Nanoformulations from olive pomace to enhance the efficacy of hydroxytyrosol as a natural pest control agent†
Received
20th March 2024
, Accepted 29th June 2024
First published on 16th July 2024
Abstract
Bioactive principles administered in the form of finely dispersed particles are a major innovation in plant protection. At present, the most promising formulations are those in which natural functional compounds are transported by vectors obtained from agrifood by-products, in that these systems are able to maximize both efficacy and biocompatibility. Here we present new nanovectors obtained by combining olive pomace (OP) and egg yolk phosphatidylcholine (EPC) to deliver hydroxytyrosol (HT) as a pest control agent. In this way, a valuable antioxidant found in agricultural waste can be reused to develop biopesticides according to the concept of circular economy. Specifically, nanocarriers obtained by natural lipid mixtures were loaded with HT and dispersed in water. The stable and reproducible character of these formulations was assessed by in-depth physicochemical characterization. For this purpose, empty and HT-loaded nanosystems were investigated by dynamic light scattering, zeta potential, and small-angle X-ray scattering. Combining these tools allowed reaching a comprehensive picture of the obtained particles in terms of size and structure at the submicron scale. The concentration of HT used was 2.5–5 mg mL−1, a range of values which are not attainable in the absence of encapsulating objects. The newly prepared biopesticides were subsequently tested against the Mediterranean fruit fly Ceratitis capitata (Wiedemann, 1824), belonging to Diptera, Tephritidae, and Sitophilus oryzae (Linnaeus, 1763), belonging to Coleoptera, Curculionidae. Multifactorial analysis was used to examine the relationships among different groups of variables, such as number of insect eggs and number of egg clusters. This in-depth data treatment showed increased repellency and limited oviposition in samples protected by formulations, thus opening new perspectives for applications in sustainable agriculture.
Environmental significance
Pesticides protect crops from harmful insects, but their widespread use raises environmental and health concerns. There is a growing demand for eco-friendly alternatives, such as biopesticides. These can be produced naturally by plants or obtained from plant extracts. Processing agricultural by-products is another viable route to obtain biopesticides. Olive pomace (OP), derived from the olive oil industry, is known for its phytotoxicity and non-biodegradability. It contains hydroxytyrosol (HT), a phytoalexin active against pests. HT, already used as an antimicrobial, can be developed into a biopesticide. Here, we propose to use biocompatible nanoformulations made from OP and egg yolk phosphatidylcholine to deliver HT. This sustainable method could reduce environmental impact, improve pest control, and align with global efforts toward sustainable agriculture.
|
Introduction
Pesticides are crucial for protecting crops against harmful insects, but their extensive use has raised environmental and health concern.1 For these reasons, increasingly strict regulation has been issued to limit pesticide spread,2 driving demand for eco-friendly alternatives, such as biopesticides. These include compounds commonly produced by plants as natural protection against pests, and can be obtained from plant extracts (e.g. essential oils) or agricultural by-products, which offer a cost-effective source of chemicals for potential application in environmentally safe procedures.3,4 As a prominent example, in the Mediterranean area, several organic wastes from olive oil production represent a valuable source of chemicals for promoting plant growth and agriculture.5 Indeed, the olive (Olea europaea L.) oil industry results in large amounts of mill wastewater and olive pomace (OP), generating disposal challenges. In particular OP, known for its phytotoxicity and non-biodegradability,6 contains fiber, minerals, fatty acids, and phenol derivatives.7 Such products can be reused to develop new biopesticides, thus supporting the circular economy. Here, submicron carriers were obtained with OP and other lipids from natural sources (egg yolk phospholipids, EPC). A possible drawback encountered when using complex blends is that the overall amphiphilic character may be low, resulting in limited self-aggregation in water medium and ill-defined structures. In such cases, the addition of purified lipids, e.g. EPC, can be of help.8 In-depth characterization of the newly conceived carriers provided a satisfactory picture of their structure and relevant physicochemical properties.
In their leaves and fruits, olive species contain oleuropein, which is hydrolyzed to release the phytoalexin hydroxytyrosol (HT), during a biotic attack or the ripening. This molecule is a natural polyphenol active against pathogens and herbivores that has already been used as an antimicrobial agent for applications in medicine.9–11 Olive oil, olive mill wastewater and olive leaf extracts are particularly rich in HT, thus representing a valuable source of this molecule. Encapsulating active substances in submicron carriers enhances their effectiveness through broader contact and sustained release.12 The goal of this work was to exploit olive tree-derived materials (OP) as starting molecules for building submicron particles to be loaded with HT.
The biocide activity of the present formulations was evaluated towards two insect species of agricultural interest, namely the Mediterranean fruit fly Ceratitis capitata Wiedemann, 1824 (Diptera, Tephritidae) and the rice weevil Sitophilus oryzae Linnaeus, 1763 (Coleoptera, Curculionidae). C. capitata, is also a critical pest in global agriculture due to its broad diet, severe impact on a wide range of fruits,13 and its role in facilitating further damage through the diffusion of pathogens. Females deposit eggs inside a wide range of economically important fruits, and the larvae then feed on the fruit pulp. The damage inflicted by this invasive insect significantly affects fruit production and international trade,14,15 thus making its management crucial for protecting the agricultural industry and ensuring food security. The selection of a host and the subsequent oviposition are critical behaviors for the reproductive success of insects. Insects must choose suitable environments for laying their eggs to ensure the survival of their larvae and avoid high mortality rates, which can impact population dynamics.
The rice weevil, S. oryzae, a global pest found in grain storage facilities, significantly impacts both food safety and the economy.16 Female weevils lay eggs inside cereal grains, and the larvae develop within the kernels, causing substantial damage.17 These infestations directly affect the grains and create conditions favorable for secondary infestations by mites and pathogens, further impacting stored products.18 The overall outcome is a decrease of the weight and quality of essential crops such as rice, wheat, and maize. The current methods for controlling these pests, often involving pesticides, pose environmental and health concerns.19 However, effective management of S. oryzae is essential to maintain the integrity and safety of the global food supply.
Here a new and sustainable approach is offered to the problem of pest control20–24 by exploring natural substances which were formulated into highly dispersed particles.
Experimental
Materials
Egg phosphatidylcholine (EPC) was purchased from Avanti Polar Lipids Inc. All the solvents used were from Merck (Darmstadt, Germany). Hydroxytyrosol (HT), with a MW of 154.16 g mol−1, was extracted from free-oil olive pulp at the PhytoLab, University of Florence, following the procedure described by Romani et al., 2017.25 Olive pomace (OP) powder was obtained using a sustainable process described by Romani et al., 2020.26 OP is rich in natural bioactive compounds and it has been estimated that 1 g of OP contains the same amount of polyphenols as 200 g of extra-virgin olive oil.27 The composition of OP powder polyphenols, reported in Table 1, was evaluated by HPLC-DAD-MS (Agilent Technologies, Palo Alto, CA, USA) as described in ref. 26. OP is also known as a source of dietary fiber; in recent years several studies have been carried out on the fibrous component of this innovative and sustainable product. Specific studies on OP report that the fiber yield can vary from 92.12% to 62.06%, of which from 21.68% to 3.5% are soluble dietary fiber and from 76.1% to 52.17% are insoluble dietary fiber.28–30 This evidence suggests that OP can be an effective, innovative functional product to be used in agronomic, food, cosmetic and feed sectors.
Table 1 Composition of polyphenols in olive pomace powder
Identified molecules |
Olive pomace 2022 mg g−1 |
Glycol hydroxytyrosol |
1.23 |
Hydroxytyrosol |
3.52 |
Tyrosol |
1.41 |
Oleoside |
0.90 |
Verbascoside |
2.95 |
Verbascoside derivates |
0.34 |
Oleuropein |
6.82 |
Glucoside luteolin |
0.46 |
Luteolin derivates |
0.26 |
Total |
17.87 |
Protocol for nanoformulations
EPC stock solutions (900 μL at 7.5 mg mL−1 in ethanol) were mixed with two different volumes (0.5 mL and 1 mL) of 15 mg mL−1 HT solution in acetone. The organic solvent was left to evaporate overnight under reduced pressure. The dry films were then rehydrated with 3 mL of OP at a concentration of 3.75 mg mL−1 in aqueous KOH at pH 13.5. All the samples were homogenized by five freeze–thaw cycles in liquid nitrogen and water bath at 50 °C and then sonicated by 5 cycles of 3 min (75% power level with a Bandelin Electronic Sonopuls instrument, Berlin, Germany). In loaded vectors, the starting concentration of HT was 2.5 mg mL−1 and 5 mg mL−1, respectively. The quantitative evaluation of loading rates was performed spectrophotometrically, after HT extraction with CH2Cl2 and detection of the absorption intensity at 280 nm.31
After sonication, 196–200 μL of HCl (36% v/v) were added to lower the pH to a neutral value. The acronyms of the samples tested against C. capitata and S. oryzae are listed in Table 2.8 Control is only water; HT2.5 and HT5 represent two aqueous macrodispersions (slurries) of hydroxytyrosol at concentrations of 2.5 mg mL−1 and 5 mg mL−1. EPC-OP refers to the empty aggregates and EPC-OP-HT2.5 and EPC-OP-HT5 are the nanoformulations loaded with HT.
Table 2 Experimental layout of HT and nanoformulations used in the repellence and anti-ovideponent activity assays with diluted (2.5 mg mL−1) and concentrated (5 mg mL−1) bioactive molecules
Tested sample |
EPC (mg mL−1) |
OP (mg mL−1) |
HT content (mg mL−1) |
Control = distilled water; EPC = egg phosphatidylcholine, OP = olive pomace, HT = hydroxytyrosol; the final pH of all the formulations was 6.5. |
Control (water) |
— |
— |
— |
HT2.5 |
— |
— |
2.5 |
HT5 |
— |
— |
5 |
EPC-OP |
7.5 |
3.75 |
— |
EPC-OP-HT2.5 |
7.5 |
3.75 |
2.5 |
EPC-OP-HT5 |
7.5 |
3.75 |
5 |
Nanosystem characterization
Zeta potential (ζ).
Zeta potential (ζ) measurements were carried out on a Zetasizer Pro instrument (Malvern Panalytical Co. Ltd., Malvern, UK) in DTS1070 cells, at 25 °C. The ζ values were measured in a mixed mode, using phase analysis light scattering (M3-PALS). Samples were diluted 1
:
40 with Milli-Q water. The measurement was repeated 3 times, and the surface charges are shown as the mean (mV) ± SD in Table 3.
Table 3 Surface charge of the nanoformulations
Tested sample |
ζ (mV) |
EPC-OP |
−8 ± 0.1 |
EPC-OP-HT2.5 |
−25 ± 1 |
EPC-OP-HT5 |
−30 ± 1 |
Dynamic light scattering (DLS)
The size distributions of nanoformulations were analyzed by dynamic light scattering (DLS) measurements performed on a Malvern Zetasizer Nano ZS (ZEN 1600 model, Malvern Instruments Southborough, MA) equipped with a He–Ne 633.4 mW laser with backscattering detection. DLS analyses were performed over 11 runs and in duplicate. Samples were diluted 1
:
40 with Milli-Q water before measuring to adjust turbidity.
Small-angle X-ray scattering (SAXS)
Small-angle X-ray scattering (SAXS) experiments were performed at the high brilliance beamline ID02 of the European Synchrotron Radiation Facility (ESRF), Grenoble, France. The wavelength of the incident photons was λ = 1 Å. The scattering vector (
, where θ is the scattering angle) was in the range 0.216 × 10−4 to 0.75 nm−1. Samples were diluted at 1
:
40 in Milli-Q water and placed in quartz capillaries of 1.5 mm diameter. The obtained SAXS intensity profiles were fitted using SasView 5.0.5 (https://www.sasview.org/). The different contributions were superimposed using OriginPro (https://www.originlab.com).
Insect bioassays
The effectiveness of chemical compounds, as detailed in Table 2, was evaluated for their ability to discourage insects from approaching a designated area or ovipositing.32
The bioassays used two agricultural insect pests: the fruit fly C. capitata and the rice weevil S. oryzae. Both species were reared in the Plant Pathology and Entomology Laboratory of the Department of Agriculture, Food, Environment, and Forestry of the University of Florence. The C. capitata was maintained as described by Economopoulos and Judt.33 The weevils were reared in cylindrical plastic containers (diameter 31 cm × height 33.5 cm) and maintained as described by Stefanazzi et al.23
Experiment on C. capitata
Two-choice tests were conducted using cylindrical plastic cups (diameter 6 cm × height 8 cm) covered with a transparent nylon net as an oviposition substrate divided into two sections with a 2 mm wide paper tape. One half was treated with 4 ml of the formulations from Table 2, while the other half received distilled water. Five C. capitata females were introduced into each container. The females deposited eggs in clusters by piercing the net with their ovipositors. Seven replicates were performed with randomly arranged cups to minimise positional errors.
The medflies were left undisturbed for one hour, then observed at hourly intervals for 240 minutes, recording the number of females on each side of the net. The positional aversion was assessed using the female contact (FC) rate, calculated as the ratio of females on each half of the net to the total number of females in the container.32
To evaluate oviposition activity (OA), medflies were allowed to lay eggs for 72 hours, and egg clusters (OAEC), total eggs (OAE), and the average number of eggs per cluster (E/EC) were counted at 5-hour and 72-hour intervals.
To assess the inhibitory effects of the treatments (Table 2), repellency and oviposition deterrence indices were calculated. Positional aversion was evaluated using the repellence index (RIFC), while egg-laying activity was assessed using oviposition deterrence indices for egg clusters (ODIEC) and individual eggs (ODIE).34 The indices were calculated using the formula:
Index = (treatment − control)/(treatment + control) |
In this equation, “treatment” represents the measured parameter value (FC, EC, E) in the treated area, and “control” represents the value recorded in the control area.
Experiment on S. oryzae
The repellent effect of HT and HT-loaded nanoformulations was tested on adults of S. oryzae in a multi-choice test arena with six traps (Fig. 1). Each trap contained 15 ± 0.05 g of wheat grains treated with 5 mL of the samples indicated in Table 2. Sixty unsexed adult beetles were placed in the centre of the arena, with the possibility of entering the traps freely. Each bioassay ended when at least 90% of the beetles had entered the traps or after two hours. Seven replicates were conducted, with trap arrangements randomly rotated to reduce positional bias.
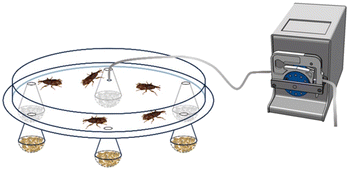 |
| Fig. 1 Schematic representation of the arena employed in the experimental setup to assess the repellent activity on S. oryzae. The arena included a white cardboard base (30 cm in diameter and 0.4 cm in thickness) equipped with six equidistant holes (0.5 cm in diameter), positioned 20 cm from the centre and connected to traps. The traps were obtained by modifying disposable 140 ml plastic flutes which ended in perforated hemispheres (4.5 cm in diameter) containing wheat kernels treated with the samples indicated in Table 2. The cardboard was covered with a transparent plastic cover (25 cm in diameter and 5 cm in height) connected in the centre to a peristaltic pump ISMATEC BVP Standard ISM 444, 240 rpm, which created an airflow from the spheres toward the arena, rotating counterclockwise. | |
Adult choice (AC) was determined by counting the insects in each trap and treatment efficacy was evaluated using a repellence index (RIAC), calculated with the same formula as in the C. capitata experiment. The index was interpreted according to Stefanazzi et al.23
In a second experiment, the effectiveness of the formulations in dissuading female S. oryzae from laying eggs was evaluated. Wheat grains (8 ± 0.05 g) of the Inalettabile variety were counted using the open-source software ImageJ Fiji distribution,35 version 1.54f, (accessed in July 2023) using the Analyze Particle command applied to binary thresholded images of the kernels samples. The seeds were uniformly treated with approximately 60 μL of the formulations (Table 2) and left to dry at 25 °C for 15 minutes in a static oven. Subsequently, the seeds were transferred into cylindrical plastic jars (diameter 4 cm × height 5.5 cm), each containing eight randomly selected S. oryzae adults of mixed sex and age. The jars were stored in the dark at 28 ± 5 °C and 35 ± 5% RH.
After 21 days,36,37 the experiment was concluded, and the insects were collected to identify females using a stereomicroscope to observe genital structures. In addition, kernels were stained38,39 with gentian violet to highlight the oviposition plugs and seeds with one or more plugs (EP) were counted. Oviposition activity (OAEP) was calculated by determining the ratio of seeds with egg caps to the total number of kernels and females in the container.
To quantify the effectiveness of the treatments in reducing egg laying, we used the oviposition deterrence index (ODIEP).40 This index was calculated as:
ODIEP = (control − treatment)/control |
In this formula, “control” represents the count of egg-plugged kernels in the control group, and “treatment” is the count in the treatment group.
Statistical analysis
Statistical analyses of the experiments were performed using R software version 4.2.2,41 accessed in August 2023. Data on female contact (FC) rate in the study of repellence in C. capitata were analyzed using chi-square goodness of fit test. The results from both untreated and treated sides of the nylon net were compared to assess the null hypothesis (H0 = 1
:
1).
Oviposition activity data were analysed using multiple factor analysis (MFA), which can comprehensively explore complex data sets and provide insight into combined effects. In natural oviposition behaviour, C. capitata is highly adaptive; females encountering unfavourable substrates may reduce egg stinging and the total number of released eggs. When competition is high, females may lay fewer egg clusters but more eggs per cluster.22 Medflies release marking pheromones that cause other females to redistribute egg laying to less favourable areas to reduce competition.42,43 To assess oviposition activity, we measured the number of egg clusters (EC), the number of eggs (E), and the average number of eggs per cluster (E/EC) in both treated and untreated areas. These parameters were evaluated at two exposure times (5 and 72 hours), during which the number of ovipositions can increase.
MFA analysis was performed using the FactoMineR and FactoExtra packages within Rstudio.44,45 Data were categorized into three groups of quantitative variables: the number of egg clusters (EC), the number of eggs (E), and the average number of eggs per cluster (E/EC). Each group included data from both the treated and untreated areas of the net, with treatments and time considered as qualitative variables.
The MFA results (detailed in the ESI†) showed that data variation was mainly due to the number of egg clusters (EC) and eggs (E) providing similar insights (internal relationship RV = 0.90). However, the eggs group better represented the observed variability (overall MFA RV = 0.75). In contrast, the average number of eggs per cluster (E/EC) had a minimal effect on the overall variability possibly due to the consistent number of eggs per cluster under different conditions. Time was the main contributor to dimension 1 (26.09%), indicating that temporal differences are a major source of variability. There was no significant correlation between time and treatment (RV = 0.00), showing that temporal dynamics are independent of the treatment effects.
Based on these results, an inferential analysis was performed considering only the total number of eggs (E) at the two time points. A chi-square goodness-of-fit test was employed, where the null hypothesis (H0) assumed a 1
:
1 egg ratio between the untreated and the treated areas.
For the S. oryzae experiment, data on the adult beetle's choice (AC) measuring the repellence activity were analyzed using a chi-square goodness-of-fit test by comparing the frequencies of individuals in the trap against the null hypothesis (H0 = 1
:
1
:
1
:
1
:
1
:
1). The data on the oviposition deterrent activity (OA) met the distribution assumptions; the means were compared using a one-way ANOVA, followed by multiple pairwise comparisons with an improved Fisher–Hayter test for better control of the familywise error rate.46 The Tietjen-Moore Test for multiple simultaneous outlier detection was employed.47
Results
Nanovector characterization
Zeta potential (ζ) and dynamic light scattering (DLS).
The surface charge of the submicron aggregates was negative in all nanoformulations prepared in this work, as revealed by the ζ values reported in Table 3. The ζ of empty vectors was rather low in absolute value, i.e. −8 mV, while it was more marked in the nanoformulations loaded with HT (−25–−30 mV). Considering that the final pH of these samples was close to neutrality, the polyphenol units were not ionized. We thus inferred that the presence of HT induced a change in the original molecular arrangement, which was reflected in a different propensity to absorb negatively charged species at the interface with the aqueous medium.
DLS measurements showed that the loaded vectors had an average size of 250–350 nm while their plain counterparts were much larger (mean diameter ≈1000 nm). This finding was explained considering the lower surface charge of empty particles, which promote growth. The polydispersity index (PDI) of HT loaded nanovectors was in the range of 0.2–0.3, while it was almost doubled in empty aggregates, indicating that the HT presence imparted structural constraints and therefore, more regular packing.
SAXS
The scattering intensity diagrams of empty and loaded nanoaggregates at neutral pH are reported in Fig. 2. All the curves showed the typical SAXS profile of liposomes,48–50 indicating that the basic structure of the nanoparticles obtained from a mixture of OP and EPC was a single bilayer. Adding HT molecules did not significantly alter this property, although an increase of correlation among bilayers was observed at 5 mg mL−1 HT concentration, probably due to the onset of oligolamellar stacking as suggested by the appearance of a small Bragg peak at q = 1.3 nm−1. The overall size of plain and loaded aggregates exceeded the detectable length scale in these SAXS diagrams (2π/qmin ≈ 50 nm) and therefore this length was not accessed by SAXS experiments.
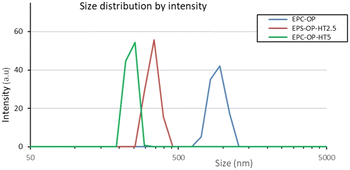 |
| Fig. 2 Size distributions of EPC-OP, EPC-OP-HT2.5 and EPC-OP-HT5 obtained from intensity weighted DLS results. | |
The enlargement in the inset of Fig. 3 shows the fitting obtained by modelling the experimental patterns as a lipid bilayer. The values used for the length and electron density of the different regions in the bilayer are listed in Table 4 and are consistent with those reported in the literature for similar systems.48–50
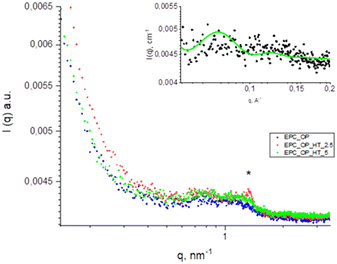 |
| Fig. 3 SAXS intensity diagrams of EPC-OP samples at neutral pH and corresponding best fit (inset) the asterisk shows the onset of a Bragg peak indicating that HT loading increases the correlation of bilayers in the liposomal vectors. | |
Table 4 Best-fit parameters of the EPC-OP SAXS curves obtained by SasView. tc tail thickness; th head thickness; ρc,e tail scattering length density; ρh,e head scattering length density; ρs,e solvent scattering length density; lc length tail; lh length head
Parameters |
Average diameter (nm) |
t
c (Å) |
67 |
t
h (Å) |
17 |
ρ
c,e (cm−2) |
7 × 10−10 |
ρ
h,e (cm−2) |
1.4 × 10−11 |
ρ
s,e (cm−2) |
9.5 × 10−10 |
Polydispersity lc (Å) |
0.1 |
Polydispersity lh (Å) |
0.1 |
Thus, from SAXS measurements we can infer that the structures of the submicron vectors studied in this work were oligolamellar liposomes. This is consistent with their composition, since the main components are phospholipids (EPC) and amphiphilic molecules (OP).
Experiment on C. capitata females
C. capitata female repellence.
In studying the aversion of C. capitata toward the systems listed in Table 2, the experimental setup revealed no positional bias (control = 39.53%, p = 0.19). As shown in Table 5, the EPC-OP-HT5 submicron particles, enriched with a higher HT content, yielded statistically significant results. The female contact rate with the treated area of the nylon net was 25.93%, lower than the control halves rate (p < 0.01). Additionally, the EPC-OP-HT5 nanoformulation achieved a repellence index (RIFC) of −0.22, a value which reflects repellence and demonstrates the effectiveness of this formulation in comparison with the other treatments (Fig. 4). EPC-OP, EPC-OP-HT2.5, and HT5 treatments had repellence index values (RIFC) close to zero (−0.05, −0.05, and −0.08, respectively), suggesting scarce repellence. On the other hand, HT2.5 treatment with an RIFC of 0.31, indicated a moderate attraction of C. capitata females in response to low concentrations of HT compared to the control bioassays (Fig. 4).
Table 5 Results of the C. capitata females contact rate (FC) on the treated and untreated halves of the nylon net expressed as percentages, and outcomes of the chi-square goodness-of-fit test comparing the two halves. For the control group, the test compares the contact rates between the left and the right halves of the net
Treatments |
n
|
FC rate (%) |
χ
2
|
df |
p
|
Control |
24 |
39.53 |
0.66 |
1.00 |
0.19 |
EPC-OP |
24 |
50.00 |
0.32 |
1.00 |
1.00 |
HT2.5 |
24 |
61.76 |
0.68 |
1.00 |
0.17 |
EPC-OP-HT2.5 |
24 |
34.48 |
0.76 |
1.00 |
0.09 |
HT5 |
24 |
40.63 |
0.59 |
1.00 |
0.29 |
EPC-OP-HT5 |
24 |
25.93 |
0.91 |
1.00 |
0.01 |
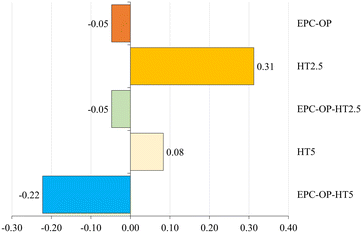 |
| Fig. 4 Results of the repellence index (RIFC) of the bioactive molecules HT and nanoformulation treatments on C. capitata females contacting the treated area of the nylon net. Index values range from −1 to +1, with positive values indicating an increase in the treated area, negative values showing a decrease, and zero means no difference. | |
Oviposition deterrence on C. capitata.
The experiment examining the oviposition activity of C. capitata in response to the compounds used in bioassays highlights the impact that these treatments have over time. Table 6 shows the analysis of the results on the percentages of eggs laid at the two time intervals of 5 and 72 hours. Fig. 5A and B report the results of the oviposition deterrence index (ODIE) at the same time intervals.
Table 6 Number of eggs laid by C. capitata on treated halves of the nylon net within experimental containers at 5 and 72 hours. Data are presented as percentages. The goodness-of-fit chi-square test was used for analysis; in the control treatment, comparisons were made between the left and right halves of the net
Time |
Treatments |
Eggs (E) (%) |
χ
2
|
p
|
5 hour |
Control |
56.6 |
3.41 |
0.06 |
EPC-OP |
10.8 |
90.92 |
<0.01 |
HT2.5 |
46.8 |
0.58 |
0.45 |
EPC-OP-HT2.5 |
0.00 |
— |
— |
HT5 |
30.4 |
26.25 |
<0.01 |
EPC-OP-HT5 |
0.00 |
— |
— |
72 hour |
Control |
48.00 |
1.66 |
0.20 |
EPC-OP |
47.8 |
1.37 |
0.24 |
HT2.5 |
53.00 |
2.82 |
0.09 |
EPC-OP-HT2.5 |
21.5 |
366.04 |
<0.001 |
HT5 |
46.5 |
3.48 |
0.06 |
EPC-OP-HT5 |
22.3 |
259.51 |
<0.001 |
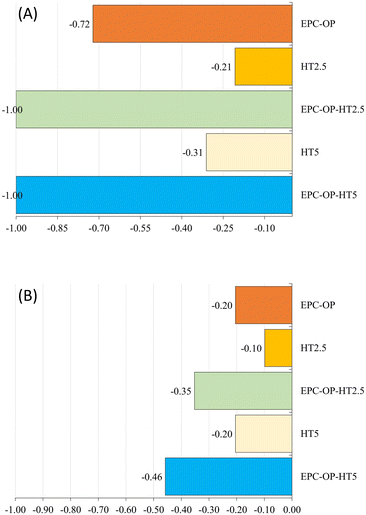 |
| Fig. 5 Results of the oviposition deterrence index (ODIE) assessing the effects of HT and nanoformulations on deterrence of C. capitata egg laying at 5 hour (A) and 72 hour (B) intervals. Index values range from −1 to +1, with positive values indicating an increase in the treated area, negative values showing a decrease, and zero means no difference. | |
The experiments of HT administration with submicron carriers, i.e. the treatment with EPC-OP-HT2.5 and EPC-OP-HT5 samples, were highly effective in deterring C. capitata from laying eggs. At the 5-hour interval, no oviposition occurred in areas treated with these systems. However, by the 72-hour mark, egg deposition rose to 21.5% and 22.3% for EPC-OP-HT2.5 and EPC-OP-HT5 (both with p-values below 0.01), respectively. This demonstrated that while the intensity of the treatments tended to decrease over time, encapsulated HT maintained a marked inhibition effect. Concerning the deterrence indices for the treatments, EPC-OP-HT2.5 and EPC-OP-HT5 showed a decrease at the 72-hour mark, yet remaining substantially high (ODIE = −0.35 for EPC-OP-HT2.5 and ODIE = −0.46 for EPC-OP-HT5). Therefore, despite a slight reduction, the efficacy of these treatments in deterring egg laying was still significant.
In the case of treatment with HT2.5 without nanoformulations, C. capitata deposited 46.8% of eggs in the treated area within the first 5 hours (p = 0.45). By the 72-hour interval, this percentage increased to 53% (p = 0.09), indicating that the egg-laying behavior was not significantly affected during these two time intervals. In this treatment, the oviposition deterrence index for eggs (ODIE) was −0.21 at the 5-hour mark, indicating a minimal deterrence effect, which further decreased to −0.10 at 72 hours.
In the experiment using the higher concentration of hydroxytyrosol (HT5) without submicron vectors, the administration of this bioactive molecule was initially effective by itself since it reduced egg deposition, with only 30.4% of eggs observed at the 5-hour mark (p < 0.01). However, the percentage of egg deposition in the treated area increased to 46.5% (p = 0.06) by the 72-hour point, suggesting a reduction in the effectiveness of this treatment over time. Additionally, in the HT5 treatment, the oviposition deterrence index (ODIE) was −0.31 after 5 hours, demonstrating strong deterrence at the higher HT concentration. By the 72-hour point this ODIE value decreased to −0.20, reflecting a reduction in the deterrent effect.
In the treatment with empty EPC-OP formulation, a significant reduction in the oviposition activity of C. capitata was observed initially. The egg deposition in the area treated with EPC-OP was only 10.81% within the first 5 hours (p < 0.01), demonstrating a decrease in oviposition compared to the untreated area. However, this effect was reduced over time, and by the 72-hour mark, egg deposition increased again to 47.78% (p = 0.24), equaling the levels in the area simply treated with distilled water. In line with this, the oviposition deterrence index (ODIE) started high at −0.72, indicating strong deterrence initially, but decreased to −0.20 by 72 hours, showing a decline in the long-term effectiveness of the treatment.
Experiment on S. oryzae adults
S. oryzae adult repellence.
The repellent activity of HT-loaded nanoformulations on adults of S. oryzae resulted in significant differences in trap choices by the weevils with respect to control treatments (chi-square = 25.34, p < 0.001). Traps with distilled water for the control and EPC-OP nanocarrier attracted 22.2% and 20.3% of adults, respectively. Conversely, traps where grains were exposed to high concentrations of HT alone or loaded into submicron vectors (HT5, EPC-OP-HT2.5, and EPC-OP-HT5) showed significantly lower adult entry rates, between 13.1% and 13.3% (Table 7). However, traps with grains treated with lower concentrations of hydroxytyrosol showed adult choices similar to those for the control and EPC-OP treatments (AC = 17.95%).
Table 7 Result of the S. oryzae trap selection using chi-square goodness-of-fit test across treatments (expected proportions 1
:
1
:
1
:
1
:
1
:
1)
Treatments |
S. oryzae trap selection % |
Chi |
p
|
Total number of S. oryzae: 518; replicates per treatment, n = 9; p = p-value of overall significance. Pairwise comparisons were performed using the Bonferroni correction. Different letters indicate significant differences between groups. |
Control |
22.20 a |
25.34 |
<0.001 |
EPC-OP |
20.27 a |
|
|
HT2.5 |
17.95 a,b |
|
|
EPC-OP-HT2.5 |
13.13 b |
|
|
HT5 |
13.13 b |
|
|
EPC-OP-HT5 |
13.32 b |
|
|
Analyzing the repellence index (RIAC) as established by Stefanazzi et al.,23 treatments had distinct impacts on the adults of S. oryzae (Fig. 6). The carrier EPC-OP, when used alone, showed no repellent effect (RIAC = 0.00), indicating its limited effectiveness as a stand-alone agent. The treatment with HT2.5 exhibited minor repellency (RIAC = −0.10), while HT5 resulted in a more noticeable repellent effect (RIAC = −0.18), although this level was still relatively low. It is important to note that EPC-OP, despite lacking inherent repellent properties, enhances the effectiveness of HT against adult of S. oryzae. The EPC-OP-HT2.5 formulation produced a more significant repellent effect (RIAC = −0.19) on these adults, comparable to the effect of HT used alone at higher concentration. In summary, the combination of EPC-OP with HT5 exhibited the most relevant effect, achieving an index of −0.28 against adult S. oryzae.
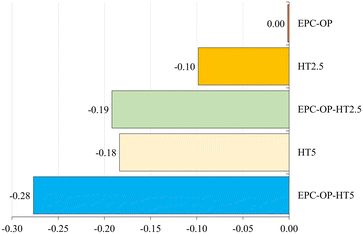 |
| Fig. 6 Results of the repellence index (RIAC) of the bioactive molecules hydroxytyrosol and nanoformulations treatments on S. oryzae in trap choices by the weevils. Index values range from −1 to +1, with positive values indicating an increase in the treated area, negative values showing a decrease, and zero means no difference. | |
Oviposition deterrence on S. oryzae
The ANOVA results, assessing the impact of nanoformulation on the oviposition activity (OAEP) of S. oryzae, indicated a highly significant difference (F = 4.580, p < 0.003), as reported in Fig. 7. Treatments involving EPC-OP-HT2.5 (0.06 ± 0.01) and EPC-OP-HT5 (0.05 ± 0.01) led to fewer wheat seeds with egg plugs compared to other treatments, demonstrating greater effectiveness than HT-only treatments HT2.5 (0.09 ± 0.01) and HT5 (0.09 ± 0.01). However, these experiments still showed significantly fewer infested kernels than the control (0.10 ± 0.01) and the EPC-OP treatment (0.10 ± 0.01). The oviposition deterrence index (ODIEP) corroborated the ANOVA results, with EPC-OP-HT2.5 (ODIEP = −0.15) and EPC-OP-HT5 (ODIEP = −0.26) being the only treatments with negative values (Fig. 8). These results imply a moderate deterrence of S. oryzae oviposition, yet indicating fewer infested kernels in the treated group than in the control. In contrast, the other treatments, such as the submicron carrier EPC-OP and the HT bioactive molecule HT2.5, and HT5, exhibited a near-zero ODIEP (0.09, 0.00, and 0.05, respectively).
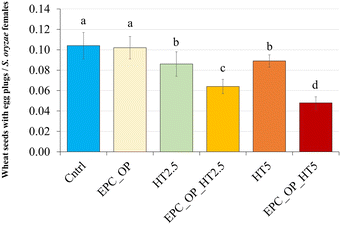 |
| Fig. 7 Results of ANOVA of the oviposition activity (OAEP) of S. oryzae. The graph displays the average number of wheat seeds with egg plugs relative to the total number of grains, and the number of female weevils. Statistically significant differences between treatments are denoted by distinct letters (p < 0.05). Error bars = standard errors. | |
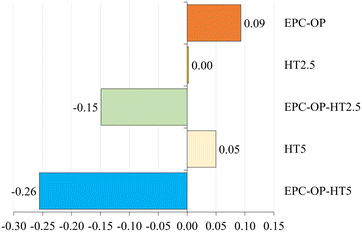 |
| Fig. 8 Results of the oviposition deterrence index (RIAC) of the bioactive molecules HT and nanoformulation treatments on S. oryzae females. The index was calculated using the number of wheat seeds with egg plugs relative to the total number of grains, and the number of female weevils. Index values from −1.00 to −0.10 mean repellence, between −0.10 and +0.10 indicate neutrality, while the range +0.10 to +1.00 suggests attraction. | |
Discussion
DLS experiments showed that the protocol devised here to obtain vectors for HT delivery from OP and EPC mixtures allows the production of nanoformulations containing particles with size in the appropriate range for drug transport, in that the diameter of HT-loaded aggregates was mostly in the range of 200–300 nm.51,52 These particles were also homogeneous in size, the measured PDI being 0.2–0.3. On the contrary, plain nanoformulations had a size of approximately 1000 nm with broad size distribution (PDI ≈0.6). This meant that HT association strongly influenced the structural feature of EPC-OP vectors. Considering that the HT molecular shape is markedly different from that of most lipids in the starting mixture, we think that host HT molecules can lower the particle size by increasing its local curvature. Finer structural details could be obtained by SAXS, showing that the aggregates in solution were substantially isolated unilamellar liposomes with polydispersity in the same range as measured by DLS. A small degree of lamellarity (2–3 concentric shells) was detected for HT loaded aggregates, confirming that the HT molecules are located near the bilayer surface where they can increase the interaction among bilayers. The negative surface charge characterizing all the nanoformulations is probably the main factor which keeps the nanoaggregates dispersed in solution and should be regarded as a favorable property for applications. The observed physicochemical features were mirrored in the performance of EPC-OP lipid particles as delivery agent for the bioactive molecules already present in OP and for the additional HT that we had loaded by coformulation. Indeed, these submicron-assemblies were able to extend the efficacy of HT over time (e.g. 5 h and 72 h) demonstrating its controlled release. In particular, these systems were able to inhibit the contact of C. capitata females with treated surfaces and discourage their egg-laying. The efficacy of HT delivery by submicron carriers was also observed in trials against S. oryzae, although with less pronounced strength with respect to the Mediterranean fruit fly. The adverse response of C. capitata was most pronounced for the formulation EPC-OP-HT5, since only a small number of females were in contact with the treated area, showing marked repellent impact. The other treatments, including low-dose nanosystems, showed less evident differences in the contact by medflies between treated and untreated zones. In a previous study, application of encapsulated HT at the same dose of 2.5 mg mL−1 was able to prevent fruit flies from landing on treated fruits.53 Here, this effect obtained` with EPC-OP-HT5 might be explained by the restricted space of the setup used in these experiments, which could hamper the females from flying and finding better egg laying sites.42,54 Therefore, a concentration higher than 2.5 mg mL−1 was needed in the present case to achieve the aversive effect.
However, our work shows that nanoformulations significantly enhanced the effectiveness of HT in deterring female medflies from laying eggs. Notably, after the treatments with both nanosystems EPC-OP-HT2.5 and EPC-OP-HT5, C. capitata did not lay any eggs at all for five hours and had a detectable effect of deterrence for at least 72 hours. We can thus suppose that encapsulation ensured HT stability from chemical degradation and prevented loss of effectiveness.55
Numerous studies indicate that female medflies release a pheromone after laying eggs, which deters other females from ovipositing in the same area.42,43,56 Besides, some authors indicate that feces from C. capitata females act as an oviposition-deterrent signal.42,57 Although our experiments did not give a direct indication on substance release, the decrease in oviposition deterrence that we observed after 72 hours can be reasonably assumed as an adaptive response which led medflies to lay eggs in less preferred areas of the net.
The role of the nanocarrier alone also warrants attention, as it could inhibit the egg laying of medflies within the first 5 hours. This effect could be partly explained by the presence of a certain amount of HT, i.e. 3.52 mg g−1 in the OP mixture, and of oleuropein (6.82 mg g−1) that could release it. In addition, the OP used to prepare our formulations also contains other polyphenols, like verbascoside (2.95 mg g−1) as well as oleuropein, which exhibited antinutritive effects against a variety of herbivorous insects (Abdellaoui et al., 2019; Debo et al., 2011; Konno et al., 1999; Muñoz et al., 2013). Oleuropein is also known to actively repel the olive fly, Bactrocera oleae (Rossi, 1790).58,59 The effectiveness of our formulations highlights their potential as bioactive agents, possibly due to synergic effect.
HT effectively reduces the number of S. oryzae adults entering traps, with a notable increase in repellency at higher HT concentrations (HT5) compared to lower concentrations (HT2.5). Furthermore, the use of HT-loaded nanocarriers (EPC-OP-HT) has shown enhanced effectiveness compared to pure HT. Thus, the number of adults captured in traps containing caryopses treated with the EPC-OP-HT2.5 and EPC-OP-HT5 was significantly lower, indicating an improvement in the repellent efficacy attributable to the nanosystem.
As previously stated, compounds containing HT repel B. oleae and C. capitata upon surface contact.53,58 Our study's results show that repellency was induced in the beetles without direct contact with the treated kernels, indicating a distinct process from contact-based effects. It suggests a possible involvement of volatile elements influencing the behavior of S. oryzae.
EPC-OP-HT2.5 and EPC-OP-HT5 nanoformulations also effectively deterred oviposition, as evidenced by a lower number of wheat seeds with egg plugs and high scores on the oviposition deterrence index (ODIEP). Even HT prevents adult weevils from ovipositing on wheat grains; HT2.5 and HT5 treatments had fewer infested kernels than the control and grains treated with EPC-OP. However, HT2.5 and HT5 displayed a near-zero ODI, and the effectiveness of the HT was not so pronounced. The existing literature on stored pest control mainly focuses on submicron structures encapsulating essential oils for managing insect infestations.19,60 Research on S. oryzae focuses on inducing mortality with essential oils, with few studies examining reproductive alteration of oviposition behavior.23,61,62 Besides, few studies explored nanoformulations for controlling S. oryzae using essential oils.63 The approach of our study could, therefore, mark a significant advance in the development of effective and targeted pest control strategies against S. oryzae.
Conclusions
In this work the effects of encapsulated HT on C. capitata and S. oryzae were investigated, revealing the superior performance of bioactive compounds when administered in the form of dispersed tiny particles. To optimize the biocompatibility of the carriers we used a mixture of natural lipids and other organic molecules, i.e. egg yolk phosphatidylcholine and olive pomace, obtaining well-defined and stable submicron aggregates. The structural investigation of these water formulations was performed by DLS and SAXS and allowed us to establish that the particle size decreased from ≈1 μm in the empty vectors to 250–350 nm in the loaded ones. This effect was due to a marked increase in the local curvature induced by HT association, suggesting that HT molecules were located on the external surface, i.e. with their polar moieties in contact with water. The basic structure of the aggregates was in all cases a bilayer, as established by SAXS, and it was clearly imparted by the large fraction of phospholipids present in the starting mixture. On the other hand, the negatively charged surface ensured stability in water, thus facilitating storage and administration. HT was loaded at concentrations of 2.5 and 5 mg mL−1 nanoformulations made from EPC and OP and the effectiveness of HT against C. capitata and S. oryzae was markedly increased. An important aspect to underline is that EPC-OP loaded formulations maintained their efficacy over extended periods (up to 72 hours for C. capitata and up to 21 days for S. oryzae), which is useful for parasite protection.
This study also reveals that the efficacy of HT could be dose-dependent.
In summary, we showed that HT-loaded carriers have the potential to reduce environmental impact and harm to non-target species, possibly leading to new pest management methods. This aligns with the global tendency towards sustainable, environmentally conscious agriculture, and holds promise for more targeted and efficient pest control strategies. Our research also lays the groundwork for future studies that will focus on optimizing the concentration and longevity of the submicron vectors as well as understanding how these compounds could work against complex organisms such as pest insects.
Author contributions
Sara Falsini: conceptualization, methodology, formal analysis, investigation, data curation, writing—review and editing. Marzia Cristiana Rosi: methodology, resources, supervision, project administration, funding acquisition. Annalisa Andreani and Silvia Urciuoli: resources, writing—review and editing. Alessio Papini: data curation, resources, writing—review and editing. Cristina Gonnelli: conceptualization, methodology, resources, writing—review and editing, supervision, project administration, funding acquisition. Sandra Ristori: conceptualization, methodology, resources, writing—review and editing, supervision, project administration, funding acquisition. All authors read and approved the final manuscript.
Conflicts of interest
There are no conflicts to declare.
Acknowledgements
We acknowledge the European Synchrotron Radiation Facility (ESRF) for beamtime provision. In particular, we would like to thank Dr. Theyencheri Narayanan and Dr. Michael Szutcki for their assistance and support in using the ID02 beamline. This work was supported by the Università degli Studi di Firenze (grant number Ricaten 2022, UniFi), Prof Sandra Ristori. This study was carried out within the Agritech National Research Center and received funding from the European Union Next-GenerationEU (Piano Nazionale di ripresa e Resilienza (PNRR) – Missione 4 Componente 2, Investimento 1.4 – D.D. 1032 17/06/2022, CN00000022). This manuscript reflects only the authors' views and opinions, neither the European Union nor the European Commission can be considered responsible for them.
References
- WT/CTE/W/193. The Stockholm Convention on Persistent Organic Pollutants. World Trade Organization. Available at: https://docs.wto.org/dol2fe/Pages/FE_Search/FE_S_S009-DP.aspx?language=E&CatalogueIdList=49699.
- S. Shekhar, S. Sharma and A. Kumar,
et al., The framework of nanopesticides: a paradigm in biodiversity, Mater. Adv., 2021, 2(20), 6569–6588, 10.1039/D1MA00329A.
- G. Benelli, B. Conti and R. Garreffa,
et al., Shedding light on bioactivity of botanical by-products: neem cake compounds deter oviposition of the arbovirus vector Aedes albopictus (Diptera: Culicidae) in the field, Parasitol. Res., 2014, 113(3), 933–940, DOI:10.1007/s00436-013-3725-4.
-
A. A. Gupta and A. R. Gupta, The Potential of Nano-Based Seed Priming for Sustainable Agriculture, Nanopriming Approach to Sustainable Agriculture, ed. A. Singh, V. Rajput, K. Ghazaryan, S. Gupta and T. Minkina, 2023, pp. 43–88 Search PubMed.
- A. C. Barbera, C. Maucieri and V. Cavallaro,
et al., Effects of spreading olive mill wastewater on soil properties and crops, a review, Agric. Water Manag., 2013, 119, 43–53, DOI:10.1016/j.agwat.2012.12.009.
-
M. K. Doula, Olive mill waste: recent advances for the sustainable development of olive oil industry, ed. C. M. Galanakis, 2017, ch. 2, pp. 29–56 Search PubMed.
-
M. F. Andres and A. González Coloma, Agro-industrial by-products and waste as sources of biopesticides, Biocontrol of Plant Disease: Recent Advances and Prospects in Plant Protection, John Wiley & Sons, Ltd., 2022, pp. 91–119 Search PubMed.
- I. Clemente, S. Falsini and E. Di Cola,
et al., Green Nanovectors for Phytodrug Delivery: In-Depth Structural and Morphological Characterization, ACS Sustainable Chem. Eng., 2019, 7(15), 12838–12846, DOI:10.1021/acssuschemeng.9b01748.
- R. Bernini, M. S. Gilardini Montani and N. Merendino,
et al., Hydroxytyrosol-Derived Compounds: A Basis for the Creation of New Pharmacological Agents for Cancer Prevention and Therapy, J. Med. Chem., 2015, 58(23), 9089–9107, DOI:10.1021/acs.jmedchem.5b00669.
- M. I. Fernández-Mar, R. Mateos and M. C. García-Parrilla,
et al., Bioactive compounds in wine: Resveratrol, hydroxytyrosol and melatonin: A review, Food Chem., 2012, 130(4), 797–813, DOI:10.1016/j.foodchem.2011.08.023.
- A. Ricelli, F. Gionfra and Z. Percario,
et al., Antioxidant and Biological Activities of Hydroxytyrosol and Homovanillic Alcohol Obtained from Olive Mill Wastewaters of Extra-Virgin Olive Oil Production, J. Agric. Food Chem., 2020, 68(52), 15428–15439, DOI:10.1021/acs.jafc.0c05230.
- X. Pan, X. Guo and T. Zhai,
et al., Nanobiopesticides in sustainable agriculture: developments, challenges, and perspectives, Environ. Sci.: Nano, 2023, 10(1), 41–61, 10.1039/D2EN00605G.
- G. Gasperi, M. Bonizzoni and L. M. Gomulski, Genetic Differentiation, Gene Flow and the Origin of Infestations of the Medfly, Ceratitis Capitata, Genetica, 2002, 116, 125–135, DOI:10.1023/A:1020971911612.
- I. Grechi, A.-L. Preterre and A. Caillat,
et al., Linking mango infestation by fruit flies to fruit maturity and fly pressure: A prerequisite to improve fruit fly damage management via harvest timing optimization, Crop Prot., 2021, 146, 105663, DOI:10.1016/j.cropro.2021.105663.
- M. Yones, G. A. Khdery and M. Aboelghar,
et al., Early detection of the Mediterranean Fruit Fly, Ceratitis capitata (Wied.) in oranges using different aspects of remote sensing applications, Egypt. J. Remote Sens. Space Sci., 2023, 26(3), 798–806, DOI:10.1016/j.ejrs.2023.08.002.
- Á. Mesterházy, J. Oláh and J. Popp, Losses in the Grain Supply Chain: Causes and Solutions, Sustainability, 2020, 12(6), 2342, DOI:10.3390/su12062342.
- A. Rita Devi, A. Thomas, K. B. Rebijith and V. V. Ramamurthy, Biology, morphology and molecular characterization of Sitophilus oryzae and S. zeamais (Coleoptera: Curculionidae), J. Stored Prod. Res., 2017, 73, 135–141, DOI:10.1016/j.jspr.2017.08.004.
- P. Trematerra, A. Valente and C. G. Athanassiou,
et al., Kernel-kernel interactions and behavioral responses of Sitophilus zeamais Motschulsky (Coleoptera: Curculionidae), Appl. Entomol. Zool., 2007, 42(1), 129–135, DOI:10.1303/aez.2007.129.
- Z. Afrose Subaitha, P. Santhoshkumar and J. A. Moses,
et al., Nonchemical strategies for stored product pest management: Exploring the potential of spices, herbs, and their formulations, Food Control, 2024, 158, 110212, DOI:10.1016/j.foodcont.2023.110212.
- O. Campolo, G. Giunti and A. Russo,
et al., Essential Oils in Stored Product Insect Pest Control, J. Food Qual., 2018, 2018, 1–18, DOI:10.1155/2018/6906105.
- P. M. Ngegba, G. Cui and M. Z. Khalid,
et al., Use of Botanical Pesticides in Agriculture as an Alternative to Synthetic Pesticides, Agriculture, 2022, 12(5), 600, DOI:10.3390/agriculture12050600.
- S. A. Papanastasiou, C. S. Ioannou and N. T. Papadopoulos, Oviposition-deterrent effect of linalool – a compound of citrus essential oils – on female Mediterranean fruit flies, Ceratitis capitata (Diptera: Tephritidae), Pest Manage. Sci., 2020, 76(9), 3066–3077, DOI:10.1002/ps.5858.
- N. Stefanazzi, T. Stadler and A. Ferrero, Composition and toxic, repellent and feeding deterrent activity of essential oils against the stored-grain pests Tribolium castaneum (Coleoptera: Tenebrionidae) and Sitophilus oryzae (Coleoptera: Curculionidae), Pest Manage. Sci., 2011, 67(6), 639–646, DOI:10.1002/ps.2102.
- V. Zeni, G. Benelli and O. Campolo,
et al., Toxics or Lures? Biological and Behavioral Effects of Plant Essential Oils on Tephritidae Fruit Flies, Molecules, 2021, 26(19), 5898, DOI:10.3390/molecules26195898.
- A. Romani, A. Scardigli and P. Pinelli, An environmentally friendly process for the production of extracts rich in phenolic antioxidants from Olea europaea L. and Cynara scolymus L. matrices, Eur. Food Res. Technol., 2017, 243(7), 1229–1238, DOI:10.1007/s00217-016-2835-5.
- A. Romani, M. Campo and S. Urciuoli,
et al., An Industrial and Sustainable Platform for the Production of Bioactive Micronized Powders and Extracts Enriched in Polyphenols From Olea europaea L. and Vitis vinifera L. Wastes, Front. Nutr., 2020, 7, 120, DOI:10.3389/fnut.2020.00120.
- A. Romani, F. Ieri and S. Urciuoli,
et al., Health Effects of Phenolic Compounds Found in Extra-Virgin Olive Oil, By-Products, and Leaf of Olea europaea L, Nutrients, 2019, 11(8), 1776, DOI:10.3390/nu11081776.
- E. Azadfar, A. H. Elhami Rad and A. Sharifi,
et al., Effect of Olive Pomace Fiber on the Baking Properties of Wheat Flour and Flat Bread (Barbari Bread) Quality, J. Food Process. Preserv., 2023, 2023(1), 1405758, DOI:10.1155/2023/1405758.
- T. B. Ribeiro, D. Campos and A. Oliveira,
et al. Study of olive pomace antioxidant dietary fibre powder throughout gastrointestinal tract as multisource of phenolics, fatty acids and dietary fibre, Food Res. Int., 2021, 142, 110032, DOI:10.1016/j.foodres.2020.110032.
- C. S. Speroni, A. B. B. Bender and J. Stiebe,
et al., Granulometric fractionation and micronization: A process for increasing soluble dietary fiber content and improving technological and functional properties of olive pomace, LWT, 2020, 130, 109526, DOI:10.1016/j.lwt.2020.109526.
- Z. Piñeiro, E. Cantos-Villar and M. Palma,
et al., Direct Liquid Chromatography Method for the Simultaneous Quantification of Hydroxytyrosol and Tyrosol in Red Wines, J. Agric. Food Chem., 2011, 59(21), 11683–11689, DOI:10.1021/jf202254t.
- E. Deletre, B. Schatz and D. Bourguet,
et al., Prospects for repellent in pest control: current developments and future challenges, Chemoecology, 2016, 26(4), 127–142, DOI:10.1007/s00049-016-0214-0.
- A. P. Economopoulos and S. Judt, Artificial Rearing of the Mediterranean Fruit Fly (Diptera: Tephritidae), Size of Oviposition Holes, J. Econ. Entomol., 1989, 82(2), 668–674, DOI:10.1093/jee/82.2.668.
- H. Lin, M. Kogan and D. Fischer, Induced Resistance in Soybean to the Mexican Bean Beetle (Coleoptera: Coccinellidae), Comparisons of Inducing Factors, Environ. Entomol., 1990, 19(6), 1852–1857, DOI:10.1093/ee/19.6.1852.
- J. Schindelin, I. Arganda-Carreras and E. Frise,
et al., Fiji: an open-source platform for biological-image analysis, Nat. Methods, 2012, 9(7), 676–682, DOI:10.1038/nmeth.2019.
- J. A. Ojo and A. A. Omoloye, Development and Life History of Sitophilus zeamais (Coleoptera: Curculionidae)
on Cereal Crops, Adv. Agric., 2016, 2016, 1–8, DOI:10.1155/2016/7836379.
- S. Majd-Marani, B. Naseri and M. Hassanpour,
et al., Life history and life table parameters of the rice weevil, Sitophilus oryzae L. (Coleoptera: Curculionidae) fed on 10 rice cultivars and lines in Iran, J. Stored Prod. Res., 2023, 102, 102118, DOI:10.1016/j.jspr.2023.102118.
-
R. Kumar, Insect Pests of Stored Grain: Biology, Behavior, and Management Strategies, Apple Academic Press, Waretown, NJ, 1st edn, 2017 Search PubMed.
- M. Milner, D. L. Barney and J. A. Shellenberger, Use of Selective Fluorescent Stains to Detect Insect Egg Plugs on Grain Kernels, Science, 1950, 112(2922), 791–792, DOI:10.1126/science.112.2922.791.
- A. Hematpoor, S. Y. Liew and M. S. Azirun,
et al., Insecticidal activity and the mechanism of action of three phenylpropanoids isolated from the roots of Piper sarmentosum Roxb, Sci. Rep., 2017, 7(1), 12576, DOI:10.1038/s41598-017-12898-z.
- R Core Team. R: A language and environment for statistical computing. R Foundation for Statistical Computing, 2022.
- J. Arredondo and F. Díaz-Fleischer, Oviposition deterrents for the Mediterranean fruit fly, Ceratitis capitata (Diptera: Tephritidae) from fly faeces extracts, Bull. Entomol. Res., 2006, 96(1), 35–42, DOI:10.1079/BER2005399.
- R. J. Prokopy, J. R. Ziegler and T. T. Y. Wong, Deterrence of repeated oviposition by fruit-marking pheromone inCeratitis capitata (Diptera: Tephritidae), J. Chem. Ecol., 1978, 4(1), 55–63, DOI:10.1007/BF00988260.
- J. Pagés and F. Husson, Multiple factor analysis with confidence ellipses: a methodology to study the relationships between sensory and instrumental data, J. Chemom., 2005, 19(3), 138–144, DOI:10.1002/cem.916.
- S. Lê, J. Josse and F. Husson, FactoMineR: An R Package for Multivariate Analysis, J. Stat. Softw., 2008, 25(1), 1–18, DOI:10.18637/jss.v025.i01.
- B. Wang and X. Cui, An improved uniformly more powerful exact Fisher-Hayter pairwise comparisons procedure: Improved Fisher-Hayter test, Biom. J., 2017, 59(4), 767–775, DOI:10.1002/bimj.201500265.
- G. L. Tietjen and R. H. Moore, Some Grubbs-Type Statistics for the Detection of Several Outliers, Technometrics, 1972, 14(3), 583–597, DOI:10.1080/00401706.1972.10488948.
- G. Pabst, M. Rappolt and H. Amenitsch,
et al., Structural information from multilamellar liposomes at full hydration: Full q -range fitting with high quality x-ray data, Phys. Rev. E, 2000, 62(3), 4000–4009, DOI:10.1103/PhysRevE.62.4000.
- S. Ristori, J. Oberdisse and I. Grillo,
et al., Structural Characterization of Cationic Liposomes Loaded with Sugar-Based Carboranes, Biophys. J., 2005, 88(1), 535–547, DOI:10.1529/biophysj.104.049080.
- F. Spinozzi, L. R. S. Barbosa and G. Corucci,
et al., Small-angle scattering from flat bilayers containing correlated scattering length density inhomogeneities, J. Appl. Crystallogr., 2023, 56(5), 1348–1360, DOI:10.1107/S1600576723006143.
- T. O. Machado, S. J. Beckers and J. Fischer,
et al., Bio-Based Lignin Nanocarriers Loaded with Fungicides as a Versatile Platform for Drug Delivery in Plants, Biomacromolecules, 2020, 21(7), 2755–2763, DOI:10.1021/acs.biomac.0c00487.
- F. Menicucci, M. Michelozzi and A. Raio,
et al., Thymol-loaded lipid nanovectors from the marine microalga Nannochloropsis sp. as potential antibacterial agents, Biocatal. Agric. Biotechnol., 2021, 32, 101962, DOI:10.1016/j.bcab.2021.101962.
- S. Falsini, M. C. Rosi and E. Ravegnini,
et al., Nanoformulations with exopolysaccharides from cyanobacteria: enhancing the efficacy of bioactive molecules in the Mediterranean fruit fly control, Environ. Sci. Pollut. Res., 2023, 30(35), 83760–83770, DOI:10.1007/s11356-023-28180-x.
-
F. Díaz-Fleischer, D. R. Papaj and R. Prokopy, et al., Evolution of Fruit Fly Oviposition Behavior, in Fruit Flies (Tephritidae), Phylogeny and Evolution of Behavior, ed. M. Aluja, A. Norrbom and D. Papaj, CRC Press, 1999, pp. 811–849 Search PubMed.
- M. Kah, N. Tufenkji and J. C. White, Nano-enabled strategies to enhance crop nutrition and protection, Nat. Nanotechnol., 2019, 14(6), 532–540, DOI:10.1038/s41565-019-0439-5.
- D. R. Papaj, R. J. Prokopy and P. T. McDonald,
et al., Differences in learning between wild and laboratory Ceratitis capitata flies, Entomol. Exp. Appl., 1987, 45(1), 65–72, DOI:10.1111/j.1570-7458.1987.tb02256.x.
- F. Díaz-Fleischer and M. Aluja, Clutch size in frugivorous insects as a function of host firmness: the case of the tephritid fly Anastrepha ludens, Ecol. Entomol., 2003, 28(3), 268–277, DOI:10.1046/j.1365-2311.2003.00517.x.
- R. Lo Scalzo, M. L. Scarpati and B. Verzegnassi,
et al., Olea europaea chemicals repellent to Dacus oleae females, J. Chem. Ecol., 1994, 20(8), 1813–1823, DOI:10.1007/BF02066224.
- A. Spadafora, S. Mazzuca and F. F. Chiappetta,
et al., Oleuropein-Specific-E-Glucosidase Activity Marks the Early Response of Olive Fruits (Olea europaea) to Mimed Insect Attack, Agric. Sci. China, 2008, 7(6), 703–712, DOI:10.1016/S1671-2927(08)60105-4.
- G. Benelli, Mode of action of nanoparticles against insects, Environ. Sci. Pollut. Res., 2018, 25(13), 12329–12341, DOI:10.1007/s11356-018-1850-4.
- K. C. Devi and S. S. Devi, Insecticidal and oviposition deterrent properties of some spices against coleopteran beetle, Sitophilus oryzae, J. Food Sci. Technol., 2013, 50(3), 600–604, DOI:10.1007/s13197-011-0377-1.
- R. R. Tangadi, G. S. Jadhav and A. A. Devarshi,
et al., Efficacy of plant essential oils against rice weevil, Sitophilus oryzae L, J. Biopestic., 2021, 14(1), 22–26 CrossRef.
- V. Rajkumar, C. Gunasekaran and C. A. Paul,
et al., Development of encapsulated peppermint essential oil in chitosan nanoparticles: characterization and biological efficacy against stored-grain pest control, Pestic. Biochem. Physiol., 2020, 170, 104679, DOI:10.1016/j.pestbp.2020.104679.
|
This journal is © The Royal Society of Chemistry 2024 |
Click here to see how this site uses Cookies. View our privacy policy here.