DOI:
10.1039/D0SC04596A
(Edge Article)
Chem. Sci., 2020,
11, 11763-11776
Rhenium versus cadmium: an alternative structure for a thermally stable cadmium carbonyl compound†
Received
21st August 2020
, Accepted 7th September 2020
First published on 9th October 2020
Abstract
An alternative description is provided for the previously reported novel tetranuclear cadmium carbonyl compound, [Cd(CO)3(C6H3Cl)]4. Specifically, consideration of single crystal X-ray diffraction data indicates that the compound is better formulated as the rhenium compound, [Re(CO)3(C4N2H3S)]4. Furthermore, density functional theory calculations predict that, if it were to exist, [Cd(CO)3(C6H3Cl)]4 would have a very different structure to that reported. While it is well known that X-ray diffraction may not reliably distinguish between atoms of similar atomic number (e.g. N/C and Cl/S), it is not generally recognized that two atoms with very different atomic numbers could be misassigned. The misidentification of two elements as diverse as Re and Cd (ΔZ = 27) is unexpected and serves as an important caveat for structure determinations.
Introduction
The early discoveries that carbon monoxide can coordinate to a metal center paved the way for many important industrial applications, as exemplified by the Monsanto acetic acid process and olefin hydroformylation.1 This area of chemistry, however, has been dominated by transition metals, a fact that may be attributed to the stabilization of the M–CO interaction by π-backbonding due to the availability of occupied metal d orbitals.2 As such, main group metals that are devoid of occupied valence d orbitals do not typically form stable metal carbonyl compounds.3 For this reason, the report of a structurally characterized thermally stable cadmium carbonyl compound is of particular significance.4 Therefore, we have investigated this issue and provide herein an alternative explanation for the proposed structure.
Results and discussion
We are currently interested in the use of main group metal compounds for catalytic conversions of organic carbonyl compounds. For example, we have employed zinc compounds as catalysts for the reduction of CO2 to the formic acid and formaldehyde oxidation levels, and also the reduction of aldehydes and ketones.5 In addition to CO2 chemistry, zinc compounds also catalyze reactions involving CO, as illustrated by the fact that zinc oxide (and also Cu/ZnO) is a catalyst for the synthesis of methanol from CO and H2.6 As such, the interaction between CO and ZnO has been investigated by a variety of techniques, which include IR spectroscopy,7,8 solid state 13C NMR spectroscopy,9,10 ultraviolet photoelectron spectroscopy,11 and scanning tunneling microscopy.12 Zinc carbonyl compounds have also been generated under matrix isolation conditions13–15 and in the gas phase,16 but there are no reports of such compounds that have been structurally characterized by X-ray diffraction. Cadmium carbonyl compounds have received even less attention than their zinc counterparts, and were first observed by IR spectroscopic studies in an argon matrix.17,18 In view of the transient nature of these Group 12 metal carbonyl compounds, the report of the synthesis of a thermally stable cadmium carbonyl compound, [Cd(CO)3(C6H3Cl)]4,4 which has been highlighted in a review,19 would be considered to be an important advance, especially given the novel synthetic approach. Specifically, [Cd(CO)3(C6H3Cl)]4 was reported to be obtained via the reaction of an aqueous methanol solution of Cd(O2CCCl3)2 with o-vanillin (Scheme 1), which was described as a green pathway since the formation of this carbonyl compound did not involve the use of carbon monoxide.
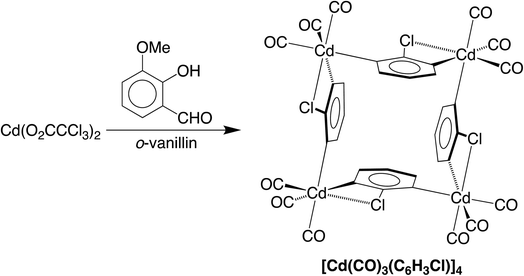 |
| Scheme 1 | |
The formation of [Cd(CO)3(C6H3Cl)]4 from Cd(O2CCCl3)2 and o-vanillin is an unusual transformation and was considered to involve two separate sequences (Scheme 2).4 First, the carbonyl ligands were proposed to originate from decomposition of the trichloroacetate anion to give chloroform, which converted in sunlight to CO via phosgene. In turn, the chloroaryl ligands were proposed to derive by a sequence that involves: (i) initial oxidation of o-vanillin to o-vanillic acid, (ii) reaction of the o-vanillic acid with the aforementioned phosgene and CO to afford 2-chlorobenzene-1,3-dicarboxylic acid upon hydrolysis, and (iii) decarboxylation of the 2-chlorobenzene-1,3-dicarboxylic acid to generate the (C6H3Cl)2− dianion which, in the presence of Cd2+ and CO, forms [Cd(CO)3(C6H3Cl)]4.
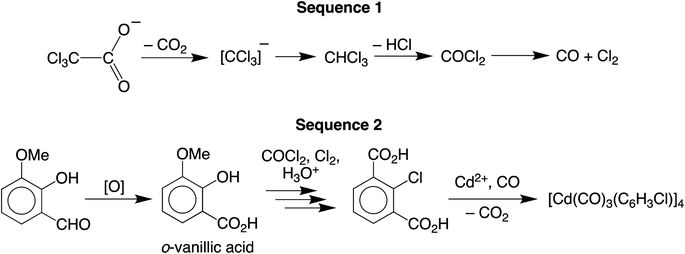 |
| Scheme 2 | |
The carbonyl compound [Cd(CO)3(C6H3Cl)]4 attracted our attention because, if it were to be extended to zinc, the system could provide novel chemistry relevant to catalysis involving CO. However, prior to embarking on such a study, we considered it pertinent to evaluate the nature of [Cd(CO)3(C6H3Cl)]4 in more detail. Since the structural characterization of [Cd(CO)3(C6H3Cl)]4 relied on single crystal X-ray diffraction, which is a technique that may be subject to misinterpretation,20,21 we retrieved the data for [Cd(CO)3(C6H3Cl)]4 that were deposited in the Cambridge Structural Database (CSD)22 for further investigation.
Atom displacement parameters are an important aspect of assessing the correctness of atom assignments, and so we evaluated the parameters both visually via an atom displacement plot (Fig. 1) and quantitatively in terms of their absolute and relative values (Table 1). Examination of the atom displacement plot (Fig. 1) indicates that the displacement parameters for the cadmium atoms are exceptionally small by comparison to the other atoms; alternatively, the displacement parameters for the outer atoms are much larger than those for cadmium. For example, the average Ueq value for the cadmium atoms is 0.020 Å2, while those for the carbonyl carbon, carbonyl oxygen and ring carbon atoms are 0.098 Å2, 0.122 Å2 and 0.074 Å2, respectively. If normalized to a value of 1.00 Å2 for cadmium, these correspond to values of 4.96 Å2, 6.19 Å2 and 3.73 Å2, respectively. Although it is not unusual for the central atom in a molecule to have smaller displacement parameters than the outer atoms, the magnitude of the difference is not usually this large.
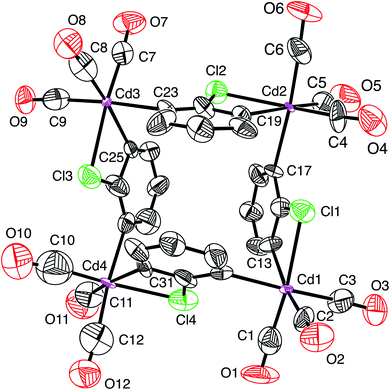 |
| Fig. 1 Atom displacement plot for [Cd(CO)3(C6H3Cl)]4 with data taken from CCDC #185365 (40% displacement parameters). | |
Table 1 Average values for equivalent isotropic displacement parameters (Å2) for some metal carbonyl compoundsa
|
U
eq(M) |
U
eq(C) |
U
eq(O) |
U
eq(M)rel |
U
eq(C)rel |
U
eq(O)rel |
Ref. |
U
eq(X)rel = Ueq(X)/Ueq(M)
|
Cr(CO)6 |
0.009 |
0.014 |
0.020 |
1.00 |
1.45 |
2.20 |
23
|
Mo(CO)6 |
0.018 |
0.025 |
0.041 |
1.00 |
1.41 |
2.28 |
24
|
W(CO)6 |
0.029 |
0.041 |
0.067 |
1.00 |
1.42 |
2.34 |
25
|
Fe(CO)5 |
0.014 |
0.022 |
0.035 |
1.00 |
1.53 |
2.46 |
26
|
Ni(CO)4 |
0.016 |
0.023 |
0.035 |
1.00 |
1.41 |
2.16 |
26
|
[Cd(CO)3(C6H3Cl)]4 |
0.020 |
0.098 |
0.122 |
1.00 |
4.96 |
6.19 |
4
|
As an illustration, the displacement parameters for some binary carbonyl compounds are summarized in Table 1.23–26 Thus, relative to a value of 1.00 Å2 for Ueq of the metal atom, the carbonyl carbon atoms of the binary carbonyl compounds range from 1.41 Å2 to 1.53 Å2, while the values for the oxygen atoms range from 2.16 Å2 to 2.46 Å2. The very large relative values of 4.96 Å2 and 6.19 Å2 for the carbon and oxygen atoms of the carbonyl ligands of [Cd(CO)3(C6H3Cl)]4, therefore, clearly indicate that the relative Ueq values for the cadmium atoms of [Cd(CO)3(C6H3Cl)]4 are anomalously small; it must be emphasized, however, that it is the relative value of Ueq for Cd that is anomalous, because the absolute value is not exceptionally unusual. For example, the value of Ueq for Cd (0.020 Å2) is within the range (0.009 Å2 to 0.029 Å2) reported for the metals in the binary carbonyl compounds (Table 1).
The unusually small displacement parameters for the cadmium atoms relative to the other atoms in [Cd(CO)3(C6H3Cl)]4 strongly indicate that the cadmium atoms have been misidentified and should be reassigned to a heavier atom.27 Support for the proposal that the metal atoms are not cadmium is provided by the fact that the density functional theory (DFT) geometry optimized structure of [Cd(CO)3(C6H3Cl)]4 (Fig. 2), using the coordinates of the experimental structure, failed to converge to a similar structure to that reported (Fig. 1).4
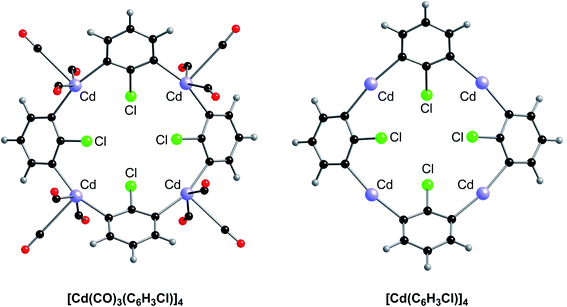 |
| Fig. 2 DFT geometry optimized structure of [Cd(CO)3(C6H3Cl)]4 (left) and [Cd(C6H3Cl)]4 (right). | |
Key differences between the geometry optimized structure (Fig. 2, left) and that derived by X-ray diffraction (Fig. 1) are summarized in Table 2. Specifically: (i) the CAr–Cd–CAr bond angles in the experimentally reported structure of [Cd(CO)3(C6H3Cl)]4 are very bent (84.4°), whereas the geometry optimized values are almost linear (162.2°); (ii) the Cd–Cl distances in the experimentally reported structure of [Cd(CO)3(C6H3Cl)]4 are asymmetric (2.53 Å and 3.68 Å), with there being a much closer interaction between each chlorine and one of the cadmium atoms, whereas each chlorine in the geometry optimized structure is located symmetrically between two Cd centers, with distances (3.19 Å and 3.21 Å) that are beyond normal bonding interactions; (iii) the three carbonyl ligands possess a fac disposition in the experimental structure (CCO–Cd–CCO bond angles in the range 86.4°–88.9°) but a mer disposition in the geometry optimized structure (CCO–Cd–CCO bond angles of 76.0°, 76.1° and 151.5°); (iv) the Cd–C–O bond angles in the experimentally determined structure are close to linear (174.6°) but become much more bent in the geometry optimized structure (163.4°); and (v) finally, and most significantly, the average Cd–CO distance increases considerably from 1.86 Å in the experimental structure to 2.90 Å in the geometry optimized structure. The latter two observations indicate that Cd–CO interactions in [Cd(CO)3(C6H3Cl)]4 are not significant. Therefore, we also geometry optimized the counterpart with no carbonyl ligands, and, importantly, the structure of [Cd(C6H3Cl)]4 is very similar to the same moiety in [Cd(CO)3(C6H3Cl)]4 (Fig. 2 and Table 2).
Table 2 Comparison of average metrical data for each Cd center of the experimentally reported structure of [Cd(CO)3(C6H3Cl)]4 and the DFT geometry optimized structures of [Cd(CO)3(C6H3Cl)]4 and [Cd(C6H3Cl)]4
|
[Cd(CO)3(C6H3Cl)]4 |
[Cd(CO)3(C6H3Cl)]4 |
[Cd(C6H3Cl)]4 |
Experimentala |
DFT |
DFT |
Data taken from ref. 4.
|
d(Cd–CO)/Å |
1.855 |
2.901 |
— |
d(Cd–CAr)/Å |
2.194 |
2.217 |
2.200 |
d(Cd–Clshort)/Å |
2.526 |
3.187 |
3.113 |
d(Cd–Cllong)/Å |
3.680 |
3.211 |
3.121 |
CAr–Cd–CAr/° |
84.44 |
162.15 |
169.98 |
CCO–Cd–CCO/° |
86.35 |
76.00 |
— |
CCO–Cd–CCO/° |
88.17 |
76.06 |
— |
CCO–Cd–CCO/° |
88.90 |
151.54 |
— |
Cd–C–O/° |
174.64 |
163.42 |
— |
While both (i) the atom displacement parameters and (ii) the deviation of the geometry optimized structure from the experimentally determined structure clearly indicate that the identity of the metal atom needs to be reassigned, consideration should also be given to the possibility that the identities of other atoms may also need to be reevaluated, especially since it is well known20,21 that atoms that have similar atomic numbers (e.g. B/C,28,29 B/N,30 C/N,31 C/O,32 N/O,28,33,34 O/F,35,36 Si/Cl36 and Cl/S37,38) are often difficult to differentiate by X-ray diffraction.
In this regard, analysis of compounds listed in the CSD indicates that, other than [Cd(CO)3(C6H3Cl)]4, there are no structurally characterized compounds that feature a bridging C6H3Cl ligand. Moreover, there are only two examples of compounds that have a related C6H4Cl ligand coordinated to a single metal center, namely a zirconium compound39 and an iridium compound.40–42 Although the paucity of compounds with a κ2-chloroaryl ligand is not a reason to exclude them from consideration, it does suggest that other possibilities should be considered. Since there are occurrences of Cl/S misidentification in the literature,37,38 the possibility that the substituent on the aromatic ring is sulfur merits serious consideration, as does the possibility that the other atoms coordinated to cadmium are not carbon, but nitrogen. Indeed, analysis of the CSD indicates that six-membered heterocyclic nitrogen ligands with sulfur coordinated to a metal are common. Specifically, there are 255 structurally characterized examples of compounds with a κ2-pyridine thiolate ligand,43 and 134 examples of compounds with κ2-pyridimine thiolate ligands.44,45
Assuming that the compound proposed to be “[Cd(CO)3(C6H3Cl)]4” is a carbonyl compound, chemical bonding considerations, as discussed earlier, dictate that the cadmium should be replaced with a transition metal. In this regard, while cadmium is located closer to the second than the third transition metal series, the fact that the displacement parameter is anomalously small relative to the other atoms requires that the new metal be selected from the third transition series. The most likely candidates are W, Re and Os, which are well known to form six-coordinate tricarbonyl compounds; of these, rhenium derivatives are the most common.46 Recognizing that transition metal carbonyl compounds typically adopt 18-electron configurations, it is evident that, according to the covalent bond classification,47 the purported “(C6H3Cl)” moiety must be reassigned to an L3 donor for W, an L2X donor for Re, and an LX2 donor for Os. Focusing on the above heterocyclic nitrogen ligands derived from 2-mercaptopyridine and 2-mercaptopyrimidine, the possible bonding situations are illustrated in Fig. 3.
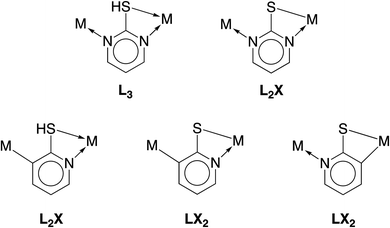 |
| Fig. 3 Covalent bond classification of potential bridging ligands derived from 2-mercaptopyrimidine (top) and 2-mercaptopyridine (bottom). | |
Of these possibilities, the L2X coordination mode afforded by the pyrimidine thiolate ligand (Fig. 3) is appealing because it is evident that potential compounds can be straightforwardly obtained from commercially available 2-mercaptopyrimidine.44,48 Indeed, pyrimidine-2-thiolate compounds are well known and the rhenium compound [Re(CO)3(C4N2H3S)]4 (Fig. 4) has been reported.44a The critical issue, however, is whether the X-ray diffraction data for [Re(CO)3(C4N2H3S)]4 could reproduce the structure reported for [Cd(CO)3(C6H3Cl)]4, not only in terms of metrical details and atom displacement parameters, but also in terms of providing reasonable refinement parameters such that the results could be considered publishable. In view of the fact that cadmium (Z = 48) and rhenium (Z = 75) differ by the substantial value of 27 in terms of their atomic numbers, such that they have very different X-ray scattering powers, it is not at all obvious that it is possible to misassign these elements and yet still achieve a publishable structure. To provide an answer to this issue, it is necessary to refine a dataset for [Re(CO)3(C4N2H3S)]4 as the cadmium compound, [Cd(CO)3(C6H3Cl)]4. While the positional parameters of the former are available,44a the required structure factors have not been reported,49 and so we collected X-ray diffraction data on [Re(CO)3(C4N2H3S)]4.
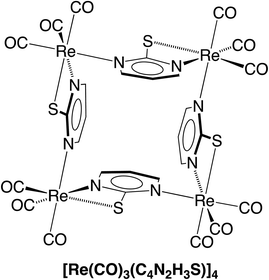 |
| Fig. 4 A tetranuclear rhenium tricarbonyl pyrimidine-2-thiolate compound. | |
As is evident from Fig. 5, the molecular structure of [Re(CO)3(C4N2H3S)]4 shows a striking resemblance to that for [Cd(CO)3(C6H3Cl)]4 (Fig. 1).4 The crystallographic data for [Re(CO)3(C4N2H3S)]4 were, therefore, subsequently refined as [Cd(CO)3(C6H3Cl)]4 (Fig. 6), which hereafter will be referred to as “[Cd(CO)3(C6H3Cl)]4-Re” to indicate that the cadmium structure is based on the rhenium data set. Significantly, there is excellent agreement between the respective bond lengths of [Cd(CO)3(C6H3Cl)]4-Re and the published structure of [Cd(CO)3(C6H3Cl)]4, as illustrated in Fig. 7, with the average deviation in all bond lengths being only 0.039 Å. In addition to similar bond lengths, the relative atom displacement parameters of the two structures are also comparable (Table 3). The body of evidence, therefore, indicates that the reported structure of the novel cadmium carbonyl compound, [Cd(CO)3(C6H3Cl)]4, is actually that of the rhenium compound, [Re(CO)3(C4N2H3S)]4.
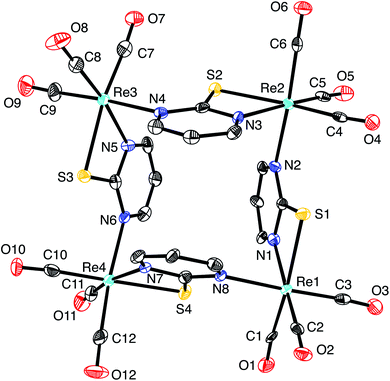 |
| Fig. 5 Atom displacement plot for [Re(CO)3(C4N2H3S)]4 (40% displacement parameters). | |
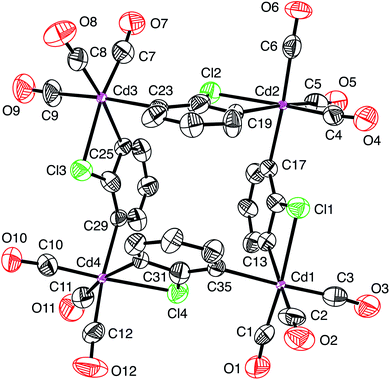 |
| Fig. 6 Atom displacement plot for [Cd(CO)3(C6H3Cl)]4-Re using experimental data for [Re(CO)3(C4N2H3S)]4 (40% displacement parameters). | |
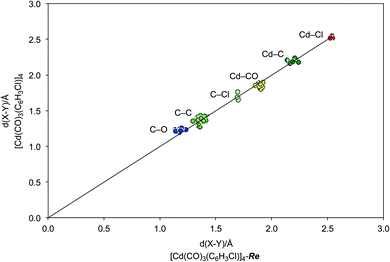 |
| Fig. 7 Comparison of the bond lengths of [Re(CO)3(C4N2H3S)]4, refined as [Cd(CO)3(C6H3Cl)]4-Re, with those of the published data for [Cd(CO)3(C6H3Cl)]4,4 demonstrating an excellent correspondence. | |
Table 3 Relative average Ueq valuesa for [Re(CO)3(C4N2H3S)]4, refined as [Cd(CO)3(C6H3Cl)]4-Re, together with those of the published data for [Cd(CO)3(C6H3Cl)]4
|
[Cd(CO)3(C6H3Cl)]4-Re |
[Cd(CO)3(C6H3Cl)]4b |
U
eq(X)rel = Ueq(X)/Ueq(Cd).
Data taken from ref. 4.
|
U
eq(Cd)rel |
1 |
1 |
U
eq(CCO)rel |
4.80 |
4.96 |
U
eq(O)rel |
6.43 |
6.19 |
U
eq(Cl)rel |
4.47 |
3.87 |
U
eq(CCdC)rel |
2.89 |
2.29 |
U
eq(Cring)rel |
4.25 |
3.73 |
The fact that the X-ray diffraction data for a rhenium compound can be interpreted as a cadmium compound is most consequential because this would not at all be expected due to the significantly different scattering powers of these elements. As such, it merits further discussion, and one factor that needs to be considered is the R value,50 since this is typically used as a criterion to evaluate the reliability of a structure determination. In this regard, while the R value for the cadmium refinement (6.78%) is, as would be expected, higher than that for the rhenium refinement (3.43%), it is certainly acceptable for publication since structures with much higher R values appear commonly in the chemistry literature. For example, of the structurally characterized cadmium compounds listed in the CSD, 20.4% have R values greater than 6.00%.51 In fact, the CSD contains structures of cadmium compounds with R values as high as 30.1%.52 Thus, the R value for [Cd(CO)3(C6H3Cl)]4-Re cannot be used in the present case as a definitive gauge of an incorrect structure.
Although the R value for [Cd(CO)3(C6H3Cl)]4-Re does not necessarily indicate an incorrect structure, the small value of Ueq for cadmium relative to the other atoms does indicate that there is a problem. To illustrate in more detail the impact of the incorrect atom assignment on the derived displacement parameters, the Ueq data for [Re(CO)3(C4N2H3S)]4 and its refinement as the cadmium complex, [Cd(CO)3(C6H3Cl)]4-Re, are compared in Table 4 and Fig. 8. These data indicate that not only is Ueq for the cadmium smaller than the value for rhenium in the correct refinement, but the values for the other atoms are all significantly larger, as indicated by the fact that they lie above the correlation line with a slope of unity (Fig. 8). More specifically, whereas Ueq(Cd)/Ueq(Re) is 0.71, the average ratios for the other groups of atoms are in the range 2.10–2.75 (Table 4).
Table 4 Average Ueq values for [Re(CO)3(C4N2H3S)]4 and the structure refined as [Cd(CO)3(C6H3Cl)]4-Re
X |
[Re(CO)3(C4N2H3S)]4 |
[Cd(CO)3(C6H3Cl)]4-Re |
U
eq(X)rela |
U
eq(X)rel = Ueq(X)[Cd(CO)3(C6H3Cl)]4-Re]/Ueq(X)[Re(CO)3(C4N2H3S)]4].
|
M |
0.021 |
0.015 |
0.71 |
S/Cl |
0.024 |
0.066 |
2.75 |
CCO |
0.029 |
0.070 |
2.41 |
O |
0.044 |
0.094 |
2.14 |
N/C |
0.020 |
0.042 |
2.10 |
Cring |
0.023 |
0.062 |
2.70 |
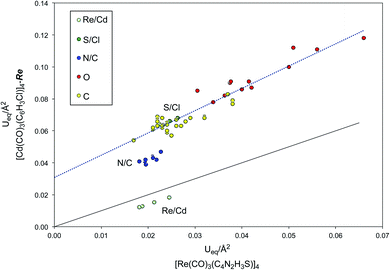 |
| Fig. 8 Comparison of Ueq values for [Re(CO)3(C4N2H3S)]4 and the structure refined as the cadmium compound, [Cd(CO)3(C6H3Cl)]4-Re. The solid line has a slope of unity and depicts the boundary that indicates whether the Ueq values for the incorrectly refined structure are larger or smaller than the correct structure. The dashed line is drawn through all atoms that have the same assignments in both structures. Note that the misidentified Re/Cd and N/C pairs are in distinct locations, in contrast to the S/Cl pairs which cannot be distinguished on this basis. | |
Examination of the trendline through the atoms that have the same assignments in both refinements (i.e. the carbonyl groups and ring carbon atoms) illustrates that there is a distinct shift of the nitrogen atoms that are incorrectly refined as carbon atoms (Fig. 8). This anomaly is of note because even close visual inspection of the atom displacement plots (Fig. 6) does not signal an obvious error in the assignment of the N/C pairs of atoms.
In contrast to the shift observed for the N/C pairs of atoms (Fig. 8), the S/Cl pairs are not discernably displaced from the trendline because the proportional difference in atomic numbers53 between S and Cl (6.25%) is less than that between N and C (16.67%). Therefore, it is not surprising that visual inspection of the atom displacement plots (Fig. 6) likewise provides no clear indication that the chlorine atoms are misassigned. In many cases, incorrect atom assignments are indicated by the observation of unusual “cigar” or “disk” shaped ellipsoids or by refinements that result in atoms becoming “non-positive definite”.21a–d However, in the present case, the incorrect atom assignments result in none of the atoms either becoming “non-positive definite” or exhibiting particularly unusual shapes. Instead, the assignment of rhenium as cadmium causes the Ueq values for all other atoms to increase in an approximately uniform manner, such that if one were to focus on the nonmetal atoms, the structure could appear to be normal. Indeed, the enlarged atom displacement parameters could also have been interpreted in terms of thermal motion.
In addition to the discrepancy in the magnitudes of the atom displacement parameters, another indication that there is a problem with atom assignments may be obtained by evaluating the results of a Hirshfeld “rigid-bond” analysis.54–56 Specifically, since vibrations involving bond stretching are of higher energy than those involved in other vibrational modes, the components of the displacement parameters of two bonded atoms should be of similar magnitude along the direction of the bond. Thus, for two atoms (A and B), where zA2 and zB2 are the mean-square amplitudes along the bond, the difference ΔAB = zA2 − zB2 is expected to be approximately zero.54,55 Indeed, the C–C, C–N and C–O bonds in organic compounds are typically characterized by values of ΔAB less than 0.001 Å2.54,55 However, for metal compounds, in which there is a large difference in mass between the metal and the coordinating atom, values of ΔAB of approximately 0.003 Å2 (i.e. √ΔAB ≈ 0.05 Å) are typical (with the lighter atom having the larger value of z2),57 although much larger values of ΔAB have been reported for metal carbonyl compounds, e.g. 0.0148 Å2 (i.e. √ΔAB ≈ 0.12 Å) for [(PPh3)-RuCo(CO)6(μ-PPh2)].58 In this regard, application of the Hirshfeld analysis to [Cd(CO)3(C6H3Cl)]4-Re indicates that the √ΔAB values for the Cd–X bonds of [Cd(CO)3(C6H3Cl)]4-Re are particularly large and range from 0.14 Å to 0.26 Å, with an average value of 0.21 Å.59,60 As such, it is clear that this test provides further evidence that the cadmium center of [Cd(CO)3(C6H3Cl)]4-Re is misassigned.
Since the displacement parameters do indeed provide evidence for the atom misassignment, some consideration needs to be given as to why it went unnoticed. With respect to this issue, it appears that the structural determination of coordination compounds often seems to focus on the atoms associated with the ligands, rather than the central metal, a bias that is presumably a consequence of the belief that the metal is not usually in doubt. However, there are certainly rational means by which a compound analyzed may not contain the presumed metal, which include (i) accidentally selecting an incorrect container of a reagent for the synthesis of the compound, (ii) unnoticed metal contamination resulting from an earlier step in the reaction sequence, and (iii) accidentally selecting the incorrect vial of crystals while preparing the sample for data collection.61 If possibilities such as these are not considered, it is understandable how, without careful scrutiny, the true identity of the central atom may go unrecognized. The present example serves as a reminder that exceptional care is often required to verify the identity of a compound, which is especially necessary when the proposed structure is novel;62,63 furthermore, in addition to spectroscopic and analytical data, a computational analysis of the proposed structure could also be used to provide useful corroborating data.
Single crystal X-ray diffraction plays a critical role in the chemical sciences,64,65 such that it is important to appreciate how misinterpretations may occur. The present example is of particular note because the ability to refine successfully a compound that contains rhenium as one that contains cadmium, two metals that differ substantially in their atomic numbers (ΔZ = 27), is unexpected. Other reports of misidentification in ordered structures typically involve atoms that possess similar atomic numbers,66–69 and so the example described here indicates that the problem may be more widespread than realized; it also emphasizes the importance of paying close attention to the displacement parameters of all atoms when evaluating a structure refinement model.
Summary
In conclusion, the structure reported for the cadmium carbonyl compound [Cd(CO)3(C6H3Cl)]4, with bridging chloroaryl ligands, is actually that of the rhenium compound [Re(CO)3(C4N2H3S)]4, with bridging pyrimidine-2-thiolate ligands. As such, three pairs of atoms, namely Re/Cd, S/Cl and N/C, have been misidentified. Of these, the misidentification involving Re and Cd is most consequential because of the significant disparity in their X-ray scattering powers due to their large difference in atomic numbers (ΔZ = 27). Thus, while it is well known that X-ray diffraction may not reliably distinguish between atoms with similar atomic number (e.g. C and N), the ability of pairs of atoms with very different atomic numbers to be misidentified is not well appreciated. The ability to refine [Re(CO)3(C4N2H3S)]4 as [Cd(CO)3(C6H3Cl)]4, with an R value that is of publication quality, is remarkable and serves as a caveat, especially when reporting the structures of novel compounds.
Experimental section
X-ray structure determination
Crystals of previously reported [Re(CO)3(C4N2H3S)]444a suitable for X-ray diffraction were obtained from CH2Cl2.70 X-ray diffraction data were collected on a Bruker Apex II diffractometer, and the structure was solved by using direct methods and standard difference map techniques, and was refined by full-matrix least-squares procedures on F2 with SHELXTL (version 2014/7).71 The asymmetric unit contains two molecules of CH2Cl2, one of which is disordered over two positions and was modeled by using SADI and EADP restraints. The structure was also refined as [Cd(CO)3(C6H3Cl)]4 to illustrate the impact of incorrect atom assignments on the atom displacement parameters and refinement parameters. The Hirshfeld test was performed with PLATON.56a,b,72 Crystallographic data have been deposited with the Cambridge Crystallographic Data Centre (CCDC 2024180-2024181).
Computational details
Calculations were carried out using DFT as implemented in the Jaguar 8.9 (release 15) suite of ab initio quantum chemistry programs.73 Geometry optimizations were performed with the B3LYP density functional using the LACVP** basis sets and Cartesian coordinates are provided in the ESI.†
Conflicts of interest
There are no conflicts to declare.
Acknowledgements
This research was supported by the U. S. Department of Energy, Office of Science, Office of Basic Energy Sciences, Catalysis Science Program, under Award DE-SC0019204.
References
- J.-B. Peng, H.-Q. Geng and X.-F. Wu, The chemistry of CO: Carbonylation, Chem, 2019, 5, 526–552 CAS.
-
(a)
R. H. Crabtree, Organometallic Chemistry of the Transition Metals, Blackwell Science Publ, Oxford, 6th edn, 2014 CrossRef;
(b)
G. L. Miessler, P. J. Fischer and D. A. Tarr, Inorganic Chemistry, Pearson, Boston, 5th edn, 2014 Search PubMed;
(c)
J. F. Hartwig, Organotransition Metal Chemistry: From Bonding to Catalysis, University Science Books, Sausalito, 2009 Search PubMed.
- A. J. Lupinetti, V. Jonas, W. Thiel, S. H. Strauss and G. Frenking, Trends in molecular geometries and bond strengths of the homoleptic d10 metal carbonyl cations [M(CO)n]x+ (Mx+ = Cu+, Ag+, Au+, Zn2+, Cd2+, Hg2+; n=1-6): A theoretical study, Chem.–Eur. J., 1999, 5, 2573–2583 CrossRef CAS.
- S. K. Dey, S. Shit, S. P. Dey, S. Mitra and K. M. A. Malik, First report on thermally stable cadmium carbonyl complex containing an interesting chloroaryl bridge: Isolation and characterization, Chem. Lett., 2011, 40, 810–812 CrossRef CAS.
-
(a) W. Sattler and G. Parkin, Zinc catalysts for on-demand hydrogen generation and carbon dioxide functionalization, J. Am. Chem. Soc., 2012, 134, 17462–17465 CrossRef CAS;
(b) M. Rauch and G. Parkin, Zinc and magnesium catalysts for the hydrosilylation of carbon dioxide, J. Am. Chem. Soc., 2017, 139, 18162–18165 CrossRef CAS;
(c) W. Sattler, D. G. Shlian and D. Sambade, Synthesis and sructural characterization of bis(2-pyridylthio)(p-tolylthio)methyl zinc complexes and the catalytic hydrosilylation of CO2, Polyhedron, 2020, 187, 114542 CrossRef CAS;
(d) W. Sattler, S. Ruccolo, M. R. Chaijan, T. N. Allah and G. Parkin, Hydrosilylation of aldehydes and ketones catalyzed by a terminal zinc hydride complex, [κ3-Tptm]ZnH, Organometallics, 2015, 34, 4717–4731 CrossRef CAS.
-
(a) J. Frenzel and D. Marx, Methanol synthesis on ZnO(000
). IV. Reaction mechanisms and electronic structure, J. Chem. Phys., 2014, 141, 124710 CrossRef;
(b) J. Kiss, J. Frenzel, B. Meyer and D. Marx, Methanol synthesis on ZnO(000
). II. Structure, energetics, and vibrational signature of reaction intermediates, J. Chem. Phys., 2013, 139, 044705 CrossRef;
(c) J. P. Hindermann, H. Idriss and A. Kiennemann, Adsorbed species on ZnO in CO-H2 and CO2-H2 reactions, Mater. Chem. Phys., 1988, 18, 513–532 CrossRef CAS;
(d) M. Bowker, H. Houghton and K. C. Waugh, Mechanism and kinetics of methanol synthesis on zinc-oxide, J. Chem. Soc., Faraday Trans. 1, 1981, 77, 3023–3036 RSC;
(e) M. Kurtz, J. Strunk, O. Hinrichsen, M. Muhler, K. Fink, B. Meyer and C. Woll, Active sites on oxide surfaces: ZnO-catalyzed synthesis of methanol from CO and H2, Angew. Chem., Int. Ed., 2005, 44, 2790–2794 CrossRef CAS;
(f) S. A. French, A. A. Sokol, S. T. Bromley, C. R. A. Catlow and P. Sherwood, Identification and characterization of active sites and their catalytic processes—the Cu/ZnO methanol catalyst, Top. Catal., 2003, 24, 161–172 CrossRef CAS.
-
(a) J. C. Lavalley, J. Saussey and T. Raïs, Infrared study of the interaction between CO and H2 on ZnO: Mechanism and sites of formation of formyl species, J. Mol. Catal., 1982, 17, 289–298 CrossRef CAS;
(b) G. Ghiotti, F. Boccuzzi and R. Scala, Infrared study of ZnO surface properties: CO Adsorption and CO/D2 interaction at 77K, J. Catal., 1985, 92, 79–97 CrossRef CAS;
(c) D. A. Seanor and C. H. Amberg, Infrared-band intensities of adsorbed carbon monoxide, J. Chem. Phys., 1965, 42, 2967–2970 CrossRef CAS;
(d) M. Buchholz, X. Yu, C. Yang, S. Heibler, A. Nefedov, Y. Wang and C. Wöll, IR-spectroscopy of CO adsorption on mixed-terminated ZnO surfaces, Surf. Sci., 2016, 652, 247–252 CrossRef CAS.
- For IR spectroscopic studies concerned with the coordination of CO to zinc containing zeolites, see: C. L. Angell and P. C. Schaffer, Infrared spectroscopic investigations of zeolites and adsorbed molecules. II. Adsorbed carbon monoxide, J. Phys. Chem., 1966, 70, 1413–1417 CrossRef CAS.
- T. R. Krawietz, D. H. Barich, L. W. Beck, T. Howard, T. Xu and J. F. Haw, NMR observation of a zinc carbonyl in zeolite ZnY, J. Am. Chem. Soc., 1995, 117, 10407–10408 CrossRef CAS.
- For solid state 13C NMR spectroscopic studies concerned with the coordination of CO to zinc containing zeolites, see ref. 9 and G. Qi, Q. Wang, Y. Chu, J. Xu, A. Zheng, J. Su, J. Chen, C. Wang, W. Wang, P. Gao and F. Deng, Room temperature stable zinc carbonyl complex formed in zeolite ZSM-5 and its hydrogenation reactivity: a solid-state NMR study, Chem. Commun., 2015, 51, 9177–9180 RSC.
- R. R. Gay, M. H. Nodine, V. E. Henrich, H. J. Zeiger and E. I. Solomon, Photoelectron study of the interaction of CO with ZnO, J. Am. Chem. Soc., 1980, 102, 6752–6761 CrossRef CAS.
- H. Shi, H. Yuan, S. Ruan, W. Wang, Z. Li and X. Shao, Adsorption and diffusion of CO on clean and CO2-precovered ZnO(10
0), J. Phys. Chem. C, 2018, 122, 8919–8924 CrossRef CAS.
- D. A. Van Leirsburg and C. W. DeKock, Interaction of matrix-isolated NiF2 and NiCl2 with CO, N2, NO, and O2 and of CaF2, CrF2, MnF2, CuF2, and ZnF2 with CO in argon matrices, J. Phys. Chem., 1974, 78, 134–142 CrossRef CAS.
-
(a) L. Jiang and Q. Xu, Experimental and theoretical evidence for the formation of zinc tricarbonyl in solid argon, J. Am. Chem. Soc., 2005, 127, 8906–8907 CrossRef CAS;
(b) L. Jiang, Y. Teng and Q. Xu, Reactions of laser-ablated zinc and cadmium atoms with CO: Infrared spectra of the Zn(CO)x (x = 1-3), CdCO−, and Cd(CO)2 molecules in solid neon, J. Phys. Chem. A, 2006, 110, 7092–7096 CrossRef CAS.
- The exact nature of one of these compounds, however, has been questioned. See: L. Jin, L. Fu and Y. Ding, Zinc(0) chemistry: does the missing 18-electron zinc tricarbonyl really exist?, Phys. Chem. Chem. Phys., 2010, 12, 10956–10962 RSC.
- H. Qu, F. Kong, G. Wang and M. Zhou, Infrared photodissociation spectroscopy of heterodinuclear iron-zinc and cobalt-zinc carbonyl cation complexes, J. Phys. Chem. A, 2017, 121, 1627–1632 CrossRef CAS.
- L. Jiang and Q. Xu, Infrared spectroscopic and density functional theory studies on the reactions of cadmium atoms with carbon monoxide in solid argon, J. Phys. Chem. A, 2005, 109, 9001–9005 CrossRef CAS.
- Mercury carbonyl compounds were also first observed in an argon matrix,a but have also been structurally characterized by X-ray diffractionb:
(a) D. Tevault, D. P. Strommen and K. Nakamoto, Reactions of mercury halides with CO, NO, N2, and unsaturated hydrocarbons in argon matrices, J. Am. Chem. Soc., 1977, 99, 2997–3003 CrossRef CAS;
(b) M. Bodenbinder, G. BalzerJollenbeck, H. Willner, R. J. Batchelor, F. W. B. Einstein, C. Wang and F. Aubke, Syntheses and vibrational and 13C MAS-NMR spectra of bis(carbonyl)mercury(II) undecafluorodiantimonate(V) ([Hg(CO)2][Sb2F11]2) and of bis(carbonyl) dimercury(I) undecafluorodiantimonate ([Hg2(CO)2][Sb2F11]2) and the molecular structure of [Hg(CO)2][Sb2F11]2, Inorg. Chem., 1996, 35, 82–92 CrossRef CAS.
-
S. B. J. Dane, T. C. King and D. S. Wright, Group 2 (Be-Ba) and Group 12 (Zn-Hg), in Organometallic Chemistry, ed. I. J. S. Fairlamb and J. M. Lynam, Royal Soc Chemistry, Cambridge, 2014, vol. 39, pp. 194–209 Search PubMed.
-
(a) G. Parkin, Bond-stretch isomerism in transition-metal complexes: A reevaluation of crystallographic data, Chem. Rev., 1993, 93, 887–911 CrossRef CAS;
(b) G. Parkin, Do bond-stretch isomers really exist?, Acc. Chem. Res., 1992, 25, 455–460 CrossRef CAS.
-
(a) P. G. Jones, Crystal-structure determination: A critical view, Chem. Soc. Rev., 1984, 13, 157–172 RSC;
(b) R. L. Harlow, Troublesome crystal structures. Prevention, detection, and resolution, J. Res. Natl. Inst. Stand. Technol., 1996, 101, 327–339 CrossRef CAS;
(c) A. L. Thompson, Chemical crystallography: When are ‘bad data’ ‘good data’?, Crystallogr. Rev., 2019, 25, 3–53 CrossRef;
(d) C. H. Schwalbe, Should we remediate small molecule structures? If so, who should do it?, Crystallogr. Rev., 2018, 24, 217–235 CrossRef CAS;
(e) P. E. Fanwick, Small molecule crystallography past, present, and future: a career long review of chemical crystallography, Crystallogr. Rev., 2016, 22, 250–279 CrossRef;
(f) J. van de Streek and M. A. Neumann, Validation of experimental molecular crystal structures with dispersion-corrected density functional theory calculations, Acta Crystallogr., Sect. B: Struct. Sci., 2010, 66, 544–558 CrossRef CAS;
(g) P. Müller, Practical suggestions for better crystal structures, Crystallogr. Rev., 2009, 15, 57–83 CrossRef;
(h) W. Clegg, Current developments in small-molecule X-ray crystallography, Comm. Inorg. Chem., 2005, 26, 165–182 CrossRef CAS;
(i) M. G. Campbell, T. M. Powers and S. L. Zheng, Teaching with the case study method to promote active learning in a small molecule crystallography course for chemistry students, J. Chem. Educ., 2016, 93, 270–274 CrossRef CAS;
(j) A. L. Spek, What makes a crystal structure report valid?, Inorg. Chim. Acta, 2018, 470, 232–237 CrossRef CAS.
- C. R. Groom, I. J. Bruno, M. P. Lightfoot and S. C. Ward, The Cambridge Structural Database (CSD version 5.39), Acta Crystallogr., Sect. B: Struct. Sci., Cryst. Eng. Mater., 2016, 72, 171–179 CrossRef CAS.
- V. L. Deringer, A. Wang, J. George, R. Dronskowski and U. Englert, Anisotropic thermal motion in transition-metal carbonyls from experiments and ab initio theory, Dalton Trans., 2016, 45, 13680–13685 RSC.
-
F. Olbrich, CSD Communication, 2015, p. 1049704 Search PubMed.
- F. Grevels, J. Jacke, W. E. Klotzbücher, F. Mark, V. Skibbe, K. Schaffner, K. Angermund, C. Krüger, C. W. Lehmann and S. Özkar, Sequential photosubstitution of carbon monoxide by (E)-cyclooctene in hexacarbonyltungsten: Structural aspects, multistep photokinetics, and quantum yield, Organometallics, 1999, 18, 3278–3293 CrossRef CAS.
- L. J. Farrugia and C. Evans, Experimental X-ray charge density studies on the binary carbonyls Cr(CO)6, Fe(CO)5, and Ni(CO)4, J. Phys. Chem. A, 2005, 109, 8834–8848 CrossRef CAS.
- The alternative possibility, in which all atoms other than cadmium be reassigned to lighter atoms, makes little chemical sense and so was not considered further.
-
(a) R.-Q. Fang, Z.-P. Xiao, P. Cao, D.-H. Shi and H.-L. Zhu, A novel heterocyclic compound: catena-poly[[[diaquasodium(I)]-di-μ-aqua] hemi(1,5-dihydroxy-4,8,9-trioxa-2,6-diazabicyclo[3.3.1]nona-2,6-diene-3,7-diolate)]. Retraction, Acta Crystallogr., Sect. C: Cryst. Struct. Commun., 2008, 64, e11 CrossRef CAS;
(b) R.-Q. Fang, Z.-P. Xiao, P. Cao, D.-H. Shi and H.-L. Zhu, A novel heterocyclic compound: catena-poly[[[diaquasodium(I)]-di-μ-aqua] hemi(1,5-dihydroxy-4,8,9-trioxa-2,6-diazabicyclo[3.3.1]nona-2,6-diene-3,7-diolate)], Acta Crystallogr., Sect. C: Cryst. Struct. Commun., 2007, 63, m192–m194 CrossRef.
-
(a) H. C. Johnson, A. P. M. Robertson, A. B. Chaplin, L. J. Sewell, A. L. Thompson, M. F. Haddow, I. Manners and A. S. Weller, Catching the first oligomerization event in the catalytic formation of polyaminoboranes: H3B·NMeHBH2·NMeH2 bound to iridium, J. Am. Chem. Soc., 2012, 134, 3932 CrossRef CAS;
(b) H. C. Johnson, A. P. M. Robertson, A. B. Chaplin, L. J. Sewell, A. L. Thompson, M. F. Haddow, I. Manners and A. S. Weller, Catching the first oligomerization event in the catalytic formation of polyaminoboranes: H3B·NMeHBH2·NMeH2 bound to iridium, J. Am. Chem. Soc., 2011, 133, 11076–11079 CrossRef CAS.
-
(a) W. T. Klooster, T. F. Koetzle, P. E. M. Siegbahn, T. B. Richardson and R. H. Crabtree, Study of the N-H⋯H-B dihydrogen bond including the crystal structure of BH3NH3 by neutron diffraction, J. Am. Chem. Soc., 1999, 121, 6337–6343 CrossRef CAS;
(b) M. Bühl, T. Steinke, P. v. R. Schleyer and R. Boese, Solvation effects on geometry and chemical shifts. An ab initio/IGLOI reconciliation of apparent experimental inconsistencies on H3B·NH3, Angew. Chem., Int. Ed. Engl., 1991, 30, 1160–1161 CrossRef;
(c)
R. Boese, N. Niederprüm and D. Bläser, The boron-nitrogen bond. Structural Investigations, in Molecules in Natural Science and Medicine, ed. Z. B. Maksic and M. Eckert-Masic, E. Horwood, Chichester, England, 1992 Search PubMed.
-
(a) Sadiq-ur-Rehman, S. Sherzaman, S. Ali, S. Shahzadi and M. Helliwell, Poly[μ2-chlorido-nonamethyl-μ3-nitrato-tritin(IV)]. Corrigendum, Acta Crystallogr., Sect. E: Struct. Rep. Online, 2008, 64, e26 CrossRef CAS;
(b) Sadiq-ur-Rehman, S. Sherzaman, S. Ali, S. Shahzadi and M. Helliwell, Poly[μ2-chlorido-nonamethyl-μ3-nitrato-tritin(IV)], Acta Crystallogr., Sect. E: Struct. Rep. Online, 2007, 63, m2329 CrossRef CAS.
-
(a) J. Hurmalainen, M. A. Land, K. N. Robertson, C. J. Roberts, I. S. Morgan, H. M. Tuononen and J. A. C. Clyburne, Comment on ‘Crystallographic snapshot of an arrested intermediate in the biomimetic activation of CO2’, Angew. Chem., Int. Ed., 2015, 54, 7484–7487 CrossRef CAS;
(b) S. L. Ackermann, D. J. Wolstenholme, C. Frazee, G. Deslongchamps, S. H. M. Riley, A. Decken and G. S. McGrady, Crystallographic snapshot of an arrested intermediate in the biomimetic activation of CO2, Angew. Chem., Int. Ed., 2015, 54, 164–168 CrossRef CAS.
-
(a) C. P. Casey, G. A. Bikzhanova, J. E. Backvall, L. Johansson, J. Park and Y. H. Kim, Unexpected formation of the isopropylamine complex [2,3,4,5-Ph4(η4-C4CO)] (CO)2Ru(H2NCHMe2) in the attempted synthesis of an isopropyl alcohol complex, Organometallics, 2002, 21, 1955–1959 CrossRef CAS;
(b) H. M. Jung, S. T. Shin, Y. H. Kim, M. J. Kim and J. Park, X-ray structure and reactivity of (η4-tetraphenylcyclopentadienone)(CO)2Ru(HOCHMe2): Unexpected stability of the neutral 2-propanol-ruthenium(0) complex with respect to β-hydride elimination, Organometallics, 2001, 20, 3370–3372 CrossRef CAS.
-
(a) H. Phull, D. Alberti, I. Korobkov, S. Gambarotta and P. H. M. Budzelaar, Fixation of CO2 by magnesium cations: A reinterpretation, Angew. Chem., Int. Ed., 2006, 45, 5331–5334 CrossRef CAS;
(b) C.-C. Chang, M.-C. Liao, T.-H. Chang, S.-M. Peng and G.-H. Lee, Aluminum-magnesium complexes with linearly bridging carbon dioxide, Angew. Chem., Int. Ed., 2005, 44, 7418–7420 CrossRef CAS.
-
(a) A. D. Ostrowski, R. O. Absalonson, M. A. De Leo, G. Wu, J. G. Pavlovich, J. Adamson, B. Azhar, A. V. Iretskii, I. L. Megson and P. C. Ford, Photochemistry of trans-Cr(cyclam)(ONO)2+, a nitric oxide precursor (vol 50, pg 4453, 2011), Inorg. Chem., 2011, 50, 5848 CrossRef CAS;
(b) A. D. Ostrowski, R. O. Absalonson, M. A. De Leo, G. Wu, J. G. Pavlovich, J. Adamson, B. Azhar, A. V. Iretskii, I. L. Megson and P. C. Ford, Photochemistry of trans-Cr(cyclam)(ONO)2+, a nitric oxide precursor, Inorg. Chem., 2011, 50, 4453–4462 CrossRef CAS.
- H. G. von Schnering and D. Vu, Are the previously described [ClF6][CuF4] and [Cu(OH2)4][SiF6] identical?, Angew. Chem., Int. Ed. Engl., 1983, 22, 408 CrossRef.
-
(a) B. M. Segal, H.
R. Hoveyda and R. H. Holm, Terminal ligand assignments based on trends in metal-ligand bond lengths of cubane-type [Fe4S4]2+,+ clusters, Inorg. Chem., 1998, 37, 3440–3443 CrossRef CAS;
(b) H. R. Hoveyda and R. H. Holm, Characterization of the self-condensation equilibrium of [Fe4S4(SH)4]2-: Spectroscopic identification of a unique sulfide-bridged acyclic tricubane cluster, Inorg. Chem., 1997, 36, 4571–4578 CrossRef CAS.
- E. Babaian-Kibala, F. A. Cotton and P. A. Kibala, A new bis(μ-sulfido)ditantalum(IV) edge-sharing bioctahedral molecule and a reassessment of some earlier ‘bis(μ-chloro)’ molecules, Inorg. Chem., 1990, 29, 4002–4005 CrossRef CAS.
- F. Wu, A. K. Dash and R. F. Jordan, Structures and reactivity of Zr(IV) chlorobenzene complexes, J. Am. Chem. Soc., 2004, 126, 15360–15361 CrossRef CAS.
- E. Ben-Ari, M. Gandelman, H. Rozenberg, L. J. W. Shimon and D. Milstein, Selective ortho C-H activation of haloarenes by an Ir(I) system, J. Am. Chem. Soc., 2003, 125, 4714–4715 CrossRef CAS.
- Furthermore, there are only eight examples of compounds with polychlorinated aryl ligands that exhibit such interactions. See:
(a) M. P. García, M. V. Jiménez, F. J. Lahoz, J. A. López and L. A. Oro, Synthesis of the homoleptic rhodium (III) complex [Rh(C6Cl5)3]. Molecular structures of [Rh(C6Cl5)3] and [Rh(C6Cl4-C6Cl4)(C6Cl5)(SC4H8)2], J. Chem. Soc., Dalton Trans., 1998, 4211–4214 RSC;
(b) P. J. Alonso, J. Forniés, M. A. García-Monforte, A. Martín, B. Menjón and C. Rillo, Synthesis and characterization of new paramagnetic tetraaryl derivatives of chromium and molybdenum, J. Organomet. Chem., 2007, 692, 3236–3247 CrossRef CAS;
(c) M. P. García, M. V. Jiménez, A. Cuestra, C. Siurana, L. A. Oro, F. J. Lahoz, J. A. López, M. P. Catalán, A. Tiripicchio and M. Lanfranchi, Synthesis and reactivity of mononuclear (pentachlorophenyl)rhodium(II) complexes. Structural relevance of rhodium- o-chlorine secondary bonding, Organometallics, 1997, 16, 1026–1036 CrossRef;
(d) M. P. García, A. P. Martínez, M. V. Jiménez, C. Siurana, L. A. Oro, F. J. Lahoz and A. Tiripicchio, Reactivity of rhodium(III) complexes containing chelating pentachlorophenyl ligands. Molecular structures of fac-[Rh(C6Cl5)3(py)] and mer-[Rh(C6Cl5)3(tBuNC)3], Inorg. Chim. Acta, 2000, 308, 51–58 CrossRef;
(e) J. Fornies, B. Menjon, R. M. Sanz-Carrillo, M. Tomas, N. G. Connelly, J. G. Crossley and A. G. Orpen, Synthesis and structural characterization of the first isolated homoleptic organoplatinum(IV) compound: [Pt(C6Cl5)4], J. Am. Chem. Soc., 1995, 117, 4295–4304 CrossRef CAS.
- It is also pertinent to note that the Ir–Cl bond distance (2.816 Å), is distinctly longer than the Cd–Cl bond lengths reported for [Cd(CO)3(C6H3Cl)]4 (2.515 Å to 2.547 Å),a despite the fact that the covalent radius of cadmium (1.44 Å) is actually larger than that of iridium (1.41 Å),b which further questions the existence of a Cd–Cl bond in the compound.
(a)
ref. 4
;
(b) B. Cordero, V. Gómez, A. E. Platero-Prats, M. Revés, J. Echeverría, E. Cremades, F. Barragán and S. Alvarez, Covalent radii revisited, Dalton Trans., 2008, 2832–2838 RSC.
- See, for example:
(a) A. Kreider-Mueller, P. J. Quinlivan, J. S. Owen and G. Parkin, Tris(2-mercaptoimidazolyl)hydroborato cadmium thiolate complexes, [TmBut]CdSAr: Thiolate exchange at cadmium in a sulfur-rich coordination environment, Inorg. Chem., 2017, 56, 4643–4653 CrossRef;
(b) M. Schlaf, A. J. Lough and R. H. Morris, [Os(η2-H2)(CO)(pyS)(PPh3)2]BF4 – A stable but highly acidic dihydrogen complex, Organometallics, 1993, 12, 3808–3809 CrossRef CAS;
(c) K. Sukcharoenphon, D. Moran, P. v. R. Schleyer, J. E. McDonough, K. A. Abboud and C. D. Hoff, Increased Reactivity of the ˙Cr(CO)3(C5Me5) radical with thiones versus thiols: A theoretical and experimental investigation, Inorg. Chem., 2003, 42, 8494–8503 CrossRef CAS;
(d) J. G. Reynolds, S. C. Sendlinger, A. M. Murray, J. C. Huffman and G. Christou, Synthesis and characterization of vanadium(II,III,IV) complexes of pyridine-2-thiolate, Inorg. Chem., 1995, 34, 5745–5752 CrossRef CAS;
(e) S. Demir, T. J. Mueller, J. W. Ziller and W. J. Evans, σ Bond metathesis reactivity of allyl scandium metallocenes with diphenyldichalcogenides, PhEEPh (E = S, Se, Te), Organometallics, 2011, 30, 3083–3089 CrossRef CAS;
(f) E. C. Constable, C. A. Palmer and D. A. Tocher, The synthesis, crystal and molecular structure of mer-tris(2-pyridinethiolato)cobalt(III), Inorg. Chim. Acta, 1990, 176, 57–60 CrossRef CAS;
(g) S. Park, A. J. Lough and R. H. Morris, Iridium(III) complex containing a unique bifurcated hydrogen bond interaction involving Ir−H···H(N)···F−B atoms. Crystal and molecular structure of [IrH(η1-SC5H4NH)(η2-SC5H4N)(PPh3)2](BF4)·0.5C6H6, Inorg. Chem., 1996, 35, 3001–3006 CrossRef CAS;
(h) M. Islam, C. A. Johns, S. E. Kabir, K. Kundu, K. M. A. Malik and S. M. B. Ullah, Reactions of Mn2(μ-pyS)2(CO)6 (pySH = pyridine-2-thiol) with triphenylphosphine (PPh3), diphenylphosphine (PHPh2) and bis(diphenylphosphino)methane (dppm): X-ray crystal structure of Mn(pyS)(η1-dppm)2(CO)2, J. Chem. Crystallogr., 1999, 29, 1001–1007 CrossRef CAS.
- See, for example:
(a) S. E. Kabir, J. Alam, S. Ghosh, K. Kundu, G. Hogarth, D. A. Tocher, G. M. G. Hossain and H. W. Roesky, Synthesis, structure and reactivity of tetranuclear square-type complexes of rhenium and manganese bearing pyrimidine-2-thiolate (pymS) ligands: versatile and efficient precursors for mono- and polynuclear compounds containing M(CO)3 (M = Re, Mn) fragments, Dalton Trans., 2009, 4458–4467 RSC;
(b) S. Alvarez, G. Aullon, R. Fandos, J. L. G. Fierro, P. Ocon, A. Otero, S. Rojas and P. Terreros, A pyrimidine thiolate Rh(I) complex: structure, bonding and one-dimensional interactions in solid and in solution, Dalton Trans., 2005, 938–944 RSC;
(c) Z. Han, L. Shen, W. W. Brennessel, P. L. Holland and R. Eisenberg, Nickel pyridinethiolate complexes as catalysts for the light-driven production of hydrogen from aqueous solutions in noble-metal-free systems, J. Am. Chem. Soc., 2013, 135, 14659–14669 CrossRef CAS;
(d) I. A. Latham, G. J. Leigh, C. J. Pickett, G. Huttner, I. Jibrill and J. Zubieta, The anion of pyrimidine-2-thiol as a ligand to molybdenum, tungsten, and iron. Preparation of complexes, their structure and reactivity, J. Chem. Soc., Dalton Trans., 1986, 1181–1187 RSC;
(e) S. G. Rosenfield, H. P. Berends, L. Gelmini, D. W. Stephan and P. K. Mascharak, New octahedral thiolato complexes of divalent nickel: syntheses, structures, and properties of (Et4N)[Ni(SC5H4N)3] and (Ph4P)[Ni(SC4H3N2)3]·CH3CN, Inorg. Chem., 1987, 26, 2792–2797 CrossRef CAS;
(f) C. D. Bush, T. A. Hamor, W. Hussain, C. J. Jones, J. A. McCleverty and A. S. Rothin, Synthesis and molecular structure of tetrabutylammonium tetrachloro(pyrimidine-2-thiolato)technetate(IV), Acta Crystallogr., Sect. C: Cryst. Struct. Commun., 1987, 43, 2088–2091 CrossRef;
(g) F. J. Femia, J. W. Babich and J. Zubieta, Structural systematics of the {ReO}3+ core with ‘3+2’ ligand donor sets, Inorg. Chim. Acta, 2000, 300–302, 462–470 CrossRef CAS;
(h) D. J. Rose, K. P. Maresca, T. Nicholson, A. Davison, A. G. Jones, J. Babich, A. Fischman, W. Graham, J. R. D. DeBord and J. Zubieta, Synthesis and characterization of organohydrazino complexes of technetium, rhenium, and molybdenum with the {M(η1-HxNNR)(η2-HyNNR)} core and their relationship to radiolabeled organohydrazine-derivatized chemotactic peptides with diagnostic applications, Inorg. Chem., 1998, 37, 2701–2716 CrossRef CAS;
(i) D. J. Rose, K. P. Maresca, P. B. Kettler, Y. D. Chang, V. Soghomomian, Q. Chen, M. J. Abrams, S. K. Larsen and J. Zubieta, Synthesis and characterization of rhenium thiolate complexes. Crystal and molecular structures of [NBu4][ReO(H2O)Br4)]·2H2O, [Bu4N][ReOBr4(OPPh3)], [ReO(SC5H4N)3], [ReO(SC4H3N2)3][ReO(OH)(SC5H4N-3,6-(SiMe2But)2)2], [Re(N2COC6H5)(SC5H4N)Cl(PPh3)2], and [Re(PPh3)(SC4H3N2)3], Inorg. Chem., 1996, 35, 3548–3558 CrossRef CAS.
- In contrast to the many examples of compounds with κ2-N,S-ligation of heterocyclic nitrogen compounds, there is only one example of a κ2-C,S-ligation derived from a benzene thiolate ligand. See: R. Beck, M. Frey, S. Camadanli and H. F. Klein, Four- and five-membered cobaltacycles by regioselective cyclometallation of benzyl sulfide derivatives via Co(V) intermediates, Dalton Trans., 2008, 4981–4983 RSC.
- For example, the distribution in the number of metal tricarbonyl compounds for the third transition series metals is: Sc (0), Hf (1), Ta (6), W (2,754), Re (4,339), Os (2,207), Ir (157) and Pt (0). See ref. 22.
-
(a) M. L. H. Green and G. Parkin, Application of the Covalent Bond Classification method for the teaching of inorganic chemistry, J. Chem. Educ., 2014, 91, 807–816 CrossRef CAS;
(b) M. L. H. Green, A new approach to the formal classification of covalent compounds, J. Organomet. Chem., 1995, 500, 127–148 CrossRef CAS;
(c)
G. Parkin, Classification of organotransition metal compounds, Comprehensive Organometallic Chemistry III, ed. R. H. Crabtree and D. M. P. Mingos, Elsevier, Oxford, 2006, vol. 1, ch. 1 Search PubMed.
- In this regard, it is worth noting that there are no structurally characterized examples of the L2X and LX2 motifs derived from 2-mercaptopyridine, or L3 motifs derived from 2-mercaptopyrimidine.
- Likewise, the structure factors for [Cd(CO)3(C6H3Cl)]4 are not published.
-
R refers to the conventional discrepancy index of R1 = [Σ||Fo| – |Fc||]/[Σ|Fo|] for I > 2σ(I).
- These percentages are for compounds for which the R value is listed.
- For recent examples of cadmium complexes with high R values, see:
(a) X. Wang, L. Zhou, Q. Ye, F. Geng, H. Ye, D. Fu and Y. Zhang, A spiro-type ammonium based switchable dielectric material with two sequential reversible phase transitions above room temperature, RSC Adv., 2016, 6, 74117–74123 RSC;
(b) T. Prakasam, R. A. Bilbeisi, M. Lusi, J. Olsen, C. Platas-Iglesias and A. Trabolsi, Post-synthetic modifications of cadmium-based knots and links, Chem. Commun., 2016, 52, 7398–7401 RSC;
(c) W. Liao, Y. Tang, P. Li, Y. You and R. Xiong, Competitive halogen bond in the molecular ferroelectric with large piezoelectric response, J. Am. Chem. Soc., 2018, 140, 3975–3980 CrossRef CAS.
- The proportional difference between atoms A and B is defined as 100(ZA – ZB)/ZB where ZA > ZB.
- F. L. Hirshfeld, Can X-ray data distinguish bonding effects from vibrational smearing?, Acta Crystallogr., Sect. A: Cryst. Phys., Diffr., Theor. Gen. Crystallogr., 1976, 32, 239–244 CrossRef.
-
(a) R. E. Rosenfield, K. N. Trueblood and J. D. Dunitz, Test for rigid-body vibrations, based on a generalization of Hirshfeld rigid-bond postulate, Acta Crystallogr., Sect. A: Cryst. Phys., Diffr., Theor. Gen. Crystallogr., 1978, 34, 828–829 CrossRef;
(b) K. Chandrasekhar and H. B. Bürgi, Dynamic processes in crystals examined through difference vibrational parameters ΔU: The low-spin-high-spin transition in tris(dithiocarbamato)iron(III) complexes, Acta Crystallogr., Sect. B: Struct. Sci., 1984, 40, 387–397 CrossRef.
-
(a) A. L. Spek, checkCIF validation ALERTS: what they mean and how to respond, Acta Crystallogr., Sect. E: Crystallogr. Commun., 2020, 76, 1–11 CrossRef CAS;
(b) A. L. Spek, Structure validation in chemical crystallography, Acta Crystallogr., Sect. D: Biol. Crystallogr., 2009, 65, 148–155 CrossRef CAS;
(c) A. L. Spek, Single-crystal structure validation with the program PLATON, J. Appl. Crystallogr., 2003, 36, 7–13 CrossRef CAS.
-
(a) J. D. Dunitz, E. F. Maverick and K. N. Trueblood, Atomic motions in molecular crystals from diffraction measurements, Angew. Chem., Int. Ed. Engl., 1988, 27, 880–895 CrossRef;
(b) J. D. Dunitz, V. Schomaker and K. N. Trueblood, Intrepretation of atomic displacement parameters from diffraction studies of crystals, J. Phys. Chem., 1988, 92, 856–867 CrossRef CAS.
- D. Braga and T. F. Koetzle, A mean-square displacement amplitude analysis of terminally bound CO groups in transition-metal clusters, Acta Crystallogr., Sect. B: Struct. Sci., 1988, 44, 151–155 CrossRef.
- The other X–Y bonds in the molecule have √ΔAB values that range from 0.03 Å to 0.23 Å, with an average value of 0.12 Å.
- In addition to the absolute magnitude of ΔAB, the ratio of ΔAB to its standard uncertainty (σ) has also been considered as an indicator of incorrect atom assignment (ref. 53) and, in this regard, many Cd–X bonds fail at the 5σ level: Cd1–C1 (5.15), Cd1–C3 (5.23), Cd2–C6 (5.23), Cd3–Cl3 (14.67), Cd4-Cl4 (15.67), Cd2–Cl2 (16.00) and Cd1–Cl1 (16.33).
- In this regard, an author of ref. 4 has published structures of a variety of rhenium compounds. See, for example:
(a) A. J. Amoroso, A. Banu, M. P. Coogan, P. G. Edwards, G. Hossain and K. M. A. Malik, Functionalisation of terpyridine complexes containing the Re(CO)3+ moiety, Dalton Trans., 2010, 39, 6993–7003 RSC;
(b) S. Dinda, D. K. Hazra, S. RoyChowdhury, M. Helliwell, K. M. A. Malik, M. Mukherjee and R. Bhattacharyya, Reductive thiocyanolysis of tetraoxorhenate (VII): Synthesis, crystal structure, catalytic oxidation and kinetic studies of (PPh4)2[Re(NCS)6] and (PPh4)2[ReO(NCS)5], Inorg. Chim. Acta, 2009, 362, 2108–2116 CrossRef CAS;
(c) M. L. Creber, K. G. Orrell, A. G. Osborne, V. Šik, M. B. Hursthouse and K. M. A. Malik, Dynamic NMR investigations of fluxionality of 2-(dimethoxymethyl)pyridine and 2,6-bis(dimethoxymethyl)pyridine in rhenium(I) and platinum(IV) complexes, J. Chem. Soc., Dalton Trans., 2000, 4218–4226 RSC;
(d) R. J. Baker, P. G. Edwards, J. Garcia-Mora, F. Ingold and K. M. A. Malik, Manganese and rhenium triphosphorus macrocycle complexes and reactions with alkenes, J. Chem. Soc., Dalton Trans., 2002, 3985–3992 RSC;
(e) A. Gelling, K. G. Orrell, A. G. Osborne, V. Šik, M. B. Hursthouse, D. E. Hibbs and K. M. A. Malik, Synthesis and dynamic NMR studies of Rhenium(I) tricarbonyl bromide complexes of benzimidazolylpyridine ligands. Crystal structure of [ReBr(CO)3{2,6-bis(1’,5’,6’-trimethylbenzimidazol-2’-yl)pyridine}], Polyhedron, 1998, 17, 2141–2151 CrossRef CAS;
(f) K. A. Azam, M. A. Hossain, M. B. Hursthouse, S. E. Kabir, K. M. A. Malik and H. Vahrenkamp, Photochemical reaction of Re2(CO)10 with tetramethylthiourea; structural characterization of two novel pentarhenium carbonyl complexes containing sulfido and tetramethyldiaminocarbene ligands, [Re5(CO)17(μ-R)(μ4-S)2{C(NMe2)2}2] (R = SH or OH), J. Organomet. Chem., 1998, 555, 285–292 CrossRef CAS;
(g) K. G. Orrell, A. G. Osborne, V. Šik, M. W. da Silva, M. B. Hursthouse, D. E. Hibbs, K. M. A. Malik and N. G. Vassilev, Stereochemically non-rigid transition metal complexes of 2,6-bis[(1-phenylimino)ethyl]pyridine (BIP) Part 2. Dynamic NMR studies of fac-[ReX(CO)3(BIP)] (X = Cl, Br, or I). Crystal structure of fac-[ReBr(CO)3(BIP)], J. Organomet. Chem., 1997, 538, 171–183 CrossRef CAS;
(h) M. T. Ahmet, C. Lu, J. R. Dilworth, J. R. Miller, Y. Zheng, D. E. Hibbs, M. B. Hursthouse and K. M. A. Malik, Synthesis of complexes of ReV, RuII, OsII, RhI, IrI and IrIII. Crystal and molecular structures of [ReO(OH)(SC6H4SiPh3-2)2(PPh3)], [ReH4(SC6H4SiPh3-2)(PPh3)3] and [IrH(SC6H4SiPh3-2)2(PMePh2)3], J. Chem. Soc., Dalton Trans., 1995, 3143–3152 RSC;
(i) M. T. Ahmet, B. Coutinho, J. R. Dilworth, J. R. Miller, S. J. Parrott, Y. Zheng, M. Harman, M. B. Hursthouse and A. Malik, Synthesis and characterization of organoimido- and organoamido-rhenium(V) complexes. Crystal structures of [ReCl2(NC6H4PPh2-2)(HNC6H4PPh2-2)], [Re(8-HNC9H6N)2O(PPh3)][BPh4] and [Re(NPh)(NC5H3S-2-SiMe3-3)2(PPh3)][BPh4], J. Chem. Soc., Dalton Trans., 1995, 3041–3048 RSC.
- As an illustration, the 13C NMR spectroscopic signals of the carbonyl groups of [Cd(CO)3(C6H3Cl)]4 would be expected to exhibit cadmium satellites (111Cd, 12.80%; 113Cd,12.22%) that would provide excellent evidence for a Cd–CO moiety, but none were reported; also, chlorine elemental analysis would have provided convincing evidence for the chloroaryl ligand, but was not listed (ref. 4).
- Co-crystallization of platinum, palladium and gold polyoxometallates with the tungsten derivative has also resulted in the misidentification of compounds. See: K. P. O'Halloran, C. C. Zhao, N. S. Ando, A. J. Schultz, T. F. Koetzle, P. M. B. Piccoli, B. Hedman, K. O. Hodgson, E. Bobyr, M. L. Kirk, S. Knottenbelt, E. C. Depperman, B. Stein, T. M. Anderson, R. Cao, Y. V. Geletii, K. I. Hardcastle, D. G. Musaev, W. A. Neiwert, X. K. Fang, K. Morokuma, S. X. Wu, P. Kogerler and C. L. Hill, Revisiting the polyoxometalate-based late-transition-metal-oxo complexes: The ‘oxo wall’ stands, Inorg. Chem., 2012, 51, 7025–7031 CrossRef.
- S. J. Coles and P. A. Gale, Changing and challenging times for service crystallography, Chem. Sci., 2012, 3, 683–689 RSC.
- M. B. Hursthouse and S. J. Coles, The UK National Crystallography Service; its origins, methods and science, Crystallogr. Rev., 2014, 20, 117–154 CrossRef.
- In addition to the aforementioned instances of misidentification involving nonmetals,28–38 other examples include Cu being incorrectly refined as Co,a-c Br being incorrectly refined as Cu,d,e O being incorrectly refined as S,f,g Br being incorrectly refined as Ag,d,e Zn being incorrectly refined as Mo,h,i Sn being incorrectly refined as Se,j,k,l Ni (or possibly Co or Zn) being incorrectly refined as Pd,m,n and a combination of Ni/Cl/N being incorrectly refined as Ln/Ni/Cl (Ln = Eu, Ce, Gd).o,p
(a) V. D. Vreshch, J. H. Yang, H. T. Zhang, A. S. Filatov and E. V. Dikarev, Monomeric square-planar cobalt(II) acetylacetonate: mystery or mistake?, Inorg. Chem., 2010, 49, 8430–8434 CrossRef CAS;
(b) J. Burgess, J. Fawcett and D. R. Russell, Bis(2,4-pentanedionato)cobalt(II), Acta Crystallogr., Sect. C: Cryst. Struct. Commun., 2011, 67, e13 CrossRef CAS;
(c) F. A. Cotton and R. H. Holm, Magnetic investigations of spin-free cobaltous complexes. III. On the existence of planar complexes, J. Am. Chem. Soc., 1960, 82, 2979–2983 CrossRef CAS;
(d) A. Haaland, K. Rypdal, H. P. Verne, W. Scherer and W. R. Thiel, The crystal-structures of base-free, monomeric arylcopper(I) and arylsilver(I) compounds; 2 cases of mistaken identity?, Angew. Chem., Int. Ed., 1994, 33, 2443–2445 CrossRef;
(e) R. Lingnau and J. Strähle, 2,4,6-Ph3C6H2M (M = Cu, Ag), monomeric CuI-complexes and AgI-complexes with coordination number 1, Angew. Chem., Int. Ed., 1988, 27, 436 CrossRef;
(f) A. Ienco, M. Caporali, F. Zanobini and C. Mealli, Is 2.07 Å a record for the shortest Pt-S distance? Revision of two reported X-ray structures, Inorg. Chem., 2009, 48, 3840–3847 CrossRef CAS;
(g) H. Li, G. B. Carpenter and D. A. Sweigart, Models for homogeneous deep hydrodesulfurization. Intramolecular CO substitution by the sulfur in [(η6-2-methylbenzothiophene)Mn(CO)3]+ and [(η6-dibenzothiophene)Mn(CO)3]+ after regiospecific insertion of platinum into a C-S bond, Organometallics, 2000, 19, 1823–1825 CrossRef CAS;
(h) F. A. Cotton and G. Schmid, Proposed reformulation of recently reported ‘tetrahedral molybdenum(II)’ complexes: Trimethylphosphine complexes of zinc chloride, Polyhedron, 1996, 15, 4053–4059 CrossRef CAS;
(i) K. Fromm, M. Plaikner and E. Hey-Hawkins, Synthesis and molecular structures of the molybdenum(II) complexes MoCl2(PMe3)2 and [Mo(μ-PPh2)(PPh2)(PMe3)]2, Z. Naturforsch., B: J. Chem. Sci., 1995, 50, 894–898 CAS;
(j) E. Rufino-Felipe, E. Osorio, G. Merino and M. A. Munoz-Hernandez, Do planar tetracoordinate tin complexes really exist?, Dalton Trans., 2013, 42, 11180–11185 RSC;
(k) R. Cea-Olivares, J. Novosad, J. D. Woollins, A. M. Z. Slawin, V. Garcia-Montalvo, G. Espinosa-Perez and P. G. Y. Garcia, A true square-planar tin(II) spiro complex: Molecular structure of bis(imidotetraphenyldiselenodiphosphino-Se,Se')tin(II) and its distorted tetragonal-pyramidal isomer, Chem. Commun., 1996, 519–520 RSC;
(l) R. Cea-Olivares, M. Moya-Cabrera, V. Garcia-Montalvo, R. Castro-Blanco, R. A. Toscano and S. Hernandez-Ortega, True square planar [M{N(SePiPr2)2-Se,Se’}2] [M = Sn, Se] complexes. An extraordinary geometrical arrangement for well known centers [Sn(II), Se(II)], Dalton Trans., 2005, 1017–1018 RSC;
(m) W. H. Zhang and T. S. A. Hor, Complexation of 1,1’-bis(diphenylphosphino)ferrocene dioxide (dppfO2) with 3d metals and revisit of its coordination to Pd(II), Dalton Trans., 2011, 40, 10725–10730 RSC;
(n) J. S. L. Yeo, J. J. Vittal and T. S. A. Hor, PdCl2(dppfO2-O,O’): a simple palladium(II) complex with a rare tetrahedral structure, Chem. Commun., 1999, 1477–1478 RSC;
(o) B. Baldo, F. Rubio, E. Flores, A. Vega, N. Audebrand, D. Venegas-Yazigi and V. Paredes-Garcia, Ni2[LnCl6] (Ln = EuII, CeII, GdII): the first LnII compounds stabilized in a pure inorganic lattice, Chem. Commun., 2018, 54, 7531–7534 RSC;
(p) B. Baldo, F. Rubio, E. Flores, A. Vega, N. Audebrand, D. Venegas-Yazigi and V. Paredes-Garcia, Retraction: Ni2[LnCl6] (Ln = EuII, CeII, GdII): the first LnII compounds stabilized in a pure inorganic lattice, Chem. Commun., 2019, 55, 13183 RSC.
- In this regard, it is pertinent to note that Donahue reported that a zinc compound had been refined as its Cr, Mo and W counterparts, but this was only possible by refining the heavy atom with partial occupancy such that it could artificially resemble the lighter zinc atom. See:
(a) T. Turki, T. Guerfel and F. Bouachir, Synthesis, characterization and crystal structure of diamagnetic tetracoordinated chromium (II) complexes supported by α-diimine ligands, Inorg. Chem. Commun., 2006, 9, 1023–1025 CrossRef CAS;
(b) T. Turki, T. Guerfel and F. Bouachir, Synthesis and crystal structure of tetracoordinated molybdenum(II) complexes containing rigid α-diimine ligands, Polyhedron, 2006, 25, 1142–1146 CrossRef CAS;
(c) T. Turki, T. Guerfel and F. Bouachir, Preparation and structural characterization of tetracoordinated tungsten(II) diazadiene complexes, J. Organomet. Chem., 2006, 691, 1857–1861 CrossRef CAS;
(d) A. F. Greene, P. Chandrasekaran, Y. Yan, J. T. Mague and J. P. Donahue, Element misidentification in X-ray crystallography: A reassessment of the [MCl2(diazadiene)] (M = Cr, Mo, W) series, Inorg. Chem., 2014, 53, 308–317 CrossRef CAS.
- For an example of a disordered structure in which a half-occupancy Re site is refined as a full-occupancy Tc site, see:
(a) A. K. Burrell, F. A. Cotton, L. M. Daniels and V. Petricek, Structure of crystalline (C5Me5)ReO3 and implied nonexistence of “(C5Me5)Tc2O3”, Inorg. Chem., 1995, 34, 4253–4255 CrossRef CAS;
(b) B. Kanellakopulos, B. Nuber, K. Raptis and M. L. Ziegler, A polymeric technetium compound of the composition [Tc2O3(C5Me5)]n, Angew. Chem., Int. Ed. Engl., 1989, 28, 1055 CrossRef.
- It should also be noted that, in addition to the accidental misidentification of elements, there are also deliberate examples that have resulted in many retractions. See, for example:
(a) Editorial. W. T. A. Harrison, J. Simpson and M. Weil, Acta Crystallogr., Sect. E: Struct. Rep. Online, 2010, 66, e1–e2 CrossRef CAS;
(b) Retraction of articles by H. Zhong et al. H. Zhong, S. H. Duan, Y.-P. Hong, M.-L. Li, Y.-Q. Liu, C.-J. Luo, S.-Z. Xiao, H.-L. Xie, Y.-P. Xu, X.-M. Yang, X.-R. Zeng and Q. Y. Zhong, Acta Crystallogr., Sect. E: Struct. Rep. Online, 2010, 66, e11–e12 CrossRef CAS;
(c) Retraction of articles by T. Liu et al. T. Liu, Y.-X. Wang, Z.-W. Wang, Z.-P. Xie and J. Y. Zhu, Acta Crystallogr., Sect. E: Struct. Rep. Online, 2010, 66, e13–e14 CrossRef CAS;
(d) Retraction of articles, Acta Crystallogr.,
Sect. E: Struct. Rep. Online, 2010, 66, e21–e22 Search PubMed;
(e) Retraction of articles, Acta Crystallogr., Sect. E: Struct. Rep. Online, 2011, 67, e14 Search PubMed.
- In addition to the dichoromethane solvate reported here, [Re(CO)3(C4N2H3S)]4 has also been crystallized as an ethanol solvate (ref. 44a) and as a hydrate when reported as [Cd(CO)3(C6H3Cl)]4 (ref. 4).
-
(a)
G. M. Sheldrick, SHELXTL, An Integrated System for Solving, Refining, and Displaying Crystal Structures from Diffraction Data, University of Göttingen, Göttingen, Federal Republic of Germany, 1981 Search PubMed;
(b) G. M. Sheldrick, A short history of SHELX, Acta Crystallogr., Sect. A: Found. Crystallogr., 2008, 64, 112–122 CrossRef CAS;
(c) G. M. Sheldrick, Crystal structure refinement with SHELXL, Acta Crystallogr., Sect. A: Found. Adv., 2015, 71, 3–8 CrossRef.
-
https://www.platonsoft.nl/platon/
.
-
(a)
Jaguar, version 8.9, Schrodinger, Inc., New York, NY, 2015 Search PubMed;
(b) A. D. Bochevarov, E. Harder, T. F. Hughes, J. R. Greenwood, D. A. Braden, D. M. Philipp, D. Rinaldo, M. D. Halls, J. Zhang and R. A. Friesner, Jaguar: A high-performance quantum chemistry software program with strengths in life and materials sciences, Int. J. Quantum Chem., 2013, 113, 2110–2142 CrossRef CAS.
Footnote |
† Electronic supplementary information (ESI) available: Crystallographic data (CIFs), and Cartesian coordinates for geometry optimized structures. CCDC 2024180 and 2024181. For ESI and crystallographic data in CIF or other electronic format see DOI: 10.1039/d0sc04596a |
|
This journal is © The Royal Society of Chemistry 2020 |
Click here to see how this site uses Cookies. View our privacy policy here.