (q, J = 34.4 Hz), 126.11, 125.09, 123.33, 122.17 (q, J = 273.4 Hz); HR-MS (ESI): calculated for C14H6BrClF3N3O [M + H]+: 403.94076, found: 403.94049.
2-(3-Chloro-5-(trifluoromethyl)pyridin-2-yl)-5-(3,4-dichlorophenyl)-1,3,4-oxadiazole (E15). Yield 36.6%; gray solid; mp 142.6–143.7 °C; 1H NMR (500 MHz, chloroform-D) δ 8.97–8.93 (m, 1H, pyridine-H), 8.29 (d, J = 2.0 Hz, 1H, pyridine-H), 8.21 (d, J = 1.3 Hz, 1H, Ph-H), 8.05 (dd, J = 8.4, 2.0 Hz, 1H, Ph-H), 7.64 (d, J = 8.4 Hz, 1H, Ph-H); 19F NMR (471 MHz, chloroform-D) δ −62.43; 13C NMR (125 MHz, chloroform-D) δ 164.02, 161.52, 144.68, 143.69, 137.20, 136.85, 134.03, 132.40, 131.53, 129.20, 129.00 (q, J = 273.5 Hz), 126.55, 123.00, 121.05; HR-MS (ESI): calculated for C14H5Cl3F3N3O [M + H]+: 393.95231, found: 393.95209.
2-(3-Chloro-5-(trifluoromethyl)pyridin-2-yl)-5-(4-chlorophenyl)-1,3,4-oxadiazole (E16). Yield 17.5%; white solid; mp 169.8–170.3 °C; 1H NMR (500 MHz, chloroform-D) δ 8.94 (s, 1H, pyridine-H), 8.19 (s, 1H, pyridine-H), 8.13 (d, J = 8.4 Hz, 2H, Ph-H), 7.52 (d, J = 8.4 Hz, 2H, Ph-H); 13C NMR (125 MHz, chloroform-D) δ 164.99, 161.29, 144.64 (d, J = 3.6 Hz), 143.90, 138.93, 136.77 (d, J = 3.1 Hz), 132.25, 129.70, 128.84 (q, J = 34.0 Hz), 128.82, 122.17 (d, J = 273.4 Hz), 121.72; HR-MS (ESI): calculated for C14H6Cl2F3N3O [M + H]+: 359.99128, found: 359.99078.
2-(3-Chloro-5-(trifluoromethyl)pyridin-2-yl)-5-(3-chlorophenyl)-1,3,4-oxadiazole (E17). Yield 40.6%; pink solid; mp 93.6–94.8 °C; 1H NMR (500 MHz, chloroform-D) δ 8.96 (s, 1H, pyridine-H), 8.20 (d, J = 0.9 Hz, 1H, pyridine-H), 8.20–8.18 (m, 1H, Ph-H), 8.13–8.08 (m, 1H, Ph-H), 7.58–7.55 (m, 1H, Ph-H), 7.50 (t, J = 7.9 Hz, 1H, Ph-H); 19F NMR (471 MHz, chloroform-D) δ −62.43; 13C NMR (125 MHz, chloroform-D) δ 164.67, 161.45, 144.67, 143.85, 136.80, 135.49, 132.61, 132.33, 130.67, 128.93 (q, J = 33.9 Hz), 127.48, 125.69, 124.87, 122.16 (q, J = 273.5 Hz); HR-MS (ESI): calculated for C14H6Cl2F3N3O [M + H]+: 359.99128, found: 359.99078.
2-(3-Chloro-5-(trifluoromethyl)pyridin-2-yl)-5-(2-(trifluoromethyl)phenyl)-1,3,4-oxadiazole (E18). Yield 55.0%; yellow solid; mp 60.8–61.5 °C; 1H NMR (500 MHz, chloroform-D) δ 8.96 (s, 1H, pyridine-H), 8.20 (s, 1H, pyridine-H), 8.15–8.12 (m, 1H, Ph-H), 7.93–7.88 (m, 1H, Ph-H), 7.77–7.74 (m, 2H, Ph-H); 19F NMR (471 MHz, chloroform-D) δ −59.71, −62.52; 13C NMR (125 MHz, chloroform-D) δ 164.14, 162.29, 144.77 (d, J = 3.9 Hz), 143.91, 136.71 (d, J = 3.4 Hz), 132.40, 132.31, 132.22, 129.38 (q, J = 32.6 Hz), 129.02 (q, J = 33.9 Hz), 127.26 (q, J = 5.0 Hz), 123.17 (q, J = 273.6 Hz), 121.74, 122.17 (q, J = 273.5 Hz); HR-MS (ESI): calculated for C15H6ClF6N3O [M + H]+: 394.01764, found: 394.01730.
2-(3-Chloro-5-(trifluoromethyl)pyridin-2-yl)-5-(4-(trifluoromethyl)phenyl)-1,3,4-oxadiazole (E19). Yield 45.0%; light yellow solid; mp 109.9–111.0 °C; 1H NMR (500 MHz, chloroform-D) δ 8.97 (s, 1H, pyridine-H), 8.35 (s, 1H, pyridine-H), 8.34 (s, 1H, Ph-H), 8.21 (s, 1H, Ph-H), 7.83 (d, J = 8.2 Hz, 2H, Ph-H); 19F NMR (471 MHz, chloroform-D) δ −62.47, −63.10; 13C NMR (125 MHz, chloroform-D) δ 164.59, 161.58, δ 144.69 (d, J = 3.7 Hz), 143.79, δ 136.81 (d, J = 3.4 Hz), δ 134.09 (q, J = 33.2 Hz), 132.42, δ 129.02 (q, J = 34.4 Hz), 127.92, 126.51, δ 126.33 (d, J = 3.6 Hz), δ 123.55 (q, J = 272.6 Hz), δ 122.15 (q, J = 273.5 Hz); HR-MS (ESI): calculated for C15H6ClF6N3O [M + H]+: 394.01764, found: 394.01740.
2-(3-Chloro-5-(trifluoromethyl)pyridin-2-yl)-5-(2-methoxyphenyl)-1,3,4-oxadiazole (E20). Yield 70.3%; purple solid; mp 67.0–68.2 °C; 1H NMR (500 MHz, chloroform-D) δ 8.95 (d, J = 0.7 Hz, 1H, pyridine-H), 8.18 (s, 1H, pyridine-H), 8.07 (d, J = 6.6 Hz, 1H, Ph-H), 7.57–7.52 (m, 1H, Ph-H), 7.09 (dd, J = 14.3, 7.8 Hz, 2H, Ph-H), 3.99 (s, 3H–CH3); 19F NMR (471 MHz, chloroform-D) δ −62.42; 13C NMR (125 MHz, chloroform-D) δ 164.70, 161.01, 158.34, 144.63, 144.36, 136.65, 133.88, 132.11, 131.01, 128.60 (q, J = 34.5 Hz), 122.25 (q, J = 272.8 Hz), 120.92, 112.36, 112.08, 56.17; HR-MS (ESI): calculated for C15H9ClF3N3O2 [M + H]+: 355.03299, found: 355.03272.
2-(4-Chloro-3-fluorophenyl)-5-(3-chloro-5-(trifluoromethyl)pyridin-2-yl)-1,3,4-oxadiazole (E21). Yield 23.9%; light purple solid; mp 140.7–141.7 °C; 1H NMR (500 MHz, chloroform-D) δ 8.96 (s, 1H, pyridine-H), 8.21 (s, 1H, pyridine-H), 8.02–7.95 (m, 2H, Ph-H), 7.61 (t, J = 7.7 Hz, 1H, Ph-H); 19F NMR (471 MHz, chloroform-D) δ −62.46, −112.35; 13C NMR (125 MHz, chloroform-D) δ 164.10 (d, J = 2.1 Hz), 161.50, 158.45 (d, J = 251.2 Hz), 144.68 (d, J = 3.6 Hz), 143.73, 136.81 (d, J = 3.4 Hz), 132.38, 131.88, 129.00 (q, J = 34.4 Hz), 125.93 (d, J = 17.8 Hz), 123.91 (d, J = 3.7 Hz), 123.39 (d, J = 7.5 Hz), 122.15 (q, J = 273.6 Hz), 115.64 (d, J = 24.1 Hz); HR-MS (ESI): calculated for C14H5Cl2F4N3O [M + H]+: 377.98186, found: 377.98135.
2-(3-Chloro-5-(trifluoromethyl)pyridin-2-yl)-5-(4-fluorophenyl)-1,3,4-oxadiazole (E22). Yield 52.5%; light red solid; mp 99.1–100.4 °C; 1H NMR (500 MHz, chloroform-D) δ 8.94 (d, J = 1.8 Hz, 1H, pyridine-H), 8.23–8.22 (m, 1H, pyridine-H), 8.21–8.18 (m, 2H, Ph-H), 7.26–7.21 (m, 2H, Ph-H); 19F NMR (471 MHz, chloroform-D) δ −62.43, −105.33. 13C NMR (125 MHz, chloroform-D, ppm) δ 165.33 (d, J = 254.4 Hz), 165.00, 161.22, 144.64 (d, J = 3.4 Hz), 143.96, 136.77 (d, J = 3.4 Hz), 132.20, 129.96 (d, J = 8.9 Hz), 128.80 (q, J = 34.1 Hz), 122.18 (q, J = 273.4 Hz), 119.57, 116.71 (d, J = 22.2 Hz); HR-MS (ESI): calculated for C14H6ClF4N3O [M + H]+: 344.02083, found: 344.02029.
2-(3-Chloro-5-(trifluoromethyl)pyridin-2-yl)-5-(3-(trifluoromethyl)phenyl)-1,3,4-oxadiazole (E23). Yield 45.8%; yellow solid; mp 81.9–83.0 °C; 1H NMR (500 MHz, chloroform-D) δ 8.97 (dd, J = 1.8, 0.7 Hz, 1H, pyridine-H), 8.47 (d, J = 0.6 Hz, 1H, pyridine-H), 8.42 (d, J = 7.8 Hz, 1H, Ph-H), 8.22 (dd, J = 1.2, 0.7 Hz, 1H, Ph-H), 7.86 (dd, J = 7.8, 0.7 Hz, 1H, Ph-H), 7.72 (t, J = 7.8 Hz, 1H, Ph-H); 19F NMR (471 MHz, chloroform-D) δ −62.49, −62.88; 13C NMR (125 MHz, chloroform-D) δ 164.57, 161.55, 144.68 (d, J = 3.6 Hz), 143.80, 136.79 (d, J = 3.4 Hz), 132.40, 132.04 (d, J = 33.2 Hz), 130.66, 130.01, 129.02 (d, J = 3.4 Hz), 129.00 (q, J = 33.9 Hz), 123.50 (q, J = 272.7 Hz), 124.39 (d, J = 3.7 Hz), 124.16, 122.15 (q, J = 273.5 Hz). HR-MS (ESI): calculated for C15H6ClF6N3O [M + H]+: 394.01764, found: 394.01685.
2-(3-Chloro-5-(trifluoromethyl)pyridin-2-yl)-5-(3-iodophenyl)-1,3,4-oxadiazole (E24). Yield 45.0%; light yellow solid; mp 121.4–123.6 °C; 1H NMR (500 MHz, chloroform-D) δ 8.96 (dd, J = 1.7, 0.6 Hz, 1H, pyridine-H), 8.53 (t, J = 1.6 Hz, 1H, pyridine-H), 8.21–8.16 (m, 2H, Ph-H), 7.92 (ddd, J = 7.9, 1.7, 1.1 Hz, 1H, Ph-H), 7.29 (t, J = 7.9 Hz, 1H, Ph-H); 19F NMR (471 MHz, chloroform-D) δ −62.43; 13C NMR (125 MHz, chloroform-D) δ 164.32, 161.43, 144.68 (d, J = 3.6 Hz), 143.86, 141.44, 136.81 (d, J = 3.3 Hz), 136.05, 132.33, 130.88, 128.92 (q, J = 34.4 Hz), 126.70, 125.06, 122.17 (d, J = 273.5 Hz), 94.56; HR-MS (ESI): calculated for C14H6ClF3IN3O [M + H]+: 451.92689, found: 451.92661.
2-(3-Chloro-5-(trifluoromethyl)pyridin-2-yl)-5-(2-iodophenyl)-1,3,4-oxadiazole (E25). Yield 66.0%; yellow solid; mp 54.4–55.4 °C; 1H NMR (500 MHz, chloroform-D) δ 8.95 (dd, J = 1.7, 0.7 Hz, 1H, pyridine-H), 8.21–8.18 (m, 1H, pyridine-H), 8.08 (dd, J = 8.0, 1.1 Hz, 1H, Ph-H), 7.94 (dd, J = 7.8, 1.7 Hz, 1H, Ph-H), 7.52 (td, J = 7.6, 1.2 Hz, 1H, Ph-H), 7.26–7.23 (m, 1H, Ph-H); 19F NMR (471 MHz, chloroform-D) δ −62.43; 13C NMR (125 MHz, chloroform-D) δ 165.43, 161.61, 144.74, 144.71, 143.98, 141.59, 136.79, 130.81, 132.36, 131.87, 129.04, 128.92 (q, J = 34.4 Hz), 128.44, 122.17 (q, J = 273.6 Hz). 94.60; HR-MS (ESI): calculated for C14H6ClF3IN3O [M + H]+: 451.92689, found: 451.92636.
3-(5-(3-Chloro-5-(trifluoromethyl)pyridin-2-yl)-1,3,4-oxadiazol-2-yl)-N,N-dimethylaniline (E26). Yield 29.4%; yellow solid; mp 76.5–78.6 °C; 1H NMR (500 MHz, chloroform-D) δ 8.95 (dd, J = 1.8, 0.7 Hz, 1H, pyridine-H), 8.18 (dd, J = 1.3, 0.6 Hz, 1H, pyridine-H), 7.54–7.47 (m, 2H, Ph-H), 7.40–7.35 (m, 1H, Ph-H), 6.94–6.89 (m, 1H, Ph-H), 3.04 (s, 6H, –CH3); 19F NMR (471 MHz, chloroform-D) δ −62.43; 13C NMR (125 MHz, chloroform-D) δ 166.62, 161.05, 150.81, 144.61 (d, J = 3.5 Hz), 144.31, 136.64 (d, J = 3.2 Hz), 132.09, 129.92, 128.65 (q, J = 33.9 Hz), 123.80, 122.25 (d, J = 273.5 Hz), 116.28, 115.40, 110.62, 40.53; HR-MS (ESI): calculated for C16H12ClF3N4O [M + H]+: 369.07246, found: 369.07220.
2-(3-Chloro-5-(trifluoromethyl)pyridin-2-yl)-5-(4-fluorobenzyl)-1,3,4-oxadiazole (E27). Yield 53.2%; yellow solid; mp 90.1–91.4 °C; 1H NMR (500 MHz, chloroform-D) δ 8.89 (s, 1H, pyridine-H), 8.15 (s, 1H, pyridine-H), 7.35 (dd, J = 8.1, 5.4 Hz, 2H, Ph-H), 7.04 (t, J = 8.4 Hz, 2H, Ph-H), 4.33 (s, 2H, –CH2–); 13C NMR (125 MHz, chloroform-D) δ 166.59 (d, J = 1.2 Hz), 161.79, 162.41 (d, J = 246.6 Hz), 144.57 (q, J = 3.8 Hz), 136.75 (q, J = 3.8 Hz), 132.23, 130.71, 130.64, 129.03 (d, J = 3.3 Hz), 128.86 (d, J = 34.3 Hz), 122.13 (d, J = 273.5 Hz), 116.04 (d, J = 21.7 Hz), 31.15. HR-MS (ESI): calculated for C15H8ClF4N3O [M + H]+: 358.03648, found: 358.03482.
2-(3-Chloro-5-(trifluoromethyl)pyridin-2-yl)-5-(2,4-dichlorophenyl)-1,3,4-oxadiazole (E28). Yield 50.3%; gray solid; mp 80.0–83.8 °C; 1H NMR (500 MHz, chloroform-D) δ 8.96 (d, J = 0.9 Hz, 1H, pyridine-H), 8.20 (d, J = 1.1 Hz, 1H, pyridine-H), 8.09 (d, J = 8.5 Hz, 1H, Ph-H), 7.62 (d, J = 2.0 Hz, 1H, Ph-H), 7.44 (dd, J = 8.5, 2.0 Hz, 1H, Ph-H); 19F NMR (471 MHz, chloroform-D) δ −62.46; 13C NMR (125 MHz, chloroform-D) δ 163.52, 161.66, 144.78, 143.86, 138.94, 136.78, 134.49, 132.41, 132.36, 131.46, 129.02 (q, J = 34.0 Hz), 127.87, 122.16 (q, J = 273.5 Hz), 121.15; HR-MS (ESI): calculated for C14H5Cl3F3N3O [M + H]+: 393.95231, found: 393.95184.
Insecticidal activity
The insecticidal activity was tested at 25 ± 1 °C according to statistical requirements. Mortalities were calculated and based on a percentage scale using Abbott's formula.41
Insecticidal activity against oriental armyworm. The insecticidal activity against oriental armyworm was investigated using reported procedure.38 The corn leaves were put on the moistened filter paper in a Petri dishes (diameter 9 cm) and sprayed with the solution containing the tested compounds. After drying, 10 fourth instar oriental armyworm larvae were then transferred to the dishes. Mortalities were calculated after treatment for 4 days. Commercial avermectin was also tested under the same conditions. Three replicates and at least five concentrations were performed for each experiment for LC50.
Insecticidal activity against P. xylostella. The insecticidal activities against P. xylostella were evaluated using previously procedure.39 Fresh cabbage discs were dipped into the prepared solutions and placed in a Petri dish (diameter 9 cm) lined with moistened filter paper. Fifteen larvae of second instar P. xylostella were carefully transferred to the Petri dish and cultivated for 72 h. Commercial chlorpyrifos was tested under the same conditions as positive controls, four replicates were performed for each experiment at different concentration.
Insecticidal activity against Nilaparvata lugens and Aphis craccivora. Insecticidal activities against N. lugens and A. craccivora were tested according to our using the Potter spray method.41 Forty third-instar larvae of N. lugens (or A. craccivora) were treated at corresponding concentration at a pressure of 4.35 mg cm−2 under a Potter spray tower. Mortalities were then counted after rearing for 72–96 h. The treatments of imidacloprid and water were conducted as controls. Four replicates were measured for each treatment.
3D-QSAR study
3D-QSAR study was performed according to our provirus work42 using Sybyl 2.0X software (Tripos Inc., St Louis, MO). All the structures were drawn using the SKETCH option and minimized using the Gasteiger–Hückel charge, tripos force field and Powell conjugate gradient algorithm with a convergence criterion of 0.05 kcal mol−1. Then lowest energy conformation was determined using protocol of genetic algorithm (GA) conformational searches using the default setting in Sybyl. The structures were then aligned on a common substructure with compound E20 as the template molecule (Fig. S1 in ESI†). Compounds E7, E8, E9, E16, E22 and E26 were randomly chosen as a test set. The results of alignment are shown in Fig. S1, B (ESI†). Then, CoMFA was performed using the partial least-squares (PLS) protocols to yield all the corresponding parameter (see Table S1 in ESI†). CoMSIA analysis could be done in the similar PLS protocol as CoMFA.
Conflicts of interest
There are no conflicts to declare.
Acknowledgements
This work was supported by the National Natural Science Foundation of China (No. 21762012, 21562012), the Special Foundation of S&T for Outstanding Young Talents in Guizhou (No. 2015-15#) and the Innovative Foundation for Graduated Student of Guizhou University (No. 2017058).
Notes and references
- E. C. Oerke, J. Agric. Sci., 2005, 144, 31–43 CrossRef
. - H. X. Bian, H. F. Ma, X. X. Zheng, M. H. Peng, Y. P. Li, J. F. Su, H. Wang, Q. Li, R. X. Xia, Y. Q. Liu and X. F. Jiang, Sci. Rep., 2017, 7, 2324 CrossRef PubMed
. - Z. Y. Li, X. Feng, S. S. Liu, M. S. You and M. J. Furlong, Annu. Rev. Entomol., 2016, 61, 277–296 CrossRef CAS PubMed
. - W. J. Kang, H. N. Koo, D. H. Jeong, H. K. Kim, J. Kim and G. H. Kim, Entomol. Res., 2017, 47, 394–403 CrossRef CAS
. - Y. Zuo, H. Wang, Y. Xu, J. Huang, S. Wu, Y. Wu and Y. Yang, Insect Biochem. Mol. Biol., 2017, 89, 79–85 CrossRef CAS PubMed
. - Y. Zhang, B. Yang, J. Li, M. Liu and Z. Liu, Insect Mol. Biol., 2017, 26, 453–460 CrossRef CAS PubMed
. - S. Zhang, X. Zhang, J. Shen, D. Li, H. Wan, H. You and J. Li, Pestic. Biochem. Physiol., 2017, 140, 85–89 CrossRef CAS PubMed
. - P. A. Umina, A. Lord, S. Micic and O. Edwards, Pest Manage. Sci., 2017, 73, 1719–1724 CrossRef CAS PubMed
. - H. X. Deng, Zhejiang Chem. Ind., 2011, 42, 1–3 CAS
. - P. J. M. Jung, A. Edmunds, A. Jeanguenat and M. Muehlebach, Chem. Abstr., 2016, 164, 507520 Search PubMed
. - P. J. M. Jung, A. Edmunds, M. Muehlebach and R. G. Hall, Chem. Abstr., 2017, 167, 44589 Search PubMed
. - I. Yonemura, A. Suwa and S. Matsuo, Chem. Abstr., 2016, 164, 394066 Search PubMed
. - A. Edmunds, M. Muehlebach, P. J. M. Jung and A. Jeanguenat, Chem. Abstr., 2016, 165, 212702 Search PubMed
. - I. Yonemura, A. Suwa, S. Matsuo and T. Aoki, Chem. Abstr., 2016, 165, 247300 Search PubMed
. - H. Hiroki, Y. Kobayashi, J. Suzuki, S. Onoue, K. Iwasaki, A. Ootaka, H. Doi, E. Matsuo, M. Onoue and A. Okimoto, Chem. Abstr., 2017, 167, 68507 Search PubMed
. - J. M. Babcock, C. B. Gerwick, J. X. Huang, M. R. Loso, G. Nakamura, S. P. Nolting, R. B. Rogers, T. C. Sparks, J. Thomas, G. B. Watson and Y. Zhu, Pest Manage. Sci., 2011, 67, 328–334 CrossRef CAS PubMed
. - M. Bachwani, V. Sharma and R. Kumar, Int. Res. J. Pharm., 2011, 2, 84–89 CAS
. - Y. Zhou, B. Wang, F. Di, L. Xiong, N. Yang, Y. Li, Y. Li and Z. Li, Bioorg. Med. Chem. Lett., 2014, 24, 2295–2299 CrossRef CAS PubMed
. - T. P. Mohan, B. Vishalakshi, K. S. Bhat, K. S. Rao and G. N. Kendappa, Indian J. Chem., Sect. B: Org. Chem. Incl. Med. Chem., 2004, 43, 1798–1801 Search PubMed
. - Q. Liu, K. Chen, Q. Wang, J. Ni, Y. Li, H. Zhu and Y. Ding, RSC Adv., 2014, 4, 55445–55451 RSC
. - D. He and H. Lu, Chem. Abstr., 2011, 160, 117899 Search PubMed
. - D. He and X. Li, Chem. Abstr., 2011, 155, 352554 Search PubMed
. - Y. Guo, L. Qu, X. Wang, M. Huang, L. Jia and Y. Zhang, RSC Adv., 2016, 6, 93505–93510 RSC
. - H. Dai, Y. Shi, H. He, Y. Li, Z. Jin, Y. Xiao, Y. Yuan and Y. Fang, Chem. Abstr., 2016, 165, 223979 Search PubMed
. - W. Xu, J. He, M. He, F. Han, X. Chen, Z. Pan, J. Wang and M. Tong, Molecules, 2011, 16, 9129–9141 CrossRef CAS PubMed
. - S. Shen, X. Sun, Y. Liu, B. Chen, R. Jin and H. Ma, J. Heterocycl. Chem., 2015, 1296–1301 CrossRef CAS
. - S. Shen, X. Sun, Y. Liu, B. Chen, R. Jin and H. Ma, Chem. J. Chin. Univ., 2014, 35, 1427–1432 CAS
. - A. K. Mittal, D. V. Singh and S. Tripathi, J. Chem., Biol. Phys. Sci., 2012, 2, 699–707 CAS
. - J. Liu, W. Wang and H. He, Chin. J. Org. Chem., 2014, 34, 1447–1451 CrossRef CAS
. - Z. N. Cui, Y. X. Shi, L. Zhang, Y. Ling, B. J. Li, Y. Nishida and X. L. Yang, J. Agric. Food Chem., 2012, 60, 11649–11656 CrossRef CAS PubMed
. - J. Wu, S. Kang, L. Luo, Q. Shi, J. Ma, J. Yin, B. Song, D. Hu and S. Yang, Chem. Cent. J., 2013, 7, 64 CrossRef PubMed
. - Q. Mo, W. Duan, X. Li, D. Huang and Z. Luo, Chin. J. Org. Chem., 2011, 31, 1114–1121 CAS
. - M. Kalhor and A. Dadras, J. Heterocycl. Chem., 2013, 50, 220–224 CrossRef CAS
. - W. G. Duan, X. R. Li, Q. J. Mo, J. X. Huang, B. Cen, X. T. Xu and F. H. Lei, Holzforschung, 2011, 65, 191–197 CrossRef CAS
. - Y. Guo, L. Qu, X. Wang and M. Huang, Chem. Abstr., 2016, 166, 283974 Search PubMed
. - Y. Y. Wang, X. M. Lu, J. Shi, J. H. Xu, F. H. Wang, X. Yang, G. Yu, Z. Q. Liu, C. H. Li, A. L. Dai, Y. H. Zhao and J. Wu, Monatsh. Chem., 2018 DOI:10.1007/s00706-017-2060-3
. - P. Li, L. Shi, X. Yang, L. Yang, X. W. Chen, F. Wu, Q. C. Shi, W. M. Xu, M. He, D. Y. Hu and B. A. Song, Bioorg. Med. Chem. Lett., 2014, 24, 1677–1680 CrossRef CAS PubMed
. - Q. Q. Zhao, J. Shang, Y. X. Liu, K. Wang, F. C. Bi, R. Huang and Q. M. Wang, J. Agric. Food Chem., 2007, 55, 559614–559619 Search PubMed
. - J. H. Xing, W. G. Cai, S. R Wang, J. Chen, S. T. Zhang, R. X. Fu and Q. M. Fu, Plant Prot. Sci., 2007, 33, 116–118 Search PubMed
. - C. Potter, Ann. Appl. Biol., 1952, 39, 1–28 CrossRef
. - W. S. Abbott, J. Am. Mosq. Control Assoc., 1987, 3, 302–303 CAS
. - J. Wu, B. A. Song, D. Y. Hu, M. Yue and S. Yang, Pest Manage. Sci., 2012, 55, 801–810 CrossRef PubMed
.
Footnotes |
† Electronic supplementary information (ESI) available: The copies of 1H NMR, 19F NMR 13C NMR and HR-MS spectrograms for all the synthesized compounds can be found in the ESI. See DOI: 10.1039/c8ra00161h |
‡ Co-first author for the manuscript. |
|
This journal is © The Royal Society of Chemistry 2018 |
Click here to see how this site uses Cookies. View our privacy policy here.