DOI:
10.1039/D3SC03309K
(Edge Article)
Chem. Sci., 2023,
14, 9452-9460
Azido-alkynylation of alkenes through radical-polar crossover†
Received
29th June 2023
, Accepted 10th August 2023
First published on 11th August 2023
Abstract
We report an azido-alkynylation of alkenes allowing a straightforward access to homopropargylic azides by combining hypervalent iodine reagents and alkynyl-trifluoroborate salts. The design of a photocatalytic redox-neutral radical polar crossover process was key to develop this transformation. A variety of homopropargylic azides possessing electron-rich and -poor aryls, heterocycles or ether substituents could be accessed in 34–84% yield. The products are synthetically useful building blocks that could be easily transformed into pyrroles or bioactive amines.
Introduction
The azide moiety is widely recognized as a versatile functional group (FG) and it has found broad application in the pharmaceutical industry1 and in material science.2 It is both a form of protected amine and a powerful synthetic handle with a unique downstream chemistry.3 In recent years, the renaissance of radical chemistry triggered by photoredox catalysis led to the development of novel methods to introduce azides in organic molecules.4 In particular, the formation of azide radicals and their addition onto alkenes was demonstrated to be an efficient strategy for the synthesis of difunctionalized products (Scheme 1A).4a,f After addition of the azide radical, different substituents including heteroatoms5 and aryls6 have been introduced on the intermediate carbon-centered radical. This represents a powerful strategy to quickly gain molecular complexity with the benefit of a highly regiospecific outcome resulting from the formation of the more stable carbon-centered radical.
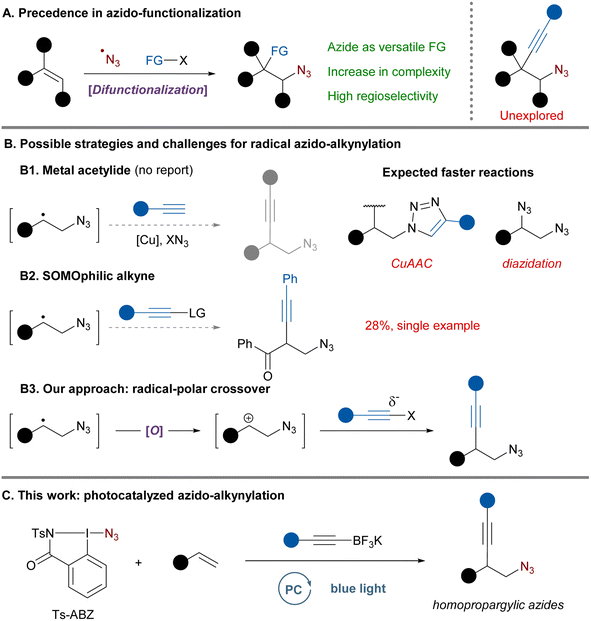 |
| Scheme 1 Azido-functionalization state of the art and challenges. | |
Among all the difunctionalization methods developed, one of the potentially most useful – the azido-alkynylation – has surprisingly not yet been explored, except for the single example of the azido-alkynylation of phenyl-vinyl ketone in 28% yield reported as part of a mechanistic study (Scheme 1B).7 Alkynes are highly useful handles for further derivatization via cycloaddition or other triple bond functionalization methods.8 In this specific case, the resulting homopropargylic azides are interesting synthetic intermediates known to undergo cyclization to form pyrroles.9 Moreover, upon reduction they would afford homopropargylic amines which can be found in bioactive molecules.10 Homopropargylic azides are currently accessed from epoxides using a sequence of ring opening with lithium acetylide, mesylation of the resulting alcohol and displacement by azide anions.9d,f Consequently, more direct synthetic approaches to access such motifs would be of general interest and high synthetic value.
Developing a radical azido-alkynylation of alkenes would initially involve azide radical addition to the double bond. From there multiple approaches could be envisaged to transfer the alkyne to the intermediate carbon radical (Scheme 1B).11 Classical strategies based on the recombination with a metal acetylide followed by reductive elimination12 would not be compatible in the case of azidation (Scheme 1B1). Copper acetylides are the most classical intermediates used in this chemistry but the presence of azides, free alkynes and copper would lead to cycloaddition reactions.1b Additionally, copper catalysts and different azide sources are known to effectively promote the diazidation of alkenes, often proceeding via radical intermediates.13 It is therefore not surprising that no azido-alkynylation following this mechanism has been reported so far. A second approach solely based on open-shell species would use SOMOphilic alkynes in an addition–elimination process to provide the desired product (Scheme 1B2).12a,14 Nevertheless, this system would have major limitations as commonly used alkyne-transfer reagents (ethynylbenziodoxolone and alkynyl sulfones) often require aryl substituents to perform efficiently.15 Moreover, while frequently used to trap alkyl radicals, only a few examples exist for more stabilized benzylic radicals and are often associated with a lower yield,16 a narrow scope17 or a high excess of radical.18 All those factors could explain why there is only one report of such an approach for azido-alkynylation proceeding in 28% yield on phenyl vinyl ketone as a very activated substrate.7
In order to overcome this gap in existing synthetic methodologies, we thought of an alternative pathway involving the merger of radical and polar chemistry.19 Transformations involving radical-polar crossover (RPC) mechanisms have recently received increased attention as they enable the combination of orthogonal reagents only active in either radical or polar regime. Additionally, redox-neutral processes can be developed by careful design of the catalytic cycle. Upon oxidation of the intermediate C-centered radical, the carbocation formed could be trapped by a nucleophilic alkyne affording the desired product (Scheme 1B3). In fact, Xu20 and Molander21 elegantly demonstrated that alkene radical cations and benzylic carbocations could be trapped by nucleophilic trifluoroborate salts. In addition, rare cases of RPC reactions involving azide radicals have been reported, but the nucleophiles were limited to methanol,13b carboxylic acids22 or alkyl groups during a semipinacol rearrangement.23
Although the envisaged 3-component synthesis of homopropargylic azides based on a RPC approach looks promising, there are still significant challenges to overcome: a non-nucleophilic azide radical precursor needs to be selected to limit diazidation and a nucleophilic alkyne efficient enough for carbocation trapping before decomposition is required. Herein, we report the photocatalyzed azido-alkynylation of styrenes using the combination of an azidoiodane reagent and alkynyl-trifluoroborate salts (Scheme 1C). Using this radical-polar crossover strategy a large variety of homopropargylic azides could be accessed in a single step.
Results and discussion
Following optimization studies, the azido-alkynylation of styrene 1a was achieved using Ts-ABZ as azide radical source upon single electron reduction. This hypervalent iodine reagent is a safer version of the more commonly used azidobenziodoxolone, also known as Zhdankin reagent,24 which showed an explosion hazard.23 Potassium alkynyl-trifluoroborates were selected as nucleophilic alkynes as they had been previously employed for the trapping of similar carbocations.20,21 The reaction was performed under photoredox conditions with BF3·Et2O as additive to afford 3a in 74% yield after 1.5 hours at −20 °C (Table 1, entry 1).
Table 1 Reaction optimizationa
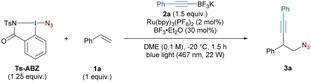
|
Entry |
Variation from standard conditions |
Yieldb (%) |
Reactions were carried out on 0.1 mmol scale. Light irradiation was carried out using a single Kessil lamp.
NMR yield determined using CH2Br2 as internal standard.
|
1 |
None |
74 |
2 |
Ts-ABZ as limiting reagent |
69 |
3 |
2a as limiting reagent |
44 |
4 |
C = 0.05 M |
74 |
5 |
C = 0.2 M |
60 |
6 |
Room temperature |
50 |
7 |
No BF3·Et2O |
49 |
8 |
No light or photocatalyst |
<5 |
Both styrene (1a) and Ts-ABZ can be used as the limiting reagent, but a lower yield was observed when 1.0 equivalent of 2a was used (entries 1–3). Lower and higher concentrations had little to no impact on the reaction outcome (entries 4 and 5). Raising the temperature to 21 °C led to a decrease in yield (entry 6). A similar result was observed when BF3·Et2O was not added (entry 7). Finally, control experiments in the absence of light or photocatalyst afforded only traces of the desired product (entry 8). Full optimization tables, including screening of photocatalyst, solvents, equivalents, light sources and additives can be found in the ESI (Tables S1–S8).†
With optimized conditions in hand, the scope of styrenes was investigated (Scheme 2). In all reactions, full conversion of the alkene was achieved. As no other small molecule side products were observed, we assign the different isolated yields observed to different levels of oligomerization/polymerization of the alkenes. The model substrate 3a was obtained in 73% yield on a 0.3 mmol scale. Styrenes bearing a tert-butyl or a phenyl group in para position gave products 3b and 3c in 78% and 76% yield, respectively. Steric hindrance on the aryl ring was well tolerated as 3d and 3e possessing one or two ortho substituents could be obtained in >78% yield. Oxygen-substituted aryls with substituents such as methoxy and acetoxy could also be used (3f–g). A slight decreased in yield was observed in the presence of the medicinal chemistry relevant trifluoromethoxy substituent (3h).25 Pleasingly, the presence of nucleophilic functional groups, which could have compete with 2a for the trapping of the carbocation, did not hinder the reaction: products 3i or 3j bearing an acetamide and a free phenol could be accessed in 68% and 47% yield, respectively. Additionally, an X-ray structure of 3i was obtained. Halogen-substituted arenes afforded the corresponding azido-alkynylated products 3k–l in 71% and 53% yield, respectively.
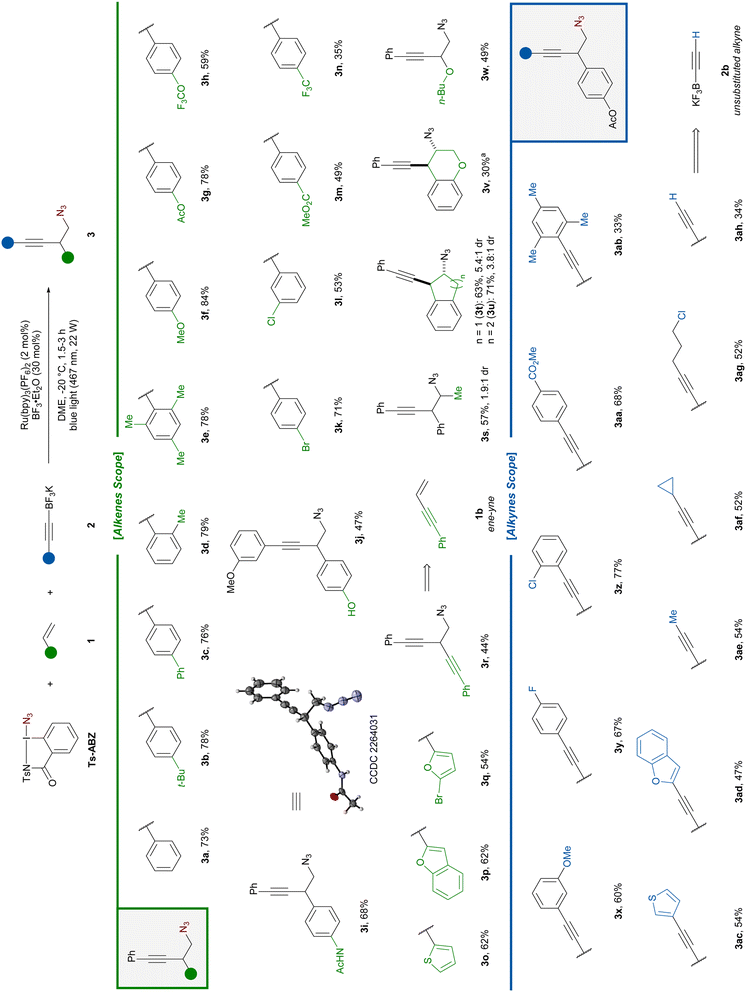 |
| Scheme 2 Scope of the azido-alkynylation. Reaction conditions: 1 (1 equiv.), 2 (1.5 equiv.), Ts-ABZ (1.25 equiv.), Ru(bpy)3(PF6)2 (2 mol%), BF3·Et2O (30 mol%), DME (0.1 M), −20 °C, blue light (467 nm, 22 W), 1.5 to 3 h. The major diastereoisomer is drawn, the dr was determined on the crude reaction mixture by 1H NMR. aOnly diastereoisomer observed. | |
Electron-withdrawing group (EWG), which could be expected to destabilize the carbocation intermediate, were still tolerated in the reaction. Substrates bearing a para ester and CF3 group afforded the corresponding products 3m and 3n in 49% and 35% yield. Moreover, homopropargylic azides 3o–p containing electron-rich heterocycles such as thiophene and benzofuran were obtained in 62% yield. Carrying out the reaction on a sensitive bromo-substituted vinyl-furan, which quickly polymerizes after synthesis, successfully gave product 3q in 54% yield.
Eneyne 1b could be exclusively 1,2-functionalized to give diyne 3r in 44% yield. The reaction tolerated β-substitution on the styrene: product 3s bearing a methyl substituent was obtained in 57% yield as a 1.9
:
1 mixture of diastereoisomers. Using the less flexible cyclic indene, the diastereoselectivity of the reaction could be increased to 5.4
:
1 in favor of the trans3t isomer. Increasing the ring size slightly improved the yield, but lowered the dr (3u). When chromene was used, the azido-alkynylated product 3v was formed in 30% yield. No other diastereoisomer was observed. Gratifyingly, vinyl butyl ether could be azido-alkynylated to afford 3w in 49% yield. Unfortunately, alkenes bearing aliphatic substituents only could not be used.
Next, the scope of nucleophilic alkynes was studied. Aryl-alkynes bearing either EDG (OMe) or EWG (F, Cl, CO2Me) at different positions gave the corresponding products 3x–aa in 60–77% yield. In this case, the steric hindrance of the nucleophile seems to be an important factor as the use of mesityl-alkyne led to 3ab in only 33% yield. We were pleased to see that heteroaryl such at 3-thiophene or 2-benzofuran afforded the desired product 3ac and 3ad in 54% and 47% yield, respectively. Alkyl-substituted alkynes bearing methyl, cyclopropyl or a propyl chain possessing a chloride were well tolerated affording the corresponding products 3ae–ag in 52–54% yields. Finally, using unsubstituted alkynyl-BF3K 2b, terminal alkyne 3ah was obtained in 34% yield allowing for potential further diversification via cross-coupling.
To demonstrate the synthetic utility of the homopropargylic azides, various post functionalizations were carried out (Scheme 3). First, the azido-alkynylation was performed on 1 mmol scale using styrene 1c bearing a para phenoxy group affording the desired product 3ai in 80% yield under the same reaction conditions. Further reduction of the azide afforded primary amine 4 in high yield. The corresponding HCl salt is a known agonist for G protein-coupled receptors currently synthesized through a 4-step sequence in 19% overall yield.10d
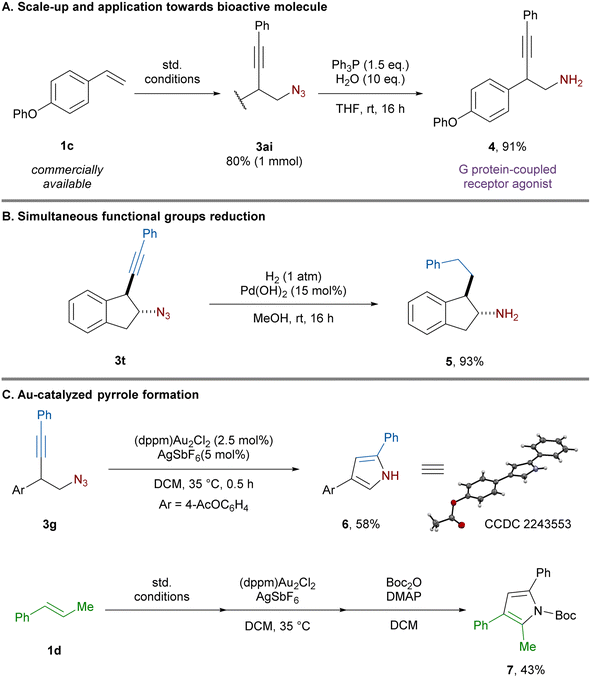 |
| Scheme 3 Product modifications. | |
Upon reduction of both the alkyne and azide, 5 was obtained in 93% yield affording a formal 2-step amino-alkylation. Pyrroles play a crucial role in the pharmaceutical industry as they are one of the most frequently encountered heterocycles in bioactive compounds.26 Applying conditions developed by Toste using gold catalysis,9a homopropargylic azide 3g underwent 5-endo-dig cyclization to afford 6. Non-cyclic β-substituted alkenes afforded poor diastereoselectivity in the azido-alkynylation reaction (Scheme 2, 3s). This issue is inconsequential for pyrrole synthesis, as all stereoisomers are converted in a single product. For example, styrene 1d was effectively converted to trisubstituted pyrrole 7 in 43% yield over a 3 step-sequence of azido-alkynylation, cyclization and protection.
To gain insight into the reaction mechanism, control experiments were performed. In the absence of light and photocatalyst only traces of the product could be obtained (Table 1, entry 8). Replacing the alkyne nucleophile by diphenyl phosphate led to the formation of azido-phosphonylated product 8 in 59% yield, presumably resulting from trapping of the carbocation intermediate (Scheme 4A).
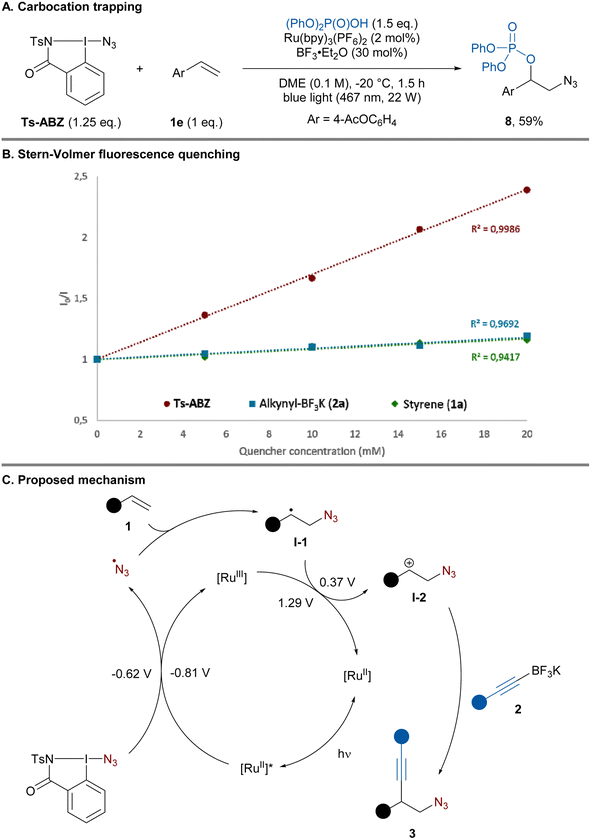 |
| Scheme 4 Mechanistic experiments and proposed mechanism. | |
Next, Stern–Volmer quenching experiments were performed. Ts-ABZ proved to be the most efficient quencher of the excited state photocatalyst compared to alkyne 2a and styrene 1a (Scheme 4B). Based on these experiments and literature precedents, a plausible mechanism could be proposed (Scheme 4C).13b,20,21 Under blue light irradiation, excited state Ru(bpy)32+* (E1/2 [RuIII/RuII*] = −0.86 V vs. SCE)27 is capable of reducing Ts-ABZ (E1/2red = −0.62 V vs. SCE)28 generating the azide radical. Addition of the latter to alkene 1 would lead to carbon-centered radical I-1 (E1/2ox = 0.37 V vs. SCE)29 which can be oxidized by the previously formed Ru(bpy)3+ (E1/2 [RuIII/RuII] = + 1.29 V vs. SCE)27 regenerating the ground state photocatalyst. Finally, the resulting carbocation I-2 would be trapped by the nucleophilic alkynyl-BF3K 2 affording homopropargylic azide 3. Establishing the mechanism of this addition step would need further studies, but a concerted C–C bond formation and C–B bond cleavage could be operative, in analogy to what has been proposed for alkenyl boronate salts.20 The exact role of BF3·Et2O is still unclear, it is known to abstract fluoride from alkynyl-BF3K to form alkynyl-BF2.30 Control experiment involving preformation of alkynyl-BF2 and its subsequent addition instead of BF3·Et2O led to comparable yield hinting at the potential formation of alkynyl-BF2 under the standard conditions (see the ESI† Section 9.3).
Conclusions
In summary, a photocatalyzed azido-alkynylation of alkenes using Ts-ABZ as azide radical source and nucleophilic alkynyl-trifluoroborate salts was developed. The reaction proceeds in high yield for electron-rich and electron-poor styrenes. Various aryl-, alkyl- or unsubstituted alkynes were successfully transferred to generate azido-alkynylated scaffolds. Moreover, heterocycles were compatible on both the alkene and alkyne fragment. The homopropargylic azides could be further derivatized, giving access to valuable pyrroles and the efficient 2-step synthesis of a G protein-coupled receptor agonist. The reaction is proposed to proceed through an overall redox-neutral process via a radical-polar crossover mechanism.31,32
Data availability
ESI† available: Experimental procedures, characterization data and scan of NMR spectra. Crystallographic data is available at CCDC (see note 31). Raw data for compound characterization, including NMR, IR and MS is available at zenodo.org: https://doi.org/10.5281/zenodo.8239023.
Author contributions
J. B. conceived the project, optimized the reaction, performed the investigation on the scope of the reaction, the modification of the products and prepared the experimental parts and first draft of the manuscript. J. W. supervised the project, edited the manuscript and proofread the experimental part.
Conflicts of interest
There are no conflicts to declare.
Acknowledgements
The authors thank Ecole Polytechnique Fédérale de Lausanne for financial support. This publication was created as a part of NCCR Catalysis, a National Center of Competence in Research funded by the Swiss National Science Foundation (Grant No. 180544). Dr Rosario Scopelliti and Dr Farzaneh Fadaei Tirani (ISIC, EPFL) are acknowledged for the X-ray study.
Notes and references
-
(a) H. C. Kolb and K. B. Sharpless, Drug Discov. Today, 2003, 8, 1128–1137 CrossRef CAS PubMed
;
(b) M. Meldal and C. W. Tornøe, Chem. Rev., 2008, 108, 2952–3015 CrossRef CAS PubMed
;
(c) G. C. Tron, T. Pirali, R. A. Billington, P. L. Canonico, G. Sorba and A. A. Genazzani, Med. Res. Rev., 2008, 28, 278–308 CrossRef CAS PubMed
;
(d) J. C. Jewett and C. R. Bertozzi, Chem. Soc. Rev., 2010, 39, 1272–1279 RSC
;
(e) P. Thirumurugan, D. Matosiuk and K. Jozwiak, Chem. Rev., 2013, 113, 4905–4979 CrossRef CAS PubMed
;
(f) A. Herner and Q. Lin, Top. Curr. Chem., 2015, 374, 1 Search PubMed
.
-
(a)
J. Lahann, Click Chemistry for Biotechnology and Materials Science, Wiley, 2009 CrossRef
;
(b) M. Schock and S. Bräse, Molecules, 2020, 25, 1009 CrossRef CAS PubMed
;
(c) K. Li, D. Fong, E. Meichsner and A. Adronov, Chem. –Eur. J., 2021, 27, 5057–5073 CrossRef CAS PubMed
.
-
(a) S. Bräse, C. Gil, K. Knepper and V. Zimmermann, Angew. Chem., Int. Ed., 2005, 44, 5188–5240 CrossRef PubMed
;
(b)
S. Bräse and K. Banert, Organic Azides: Syntheses and Applications, Wiley, 2009 CrossRef
;
(c) H. Tanimoto and K. Kakiuchi, Nat. Prod. Commun., 2013, 8, 1021–1034 CrossRef CAS PubMed
;
(d) D. Huang and G. Yan, Adv. Synth. Catal., 2017, 359, 1600–1619 CrossRef CAS
;
(e) A. A. Nayl, A. A. Aly, W. A. A. Arafa, I. M. Ahmed, A. I. Abd-Elhamid, E. M. El-Fakharany, M. A. Abdelgawad, H. N. Tawfeek and S. Bräse, Molecules, 2022, 27, 3716 CrossRef CAS PubMed
.
-
(a) K. Wu, Y. Liang and N. Jiao, Molecules, 2016, 21, 352 CrossRef PubMed
;
(b) X. Huang and J. T. Groves, ACS Catal., 2016, 6, 751–759 CrossRef CAS
;
(c) M. Goswami and B. de Bruin, Eur. J. Org. Chem., 2017, 2017, 1152–1176 CrossRef CAS PubMed
;
(d) L. Ge, M.-F. Chiou, Y. Li and H. Bao, Green Synth. Catal., 2020, 1, 86–120 CrossRef
;
(e) P. Sivaguru, Y. Ning and X. Bi, Chem. Rev., 2021, 121, 4253–4307 CrossRef CAS PubMed
;
(f) M. Shee and N. D. P. Singh, Chem. Soc. Rev., 2022, 51, 2255–2312 RSC
.
- Selected examples:
(a) B. Zhang and A. Studer, Org. Lett., 2013, 15, 4548–4551 CrossRef CAS PubMed
;
(b) Z. Li, C. Zhang, L. Zhu, C. Liu and C. Li, Org. Chem. Front., 2014, 1, 100–104 RSC
;
(c) X. Sun, X. Li, S. Song, Y. Zhu, Y.-F. Liang and N. Jiao, J. Am. Chem. Soc., 2015, 137, 6059–6066 CrossRef CAS PubMed
;
(d) R. Zhu and S. L. Buchwald, J. Am. Chem. Soc., 2015, 137, 8069–8077 CrossRef CAS PubMed
;
(e) P. Wang, Y. Luo, S. Zhu, D. Lu and Y. Gong, Adv. Synth. Catal., 2019, 361, 5565–5575 CrossRef CAS
.
- Selected examples:
(a) K. Matcha, R. Narayan and A. P. Antonchick, Angew. Chem., Int. Ed., 2013, 52, 7985–7989 CrossRef CAS PubMed
;
(b) W. Kong, E. Merino and C. Nevado, Angew. Chem., Int. Ed., 2014, 53, 5078–5082 CrossRef CAS PubMed
;
(c) J. Chen, S. Zhu, J. Qin and L. Chu, Chem. Commun., 2019, 55, 2336–2339 RSC
;
(d) J. M. Lear, J. Q. Buquoi, X. Gu, K. Pan, D. N. Mustafa and D. A. Nagib, Chem. Commun., 2019, 55, 8820–8823 RSC
.
- A single example of this transformation was reported in low yield as a control experiment during the development of an hydroazidation: R. P. Shirke and S. S. V. Ramasastry, Org. Lett., 2017, 19, 5482–5485 CrossRef CAS PubMed
.
-
(a)
F. Diederich, P. J. Stang and R. R. Tykwinski, Acetylene Chemistry: Chemistry, Biology, and Material Science, Wiley-VCH Verlag, 2005 Search PubMed
;
(b)
B. M. Trost and C.-J. Li, Modern Alkyne Chemistry: Catalytic and Atom-Economic Transformations, Wiley-VCH Verlag, 2014 Search PubMed
;
(c) S. Bhunia, P. Ghosh and S. R. Patra, Adv. Synth. Catal., 2020, 362, 3664–3708 CrossRef CAS
;
(d) W. Liu and W. Kong, Org. Chem. Front., 2020, 7, 3941–3955 RSC
;
(e) N. Chalotra, J. Kumar, T. Naqvi and B. A. Shah, Chem. Commun., 2021, 57, 11285–11300 RSC
.
-
(a) D. J. Gorin, N. R. Davis and F. D. Toste, J. Am. Chem. Soc., 2005, 127, 11260–11261 CrossRef CAS PubMed
;
(b) K. Hiroya, S. Matsumoto, M. Ashikawa, K. Ogiwara and T. Sakamoto, Org. Lett., 2006, 8, 5349–5352 CrossRef CAS PubMed
;
(c) C. A. Witham, P. Mauleón, N. D. Shapiro, B. D. Sherry and F. D. Toste, J. Am. Chem. Soc., 2007, 129, 5838–5839 CrossRef CAS PubMed
;
(d) P. Wyrębek, A. Sniady, N. Bewick, Y. Li, A. Mikus, K. A. Wheeler and R. Dembinski, Tetrahedron, 2009, 65, 1268–1275 CrossRef
;
(e) H. Yamamoto, I. Sasaki, M. Mitsutake, A. Karasudani, H. Imagawa and M. Nishizawa, Synlett, 2011, 2011, 2815–2818 CrossRef
;
(f) J. Tian, K. Feng, K.-N. Yuan, X. Li, H.-H. Chang and W.-C. Gao, J. Org. Chem., 2022, 87, 2402–2409 CrossRef CAS PubMed
.
-
(a) L. I. Kruse, C. Kaiser, W. E. DeWolf, P. A. Chambers, P. J. Goodhart, M. Ezekiel and E. H. Ohlstein, J. Med. Chem., 1988, 31, 704–706 CrossRef CAS PubMed
;
(b) A. N. Shaw, R. E. Dolle and L. I. Kruse, Tetrahedron Lett., 1990, 31, 5081–5084 CrossRef CAS
;
(c)
J.-N. Xiang, I. K. Osifo, J. M. Karpinski and S. B. Christensen, WO2000009115A1, 2000
;
(d) E. S. Tan, M. Miyakawa, J. R. Bunzow, D. K. Grandy and T. S. Scanlan, J. Med. Chem., 2007, 50, 2787–2798 CrossRef CAS PubMed
.
- J. Huang and Z.-M. Chen, Chem. –Eur. J., 2022, 28, e202201519 CAS
.
-
(a) F. Le Vaillant and J. Waser, Chem. Sci., 2019, 10, 8909–8923 RSC
;
(b) Z. Zhang, P. Chen and G. Liu, Chem. Soc. Rev., 2022, 51, 1640–1658 RSC
.
-
(a) M.-Z. Lu, C.-Q. Wang and T.-P. Loh, Org. Lett., 2015, 17, 6110–6113 CrossRef CAS PubMed
;
(b) G. Fumagalli, P. T. G. Rabet, S. Boyd and M. F. Greaney, Angew. Chem., Int. Ed., 2015, 54, 11481–11484 CrossRef CAS PubMed
;
(c) H. Zhou, W. Jian, B. Qian, C. Ye, D. Li, J. Zhou and H. Bao, Org. Lett., 2017, 19, 6120–6123 CrossRef CAS PubMed
;
(d) C.-Y. Cai, Y.-T. Zheng, J.-F. Li and H.-C. Xu, J. Am. Chem. Soc., 2022, 144, 11980–11985 CrossRef CAS PubMed
.
- D. Ge, X. Wang and X.-Q. Chu, Org. Chem. Front., 2021, 8, 5145–5164 RSC
.
- Selected examples for ethynylbenziodoxolone:
(a) Q.-Q. Zhou, W. Guo, W. Ding, X. Wu, X. Chen, L.-Q. Lu and W.-J. Xiao, Angew. Chem., Int. Ed., 2015, 54, 11196–11199 CrossRef CAS PubMed
;
(b) Z. Liu, Y. Pan, P. Zou, H. Huang, Y. Chen and Y. Chen, Org. Lett., 2022, 24, 5951–5956 CrossRef CAS PubMed
;
(c) K. Lu, Y. Ma, S. Liu, S. Guo and Y. Zhang, Chin. J. Chem., 2022, 40, 681–686 CrossRef CAS
;
(d) Z. Zuo and A. Studer, Org. Lett., 2022, 24, 949–954 CrossRef CAS PubMed
; Selected examples for alkynyl-sulfones:;
(e) K. Jana, A. Bhunia and A. Studer, Chem, 2020, 6, 512–522 CrossRef CAS
;
(f) Z. Xiong, F. Zhang, Y. Yu, Z. Tan and G. Zhu, Org. Lett., 2020, 22, 4088–4092 CrossRef CAS PubMed
;
(g) L. Capaldo and D. Ravelli, Org. Lett., 2021, 23, 2243–2247 CrossRef CAS PubMed
.
- Lower yield observed with alkynyl-sulfones:
(a) S. Zhou, T. Song, H. Chen, Z. Liu, H. Shen and C. Li, Org. Lett., 2017, 19, 698–701 CrossRef CAS PubMed
;
(b) H. Jiang, Y. He, Y. Cheng and S. Yu, Org. Lett., 2017, 19, 1240–1243 CrossRef CAS PubMed
;
(c) W. Jin, M. Wu, Z. Xiong and G. Zhu, Chem. Commun., 2018, 54, 7924–7927 RSC
;
(d) J.-B. Han, H. H. San, A. Guo, L. Wang and X.-Y. Tang, Adv. Synth. Catal., 2021, 363, 2366–2370 CrossRef CAS
; Reaction with ethynylbenziodoxolone not working with benzylic radicals:;
(e) S. P. Morcillo, E. M. Dauncey, J. H. Kim, J. J. Douglas, N. S. Sheikh and D. Leonori, Angew. Chem., Int. Ed., 2018, 57, 12945–12949 CrossRef CAS PubMed
;
(f) X. Yang and G. C. Tsui, Org. Lett., 2019, 21, 8625–8629 CrossRef CAS PubMed
.
-
(a) F. Chen and A. S. K. Hashmi, Org. Lett., 2016, 18, 2880–2882 CrossRef CAS PubMed
;
(b) X. Li, S. Li, S. Sun, F. Yang, W. Zhu, Y. Zhu, Y. Wu and Y. Wu, Adv. Synth. Catal., 2016, 358, 1699–1704 CrossRef CAS
;
(c) T. V. T. Nguyen, M. D. Wodrich and J. Waser, Chem. Sci., 2022, 13, 12831–12839 RSC
.
- For alkynyl-sulfone:
(a) M. Ociepa, J. Turkowska and D. Gryko, ACS Catal., 2018, 8, 11362–11367 CrossRef CAS
; For ethynylbenziodoxolone:;
(b) R.-Y. Zhang, L.-Y. Xi, L. Shi, X.-Z. Zhang, S.-Y. Chen and X.-Q. Yu, Org. Lett., 2016, 18, 4024–4027 CrossRef CAS PubMed
;
(c) Y. Li, R. Lu, S. Sun and L. Liu, Org. Lett., 2018, 20, 6836–6839 CrossRef CAS PubMed
;
(d) X. Liu, R. Liu, J. Dai, X. Cheng and G. Li, Org. Lett., 2018, 20, 6906–6909 CrossRef CAS PubMed
;
(e) Y. Pan, K. Jia, Y. Chen and Y. Chen, Beilstein J. Org. Chem., 2018, 14, 1215–1221 CrossRef CAS PubMed
.
-
(a) R. J. Wiles and G. A. Molander, Isr. J. Chem., 2020, 60, 281–293 CrossRef CAS PubMed
;
(b) S. Sharma, J. Singh and A. Sharma, Adv. Synth. Catal., 2021, 363, 3146–3169 CrossRef CAS
;
(c) H. Yao, W. Hu and W. Zhang, Molecules, 2021, 26, 105 CrossRef CAS PubMed
;
(d) S. Kumar Nanda, Adv. Synth. Catal., 2023, 365, 834–853 CrossRef CAS
.
- P. Xiong, H. Long, J. Song, Y. Wang, J.-F. Li and H.-C. Xu, J. Am. Chem. Soc., 2018, 140, 16387–16391 CrossRef CAS PubMed
.
-
(a) M. J. Cabrera-Afonso, A. Sookezian, S. O. Badir, M. El Khatib and G. A. Molander, Chem. Sci., 2021, 12, 9189–9195 RSC
;
(b) A. Sookezian and G. A. Molander, Org. Lett., 2023, 25, 1014–1019 CrossRef CAS PubMed
.
- S. Alazet, F. Le Vaillant, S. Nicolai, T. Courant and J. Waser, Chem. –Eur. J., 2017, 23, 9501–9504 CrossRef CAS PubMed
.
- S. Alazet, J. Preindl, R. Simonet-Davin, S. Nicolai, A. Nanchen, T. Meyer and J. Waser, J. Org. Chem., 2018, 83, 12334–12356 CrossRef CAS PubMed
.
-
(a) V. V. Zhdankin, C. J. Kuehl, A. P. Krasutsky, M. S. Formaneck and J. T. Bolz, Tetrahedron Lett., 1994, 35, 9677–9680 CrossRef CAS
;
(b) V. V. Zhdankin, A. P. Krasutsky, C. J. Kuehl, A. J. Simonsen, J. K. Woodward, B. Mismash and J. T. Bolz, J. Am. Chem. Soc., 1996, 118, 5192–5197 CrossRef CAS
.
- A. Tlili, F. Toulgoat and T. Billard, Angew. Chem., Int. Ed., 2016, 55, 11726–11735 CrossRef CAS PubMed
.
-
(a) V. Bhardwaj, D. Gumber, V. Abbot, S. Dhiman and P. Sharma, RSC Adv., 2015, 5, 15233–15266 RSC
;
(b) S. Ahmad, O. Alam, M. J. Naim, M. Shaquiquzzaman, M. M. Alam and M. Iqbal, Eur. J. Med. Chem., 2018, 157, 527–561 CrossRef CAS PubMed
;
(c) G. Li Petri, V. Spanò, R. Spatola, R. Holl, M. V. Raimondi, P. Barraja and A. Montalbano, Eur. J. Med. Chem., 2020, 208, 112783 CrossRef CAS PubMed
.
- Y. Wu, D. Kim and T. S. Teets, Synlett, 2022, 33, 1154–1179 CrossRef CAS
.
- For the cyclic voltammetry of Ts-ABZ see ESI† (Fig. S3).
- Approximate value based on the redox potential of the corresponding radical without the azide: D. D. M. Wayner, D. J. McPhee and D. Griller, J. Am. Chem. Soc., 1988, 110, 132–137 CrossRef CAS
.
-
(a) V. V. Bardin, N. Yu. Adonin and H.-J. Frohn, J. Fluorine Chem., 2007, 128, 699–702 CrossRef CAS
;
(b) A. S. Vieira, P. F. Fiorante, T. L. S. Hough, F. P. Ferreira, D. S. Lüdtke and H. A. Stefani, Org. Lett., 2008, 10, 5215–5218 CrossRef CAS PubMed
;
(c) C.-V. T. Vo, T. A. Mitchell and J. W. Bode, J. Am. Chem. Soc., 2011, 133, 14082–14089 CrossRef CAS PubMed
;
(d) R. William, S. Wang, A. Mallick and X.-W. Liu, Org. Lett., 2016, 18, 4458–4461 CrossRef CAS PubMed
;
(e) K. Miyamoto, M. Saito, S. Tsuji, T. Takagi, M. Shiro, M. Uchiyama and M. Ochiai, J. Am. Chem. Soc., 2021, 143, 9327–9331 CrossRef CAS PubMed
.
- Deposition numbers 2264031 (for 3i) and 2243553 (for 6) contain the ESI crystallographic data for this paper. These data are provided free of charge by the joint Cambridge Crystallographic Data Centre and Fachinformationszentrum Karlsruhe Access Structures service.
- In addition, following works are cited in the ESI†:
(a) V. Smyrnov, B. Muriel and J. Waser, Org. Lett., 2021, 23, 5435–5439 CrossRef CAS PubMed
;
(b) X.-G. Yang, F.-H. Du, J.-J. Li and C. Zhang, Chem. –Eur. J., 2022, 28, e202200272 CAS
;
(c) R. J. Maza, E. Davenport, N. Miralles, J. J. Carbó and E. Fernández, Org. Lett., 2019, 21, 2251–2255 CrossRef CAS
;
(d) Y. Zhang, B. Yu, B. Gao, T. Zhang and H. Huang, Org. Lett., 2019, 21, 535–539 CrossRef CAS PubMed
;
(e) M. Su, X. Huang, C. Lei and J. Jin, Org. Lett., 2022, 24, 354–358 CrossRef CAS PubMed
;
(f) M.-J. Zhou, L. Zhang, G. Liu, C. Xu and Z. Huang, J. Am. Chem. Soc., 2021, 143, 16470–16485 CrossRef CAS
;
(g) F. C. Demidoff, F. P. de Souza and C. D. Netto, Synthesis, 2017, 49, 5217–5223 CrossRef CAS
;
(h) H. Seo, A. Liu and T. F. Jamison, J. Am. Chem. Soc., 2017, 139, 13969–13972 CrossRef CAS PubMed
;
(i) Y. Yamamoto, Y. Yamada, H. Sajiki and Y. Sawama, Bull. Chem. Soc. Jpn., 2020, 93, 1419–1423 CrossRef CAS
;
(j) X.-S. Liang, R.-D. Li, W. Sun, Z. Liu and X.-C. Wang, ACS Catal., 2022, 12, 9153–9158 CrossRef CAS
;
(k) F. Yang, K. Rauch, K. Kettelhoit and L. Ackermann, Angew. Chem., Int. Ed., 2014, 53, 11285–11288 CrossRef CAS
;
(l) R. A. Oliveira, R. O. Silva, G. A. Molander and P. H. Menezes, Magn. Reson. Chem., 2009, 47, 873–878 CrossRef CAS PubMed
;
(m) D. A. Mundal, K. E. Lutz and R. J. Thomson, J. Am. Chem. Soc., 2012, 134, 5782–5785 CrossRef CAS PubMed
;
(n) J. Borrel and J. Waser, Org. Lett., 2022, 24, 142–146 CrossRef CAS PubMed
;
(o) G. A. Molander, B. W. Katona and F. Machrouhi, J. Org. Chem., 2002, 67, 8416–8423 CrossRef CAS PubMed
;
(p) P. B. Brady and E. M. Carreira, Org. Lett., 2015, 17, 3350–3353 CrossRef CAS PubMed
;
(q) J. H. Song, P. Choi, S. E. Lee, K. H. Jeong, T. Kim, K. S. Kang, Y. S. Choi and J. Ham, Eur. J. Org. Chem., 2013, 2013, 6249–6253 CrossRef CAS
;
(r) S. Jansone-Popova and J. A. May, J. Am. Chem. Soc., 2012, 134, 17877–17880 CrossRef CAS PubMed
;
(s) Y. Thummala, G. V. Karunakar and V. R. Doddi, Adv. Synth. Catal., 2019, 361, 611–616 CrossRef CAS
;
(t) J.-F. Wang, X. Meng, C.-H. Zhang, C.-M. Yu and B. Mao, Org. Lett., 2020, 22, 7427–7432 CrossRef CAS PubMed
;
(u) A. Dasgupta, C. Thiehoff, P. D. Newman, T. Wirth and R. L. Melen, Org. Biomol. Chem., 2021, 19, 4852–4865 RSC
;
(v) T. A. Mitchell and J. W. Bode, J. Am. Chem. Soc., 2009, 131, 18057–18059 CrossRef CAS PubMed
;
(w) S. Roscales, V. Ortega and A. G. Csákÿ, J. Org. Chem., 2018, 83, 11425–11436 CrossRef CAS PubMed
;
(x) V. V. Pavlishchuk and A. W. Addison, Inorg. Chim. Acta, 2000, 298, 97–102 CrossRef CAS
.
Footnote |
† Electronic supplementary information (ESI) available: Experimental procedures, characterization data and scan of NMR spectra. ESI references are given in note 33. Details for accessing crystallographic data is given in note 31. ESI references are given in note 32. For accessing raw data, please see the data availability statement. CCDC 2264031 and 2243553. For ESI and crystallographic data in CIF or other electronic format see DOI: https://doi.org/10.1039/d3sc03309k |
|
This journal is © The Royal Society of Chemistry 2023 |
Click here to see how this site uses Cookies. View our privacy policy here.