DOI:
10.1039/D0QM00972E
(Research Article)
Mater. Chem. Front., 2021,
5, 2409-2415
Dinuclear anthracene-containing alkynylplatinum(II) terpyridine complexes with photo-modulated self-assembly behaviors†
Received
23rd November 2020
, Accepted 12th January 2021
First published on 25th January 2021
Abstract
A new series of dinuclear anthracene-containing alkynylplatinum(II) terpyridine complexes exhibited intriguing photo-modulated self-assembly behaviors in solutions. Facilitated by Pt⋯Pt, π–π stacking and hydrophobic interactions, supramolecular assemblies have been formed in their DMSO solutions, featuring interesting spectroscopic responses accompanied by drastic color changes upon varying temperature, and distinct supramolecular nanostructures. Interestingly, these complexes can undergo photooxygenation upon UV excitation, which has been shown to alter their self-assembly behaviors, as revealed by the corresponding electron microscopic and DLS studies before and after photoirradiation.
Introduction
In light of the rich and tunable spectroscopic properties as well as the pronounced tendency to form supramolecular assemblies,1–14 alkynylplatinum(II) polypyridine complexes have drawn enormous attention.1–14 In particular, the formation of different supramolecular assemblies has been known to rely on the interplay of various non-covalent interactions such as hydrogen bonding, hydrophobic and π–π stacking interactions, but the utilization of metal–metal interactions to facilitate the supramolecular aggregation is still less extensively explored.15–22 Apart from the utilization of Pt⋯Pt interactions to construct supramolecular aggregates, the modulation of Pt⋯Pt interactions by external stimuli such as temperature,23,24 solvent,10,25 pH26 and the addition of polyelectrolytes27 accompanied with intriguing spectroscopic and morphological responses has aroused tremendous interests. However, there have been limited reports on the use of light to modulate the Pt⋯Pt interactions and to alter the self-assembly behaviors of platinum(II) complexes.28 Hence, the modulation of Pt⋯Pt interactions and self-assembly behaviors of the platinum(II) complexes with the incorporation of photoresponsive moieties would be worth exploring.
As a geometrically planar molecule with large π-surface, anthracene possesses interesting and tunable photophysical and photochemical properties, and has been widely utilized as a building block for the construction of functional materials with potential applications in optoelectronics and supramolecular assembly materials.29–37 In addition to the π-conjugated surface for facilitation of self-assembly, anthracenes could undergo photoinduced oxygenation reaction to form the corresponding endoperoxides,38,39 inducing obvious structural changes which could alter their spectroscopic properties and render them as chemosensors40–43 or other photoresponsive materials.44–47 However, the studies of their supramolecular assembly properties before and after photoirradiation have been mainly confined to the organic systems.36,37 Despite reports on anthracene-containing platinum(II) complexes being known,28,33,35,48,49 they are mainly confined to those of platinum(II) phosphine complexes.28,33,35,48,49 As alkynylplatinum(II) terpyridine complexes have been found to show rich spectroscopic properties and strong tendency to self-assemble through Pt⋯Pt and π–π stacking interactions owing to their square-planar structures, it is envisaged that by taking advantage of the directional non-covalent Pt⋯Pt interaction and through the incorporation of anthracene moiety into the alkynylplatinum(II) terpyridine complexes, rich photophysical properties and interesting self-assembly behaviors could be explored through changes in their photophysical properties and supramolecular assembly before and after photoirradiation.
Herein, we report a new series of dinuclear anthracene-containing alkynylplatinum(II) terpyridine complexes, their synthesis, characterization, and photophysical, photochemical and self-assembly properties. It is anticipated that with the assistance of various kinds of non-covalent interactions such as π–π stacking, hydrophobic and particularly Pt⋯Pt interactions, the complexes would exhibit intriguing self-assembly behaviors in solutions that could lead to distinct spectroscopic responses and formation of sophisticated nanostructures. Moreover, it is envisaged that the photooxygenation of anthracene moiety could alter the self-assembly behaviors of these complexes, leading to changes in the morphologies of their photoactivation-induced aggregates. It is envisioned that this work could provide important insights into the judicious molecular design and future development of photo-modulated supramolecular assembly architectures.
Results and discussion
Synthesis and characterization
Complexes 1–3 (Scheme 1) were synthesized by the reaction of their corresponding chloroplatinum(II) terpyridine precursors with 9,10-diethynylanthracene according to modifications of procedures for the synthesis of dinuclear alkynylplatinum(II) terpyridine derivatives previously reported by Yam and coworkers.24 The final products have been obtained as dark green solids after recrystallization. All the complexes have been well-characterized by 1H NMR spectroscopy, high-resolution electrospray ionization-mass spectrometry (HR-ESI-MS) and elemental analysis.
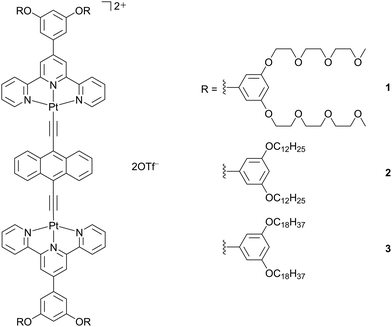 |
| Scheme 1 Molecular structures of dinuclear anthracene-containing alkynylplatinum(II) terpyridine complexes 1–3. | |
Photophysical studies
Dissolution of complexes 1–3 in dimethylsulfoxide (DMSO) gives green solutions. Their photophysical data are summarized in Table S1 (ESI†). The DMSO solutions of these complexes are found to exhibit self-assembly at room temperature even for dilute samples, probably owing to the relatively strong intermolecular noncovalent interactions such as Pt⋯Pt, π–π stacking and hydrophobic interactions under the present solvation condition, which will be discussed later. Therefore the molar extinction coefficients of the monomeric complexes in DMSO have been investigated at 368 K. Their UV–vis absorption spectra show intense absorption bands at ca. 395–475 nm and moderately intense absorption bands at ca. 495–625 nm (Fig. 1). The high-energy absorption bands of these complexes are assigned as intraligand (IL) [π → π*] transitions of the substituted terpyridine pincer ligands and anthracene-containing alkynyl ligand, while the low-energy absorption bands are assigned as a mixture of [dπ(Pt) → π*(tpy)] metal-to-ligand charge transfer (MLCT) and [π(alkynyl) → π*(tpy)] ligand-to-ligand charge transfer (LLCT) transitions. There is no significant difference in their MLCT/LLCT energies. This may be due to the similar electronic properties of various alkoxy chains that would have insignificant effect on altering the energy of the π* orbital of the terpyridine. These dinuclear platinum(II) complexes with several long alkoxy chains or oligoether units as substituents on the terpyridine ligand are found to be non-emissive in their degassed DMSO solutions. Such non-emissive behaviors can be ascribed to the possible photoinduced electron transfer due to the presence of electron-rich alkoxy or oligoether groups, similar to those observed in other related systems.26 Moreover, the floppiness of these long alkoxy chains and oligoether units could result in efficient non-radiative decay to quench the emission.
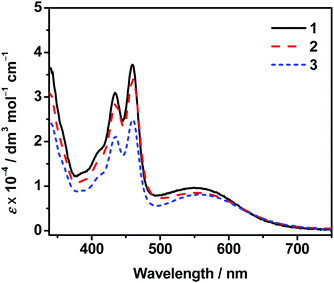 |
| Fig. 1 Electronic absorption spectra of complexes 1–3 in DMSO solutions at 368 K. | |
Self-assembly studies
The self-assembly properties of these newly synthesized dinuclear anthracene-containing alkynylplatinum(II) terpyridine complexes 1–3 have been investigated in DMSO solutions upon increasing concentrations. Complexes 1 and 2 are found to exhibit color changes from yellowish-green to green upon increasing the concentration from ∼10−6 to 10−4 M in DMSO solutions, together with the growth of low-energy absorption tails at ca. 650 nm (Fig. 2 and Fig. S1, ESI†). Owing to the limited solubility in DMSO solution, the concentration of these complexes cannot achieve higher than 1.2 × 10−4 M. It is found that the absorbance at ca. 650 nm shows a non-linear relationship with concentration, indicating deviations from Beer's Law. This reveals that ground-state aggregation occurs in the concentrated DMSO solutions of these complexes. The low-energy absorption tails are assigned as metal–metal-to-ligand charge-transfer (MMLCT) transitions due to the formation of intermolecular Pt⋯Pt, π–π stacking and hydrophobic interactions. Similar results have also been observed for complex 3 upon increasing the concentration from ∼10−7 to 10−5 M in DMSO solution (Fig. S2, ESI†). The absorbance at 730 nm is found to slightly deviate from Beer's Law, indicating the possible formation of intermolecular Pt⋯Pt interactions. However, the poorer solubility of complex 3 with longer alkoxy chains in polar DMSO solution limits the investigation of its UV-vis absorption spectroscopic properties at concentrations higher than 4.3 × 10−5 M. Although the deviations from Beer's Law are not obvious for complex 3, its DMSO solution at the concentration range of 10−5 M is found to be temperature-responsive, exhibiting drastic color changes from yellow to green upon cooling. In addition, the clear DMSO solution of complex 3 is also shown to have scattering signals in the dynamic light scattering (DLS) measurements. These temperature-dependent spectroscopic changes as well as the DLS data further support the self-assembly of complex 3 in concentrated DMSO solutions.
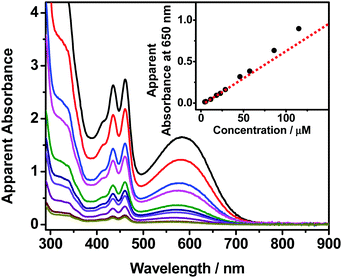 |
| Fig. 2 Concentration-dependent UV–vis absorption spectra of complex 1 in DMSO solution in the concentration range of 4.59 × 10−6 to 1.15 × 10−4 M. Inset: A plot of apparent absorbance against concentration, monitored at 650 nm. The apparent absorbance values were obtained by correcting to 1 cm path length equivalence. | |
In order to further investigate the self-assembly behaviors of the dinuclear platinum(II) complexes 1–3, their DMSO solutions with similar concentration of 10−5 M have been subject to temperature-dependent studies by cooling the sample solutions from 368 K to 293 K with a slow cooling rate of 0.5 K min−1. Upon cooling, the color of the solutions changes from yellow to green gradually (Fig. 3a), along with a growth in their MMLCT absorption bands at ca. 600 nm (Fig. 3b, Fig. S3a and S4a, ESI†), which is attributed to the formation of intermolecular Pt⋯Pt and π–π stacking interactions. Clear isosbestic points at ca. 410 nm and 475 nm have been observed, indicating a clean equilibrium between the monomer and its self-assembled aggregate species at each temperature. In addition, the plots of normalized degree of aggregation against temperature show sigmodal cooling curves, suggesting the involvement of isodesmic mechanisms in their self-assembly processes. Thus, the temperature-dependent isodesmic model has been employed to fit the variable-temperature UV-vis absorption data, and the results further confirm the isodesmic growth mechanisms for their aggregation processes. Furthermore, the equilibrium constants for their self-assembly processes can be determined from the van’t Hoff plots and the corresponding thermodynamic parameters have been obtained (Fig. 3c and Fig. S3b, S4b, ESI†). It is found that the enthalpy changes of these complexes determined from the isodesmic model show good agreement with those determined by the van’t Hoff plots. The thermodynamic parameters of complexes 1–3 have been obtained and are summarized in Table S2 (ESI†).
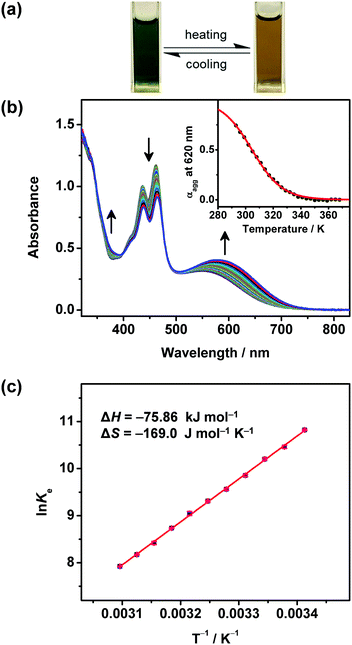 |
| Fig. 3 (a) Photographs showing the color changes of complex 2 in DMSO solution upon heating or cooling. (b) UV-Vis absorption spectral traces of complex 2 in DMSO solution upon cooling from 368 to 293 K at a rate of 0.5 K min−1. Inset: A plot of the degree of aggregation at 620 nm as a function of temperature with the curve fitted to the temperature-dependent isodesmic model. (c) Van’t Hoff plot of the equilibrium constant Ke at various temperatures with the corresponding thermodynamic parameters. | |
Interestingly, the stability of the self-assembled aggregates in DMSO solutions is found to be enhanced upon increasing the hydrophobicity of this series of complexes. By changing the substituted hydrophilic chains on the terpyridine ligand to hydrophobic chains with similar chain length, the enthalpy release of complex 2 (ΔH = −77.72 kJ mol−1) is comparable to but slightly greater than that of complex 1 (ΔH = −57.28 kJ mol−1). Moreover, upon increasing the alkoxy chain length from –OC12H25 (2) to –OC18H37 (3), the enthalpy release of complex 3 (ΔH = −101.2 kJ mol−1) is greater than that of complex 2 (ΔH = −77.72 kJ mol−1). These results suggest that, in addition to the intermolecular Pt⋯Pt and π–π stacking interactions, stronger hydrophobic interaction provided by the longer alkoxy chains can facilitate the formation of more stable aggregates. The negative ΔG values determined by the van’t Hoff plots are indicative of spontaneous self-assembly processes of these complexes. In addition, these self-assembly processes are enthalpically driven rather than entropically driven, which have been revealed by the negative values of entropy changes. Therefore, various kinds of intermolecular non-covalent interactions including Pt⋯Pt, π–π stacking and hydrophobic interactions play important roles in the spontaneous self-assembly behaviors of this series of complexes. In addition, such ground-state aggregation is further supported by the corresponding variable-temperature 1H NMR studies, in which the aromatic proton signals are downfield-shifted and sharpened upon increasing temperature (Fig. S5, ESI†).
In view of the self-assembly behaviors suggested by the concentration- and temperature-dependent UV-vis absorption studies, transmission electron microscopy (TEM) and scanning electron microscopy (SEM) have been employed to provide further insights into the self-assembly morphologies of complexes 1–3 formed in DMSO solutions at high concentrations. Only the prepared sample from complex 3 with the longest substituted alkoxy chains (–OC18H37) among these complexes exhibit well-defined morphology under the studied conditions. This can be rationalized by the stronger hydrophobic interaction in addition to the intermolecular Pt⋯Pt and π–π stacking interactions, which may facilitate the formation of larger and more stable aggregates. Interestingly, twisted fiber-like structures with ca. 500–1000 nm in length and 100–120 nm in width have been observed from the TEM images for complex 3 (Fig. 4a and b). In addition, selected-area electron diffraction (SAED) has been performed on these twisted fibrous structures. The result shows two pairs of diffraction arcs with the same d-spacing of ca. 3.53 Å along the long axes of the two individual nanofibers that are twisted together (Fig. 4c and d), suggesting the involvement of Pt⋯Pt and π–π stacking interactions along the long axes of the twisted nanofibers. Moreover, very similar twisted nanofibers with similar sizes have also been observed from the SEM image of complex 3 (Fig. 4e), further supporting the formation of these nanoaggregates facilitated by various intermolecular noncovalent interactions.
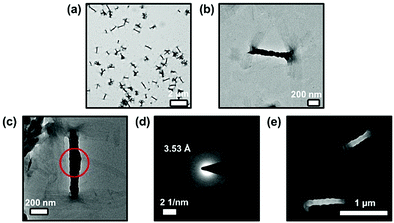 |
| Fig. 4 TEM images of complex 3 prepared from a DMSO solution with (a) lower magnification and (b) higher magnification. (c) A TEM image of complex 3 prepared from a DMSO solution with a red circle indicating where SAED was performed and (d) the corresponding SAED pattern. (e) An SEM image of complex 3 prepared from a DMSO solution. | |
Furthermore, DLS experiment has been conducted for the DMSO solution of complex 3 at room temperature. Because the DMSO solution of this complex is found to absorb the laser light of the DLS instrument at 633 nm which may interfere with the measurements, a solution of relatively lower concentration (<10−5 M) with absorbance of lower than 0.1 at this wavelength has been used to minimize such interference. The hydrodynamic diameter of the aggregates is found to be ca. 860 nm (Fig. S6, ESI†), which is in line with the TEM and SEM results. In addition, in view of the drastic changes in the temperature-dependent UV-vis absorption spectra, DLS experiment for a DMSO solution of complex 3 has been performed at a high temperature of 363 K (Fig. S6, ESI†). The hydrodynamic diameter of the aggregates at 363 K (ca. 210 nm) is found to be much smaller than that at room temperature, indicating partial deaggregation of these self-assembled aggregates upon heating. This result is in good agreement with the temperature-dependent UV-vis absorption studies.
Photochemical studies
Upon UV excitation, color changes from green to yellow have been observed for the DMSO solutions of complexes 1 and 2 (Fig. 5). It is found that the absorption bands at ca. 405–475 nm and 570 nm decrease while the high-energy absorption bands at ca. 330 nm slightly increase, with clear isosbestic points at ca. 392 nm (Fig. 5). Molecular ion clusters of [M + 2O]2+ have been observed in the HR-ESI-mass spectra of the solutions after photoirradiation (Fig. S7, ESI†), which are found to have a good agreement with the corresponding simulate isotopic patterns, indicating the formation of the corresponding endoperoxides. Interestingly, compared with complexes 1 and 2, the DMSO solution of complex 3 with the longest alkoxy chains shows similar UV-vis absorption spectral changes but to a lesser extent upon photoirradiation (Fig. S8, ESI†), exhibiting less obvious color changes. This could be due to the supramolecular aggregation induced by the stronger intermolecular Pt⋯Pt, π–π stacking and hydrophobic interactions, which would reduce the exposure of the anthracene groups to dissolved oxygen in the solution and make the molecules more difficult to interact with the oxygen.
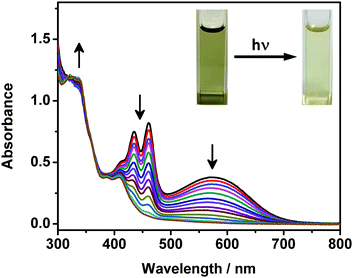 |
| Fig. 5 UV-Vis absorption spectral changes of complex 1 in DMSO solution upon photoirradiation at 365 nm at 298 K. Inset: Photographs showing the color changes of complex 1 in DMSO solution upon UV excitation. | |
More interestingly, such photooxygenation of the anthracene core unit may interrupt the π-conjugation of the originally planar anthracene surface (Fig. 6) and thus might further change the molecular packing of the complex, resulting in the formation of different morphologies. In light of the well-defined supramolecular aggregates suggested by the TEM, SEM and DLS studies of complex 3 with the longest alkoxy chain length, electron microscopy was employed to study the morphologies formed in its DMSO solution after photoirradiation. It is noteworthy that the supramolecular architecture has been found to change after photooxygenation, showing some irregular aggregates rather than well-defined twisted nanofibers in the TEM image (Fig. S9a, ESI†). Compared with the twisted fibrous structure formed from the DMSO solution of complex 3, these irregular aggregates are found to be smaller, with the size of ca. 500–600 nm. In addition, very similar morphologies with similar sizes have also been observed from the SEM image of complex 3 after photoirradiation (Fig. S9b, ESI†), further revealing the architecture of these nanoaggregates. Such drastic morphological changes might be attributed to the changes in the molecular structure upon photooxygenation which may greatly affect the molecular packing of the complex.
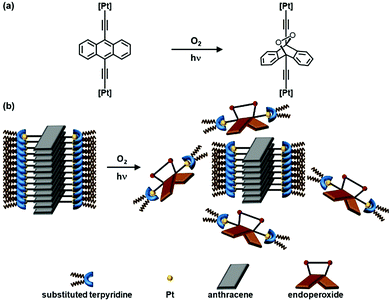 |
| Fig. 6 (a) Photooxygenation reaction of complexes 1–3 upon photoirradiation and (b) the corresponding cartoon showing the schematic drawing of proposed conformational changes upon photooxygenation at the molecular level. | |
Apart from the electron microscopic studies, DLS has also been performed, showing a hydrodynamic diameter of ca. 780 nm for these irregular aggregates (Table S3, ESI†), which is found to be slightly smaller than that of the sample before photoirradiation (ca. 860 nm). The DLS results are found to be in line with the TEM and SEM results. The size of the aggregates from the DLS study is slightly larger than that found in the TEM and SEM images, which is probably due to the shrinkage of the structures by loss of solvent during the drying process for TEM and SEM measurements.19
Conclusions
To conclude, a new series of dinuclear anthracene-containing alkynylplatinum(II) terpyridine complexes with photo-modulated self-assembly behaviors has been designed and successfully synthesized. Facilitated by various kinds of intermolecular non-covalent interactions such as π–π stacking and hydrophobic interactions as well as directional Pt⋯Pt interactions, supramolecular assemblies have been formed in concentrated DMSO solutions of these dinuclear platinum(II) complexes, showing intriguing temperature-responsive spectroscopic features with drastic color changes. Moreover, well-defined supramolecular architectures have been formed, as revealed by the electron microscopic and DLS studies. In addition to the intriguing self-assembly properties, this series of complexes can undergo photooxygenation, as revealed by their UV-vis absorption spectral changes and HR-ESI-MS analyses. More interestingly, such photooxygenation has been shown to alter the self-assembly behaviors of the complex with the longest alkoxy chains, which has been revealed by its TEM and SEM images as well as DLS studies before and after photoirradiation. By taking advantages of the directional non-covalent Pt⋯Pt interactions, the changes in the photophysical properties and self-assembly behaviors of these anthracene-containing alkynylplatinum(II) terpyridine complexes upon photoirradiation could be observed. This work is believed to provide important insights into the judicious design of platinum(II) complexes with photo-modulated self-assembly behaviors.
Conflicts of interest
There are no conflicts to declare.
Acknowledgements
V. W.-W. Y. acknowledges UGC funding administered by The University of Hong Kong for supporting the electrospray ionization quadrupole time-of-flight mass spectrometry facilities under the Support for Interdisciplinary Research in Chemical Science and the support from the Strategically Oriented Research Theme (SORT) on Functional Materials for Molecular Electronics. This work was supported by the Research Grants Council (RGC) General Research Fund (GRF) of the Hong Kong Special Administrative Region, P. R. China (HKU 17309220). S. F. acknowledges the receipt of a postgraduate studentship from The University of Hong Kong. Dr Nathan Man-Wai Wu and Dr Zhen Chen are gratefully acknowledged for their fruitful discussions on this project. We thank Mr Frankie Y.-F. Chan at the Electron Microscope Unit of The University of Hong Kong for his helpful technical assistance.
References
- W. R. Mason and H. B. Gray, Electronic structures of square-planar complexes, J. Am. Chem. Soc., 1968, 90, 5721–5729 CrossRef CAS.
- J. W. Schindler, R. C. Fukuda and A. W. Adamson, Photophysics of aqueous Pt(CN)42−, J. Am. Chem. Soc., 1982, 104, 3596–3600 CrossRef CAS.
- V. M. Miskowski and V. H. Houlding, Electronic spectra and photophysics of platinum(II) complexes with α-diimine ligands. Solid-state effects. 1. Monomers and ligand π dimers, Inorg. Chem., 1989, 28, 1529–1533 CrossRef CAS.
- V. H. Houlding and V. M. Miskowski, The effect of linear chain structure on the electronic structure of Pt(II) diimine complexes, Coord. Chem. Rev., 1991, 111, 145–152 CrossRef CAS.
- V. M. Miskowski and V. H. Houlding, Electronic spectra and photophysics of platinum(II) complexes with α-diimine ligands. Solid-state effects. 2. Metal–metal interaction in double salts and linear chains, Inorg. Chem., 1991, 30, 4446–4452 CrossRef CAS.
- H.-K. Yip, L.-K. Cheng, K.-K. Cheung and C.-M. Che, Luminescent platinum(II) complexes. Electronic spectroscopy of platinum(II) complexes of 2,2′:6′2′′-terpyridine (terpy) and p-substituted phenylterpyridines and crystal structure of [Pt(terpy)CI][CF3SO3], J. Chem. Soc., Dalton Trans., 1993, 2933–2938 RSC.
- J. A. Bailey, M. G. Hill, R. E. Marsh, V. M. Miskowski, W. P. Schaefer and H. B. Gray, Electronic spectroscopy of chloro(terpyridine)platinum(II), Inorg. Chem., 1995, 34, 4591–4599 CrossRef CAS.
- W. B. Connick, R. E. Marsh, W. P. Schaefer and H. B. Gray, Linear-chain structures of platinum(II) diimine complexes, Inorg. Chem., 1997, 36, 913–922 CrossRef CAS.
- V. W.-W. Yam, R. P.-L. Tang, K. M.-C. Wong and K.-K. Cheung, Synthesis, luminescence, electrochemistry, and ion-binding studies of platinum(II) terpyridyl acetylide complexes, Organometallics, 2001, 20, 4476–4482 CrossRef CAS.
- V. W.-W. Yam, K. M.-C. Wong and N. Zhu, Solvent-induced aggregation through metal⋯metal/π⋯π interactions: large solvatochromism of luminescent organoplatinum(II) terpyridyl complexes, J. Am. Chem. Soc., 2002, 124, 6506–6507 CrossRef CAS.
- S. Chakraborty, T. J. Wadas, H. Hester, R. Schmehl and R. Eisenberg, Platinum chromophore-based systems for photoinduced charge separation: a molecular design approach for artificial photosynthesis, Inorg. Chem., 2005, 44, 6865–6878 CrossRef CAS.
- G. S. M. Tong, Y.-C. Law, S. C. F. Kui, N. Zhu, K. H. Leung, D. L. Phillips and C.-M. Che, Ligand-to-ligand charge-transfer transitions of platinum(II) complexes with arylacetylide ligands with different chain lengths: spectroscopic characterization, effect of molecular conformations, and density functional theory calculations, Chem. – Eur. J., 2010, 16, 6540–6554 CrossRef CAS.
- K. M.-C. Wong and V. W.-W. Yam, Self-assembly of luminescent alkynylplatinum(II) terpyridyl complexes: modulation of photophysical properties through aggregation behavior, Acc. Chem. Res., 2011, 44, 424–434 CrossRef CAS.
- V. W.-W. Yam, V. K.-M. Au and S. Y.-L. Leung, Light-emitting self-assembled materials based on d8 and d10 transition metal complexes, Chem. Rev., 2015, 115, 7589–7728 CrossRef CAS.
- W. Lu, S. S.-Y. Chui, K.-M. Ng and C.-M. Che, A Submicrometer wire-to-wheel metamorphism of hybrid tridentate cyclometalated platinum(II) complexes, Angew. Chem., Int. Ed., 2008, 47, 4568–4572 CrossRef CAS.
- M.-Y. Yuen, V. A. L. Roy, W. Lu, S. C. F. Kui, G. S. M. Tong, M.-H. So, S. S.-Y. Chui, M. Muccini, J. Q. Ning, S. J. Xu and C.-M. Che, Semiconducting and electroluminescent nanowires self-assembled from organoplatinum(II) complexes, Angew. Chem., Int. Ed., 2008, 47, 9895–9899 CrossRef CAS.
- C. Po, A. Y.-Y. Tam, K. M.-C. Wong and V. W.-W. Yam, Supramolecular self-assembly of amphiphilic anionic platinum(II) complexes: a correlation between spectroscopic and morphological properties, J. Am. Chem. Soc., 2011, 133, 12136–12143 CrossRef CAS.
- C. Po, A. Y.-Y. Tam and V. W.-W. Yam, Tuning of spectroscopic properties via variation of the alkyl chain length: a systematic study of molecular structural changes on self-assembly of amphiphilic sulfonate-pendant platinum(II) bzimpy complexes in aqueous medium, Chem. Sci., 2014, 5, 2688–2695 RSC.
- H.-L. Au-Yeung, S. Y.-L. Leung, A. Y.-Y. Tam and V. W.-W. Yam, Transformable nanostructures of platinum-containing organosilane hybrids: non-covalent self-assembly of polyhedral oligomeric silsesquioxanes assisted by Pt···Pt and π–π stacking interactions of alkynylplatinum(II) terpyridine moieties, J. Am. Chem. Soc., 2014, 136, 17910–17913 CrossRef CAS.
- A. Aliprandi, M. Mauro and L. De Cola, Controlling and imaging biomimetic self-assembly, Nat. Chem., 2015, 8, 10–15 CrossRef.
- S. Y.-L. Leung, K. M.-C. Wong and V. W.-W. Yam, Self-assembly of alkynylplatinum(II) terpyridine amphiphiles into nanostructures via steric control and metal–metal interactions, Proc. Natl. Acad. Sci. U. S. A., 2016, 113, 2845–2850 CrossRef CAS.
- S. K.-M. Leung, A. K.-W. Chan, S. Y.-L. Leung, M.-Y. Leung and V. W.-W. Yam, Supramolecular assembly of bent dinuclear amphiphilic alkynylplatinum(II) terpyridine complexes: diverse nanostructures through subtle tuning of the mode of molecular stacking, Chem. Sci., 2020, 11, 499–507 RSC.
- V. W.-W. Yam, K. H.-Y. Chan, K. M.-C. Wong and B. W.-K. Chu, Luminescent dinuclear platinum(II) terpyridine complexes with a flexible bridge and “sticky ends”, Angew. Chem., Int. Ed., 2006, 45, 6169–6173 CrossRef CAS.
- K. H.-Y. Chan, H.-S. Chow, K. M.-C. Wong, M. C.-L. Yeung and V. W.-W. Yam, Towards thermochromic and thermoresponsive near-infrared (NIR) luminescent molecular materials through the modulation of inter- and/or intramolecular Pt⋯Pt and π–π interactions, Chem. Sci., 2010, 1, 477–482 RSC.
- H. L.-K. Fu, S. Y.-L. Leung and V. W.-W. Yam, A rational molecular design of triazine-containing alkynylplatinum(II) terpyridine complexes and the formation of helical ribbons via Pt⋯Pt, π–π stacking and hydrophobic–hydrophobic interactions, Chem. Commun., 2017, 53, 11349–11352 RSC.
- K. M.-C. Wong, W.-S. Tang, X.-X. Lu, N. Zhu and V. W.-W. Yam, Functionalized platinum(II) terpyridyl alkynyl complexes as colorimetric and luminescence pH sensors, Inorg. Chem., 2005, 44, 1492–1498 CrossRef CAS.
- C. Yu, K. M.-C. Wong, K. H.-Y. Chan and V. W.-W. Yam, Polymer-induced self-assembly of alkynylplatinum(II) terpyridyl complexes by metal⋯metal/π⋯π interactions, Angew. Chem., Int. Ed., 2005, 44, 791–794 CrossRef CAS.
- Z. Gao, Y. Han and F. Wang, Cooperative supramolecular polymers with anthracene–endoperoxide photo-switching for fluorescent anti-counterfeiting, Nat. Commun., 2018, 9, 3977–3985 CrossRef.
- J. E. Anthony, Functionalized acenes and heteroacenes for organic electronics, Chem. Rev., 2006, 106, 5028–5048 CrossRef CAS.
- J. Huang, J.-H. Su and H. Tian, The development of anthracene derivatives for organic light-emitting diodes, J. Mater. Chem., 2012, 22, 10977–10989 RSC.
- M. Zhu and C. Yang, Blue fluorescent emitters: design tactics and applications in organic light-emitting diodes, Chem. Soc. Rev., 2013, 42, 4963–4976 RSC.
- M. Yoshizawa and J. K. Klosterman, Molecular architectures of multi-anthracene assemblies, Chem. Soc. Rev., 2014, 43, 1885–1898 RSC.
- C. J. Kuehl, Y. K. Kryschenko, U. Radhakrishnan, S. R. Seidel, S. D. Huang and P. J. Stang, Self-assembly of nanoscopic coordination cages of D3h symmetry, Proc. Natl. Acad. Sci. U. S. A., 2002, 99, 4932–4936 CrossRef CAS.
- A. Petitjean, R. G. Khoury, N. Kyritsakas and J.-M. Lehn, Dynamic devices. Shape switching and substrate binding in ion-controlled nanomechanical molecular tweezers, J. Am. Chem. Soc., 2004, 126, 6637–6647 CrossRef CAS.
- Q.-H. Yuan, L.-J. Wan, H. Jude and P. J. Stang, Self-organization of a self-assembled supramolecular rectangle, square, and three-dimensional cage on Au(111) surfaces, J. Am. Chem. Soc., 2005, 127, 16279–16286 CrossRef CAS.
- K. Kondo, A. Suzuki, M. Akita and M. Yoshizawa, Micelle-like molecular capsules with anthracene shells as photoactive hosts, Angew. Chem., Int. Ed., 2013, 52, 2308–2312 CrossRef CAS.
- A. Suzuki, K. Kondo, M. Akita and M. Yoshizawa, Atroposelective self-assembly of a molecular capsule from amphiphilic anthracene trimers, Angew. Chem., Int. Ed., 2013, 52, 8120–8123 CrossRef CAS.
- C. S. Foote, Photosensitized oxygenations and the role of singlet oxygen, Acc. Chem. Res., 1968, 1, 104–110 CrossRef CAS.
- J.-M. Aubry, C. Pierlot, J. Rigaudy and R. Schmidt, Reversible binding of oxygen to aromatic compounds, Acc. Chem. Res., 2003, 36, 668–675 CrossRef CAS.
- K. Tanaka, T. Miura, N. Umezawa, Y. Urano, K. Kikuchi, T. Higuchi and T. Nagano, Rational design of fluorescein-based fluorescence probes. Mechanism-based design of a maximum fluorescence probe for singlet oxygen, J. Am. Chem. Soc., 2001, 123, 2530–2536 CrossRef CAS.
- X. Li, G. Zhang, H. Ma, D. Zhang, J. Li and D. Zhu, 4,5-Dimethylthio-4′-[2-(9-anthryloxy)ethylthio]tetrathiafulvalene, a highly selective and sensitive chemiluminescence probe for singlet oxygen, J. Am. Chem. Soc., 2004, 126, 11543–11548 CrossRef CAS.
- B. Song, G. Wang, M. Tan and J. Yuan, A europium(III) complex as an efficient singlet oxygen luminescence probe, J. Am. Chem. Soc., 2006, 128, 13442–13450 CrossRef CAS.
- S. Kim, T. Tachikawa, M. Fujitsuka and T. Majima, Far-red fluorescence probe for monitoring singlet oxygen during photodynamic therapy, J. Am. Chem. Soc., 2014, 136, 11707–11715 CrossRef CAS.
- W. Fudickar, A. Fery and T. Linker, Reversible light and air-driven lithography by singlet oxygen, J. Am. Chem. Soc., 2005, 127, 9386–9387 CrossRef CAS.
- W. Fudickar and T. Linker, Imaging by sensitized oxygenations of photochromic anthracene films: examination of effects that improve performance and reversibility, Chem. – Eur. J., 2006, 12, 9276–9283 CrossRef CAS.
- W. Fudickar and T. Linker, Photoimaging with singlet oxygen at the solid–air interface, Langmuir, 2009, 25, 9797–9803 CrossRef CAS.
- W. Fudickar and T. Linker, Novel anthracene materials for applications in lithography and reversible photoswitching by light and air, Langmuir, 2010, 26, 4421–4428 CrossRef CAS.
- J. Hu, R. Lin, J. H. K. Yip, K.-Y. Wong, D.-L. Ma and J. J. Vittal, Synthesis and electronic spectroscopy of luminescent cyclometalated platinum–anthracenyl complexes, Organometallics, 2007, 26, 6533–6543 CrossRef CAS.
- J. Hu, H. Xu, M.-H. Nguyen and J. H. K. Yip, Photooxidation of a platinum-anthracene pincer complex: formation and structures of PtII-anthrone and -ketal complexes, Inorg. Chem., 2009, 48, 9684–9692 CrossRef CAS.
Footnote |
† Electronic supplementary information (ESI) available: Experimental details, photophysical measurements and instrumentation, UV-vis absorption spectra, TEM and SEM images, DLS data and HR-ESI mass spectra. See DOI: 10.1039/d0qm00972e |
|
This journal is © the Partner Organisations 2021 |
Click here to see how this site uses Cookies. View our privacy policy here.