DOI:
10.1039/D2NJ02634A
(Perspective)
New J. Chem., 2022,
46, 20047-20052
A simple overview of toxicity of ionic liquids and designs of biocompatible ionic liquids
Received
26th May 2022
, Accepted 7th September 2022
First published on 23rd September 2022
Abstract
Evaluation of toxicity of ionic liquids has been less prioritized, compared to the development of new ionic liquids and their applications. Focus on the toxicity of ionic liquids should be enhanced, since ionic liquids have been intensively industrialized for decades. Their toxicity is related to not only biological applications but also others, since accidents, such as leakage, can occur at any time. This current review aims at systematically summarizing the general trend between the chemical structure and toxicity of ionic liquids across biological species. It also provides a perspective on the current strategies for the development of low-toxicity ionic liquids and their applications.
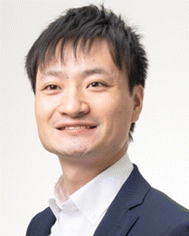
Kosuke Kuroda
| Dr Kosuke Kuroda received his PhD from the Graduate School of Engineering, Tokyo University of Agriculture and Technology in 2014. He then moved to the Graduate School of Natural Science and Technology, Kanazawa University, where he was a research assistant professor. Dr Kuroda established his independent career as an assistant professor in 2017 and as an associate professor in 2020 at the same place. The primary focus of his research is to develop novel ionic liquids, with low toxicity and other functions, such as cellulose dissolution ability. |
1 Introduction
The field of ionic liquids1–6 began with the discovery of ethyl ammonium nitrate in 1914 by Paul Walden.7 It has grown extensively after the groundbreaking report titled “air and water stable ionic liquids” by Wilkes and Zaworotko.8,9 Ionic liquids have been applied and industrialized in various fields, such as batteries,2,10 adsorbents,11,12 biomass conversion,13–15 lubricants,16 stationary and mobile phases of chromatography,17–21 catalysts,22–26 CO2 capture,27 and pharmaceutical/biomedical applications28–30 to date.31 Their toxicity, however, is a concern in all applications, besides their biological applications. For example, in an industrial application, safety concerns arise if humans come in contact with them during the process. Further concerns include problems where ionic residues remain in the final product or leak into the environment. Overall, irrespective of the application, it is difficult to completely ignore the toxicity of ionic liquids.
In the soil, near a landfill in Newcastle, England, [C8mim]+ cations (structure shown in Fig. 1) have been found and reported to be of concern to human health.32,33 The origin of this ionic liquid-like cation is unknown, and no health hazard has been reported to date. However, since ionic liquids are beginning to be used in a wide variety of practical applications, it is imperative to understand their toxicity better. This current review is aimed at discussing the toxicity of ionic liquids and ways to avoid the same.
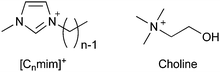 |
| Fig. 1 Cationic structures of ionic liquids relevant to this review. | |
As is quite obvious, “ionic liquid toxicology” is a relatively new field, and many aspects of it are yet to be understood. In addition, the toxicity values vary widely across species, and comparison of each value is difficult. Although there are several advanced reviews34–40 that describe the toxicities in specific species, one-by-one, they are too high level for new entrants and students to comprehend and there is no paper yet providing a simple overview of the general and fundamental toxicological principles across species, except for a review41 published at the primitive stage of this field (2007), to the best of the author's knowledge. The current review is aimed at systematically summarizing the toxicology of ionic liquids as simply as possible, as a mini-review. This would also help the readers to access appropriate references in order to understand the individual toxicity aspects.
2 Toxicity of ionic liquids
2-1. Overview of ionic liquid toxicity
The toxicity of ionic liquids has been studied using a wide range of targets, from proteins to animals. For proteins, acetylcholinesterase, an enzyme related to nerve signaling, is frequently used as a model.34,35 Many reports have been published on other proteins as well.2,34,35,42–48 This current review is focused on living organisms, in particular, cells, which are the smallest units of living organisms, and higher organisms. First, the toxicity of [C8mim]+-based ionic liquids (mentioned in the introduction) is reviewed. EC50, a commonly used indicator of toxicity, is defined as the concentration of ionic liquids at which the growth of cells and microorganisms is halved compared to that without the ionic liquid. In other words, the higher the EC50, the lower the toxicity. For example, the EC50 value of [C8mim]Cl for rat leukemia IPC-81 cells is 0.102 mmol L−1 (23 mg L−1, ≈0.002 wt%).49 This indicates that the addition of only 1 or 2 earpicks of [C8mim]Cl in a 1 liter PET bottle would inhibit cell growth. Since the EC50 of methanol is 1584 mmol L−1 (51000 mg L−1, ≈5 wt%),49 [C8mim]Cl may be considered highly toxic. In fact, many publications have reported the concept of using ionic liquids as sterilizers or anticancer agents.50–52
However, not all ionic liquids are as toxic as [C8mim]Cl; each type of ionic liquid has a different toxicity. The factor most relevant to the toxicity of ionic liquids is the alkyl chain length of the cations. The longer the alkyl chain length of the cation, the more toxic the ionic liquids are. Although there are many classifications of organisms, almost all organisms, including microorganisms,53 animal cells,49 plants,54 crustaceans,55 fish,56 and mammals,57 are affected by the toxicity, as the alkyl chain length of the cation increases. The relationship between the alkyl chain length of ionic liquids and the EC50 against IPC-81 cells is shown in Fig. 2. The EC50 value can be seen to decrease almost exponentially with the elongation of the alkyl chain from 2 to 10 carbons. For the short-chain [C2mim]Cl, the EC50 value is 7.2 mmol L−1 (1100 mg L−1, ≈0.1 wt%), an order of magnitude higher than that of [C8mim]Cl. However, the value is still much smaller than that of methanol, indicating its high toxicity. Therefore, shortening of the alkyl chain length of the cation is not sufficient to reduce toxicity. In other words, the commonly used ionic liquids are basically highly toxic.
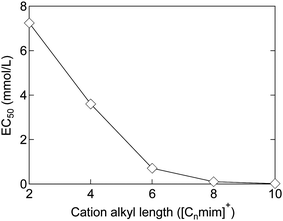 |
| Fig. 2 Relationship between the alkyl chain length and EC50 of [Cnmim]Cl against IPC-81 cells.58,59 | |
When the alkyl chain of the cation is approximately shorter than the butyl group, the cation head and the anion can also affect the toxicity.34 However, the relationship between these structures and toxicity is speculated to be due to complex interrelated factors, and a systematic theory is yet to be developed.
2-2. Toxicity to mammals
Toxicity to mammals (mice and rats) is briefly reviewed, which should be most concerned when used in applications. The dose of [C4mim]Cl at which 50% of rats die (LD50) is reported to be approximately 550 mg kg−1.60 LD50 of methanol and dimethyl sulfoxide (DMSO; a low toxicity organic solvent) is 5600 and 15
000–30
000 mg kg−1, respectively,61,62 indicating [C4mim]Cl to be highly toxic. As an adverse effect besides death, weight gain was observed. The alkyl chain length also has a significant effect on toxicity in mammals.57 The toxicity of [C2mim]Cl was hardly apparent even after multiple doses of 2000 mg kg−1 day−1 in mice. On the other hand, [C10mim]Cl caused death or fetal teratogenicity even at 100 mg kg−1 day−1.
In case of dermal, rather than oral administration, toxicity varies depending on the solvent mixed with the ionic liquid.60,63 When ionic liquids were administered using water as the solvent, LD50 for rats was >2000 mg kg−1 for both males and females. In contrast, when dimethylformamide was used as the solvent, LD50 for female rats was 800–2000 mg kg−1 (for males, >2000 mg kg−1). Other adverse effects include inflammation, decreased activity, red liquid discharge from the eyes, and intestinal discoloration.
When administered orally or transdermally, the majority of the ionic liquids were absorbed into the bloodstream and ultimately excreted relatively quickly from the body as feces and urine.63 This absorption and efflux are believed to be related to organic cation transporters. On the other hand, the absorption and efflux behaviors are reported to be less dependent on cationic species.64,65
The carcinogenicity of ionic liquids has also been investigated in vitro.66 The Ames test, which detects the changes in DNA sequences, suggested that at least some bromide-based ionic liquids are not carcinogenic. However, there are some ionic liquids that show values close to the standard value for a positive result (namely carcinogenic), and further studies would be required in that aspect.
2-3. Mechanism of toxicity
As has been explained above, the longer the alkyl chain of a cation, the higher the toxicity. The mechanism has been described in the literature as follows: the alkyl chains of cations are inserted into the cell membrane, making it impossible to maintain the cell membrane (Fig. 3).67–70 The alkyl chains of cations are inserted via the following steps: (1) a positively charged cation comes in close contact with a negatively charged phosphate group of the cell membrane; (2) the alkyl chain of the cation interacts with the acyl group (long-chain alkyl group) of the cell membrane via hydrophobic interaction and gets inserted into the cell membrane.
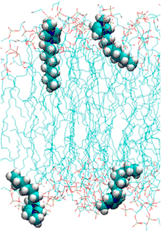 |
| Fig. 3 Cell membrane and the inserted [C8mim]+ cation. Adapted with permission from the literature.67 Copyright 2014 American Chemical Society. | |
To understand the mechanism underlying the toxicity of ionic liquids, the cation of [C2mim]Cl has been suggested to enter the cell via a transporter and affect mitochondria;71,72 it alters the membrane potential of mitochondria, ultimately causing apoptosis (cell death).
3 Designs of low-toxicity ionic liquids
3-1. Introduction of polar groups
Increasing the polarity of alkyl chains of cations can suppress their hydrophobic interactions to cell membrane molecules. For example, introduction of a hydroxy group at the end of alkyl chain improves the EC50 by an order of magnitude or more (Table 1).73,74 The polar group does not necessarily have to be at the end of the alkyl chain; introduction of an oxygen atom in the middle of the alkyl chain can also reduce EC50 greatly.73 However, it should be noted that the EC50 value may not decrease depending on the position of the oxygen atom.
Table 1 Effect of cationic side chains (–R) on EC50 against IPC-81 cells.58,59,73
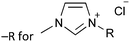
|
EC50 (mmol L−1) |
Ethyl |
7.2 |
Butyl |
3.6 |
3-Hydroxypropyl |
>20 |
Ethoxymethyl |
4.0 |
2-Methoxyethyl |
>20 |
3-2. Bio-derived ionic liquids
Ionic liquids composed of bio-derived ions (sometimes called bionic liquids) are expected to be less toxic. Bio-derived cations are not many in number, and therefore, choline cations are often used. Choline exists as a metabolite of the neurotransmitter, acetylcholine, and as a polar head group of cell membrane molecules (e.g., phosphatidylcholine). Choline cations are at least considerably less toxic than [C4mim]+ cations.75,76 Chloride, acetate, citrate, and amino acid-derived anions have been reported as anions.77,78 Among them, choline ionic liquids with chloride, acetate, glutamate, and aspartate anions have low toxicity, with the minimum growth inhibitory concentration against E. coli being 500–750 mM or higher (e.g., the case of choline chloride: >100 g L−1).76 However, bio-derived ions are not necessarily less toxic; for example, choline tryptophanate is relatively toxic (the minimum growth inhibition concentration being 23 mM, ≈7 g L−1). However, it is still less toxic than typical ionic liquids, such as [C4mim]BF4 (the minimum growth inhibition concentration being 2 mM).
3-3. Zwitterionization
Artificial ionic liquids are not necessarily toxic. Introducing an anionic moiety, the most polar functional group, at the end of a cationic alkyl chain, i.e., zwitterionization,79 can result in very low toxicity.44,80–84 While the approach would be the same as in Section 3-1, the effect would be highly powerful. For example, the EC50 of carboxylate-type zwitterions against E. coli is much higher than that of choline acetate (Table 2).80–82 While choline acetate shows no strong effect on E. coli growth (EC50), it is highly toxic to fermentation (Fig. 4 and Table 2). On the other hand, zwitterions do not show strong toxicity toward either growth or fermentation. This might be caused by the high concentration of acetate, which is known as an inhibitor. The compatibility to fermentation enables applying ionic liquids to various biological conversions such as bioethanol production from cellulosic biomass (see the next section). The results, therefore, indicate that zwitterions have one of the lowest toxicities among the various ionic liquids.
Table 2 EC50 of zwitterions and other solvents against E. coli KO11 and relative ethanol concentration in 0.5 mol L−1 zwitterions and choline acetate.80,82
|
EC50 (g L−1) |
Relative ethanol concentration (%) |
OE2imC3C |
158 |
96 |
C1imC3C |
141 |
100 |
C1imC3S |
>200 |
104 |
Choline acetate |
70 |
15 |
DMSO |
91 |
— |
Ethanol |
17 |
— |
—(Control) |
— |
100 |
 |
| Fig. 4 Structures of zwitterions relevant to this review. | |
4. Applications of low-toxicity ionic liquids: bioethanol production
Various applications of low-toxicity ionic liquids are currently being considered, like other ionic liquids; some are bio-related applications and some are not, and Gomes et al. summarized them broadly.29 Bioethanol production from cellulosic biomass with ionic liquids is another recent development and is briefly reviewed here.
Production of bioethanol involves three steps, namely (1) pretreatment of biomass by ionic liquids, (2) enzymatic or acidic hydrolysis of polysaccharides, and (3) fermentation of glucose by microorganisms, such as yeasts. Although bioethanol can be obtained via three independent steps, it critically increases energy cost and results in negative energy balance. In particular, washing out ionic liquids with a large excess of water/alcohol costs energy. Therefore, successive or simultaneous processes would be required that only low-toxic ionic liquids can realize.
Besides low toxicity, pretreatment ability is an essential characteristic for ionic liquids to convert biomass. Therefore, carboxylate-type ionic liquids are often used for the purpose. The most typical cellulose-dissolving ionic liquid is toxic [C2mim]OAc. Therefore, bio-derived choline acetate has been reported as a low-toxic solvent for biomass pretreatment.80,85 The pretreatment ability of choline acetate is somewhat lower than that of [C2mim]OAc, but it surely pretreats biomass.86–88 The toxic effect of choline acetate on fermentation has been reported in the literature although it is less toxic to growth of microorganisms.80 Choline-type ionic liquids with amino acid anions have been applied to bioethanol production.86,89,90 In particular, research groups at The Joint BioEnergy Institute and related laboratories have been researching enthusiastically for industrialization.13,14 They mainly use choline lysinate and have realized the conversion of tens of kilograms of woody biomass into ethanol.13
Imidazolium/carboxylate-type zwitterions (see Fig. 4) are also promising candidates for efficient bioethanol production.80–82,91,92 Their low toxicity is remarkable, and fermentation can occur in an aqueous solution containing more than 50 wt% of the zwitterion.80 The zwitterions can dissolve cellulose like [C2mim]OAc, which is another advantage in biomass pretreatment. Imidazolium cations play a key role in dissolving cellulose.93,94 Zwitterionization does not compromise any functions of ionic liquids, but it does lower toxicity—this is the most attractive feature of zwitterionization.
5. Conclusion
The term “green solvent,” which is a synonym for ionic liquids, is often confused with “non-toxic”. However, we have outlined in this perspective that ionic liquids are not necessarily non-toxic, rather, depending on their structure, they may be more toxic than organic solvents. We have described, in this review, how to avoid such toxicity. Since ionic liquids are currently in the process of finding many applications, they may come in contact with the human body or might leak into the environment during various processes. If their toxicity is ignored, the end result could lead to environmental destruction and health hazards.
This field, toxicology of ionic liquids, has reached an inflection point because most commercial ionic liquids and synthesizable ionic liquids have already been evaluated. New designs of ionic liquids, based on their toxicity mechanisms, are necessary to develop low-toxicity ionic liquids in future. Development of artificial ionic liquids with low toxicity has been proven through precisely design considering effective mechanisms. In addition, the artificial ionic liquids can be freely modified and therefore easily functionalized, e.g., biomass pretreatment ability. However, the problematic issue in this field is that only few researchers possess the required biological and chemical insight/techniques. Hopefully many researchers will enter this interdisciplinary field; collaborating, preferably, would be a more efficient and synergistic approach.
Conflicts of interest
There are no conflicts to declare.
Acknowledgements
I thank Dr E. Hirata, Dr K. Ninomiya, and Dr Y. Tsuge (Kanazawa Univ.) for their instruction of philosophy on cell/microbial biology. This study was also partly supported by KAKENHI (18K14281 from the Japan Society for the Promotion of Science), Leading Initiative for Excellent Young Researchers (for K. K., from Ministry of Education, Culture, Sports, Science and Technology-Japan), ACT-X (for K.K., JPMJAX1915 from Japan Science and Technology Agency), A-STEP (from Japan Science and Technology Agency), Kanazawa University SAKIGAKE project 2020 and 2022, and NIBB Collaborative Research Program (21-608).
Notes and references
- T. Welton, Chem. Rev., 1999, 99, 2071–2083 CrossRef CAS
.
- M. Armand, F. Endres, D. R. MacFarlane, H. Ohno and B. Scrosati, Nat. Mater., 2009, 8, 621–629 CrossRef CAS
.
- R. D. Rogers and K. R. Seddon, Science, 2003, 302, 792–793 CrossRef PubMed
.
- Z. Lei, B. Chen, Y. M. Koo and D. R. MacFarlane, Chem. Rev., 2017, 117, 6633–6635 CrossRef
.
- B. D. Rabideau, K. N. West and J. H. Davis, Chem. Commun., 2018, 54, 5019–5031 RSC
.
- A. Brandt, J. Gräsvik, J. P. Hallett and T. Welton, Green Chem., 2013, 15, 550–583 RSC
.
- P. Walden, Bull. Acad. Imp. Sci. St.-Petersbourg, 1914, 8, 405–422 Search PubMed
.
- J. S. Wilkes and M. J. Zaworotko, J. Chem. Soc., Chem. Commun., 1992, 965–967 RSC
.
- J. S. Wilkes, Green Chem., 2002, 4, 73–80 RSC
.
- M. Watanabe, M. L. Thomas, S. Zhang, K. Ueno, T. Yasuda and K. Dokko, Chem. Rev., 2017, 117, 7190–7239 CrossRef CAS
.
- H. B. Salah, P. Nancarrow and A. Al-Othman, Fuel, 2021, 302, 121195 CrossRef CAS
.
- M. Yu, S. Zeng, Y. Nie, X. Zhang and S. Zhang, Curr. Opin. Green Sustainable Chem., 2021, 27, 100405 CrossRef CAS
.
- C. A. Barcelos, A. M. Oka, J. Yan, L. Das, E. C. Achinivu, H. Magurudeniya, J. Dong, S. Akdemir, N. R. Baral, C. Yan, C. D. Scown, D. Tanjore, N. Sun, B. A. Simmons, J. Gladden and E. Sundstrom, ACS Sustainable Chem. Eng., 2021, 9, 4042–4053 CrossRef CAS
.
- L. Das, E. C. Achinivu, C. A. Barcelos, E. Sundstrom, B. Amer, E. E. K. Baidoo, B. A. Simmons, N. Sun and J. M. Gladden, ACS Sustainable Chem. Eng., 2021, 9, 4422–4432 CrossRef CAS
.
- H. Wang, G. Gurau and R. D. Rogers, Chem. Soc. Rev., 2012, 41, 1519–1537 RSC
.
- A. Somers, P. Howlett, D. MacFarlane and M. Forsyth, Lubricants, 2013, 1, 3–21 CrossRef
.
- H. Nan and J. L. Anderson, Trends Anal. Chem., 2018, 105, 367–379 CrossRef CAS
.
- Y. Fukaya, A. Tsukamoto, K. Kuroda and H. Ohno, Chem. Commun., 2011, 47, 1994–1996 RSC
.
- K. Kuroda, Y. Fukaya, T. Yamada and H. Ohno, Anal. Methods, 2015, 7, 1719–1726 RSC
.
- M. D. Joshi and J. L. Anderson, RSC Adv., 2012, 2, 5470–5484 RSC
.
-
M. Talebi, R. Patil and D. W. Armstrong, in Commercial Applications of Ionic Liquids, ed. M. B. Shiflett, Springer Nature; Switzerland, 2022, pp. 131–165 Search PubMed
.
- A. C. Cole, J. L. Jensen, I. Ntai, K. L. T. Tran, K. J. Weaver, D. C. Forbes and J. H. Davis, J. Am. Chem. Soc., 2002, 124, 5962–5963 CrossRef CAS
.
- K. Kuroda, K. Miyamura, H. Satria, K. Takada, K. Ninomiya and K. Takahashi, ACS Sustainable Chem. Eng., 2016, 4, 3352–3356 CrossRef CAS
.
- H. Satria, K. Kuroda, T. Endo, K. Takada, K. Ninomiya and K. Takahashi, ACS Sustainable Chem. Eng., 2017, 5, 708–713 CrossRef CAS
.
- R. Kakuchi, M. Yamaguchi, T. Endo, Y. Shibata, K. Ninomiya, T. Ikai, K. Maeda and K. Takahashi, RSC Adv., 2015, 5, 72071–72074 RSC
.
- S. Suzuki, Y. Shibata, D. Hirose, T. Endo, K. Ninomiya, R. Kakuchi and K. Takahashi, RSC Adv., 2018, 8, 21768–21776 RSC
.
- M. Ramdin, T. W. de Loos and T. J. H. Vlugt, Ind. Eng. Chem. Res., 2012, 51, 8149–8177 CrossRef CAS
.
- W. Zhuang, K. Hachem, D. Bokov, M. J. Ansari and A. T. Nakhjiri, J. Mol. Liq., 2022, 349, 118145 CrossRef CAS
.
- J. M. Gomes, S. S. Silva and R. L. Reis, Chem. Soc. Rev., 2019, 48, 4317–4335 RSC
.
- N. Adawiyah, M. Moniruzzaman, S. Hawatulaila and M. Goto, MedChemComm, 2016, 7, 1881–1897 RSC
.
- N. V. Plechkova and K. R. Seddon, Chem. Soc. Rev., 2008, 37, 123–150 RSC
.
- P. M. Probert, A. C. Leitch, M. P. Dunn, S. K. Meyer, J. M. Palmer, T. M. Abdelghany, A. F. Lakey, M. P. Cooke, H. Talbot, C. Wills, W. McFarlane, L. I. Blake, A. K. Rosenmai, A. Oskarsson, R. Figueiredo, C. Wilson, G. E. Kass, D. E. Jones, P. G. Blain and M. C. Wright, J. Hepatol., 2018, 69, 1123–1135 CrossRef CAS PubMed
.
- A. C. Leitch, T. M. Abdelghany, P. M. Probert, M. P. Dunn, S. K. Meyer, J. M. Palmer, M. P. Cooke, L. I. Blake, K. Morse, A. K. Rosenmai, A. Oskarsson, L. Bates, R. S. Figueiredo, I. Ibrahim, C. Wilson, N. F. Abdelkader, D. E. Jones, P. G. Blain and M. C. Wright, Food Chem. Toxicol., 2020, 136, 111069 CrossRef CAS PubMed
.
- K. S. Egorova and V. P. Ananikov, ChemSusChem, 2014, 7, 336–360 CrossRef CAS PubMed
.
- C. W. Cho, T. P. T. Pham, Y. Zhao, S. Stolte and Y. S. Yun, Sci. Total Environ., 2021, 786, 147309 CrossRef CAS PubMed
.
- J. Ranke, S. Stolte, R. Störmann, J. Arning and B. Jastorff, Chem. Rev., 2007, 107, 2183–2206 CrossRef CAS PubMed
.
- T. P. Pham, C. W. Cho and Y. S. Yun, Water Res., 2010, 44, 352–372 CrossRef CAS PubMed
.
- A. R. P. Gonçalves, X. Paredes, A. F. Cristino, F. J. V. Santos and C. Queirós, Int. J. Mol. Sci., 2021, 22, 5612 CrossRef
.
- J. Flieger and M. Flieger, Int. J. Mol. Sci., 2020, 21, 4253 CrossRef CAS
.
- B. Kudłak, K. Owczarek and J. Namieśnik, Environ. Sci. Pollut. Res. Int., 2015, 22, 11975–11992 CrossRef
.
- D. Zhao, Y. Liao and Z. Zhang, Clean: Soil, Air, Water, 2007, 35, 42–48 CAS
.
- T. Itoh, Chem. Rev., 2017, 117, 10567–10607 CrossRef CAS
.
- K. Fujita, Y. Nikawa and H. Ohno, Chem. Commun., 2013, 49, 3257–3259 RSC
.
- K. Kuroda, C. Kodo, K. Ninomiya and K. Takahashi, Aust. J. Chem., 2019, 72, 139–143 CrossRef CAS
.
- K. Fujita, D. R. MacFarlane, M. Forsyth, M. Yoshizawa-Fujita, K. Murata, N. Nakamura and H. Ohno, Biomacromolecules, 2007, 8, 2080–2086 CrossRef CAS PubMed
.
- S. K. Shukla and J. P. Mikkola, Front. Chem., 2020, 8, 598662 CrossRef CAS
.
- L. Bui-Le, C. J. Clarke, A. Bröhl, A. P. S. Brogan, J. A. J. Arpino, K. M. Polizzi and J. P. Hallett, Commun. Chem., 2020, 3, 55 CrossRef CAS
.
- T. Hirata, T. Takekiyo, Y. Yoshimura, Y. Tokoro, T. Ishizaki, Y. Kizuka and K. Kuroda, RSC Adv., 2022, 12, 11628–11631 RSC
.
- J. Ranke, K. Mölter, F. Stock, U. Bottin-Weber, J. Poczobutt, J. Hoffmann, B. Ondruschka, J. Filser and B. Jastorff, Ecotoxicol. Environ. Saf., 2004, 58, 396–404 CrossRef CAS PubMed
.
- J. Gravel and A. R. Schmitzer, Org. Biomol. Chem., 2017, 15, 1051–1071 RSC
.
- A. R. Dias, J. Costa-Rodrigues, M. H. Fernandes, R. Ferraz and C. Prudêncio, ChemMedChem, 2017, 12, 11–18 CrossRef CAS PubMed
.
- J. Pernak, K. Sobaszkiewicz and I. Mirska, Green Chem., 2003, 5, 52–56 RSC
.
- S. M. Lee, W. J. Chang, A. R. Choi and Y. M. Koo, Korean J. Chem. Eng., 2005, 22, 687–690 CrossRef CAS
.
- B. Jastorff, K. Mölter, P. Behrend, U. Bottin-Weber, J. Filser, A. Heimers, B. Ondruschka, J. Ranke, M. Schaefer, H. Schröder, A. Stark, P. Stepnowski, F. Stock, R. Störmann, S. Stolte, U. Welz-Biermann, S. Ziegert and J. Thöming, Green Chem., 2005, 7, 362–372 RSC
.
- M. Yu, S.-H. Wang, Y.-R. Luo, Y.-W. Han, X.-Y. Li, B.-J. Zhang and J.-J. Wang, Ecotoxicol. Environ. Saf., 2009, 72, 1798–1804 CrossRef CAS PubMed
.
- N. F. M. Hafez, M. I. A. Mutalib, M. A. B. Bustam, M. El-Harbawi and J.-M. Leveque, Procedia Eng., 2016, 148, 830–838 CrossRef CAS
.
- M. M. Bailey, P. L. Jernigan, M. B. Henson, J. Sturdivant, J. F. Rasco, A. N. Lovich, J. E. Lockhard, W. L. Hough, K. R. Di Bona, J. Beaird, J. Sherrill, R. P. Swatloski, R. D. Rogers and R. D. Hood, Birth Defects Res., Part B, 2010, 89, 233–238 CAS
.
- J. Ranke, A. Müller, U. Bottin-Weber, F. Stock, S. Stolte, J. Arning, R. Störmann and B. Jastorff, Ecotoxicol. Environ. Saf., 2007, 67, 430–438 CrossRef CAS PubMed
.
- M. H. Fatemi and P. Izadiyan, Chemosphere, 2011, 84, 553–563 CrossRef CAS PubMed
.
- T. D. Landry, K. Brooks, D. Poche and M. Woolhiser, Bull. Environ. Contam. Toxicol., 2005, 74, 559–565 CrossRef CAS PubMed
.
- S. W. Jacob and E. E. Rosenbaum, Headache: J. Head Face Pain, 1966, 6, 127–136 CrossRef CAS PubMed
.
- Safety Data Sheet, Methanol, Tokyo Chemical Industry Co., Ltd. Revision Number 2, downloaded on Dec 27, 2021.
- I. G. Sipes, G. A. Knudsen and R. K. Kuester, Drug Metab. Dispos., 2008, 36, 284–293 CrossRef CAS PubMed
.
- Y. Cheng, S. H. Wright, M. J. Hooth and I. G. Sipes, Drug Metab. Dispos., 2009, 37, 909–916 CrossRef CAS PubMed
.
- G. A. Knudsen, Y. Cheng, R. K. Kuester, M. J. Hooth and I. G. Sipes, Drug Metab. Dispos., 2009, 37, 2171–2177 CrossRef CAS PubMed
.
- K. M. Docherty, S. Z. Hebbeler and C. F. Kulpa Jr., Green Chem., 2006, 8, 560–567 RSC
.
- G. S. Lim, J. Zidar, D. W. Cheong, S. Jaenicke and M. Klähn, J. Phys. Chem. B, 2014, 118, 10444–10459 CrossRef CAS PubMed
.
- R. J. Bingham and P. Ballone, J. Phys. Chem. B, 2012, 116, 11205–11216 CrossRef CAS PubMed
.
- B. Jing, N. Lan, J. Qiu and Y. Zhu, J. Phys. Chem. B, 2016, 120, 2781–2789 CrossRef CAS PubMed
.
- C. M. N. Mendonça, D. T. Balogh, S. C. Barbosa, T. E. Sintra, S. P. M. Ventura, L. F. G. Martins, P. Morgado, E. J. M. Filipe, J. A. P. Coutinho, O. N. Oliveira Jr. and A. Barros-Timmons, Phys. Chem. Chem. Phys., 2018, 20, 29764–29777 RSC
.
- Q. Dickinson, S. Bottoms, L. Hinchman, S. McIlwain, S. Li, C. L. Myers, C. Boone, J. J. Coon, A. Hebert, T. K. Sato, R. Landick and J. S. Piotrowski, Microb. Cell Fact., 2016, 17, 5 Search PubMed
.
- S. Wu, L. Zeng, C. Wang, Y. Yang, W. Zhou, F. Li and Z. Tan, J. Hazard. Mater., 2018, 348, 1–9 CrossRef CAS PubMed
.
- S. Stolte, J. r Arning, U. Bottin-Weber, A. Müller, W.-R. Pitner, U. Welz-Biermann, B. Jastorff and J. Ranke, Green Chem., 2007, 9, 760–767 RSC
.
- B. Jastorff, K. Mölter, P. Behrend, U. Bottin-Weber, J. Filser, A. Heimers, B. Ondruschka, J. Ranke, M. Schaefer, H. Schröder, A. Stark, P. Stepnowski, F. Stock, R. Störmann, S. Stolte, U. Welz-Biermann, S. Ziegert and J. Thöming, Green Chem., 2005, 7, 362–372 RSC
.
- W. Gouveia, T. F. Jorge, S. Martins, M. Meireles, M. Carolino, C. Cruz, T. V. Almeida and M. E. Araujo, Chemosphere, 2014, 104, 51–56 CrossRef CAS PubMed
.
- X. D. Hou, Q. P. Liu, T. J. Smith, N. Li and M. H. Zong, PLoS One, 2013, 8, e59145 CrossRef CAS PubMed
.
- Y. Fukaya, Y. Iizuka, K. Sekikawa and H. Ohno, Green Chem., 2007, 9, 1155–1157 RSC
.
- S. Hu, T. Jiang, Z. Zhang, A. Zhu, B. Han, J. Song, Y. Xie and W. Li, Tetrahedron Lett., 2007, 48, 5613–5617 CrossRef CAS
.
- M. Yoshizawa, M. Hirao, K. Ito-Akita and H. Ohno, J. Mater. Chem., 2001, 11, 1057–1062 RSC
.
- K. Kuroda, H. Satria, K. Miyamura, Y. Tsuge, K. Ninomiya and K. Takahashi, J. Am. Chem. Soc., 2017, 139, 16052–16055 CrossRef CAS PubMed
.
- H. Satria, K. Kuroda, Y. Tsuge, K. Ninomiya and K. Takahashi, New J. Chem., 2018, 42, 13225–13228 RSC
.
- T. Komori, H. Satria, K. Miyamura, A. Ito, M. Kamiya, A. Sumino, T. Onishi, K. Ninomiya, K. Takahashi, J. L. Anderson, T. Uto and K. Kuroda, ACS Sustainable Chem. Eng., 2021, 9, 11825–11836 CrossRef CAS
.
- K. Kuroda, Y. Kohno and H. Ohno, Chem. Lett., 2017, 46, 870–872 CrossRef CAS
.
- F. Jesus, H. Passos, A. M. Ferreira, K. Kuroda, J. L. Pereira, F. J. M. Gonçalves, J. A. P. Coutinho and S. P. M. Ventura, Green Chem., 2021, 23, 3683–3692 RSC
.
- K. Ninomiya, C. Ogino, M. Ishizaki, M. Yasuda, N. Shimizu and K. Takahashi, Biochem. Eng. J., 2015, 103, 198–204 CrossRef CAS
.
- N. Sun, R. Parthasarathi, A. M. Socha, J. Shi, S. Zhang, V. Stavila, K. L. Sale, B. A. Simmons and S. Singh, Green Chem., 2014, 16, 2546–2557 RSC
.
- K. Ninomiya, T. Yamauchi, M. Kobayashi, C. Ogino, N. Shimizu and K. Takahashi, Biochem. Eng. J., 2013, 71, 25–29 CrossRef CAS
.
- K. Ninomiya, H. Soda, C. Ogino, K. Takahashi and N. Shimizu, Bioresour. Technol., 2013, 128, 188–192 CrossRef CAS PubMed
.
- X. D. Hou, T. J. Smith, N. Li and M. H. Zong, Biotechnol. Bioeng., 2012, 109, 2484–2493 CrossRef CAS PubMed
.
- Q. P. Liu, X. D. Hou, N. Li and M. H. Zong, Green Chem., 2012, 14, 304–307 RSC
.
- A. Hachisu, H. Tobe, K. Ninomiya, K. Takahashi and K. Kuroda, ACS Sustainable Chem. Eng., 2022, 10, 6919–6924 CrossRef CAS
.
- R. Kadokawa, T. Endo, Y. Yasaka, K. Ninomiya, K. Takahashi and K. Kuroda, ACS Sustainable Chem. Eng., 2021, 9, 8686–8691 CrossRef CAS
.
- B. D. Rabideau, A. Agarwal and A. E. Ismail, J. Phys. Chem. B, 2013, 117, 3469–3479 CrossRef CAS PubMed
.
- T. Uto, K. Yamamoto and J. I. Kadokawa, J. Phys. Chem. B, 2018, 122, 258–266 CrossRef CAS PubMed
.
|
This journal is © The Royal Society of Chemistry and the Centre National de la Recherche Scientifique 2022 |
Click here to see how this site uses Cookies. View our privacy policy here.