DOI:
10.1039/D3TB00747B
(Paper)
J. Mater. Chem. B, 2023,
11, 4523-4528
A tunable fluorescent probe for superoxide anion detection during inflammation caused by Treponema pallidum†
Received
5th April 2023
, Accepted 24th April 2023
First published on 25th April 2023
Abstract
Syphilis, caused by Treponema pallidum (T. pallidum), is associated with the oxidative stress due to its inflammation-like symptom, and detecting the reactive oxygen species (ROS) is crucial for monitoring the infectious process. Herein, we design and synthesize a perylene-based tunable fluorescent probe, PerqdOH, which can detect endogenous O2˙− during T. pallidum infection. The fluorescence peak shifted from 540 nm to 750 nm with increasing O2˙− levels. Besides, both decreased green fluorescence and enhanced red fluorescence could be observed simultaneously during the in vitro infection, providing the real-time monitoring of intracellular O2˙− caused by T. pallidum. Furthermore, the probe exhibited a remarkable signal in the treponemal lesions on the back of a rabbit model. Taken together, our synthesized PerqdOH holds great potential for application in clarifying the infectious process caused by T. pallidum in real time.
1. Introduction
Syphilis, caused by Treponema pallidum subspecies pallidum (T. pallidum), remains a significant global threat worldwide owing to its potential to cause multiple organ damage and increase the risk of other diseases.1–3 Similar to inflammation, the accumulation of inflammatory cells such as lymphocytes, neutrophils and macrophages could be found in the lesion site throughout the course of syphilis,4,5 particularly in the painless chancre of the primary stage and the obvious papule of the secondary stage.6,7 Indeed, T. pallidum infection initially destructs the tissue and triggers an inflammatory response,8–10 which subsequently activate the production of reactive oxygen species (ROS) to promote the phagocytosis and clearance by macrophages.8,11–16 The overload ROS plays a central role in the inflammatory disease due to its contribution to trigger oxidative stress and induce inflammation,17–19 and superoxide radical (O2˙−), a primary ROS,20 has been usually used as a biomarker of inflammation diagnosis.21,22 The dysregulated O2˙− can lead to oxidative stress,23 causing harm to biomolecules like DNA, proteins and lipids and inducing apoptosis.6,24,25 Moreover, the relationship between upregulated ROS and the developed syphilis has been studied,26,27 suggesting O2˙− may link to the syphilis-related inflammation. Thus, monitoring the level of O2˙− in the living cells and tissues is crucial to determine the extent of oxidative stress, estimate the biological inflammation process,28,29 and further used for alleviating the inflammation.
To date, fluorescent probes have made significant progress in monitoring oxidative stress.30–33 Specifically, several reversible fluorescent sensors based on organic molecules have been reported to dynamically monitor the redox couples, including O2˙− and GSH,34–36 which greatly expanded the detection toolkit for the oxidation state. However, a probe for monitoring syphilis-caused oxidative stress is still lacking. Herein, we develop a perylene-based fluorescent probe (PerqdOH) to detect endogenous O2˙− and determine the inflammatory progress caused by T. pallidum. In our design, pyrocatechol was oxidized to carbonyl in the presence of O2˙−, causing a red-shift in the fluorescence of our synthesized probe, which could clearly monitor intracellular O2˙− and help ascertain the O2˙− level during treponemal infection in vitro. Furthermore, the probe can monitor the inflammatory process of the treponemal lesions in vivo in real time, which makes it potentially useful to monitor oxidative stress during inflammation caused by T. pallidum.
2. Experimental section
2.1 Materials
Dopamine, phorbol-12-myristate-13-acetate (PMA), sodium peroxide, and glutathione (GSH) were purchased from Aladdin (China). Cell counting kit-8 (CCK8) was obtained from Dojindo (Japan). MitoTracker red and Hoechst 33342 were obtained from Invitrogen (USA). Acetic acid, DCM, DMF and MeOH were purchased from Guangzhou chemical Reagent Factory (China). Ultrapure water used in this study was obtained from the Milli-Q water purification system from Millipore.
2.2 Synthesis and characterization of the probe
The reactant (0.394 g, 1.0 mmol) and dopamine (0.306 g, 2.0 mmol) were dissolved in 25 mL acetic acid mixed with NMP, and the mixture was heated at 80 °C for 15 h under a nitrogen atmosphere. After the reaction was completed, the solvent was removed to give the crude product. Then, the crude product was dried and subjected to purification by flash chromatography (DCM: MeOH; 20
:
1) to give probe PerqdOH as a red solid (0.413 g, 65.3%). Then, 1HNMR, and HR-MS were utilized to characterize the structure of PerqdOH; UV-vis spectrophotometer and fluorescence spectrophotometer were separately used to determine the optical properties.
2.3 Detection of O2˙−
Probe PerqdOH was prepared in DMF, and O2˙− was derived from potassium peroxide. The concentration of PerqdOH added as a substrate in the detection was 10 μM, and the concentration of O2˙− was 100 μM, while the concentration of GSH used to reduce O2˙− was 500 μM. The spectrum detection was performed 1 min after adding O2˙− or GSH to the substrate using a fluorescence spectrophotometer, and absorption was measured using a UV-vis spectrophotometer similarly.
2.4 Intracellular fluorescence imaging
The exogenous O2˙− was first applied to investigate the imaging capability of PerqdOH. The cell lines used in the experiment were purchased from American Type Culture Collection (ATCC, Manassas, Virginia, USA). B16 cells or RAW 264.7 cells were cultured in 6-well plates at a concentration of 2 × 105 cells per well overnight, and then PerqdOH was co-cultured with the cells for 4 hours, followed by the addition of O2˙− for another 24 hours. A laser confocal microscope (Nikon A1, Japan) was used for imaging after the cells were stained with Hoechst 33342 for 0.5 h.
As reported, PMA was commonly used to stimulate the generation of endogenous O2˙−.37 The cells were first seeded at a density of 2 × 105 cells mL−1 and PerqdOH was pre-cultured with the cells for 4 h, and 0.5 μg mL−1 of PMA was stained at various time points (0 h to 36 h) to investigate the time-dependent manner; for dose-dependent manner, various concentrations (0–1.5 μg mL−1) of PMA were added into the seeded cells for 24 hours. The plates were washed three times with PBS and a laser confocal microscope was used to trace intracellular fluorescence.
2.5 Fluorescence detection after T. pallidum infection in vitro and in vivo
For in vitro detection, RAW 264.7 cells (2 × 105 cells mL−1, 2 mL) were first seeded in the plates, and different MOIs (from 1
:
10 to 1
:
1000) of T. pallidum were co-cultured with RAW 264.7 cells followed by the pre-incubated PerqdOH. The fluorescence image was then taken using a laser confocal microscope.
The experimental procedures were approved by the Institute of Pharmacology and Toxicology Academy of Military Medical Sciences PLA, Peop. Rep. China Ethical Committee on Animal Care and Use, and all efforts were made to minimize animal suffering and reduce the number of animals used for the experiments. For in vitro detection, after the rabbits were anaesthetized using isoflurane, 107T. pallidum were first seeded on the back of the rabbit to build a standard treponemal model by forming lesions, and PerqdOH was injected into the lesions; then the images were taken under an excitation of 680 nm and an emission of 750 nm, respectively.
3. Results
3.1 Rational design, synthesis and characterization
The synthesis route and oxidation mechanism of PerqdOH are provided in Scheme 1. With the oxidation of O2˙−, the pyrocatechol groups of PerqdOH changed to carbonyl groups, which led to the transform of the fluorescence behaviour. Their structures were confirmed by 1HNMR and HR-MS. We first determined the mechanism using 1HNMR (Fig. S1 and S2, ESI†), and variations of chemical shift between 6.0 and 9.0 ppm were in accordance with the oxidation mechanism, which clearly notified that pyrocatechol had been transformed to benzoquinone. Furthermore, the results of 13CNMR (Fig. S3, ESI†) and MS (Fig. S4 and S5, ESI†) verified the conversion again.
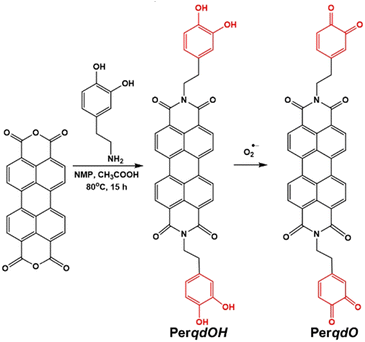 |
| Scheme 1 The synthesis route of P–OH and its interaction with O2˙−. | |
In order to confirm the recognition toward O2˙−, UV-Vis and fluorescence measurements had been carried out. As shown in Fig. 1A, upon the addition of O2˙−, the absorption band at 490 nm decreased notably and a new absorbance band centred at 700 nm, while the absorbance band at 490 nm partly resumed after the introduction of GSH. Hence, due to the maximal absorption at 490 nm and 700 nm, they were selected as the excitation wavelengths for further study. As shown in Fig. S6 (ESI†) and Fig. 1B, with the addition of O2˙−, the fluorescence intensity of PerqdOH at 540 nm (Ex = 490 nm) decreased, and the intensity at 750 nm (Ex = 700 nm) increased meanwhile. Besides, the introduction of GSH caused the resumed fluorescence intensity at 540 nm and diminution at 750 nm. Additionally, only PerqdO exhibited obvious fluorescence under the excitation of 700 nm and emission of 750 nm (Fig. S7, ESI†). These results suggested that probe PerqdOH exhibited tuneable fluorescence due to the conversion between pyrocatechol (PerqdOH) and benzoquinone (PerqdO).
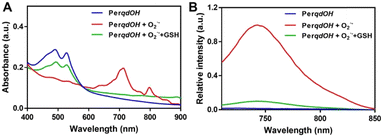 |
| Fig. 1 (A) UV-vis spectra and (B) fluorescence spectra change after adding O2˙− and GSH. | |
Subsequently, we studied the UV-vis and fluorescence of PerqdOH in the presence of various doses of O2˙− or GSH. With the addition of O2˙− (0–400 μM), the maximal absorption at 490 nm decreased and that at 700 nm increased in a dose-dependent manner (Fig. 2A), and a progressively enhanced and diminished fluorescence emission appeared at 750 nm (Fig. 2B) and 540 nm (Fig. S8, ESI†), separately. The concentration-dependent fluorescence response of probe versus O2˙− was further investigated, and the fluorescence intensity at 750 nm revealed a linear proportion (R2 = 0.9902) with the concentration of O2˙− ranging from 0 to 400 μM (Fig. S9, ESI†).
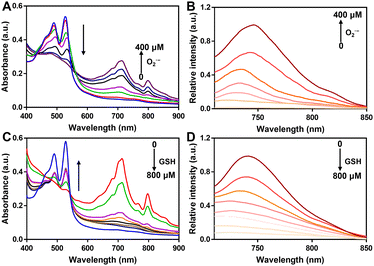 |
| Fig. 2 Optical change of the probe under oxidation and reduction. (A) UV-Vis spectra change and (B) fluorescence change of PerqdOH after adding O2˙− in a dose-dependent manner. (C) Uv-Vis spectra change and (D) fluorescence change of PerqdO after adding GSH in a dose-dependent manner. | |
What's more, as the reducing agent GSH (0–800 μM) was added to PerqdO, the maximal absorption band at 490 nm recovered and the absorption band at 700 nm reduced notably (Fig. 2C), following an enhanced emission peak at 540 nm and decreased fluorescence intensity at 750 nm (Fig. 2D and Fig. S10, ESI†). The results clearly showed that our designed probe displayed satisfactory tunable response character toward oxidation and reduction.
Owing to the high reduction activity of dopamine, it has great potential to react with many oxidants, so we evaluated the selectivity of our probe PerqdOH towards different ROS including H2O2, O2˙−, 1O2, and ClO−. Stock solutions of HOCl and H2O2 were prepared by directly diluting the commercial compounds with ultra-pure water; ˙OH was prepared by Fenton reaction between ferrous sulfate solution and hydrogen peroxide; O2˙− was delivered by dissolving potassium superoxide in DMSO; and 1O2 was obtained by irradiating Rose Bengal with laser (660 nm, 20 mW). As shown in Fig. S11A (ESI†), only the addition of O2˙− induced the increased absorption band at 700 nm, the decreased fluorescence intensity at 540 nm (Fig. S11B, ESI†) and the appearance of maximal fluorescence enhancement at 750 nm (Fig. S11C, ESI†), whereas the variations with the other analytes were negligible. Additionally, the fluorescence image, which was taken under an excitation of 680 nm and an emission of 750 nm, clearly demonstrated the high specificity of PerqdOH towards O2˙− according to the apparent fluorescence after treatment with O2˙− (Fig. S11D, ESI†).
3.2 Monitoring and imaging of O2˙−in vitro
Before carrying out the cell imaging studies of PerqdOH, cytotoxicity assays revealed that PerqdOH within 40 μM is suitable for bioimaging application (Fig. S12, ESI†). Next, we monitored the transport of probe PerqdOH in cells after staining the mitochondria (MitoTracker red) and nucleus (Hoechst 33342), and this directly showed that probe PerqdOH had crossed the cell membrane and gradually accumulated in the cells after incubation for 30 min, and then filled the cytoplasm at 60 min, which could be chosen as the time point of detection internalization time (Fig. 3). Furthermore, probe PerqdOH was co-located with mitochondria, implying that the changes of O2˙− in the mitochondria can be monitored.
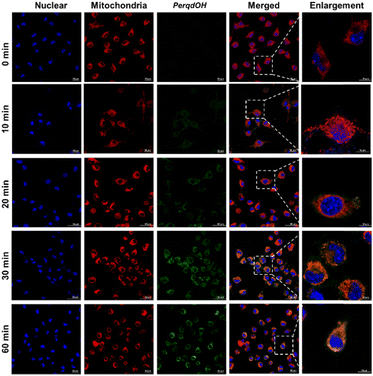 |
| Fig. 3 Intracellular trafficking of 40 μM PerqdOH in cells at different periods of time (0–60 min). Mito tracker (red) and Hoechst 33342 were used to stain mitochondria and nucleus, separately. | |
In order to demonstrate the capability for imaging endogenous O2˙− in living cells, we used several groups of cell lines for test. After 1 hour pre-incubation with PerqdOH, O2˙− was first added into the cells, and laser confocal microscopy was employed for imaging. As shown in Fig. S13 (ESI†), compared to the probe PerqdOH alone in the cells, green fluorescence (PerqdOH) was rarely noticeable in the group treated with O2˙−, while red fluorescence (PerqdO) was progressively enhanced. Then we employed the probe for endogenous O2˙− detection. For this purpose, B16 cells and RAW 264.7 cells were treated with phorbol-12-myristate-13-acetate (PMA) after co-incubating with probe PerqdOH for 1 h.
PMA is considered as an endogenous O2˙− trigger, which would induce endogenous O2˙− after incubation with cells.37,38 The increase in red fluorescent channel and decrease in green fluorescent channel were observed in a time-dependent and dose-dependent manner in PMA-treated B16 cells (Fig. 4). Besides, both fluorescence intensity in the green channel and the red channel stayed steady as the culture time elapsed continually, suggesting that the amount of produced and consumed endogenous O2˙− was balanced after 24 hour incubation with PMA. PMA-treated RAW 264.7 cells also displayed similar fluorescence behaviour to B16 cells (Fig. S14, ESI†).
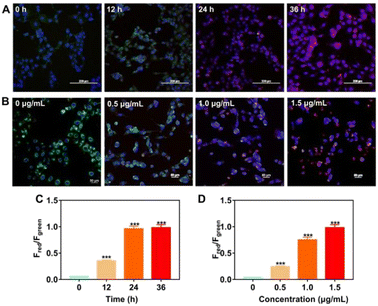 |
| Fig. 4 Monitoring endogenous O2˙− in cells. Fluorescence images of B16 cells after co-incubation with PMA in (A) a time-dependent manner (from 0 h to 36 h) and (B) a dose-dependent manner (0-1.5 μg mL−1). (C) and (D) The quantitative analysis of the relative fluorescence intensity. | |
Additionally, we determined the reversible capability of probe PerqdOH in cell lines by employing GSH as the reductant after treatment with PMA. As displayed in Fig. S15 (ESI†), the fluorescence intensity in the green channel recovered with the addition of GSH, while the red fluorescence became weaker meanwhile, illustrating that the probe could reflect the oxidative conditions via the reversible fluorescence behaviour. In a word, our designed PerqdOH has potential for the detection of endogenously generated O2˙− in cellular systems under different conditions.
3.3 Monitoring and imaging of oxidative conditions caused by T. pallidum
As reported previously, T. pallidum is eliminated by immune cells at the early stage of infection.39 Thus, we focus on the production of intracellular O2˙− during the clearance. It was first found that the exposure of synthesized PerqdOH was nearly nontoxic for T. pallidum (Fig. S16, ESI†). We then monitored the interaction between macrophages and T. pallidum in vitro using the probe. According to Fig. 5, we found the increased red fluorescence intensity as the MOI of treated T. pallidum changed from 1
:
101 to 1
:
103 after 24 hour incubation, which indicated that the endogenous O2˙− had been produced during co-culture between T. pallidum and RAW 264.7 cells.
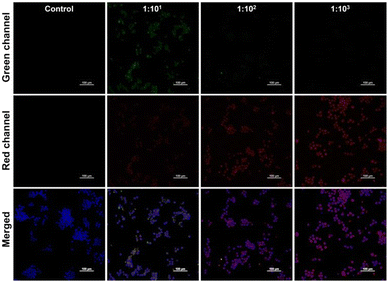 |
| Fig. 5 Fluorescence images of simulating infection by different MOIs (1 : 101, 1 : 102, 1 : 103) of T. pallidum in vitro. Scale bar: 100 μm. | |
Since probe PerqdOH could detect exogenous and endogenous O2˙−, we envisaged that the probe could have the potential to detect O2˙− under in vivo conditions. Therefore, New Zealand white rabbits were utilized as the primary syphilis model. After T. pallidum-induced lesions on the back appeared, probe PerqdOH was injected into the lesions and the adjacent skin. Obviously, as shown in Fig. 6A and B, the signal of PerqdO (Ex = 680 nm, Ex = 750 nm) in the lesions raised 8 hours after injection, and the intensity became maximal after another 4 hours, followed by a decreased signal of the probe due to the clearance by the immune system in the subsequent period. In a word, probe PerqdOH is an efficient probe for monitoring O2˙− during T. pallidum infection under in vivo conditions.
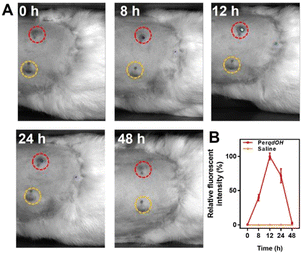 |
| Fig. 6
In vivo monitoring of endogenous O2˙− in the lesions of a primary syphilis model. (A) The images of fluorescence change in the lesions at different periods of time. (B) The relative statistics. The red circle marks the lesion injected with PerqdOH, and the orange circle marks the lesion injected with saline, while the green circle marks the adjacent area injected with PerqdOH. | |
4. Discussion
Syphilis can be regard as an inflammatory disease due to the tissue destruction character of T. pallidum through the whole process, and this process is closely related to ROS. Monitoring ROS is critical to determine the inflammatory process caused by the pathogen. O2˙− is one of the primary ROS, which could cause great damage to the cells during inflammation by causing oxidative stress. Numerous probes have been designed to detect ROS, but many of them failed to be further used owing to the lack of stability under irradiation. We thus designed a fluorescence tuneable probe (PerqdOH) which could exhibit a fluorescence shift under the stimulation of O2˙−.
We first determined the fluorescence behaviour change of the designed probe PerqdOH using UV-Vis spectrum and fluorescence spectrum. The results suggested that the maximal absorption band of PerqdOH would shift from 490 nm to 700 nm with the addition of O2˙−, following the decrease in the emission intensity at 540 nm (Ex = 490 nm) and a progressive increase in the fluorescence intensity at 750 nm (Ex = 700 nm) meanwhile, due to the conversion from pyrocatechol to benzoquinone according to 1HNMR and MS and 13CNMR. Besides, the introduction of reductant GSH led to the recovered fluorescence intensity at 540 nm. Also, the probe displayed unique selectivity towards O2˙−. Next, in vitro fluorescence behaviour was used to investigate the detection of intracellular O2˙−. With the addition of O2˙− into the cells, the fluorescence intensity in the green channel decreased and the intensity in the red channel increased, suggesting that probe PerqdOH in the cells has been oxidized to PerqdO by O2˙−. Furthermore, PMA was used to trigger endogenous O2˙−, and our designed PerqdOH responsively exhibited obvious fluorescence transform capability during the excitation of PMA, displaying the potential to monitor intracellular O2˙−. As macrophage is the first-stage of defence against T. pallidum, we simulated the immunity process of syphilis by infecting RAW 264.7 cells with T. pallidum, and monitored the intracellular O2˙− using the probe. We noticed decreasing green fluorescence intensity and increasing red fluorescence intensity with increasing number of pathogens, suggesting that T. pallidum could induce the generation of O2˙− during phagocytosis. Additionally, in vivo detection in the lesions of the primary syphilis model proved to be an efficient tool for monitoring treponemal-related O2˙− response in living systems.
5. Conclusions
In conclusion, we have rationally designed a fluorescence tuneable probe PerqdOH to enable the monitoring of intracellular O2˙−. The emission of the synthesized probe could shift from 540 nm to 750 nm with the addition of O2˙− because the pyrocatechol (PerqdOH) had been oxidized to benzoquinone (PerqdO), while the fluorescence at 540 nm recovered under the reduction of GSH, representing that fluorescence is tuneable under oxidation and reduction conditions. Besides, probe PerqdOH could responsively detect exogenous and endogenous O2˙− in cells, especially during the infection of T. pallidum in the host cells in vivo and in vitro. Taken together, our synthesized probe PerqdOH might prove to be an efficient tool for monitoring the process of inflammatory T. pallidum infection in living systems.
Author contributions
JDZ and WQL designed and performed the experiment. JLH, SG and WQL were responsible for data collation. WQL and JLH drafted the manuscript. SG, GFL, and JDZ edited and revised the manuscript draft. JDZ, GFL and YHL were responsible for providing funds and unified management of work. All authors read and approved the final manuscript.
Conflicts of interest
There are no conflicts to declare.
Acknowledgements
This research was supported by the National Key Research and Development Program (2021YFC2302200), the Natural Science Fund of Guangdong Province for Distinguished Young Scholars (2022B1515020089), the National Natural Science Foundation of China (82272248, 81972019, 82102444), Training project of National Science Foundation for Outstanding/Excellent Young Scholars of Southern Medical University (C620PF0217, 2019RC01), and Zhongnanshan Medical Foundation of Guangdong Province (ZNSA-2021012; Guang Dong Province, China).
References
- R. W. Peeling, D. Mabey, M. L. Kamb, X. S. Chen, J. D. Radolf and A. S. Benzaken, Nat. Rev. Dis. Primers, 2017, 3, 17073 CrossRef PubMed.
- J. B. Taxy and T. Cibull, Am. J. Surg. Pathol, 2020, 44, 1274–1281 CrossRef PubMed.
- Z. Li, J. Zhang, Y. Huang, J. Zhai, G. Liao, Z. Wang and C. Ning, Coord. Chem. Rev., 2022, 471, 214723 CrossRef CAS.
- S. Tuddenham, M. M. Hamill and K. G. Ghanem, JAMA, 2022, 327, 161–172 CrossRef CAS PubMed.
- L. R. Lin, W. Liu, X. Z. Zhu, Y. Y. Chen, Z. X. Gao, K. Gao, M. L. Tong, H. L. Zhang, Y. Xiao, W. D. Li, S. L. Li, H. L. Lin, L. L. Liu, Z. X. Fang, J. J. Niu, Y. Lin and T. C. Yang, BMC Immunol., 2018, 19, 28 CrossRef PubMed.
- A. Flamm, K. Parikh, Q. Xie, E. J. Kwon and D. M. Elston, J. Am. Acad. Dermatol., 2015, 73, 1025–1030 CrossRef PubMed.
- A. Flamm, V. M. Alcocer, V. Kazlouskaya, E. J. Kwon and D. Elston, J. Am. Acad. Dermatol., 2020, 82, 156–160 CrossRef PubMed.
- A. R. Cruz, L. G. Ramirez, A. V. Zuluaga, A. Pillay, C. Abreu, C. A. Valencia, C. La Vake, J. L. Cervantes, S. Dunham-Ems, R. Cartun, D. Mavilio, J. D. Radolf and J. C. Salazar, PLoS Neglected Trop. Dis., 2012, 6, e1717 CrossRef CAS PubMed.
-
J. E. Bennett, R. Dolin and M. J. Blaser, Mandell, douglas, and bennett's principles and practice of infectious diseases E-book, Elsevier Health Sciences, 2019 Search PubMed.
- S. Liu, S. Wang, Y. Wu, F. Zhao, T. Zeng, Y. Zhang, Q. Zhang and D. Gao, Sci. China: Life Sci., 2010, 53, 229–233 CrossRef CAS PubMed.
- H. J. Forman and M. Torres, Am. J. Respir. Crit. Care Med., 2002, 166, S4–S8 CrossRef PubMed.
- G. Liao, F. He, Q. Li, L. Zhong, R. Zhao, H. Che, H. Gao and B. Fang, Prog. Mater. Sci., 2020, 112, 100666 CrossRef CAS.
- G. Liao, L. Zhang, C. Li, S.-Y. Liu, B. Fang and H. Yang, Matter, 2022, 5, 3341–3374 CrossRef CAS.
- Z. Mo, X. Pan, X. Pan, L. Ye, H. Hu, Q. Xu, X. Hu, Z. Xu, J. Xiong, G. Liao and S. Yang, J. Mater. Chem. B, 2022, 10, 8760–8770 RSC.
- Z. Qin, M. Qiu, Q. Zhang, S. Yang, G. Liao, Z. Xiong and Z. Xu, J. Mater. Chem. B, 2021, 9, 8882–8896 RSC.
- L. Zhang, G. Oudeng, F. Wen and G. J. B. R. Liao, Biomater. Res., 2022, 26, 61 CrossRef CAS PubMed.
- H. Wang, K. Wan and X. Shi, Adv. Mater., 2019, 31, e1805368 CrossRef PubMed.
- Z. Li, T. Liang, S. Lv, Q. Zhuang and Z. Liu, J. Am. Chem. Soc., 2015, 137, 11179–11185 CrossRef CAS PubMed.
- J. Zheng, Y. Wu, D. Xing and T. Zhang, Nano Res., 2019, 12, 931–938 CrossRef CAS.
- K. Kobayashi, Chem. Rev., 2019, 119, 4413–4462 CrossRef CAS PubMed.
- J. Fang, T. Seki and H. Maeda, Adv. Drug Delivery Rev., 2009, 61, 290–302 CrossRef CAS PubMed.
- R. Liu, L. Zhang, Y. Chen, Z. Huang, Y. Huang and S. Zhao, Anal. Chem., 2018, 90, 4452–4460 CrossRef CAS PubMed.
- W. Lin, H. Liu, L. Chen, J. Chen, D. Zhang, Q. Cheng, F. Yang, Q. Zeng and T. Chen, Nano Today, 2021, 38, 101124 CrossRef CAS.
- A. Singh, R. Kukreti, L. Saso and S. Kukreti, Molecules, 2019, 24, 1583 CrossRef CAS PubMed.
- I. S. Harris and G. M. DeNicola, Trends Cell Biol., 2020, 30, 440–451 CrossRef CAS PubMed.
- C. Zhou, X. Zhang, W. Zhang, J. Duan and F. Zhao, J. Clin. Lab. Anal., 2019, 33, e22890 Search PubMed.
- L. R. Lin, Y. Xiao, W. Liu, Y. Y. Chen, X. Z. Zhu, Z. X. Gao, K. Gao, M. L. Tong, H. L. Zhang, S. L. Li, H. L. Lin, W. D. Li, X. M. Liang, Y. Lin, L. L. Liu and T. C. Yang, BMC Infect. Dis., 2018, 18, 101 CrossRef PubMed.
- H. Sies and D. P. Jones, Nat. Rev. Mol. Cell Biol., 2020, 21, 363–383 CrossRef CAS PubMed.
- B. Kong, T. Yang, F. Cheng, Y. Qian, C. Li, L. Zhan, Y. Li, H. Zou and C. Huang, J. Colloid Interface Sci., 2022, 611, 545–553 CrossRef CAS PubMed.
- X. Liu, Z. Yan, Y. Sun, J. Ren and X. Qu, Chem. Commun., 2017, 53, 6215–6218 RSC.
- B. Yang, Y. Chen and J. Shi, Chem. Rev., 2019, 119, 4881–4985 CrossRef CAS PubMed.
- Y. Wei, Y. Liu, Y. He and Y. Wang, J. Mater. Chem. B, 2021, 9, 908–920 RSC.
- P. Xu and G. Liao, Materials, 2018, 11, 1616 CrossRef PubMed.
- J. Zheng, Q. Zeng, R. Zhang, D. Xing and T. Zhang, J. Am. Chem. Soc., 2019, 141, 19226–19230 CrossRef CAS PubMed.
- W. Zhang, P. Li, F. Yang, X. Hu, C. Sun, W. Zhang, D. Chen and B. Tang, J. Am. Chem. Soc., 2013, 135, 14956–14959 CrossRef CAS PubMed.
- P. Gao, W. Pan, N. Li and B. Tang, Chem. Sci., 2019, 10, 6035–6071 RSC.
- Y. Wang, K. M. Dillon, Z. Li, E. W. Winckler and J. B. Matson, Angew. Chem., Int. Ed., 2020, 59, 16698–16704 CrossRef CAS PubMed.
- Y. Zhao and M. D. Pluth, Angew. Chem., Int. Ed., 2016, 55, 14638–14642 CrossRef CAS PubMed.
- S. L. Xu, Y. Lin, X. Z. Zhu, D. Liu, M. L. Tong, L. L. Liu, T. C. Yang and L. R. Lin, J. Eur. Acad. Dermatol. Venereol., 2020, 34, 2111–2119 CrossRef CAS PubMed.
|
This journal is © The Royal Society of Chemistry 2023 |
Click here to see how this site uses Cookies. View our privacy policy here.