DOI:
10.1039/C6QM00362A
(Research Article)
Mater. Chem. Front., 2017,
1, 1384-1388
Chemically stable microporous hyper-cross-linked polymer (HCP): an efficient selective cationic dye scavenger from an aqueous medium†
Received
15th December 2016
, Accepted 17th February 2017
First published on 20th February 2017
Abstract
A cost-effective and robust microporous hyper-cross-linked organic material (HCP-91) has been post-synthetically modified for the rational incorporation of sodium cations via alkali treatment (HCP-91@Na). The presence of free cations has been tapped for the selective capture of cationic dyes via rapid ion-exchange. For this study we have used three cationic dye molecules [namely, methylene blue (MB), crystal violet (CV) and rhodamine B (RB)] and one anionic dye (methyl orange). The performance of HCP-91@Na in an aqueous medium for size and charge-selective dye entrapment has been comprehensively investigated with monocomponent dye solution studies, as well as binary mixtures of cationic and anionic dye molecules.
Introduction
Last decade has witnessed the evolution of microporous organic polymers (MOPs) in the domain of materials chemistry. The high surface area, tuneable porosity and high physiochemical stability of such materials have stimulated much interest among researchers.1 A wide range of microporous organic materials have been explored in recent times, such as covalent organic frameworks (COFs), porous organic frameworks (POFs), porous aromatic frameworks (PAFs), covalent triazine frameworks (CTFs), porous organic polymer (POPs), porous polymer networks (PPNs) etc.2 Hyper-cross-linked polymers (HCPs) have emerged as a relatively new subclass in the field of MOPs.3 Single step Friedel–Crafts reaction provides a key tool to build a network of aromatic molecules via extensive hyper-cross-linked polymerization in the presence of anhydrous FeCl3 and formaldehyde dimethyl acetal (FDA) as external cross-linking agents.4 In such cases small organic aromatic building units have been connected and held together by methylene (–CH2–) groups. Such extensive cross-linking among several organic building blocks results in permanent porosity and high stability of such materials. In addition to the simple one-step easy synthesis of HCPs and high physiochemical stability, tunable porosity by varying the organic unit and low cost synthesis have attracted great attention from researchers.5 It is noteworthy to mention that HCPs are a much less-explored class of materials in the regime of microporous organic materials. To date, microporous HCPs have often been used for carbon capture and catalysis applications; but to the best of our knowledge, pollutant removal from an aqueous medium has not been investigated yet.6
Dye molecules are widely used in many industries including pharmaceuticals, paper, printing, ceramics, paints, textile, cosmetics, plastics etc. The removal of such pollutant dye molecules from colored wastewater has emerged as a big challenge for researchers, as a consequence of heavy usage of dyes in the growing industries. It has been found that there are over 100
000 dyes available in the market with a production rate of over 7 × 105 tons per year; among which 2% of dyes are released into different water bodies.7 Water pollution because of dye molecules hinders growth of bacteria which are responsible for degradation of water impurities and also affects photosynthesis of aquatic plants.8 Apart from this, a few dyes can result into aesthetic problem as well as chronic effects on exposure to organisms.9 Furthermore, organic dye molecules are very much chemically stable (even stable to oxidation and light), so the chances of biodegradation for such water pollutants are much lower.10 Several techniques based on physical, chemical and biological methods have been introduced to remove dye pollutants from wastewater. Among them adsorption based dye removal has attracted much attention, because it can produce high quality water and function at ambient temperature, is cost-effective and can feasibly remove multiple dyes simultaneously.11 Although several adsorbents have been reported in the literature for the removal of dye molecules, materials with high capacity and separation by virtue of both size and ionic selectivity are still a challenge to be addressed. With advantages like tunable porosity and separation based on ionic selectivity, metal–organic frameworks (MOFs) have set up a new avenue in the domain of pollutant removal, like removing dye molecules from wastewater.12 An ionic framework of MOFs imparts selectivity in adsorption based on charge over respective dye molecules in addition to a size factor. Despite the impactful contribution of MOFs in the field of pollutant trapping, they have a few drawbacks like poor water stability because of weaker coordination bonds and difficulties with bulk scale synthesis.13
To overcome the aforementioned problems, researchers have come up with replacement of coordination bonds with stronger covalent bonds in the form of porous covalent organic materials. Porous organic framework based pollutant trapping from wastewater is in a very early stage. In particular, dye molecule capture from colored wastewater based on such materials is very rare and to the best of our knowledge cationic dye capture has not yet been reported in this domain.14 In this report, we have strategically synthesized alkoxide based microporous HCP through a base (aqueous NaOH) treatment of free –OH groups to get free cations inside the network. Thus the obtained exchangeable cations can easily be replaced by cationic dye molecules in a water medium. Based on this concept, we have performed adsorption of cationic dyes, viz., methylene blue (MB), crystal violet (CV) and rhodamine B (RB) from water solution.
Experimental
Materials
4-Phenylphenol, FeCl3, formaldehyde dimethyl acetal (FDA), methylene blue, crystal violet and rhodamine B were purchased from Sigma-Aldrich. Methyl orange and the remaining solvents were obtained locally. These chemicals were used without further purification.
Physical measurements
All infra-red spectra were acquired by using a NICOLET 6700 FT-IR spectrophotometer using KBr pellets in the 400–4000 cm−1 range. Gas adsorption measurements were acquired using a BelSorp-max instrument from Bel Japan. FESEM was carried out by using an FEI Quanta 3D dual beam ESEM at 30 kV. UV spectra were recorded on a Shimadzu UV 2600 Spectrophotometer.
UV-Vis measurements
The dye adsorption experiment was performed with 0.02 mM aqueous solutions of the respective dye molecules. In a typical experiment, first the UV-Vis spectrum was recorded for 2 mL of 0.02 mM dye solution and then 1 mg of HCP-91@Na was added to the solution. Thus UV-Vis spectra of each dye were recorded of the supernatant solution with certain time intervals.
Synthesis of HCP-91
HCP-91 has been synthesized from cross-coupling of 4-phenylphenol (Fig. 1) by following our previous report.5b To a round bottom flask 4-phenylphenol (300 mg, 1.7625 mmol) was added and to that 20 mL of dichloroethane (C2H4Cl2) was added. Then to the reaction mixture formaldehyde dimethyl acetal (470 μl, 5.288 mmol) and FeCl3 (860 mg, 5.288 mmol) were added respectively. The reaction mixture was heated at 50 °C for 5 hours and then was allowed to reflux at 80 °C for 20 hours (Fig. 1). On completion of the reaction a brown colored precipitate was filtered off and washed with DMF, methanol, water, chloroform, dichloromethane and tetrahydrofuran (THF) repeatedly. The thus obtained brown colored solid material was then kept in a 1
:
1 CHCl3–THF mixture (25 mL) for 3 days to remove the high boiling solvents from the porous network of HCP-91. Then the solvent exchanged phase of HCP-91 was heated at 100 °C under vacuum to obtain the guest free activated material and with this phase further studies were carried out. Yield: 335 mg.
Synthesis of HCP-91@Na
Desolvated HCP-91 (100 mg) was taken in a conical flask and to that 10 mL of distilled water was added. Then NaOH (40 mg, 1 mmol) was added to the reaction mixture and allowed to stir overnight at room temperature (Fig. 1). On completion of the reaction a dark brown colored precipitate was filtered off and washed with water several times to remove excess NaOH. Then the precipitate was again washed with DMF, methanol, water, chloroform, dichloromethane and tetrahydrofuran (THF) repeatedly. The thus obtained dark brown colored solid material (HCP-91@Na) was then kept in MeOH (25 mL) for 3 days and MeOH was changed two times in a day to remove the high boiling solvents from the porous network of HCP-91@Na. Then the solvent exchanged phase of HCP-91@Na was heated at 100 °C under vacuum to obtain the guest free activated material and with this phase further studies were carried out. Yield: 115 mg.
Result and discussion
Herein, we report the highly efficient cationic dye adsorbent HCP-91@Na, synthesized from –OH group containing HCP-91via reaction with aqueous NaOH. HCP-91 was synthesized by following our previous report via C–C cross coupling reaction (Friedel–Crafts reaction) of 4-phenylphenol in the presence of FeCl3 and FDA (Fig. 1).5b The thus synthesized HCP-91 was poured into a CHCl3–THF mixture to remove high boiling solvent molecules and later desolvated under vacuum at 100 °C. Desolvated HCP-91 was treated with NaOH in a water medium to convert hydroxyl groups to –O−Na+ (HCP-91@Na) (Scheme S2, ESI†). In a similar way, the solvent exchange of HCP-91@Na was carried out in methanol and later desolvation was carried out. Both HCP-91 and HCP-91@Na have been characterized using infra-red (IR) spectroscopy, thermo-gravimetric analysis (TGA), low temperature N2 adsorption and field emission scanning electron microscopy (FESEM).
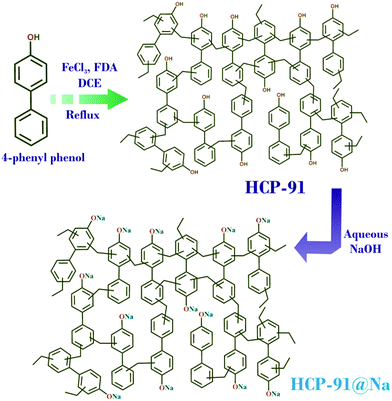 |
| Fig. 1 Schematic representation for the synthesis of HCP-91 and HCP-91@Na. | |
In IR spectroscopy, peaks at 1465 cm−1 and 1635 cm−1 in the case of HCP-91 and 1388 cm−1 and 1639 cm−1 for HCP-91@Na corresponding to the C
C double bond stretching frequencies are observed (Fig. S1, ESI†). The TGA profiles of the desolvated phases of both compounds revealed negligible loss up to ∼350 °C and after that a sharp decay was observed (Fig. S3, ESI†). As a consequence of incorporating sodium cations, the pore size of HCP-91@Na decreased as compared to HCP-91 and this is clearly reflected in the N2 adsorption isotherm at 77 K (Fig. S6, ESI†). FESEM images confirmed the retention of the agglomerated morphology of HCP-91 in HCP-91@Na too (Fig. S17, ESI†). EDAX analysis of HCP-91@Na confirmed the presence of sodium ions (Fig. S22, ESI†). To check the reversibility between HCP-91 and HCP-91@Na, we treated HCP-91@Na with 1 M HCl to get back HCP-91. The thus obtained material was characterized using IR spectroscopy, FESEM and N2 adsorption at 77 K. The IR spectra of HCP-91 and HCl treated HCP-91@Na matched suitably, and additionally the morphology was found to be similar from FESEM in both cases (Fig. S17, ESI†). N2 adsorption (at 77 K) substantiated this claim, where we found a similar adsorption of N2 for both HCP-91 and HCl treated HCP-91@Na (Fig. S6, ESI†).
To check the activity of HCP-91@Na towards cationic dye molecules, we dispersed 1 mg of the compound in 0.02 mM of methylene blue (MB). The blue colored aqueous solution transformed to a colorless solution after 20 minutes as a consequence of ion exchange. Enthused from this result, we sought to record UV-visible spectra of the dye solution at different time intervals to monitor adsorption of methylene blue by HCP-91@Na (Fig. S8, ESI†). To record UV-Vis data, 1 mg of HCP-91@Na was taken in a cuvette and 2 mL of 0.02 mM MB solution was poured into that. Then the spectrum was recorded at different times to get the respective absorbance which was directly correlated with the concentration of the dye solution. As a consequence of adsorption of dye molecules inside the network of HCP, we found a continuous decrease in the UV-Vis profile with time (Fig. 2a). From this data we found the concentration of the MB solution at different time intervals and also found the percent (%) of dye uptake by HCP-91@Na with time (Fig. S13, ESI†). In the concentration vs. time curve we observed a sharp decrease with time which lead to almost zero concentration of dye solution with saturation (Fig. S12, ESI†). As a result of methylene blue adsorption, brown colored HCP-91@Na converted to a green colored material (Fig. S8, ESI†). To validate our proposal we took another two cationic dyes with different sizes, namely, crystal violet (CV) and rhodamine B (RB). In a similar way, we performed a dye adsorption study with 1 mg of HCP-91@Na and 2 mL of 0.02 mM respective dye solutions. UV-Vis spectra revealed adsorption of both cationic dyes inside the porous network of HCP-91@Na (Fig. 2b and c). Because of the difference in size of the used dye molecules, the kinetics of adsorption also varied, i.e., the time for complete adsorption of 0.02 mM also changed (Fig. 3a). The sizes of the used dye molecules followed the order rhodamine B > crystal violet > methylene blue; consequently, the time taken for adsorption of the respective dyes followed a similar trend (Fig. 3a).
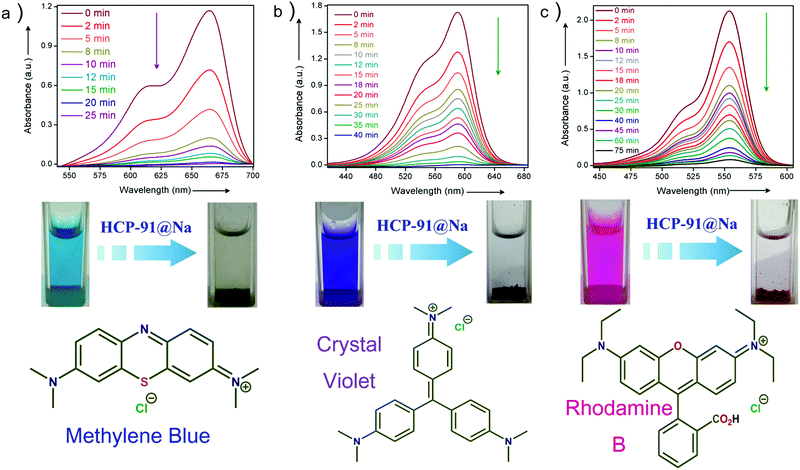 |
| Fig. 2 (a) Adsorption profile of methylene blue at different time intervals by HCP-91@Na and the corresponding decolorisation of blue solution (below), (b) UV-Vis spectra of the adsorption profile of crystal violet at different time intervals by HCP-91@Na and the corresponding decolorisation of violet solution (below), (c) UV-Vis spectra of the adsorption profile of methylene blue at different time intervals by HCP-91@Na and the corresponding decolorisation of pink solution (below). | |
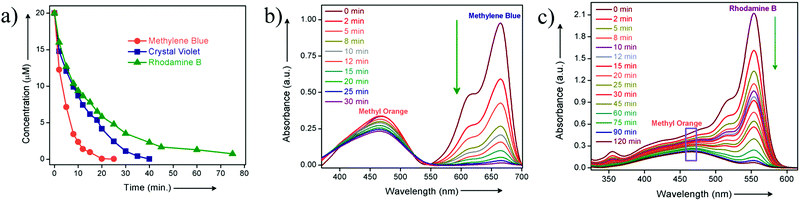 |
| Fig. 3 (a) Decrease in concentration of cationic dyes (methylene blue, crystal violet and rhodamine B) with time, (b) time dependent adsorption study of a mixture of methylene blue and methyl orange solution using UV-Vis spectroscopy, (c) time dependent adsorption study of a mixture of rhodamine B and methyl orange solution using UV-Vis spectroscopy. | |
In addition to the size factor, our synthesized HCP-91@Na showed selectivity in adsorption on the basis of charge over the adsorbates. To check ionic selectivity, we performed an adsorption study of a well known anionic dye, namely methyl orange (MO) with HCP-91@Na. Similar to the case for cationic dyes, 2 mL of 0.02 mM aqueous solution of methyl orange was poured into a cuvette containing 1 mg of HCP-91@Na and the UV-Vis spectra were recorded at different time intervals. We found a negligible change in the UV-Vis spectra of MO solution in comparison to a huge change in the case of cationic dyes solutions (Fig. S11, ESI†). These data affirmed our speculation over selectivity of HCP-91@Na based on the ionic charge of dye molecules. Furthermore, selective dye trapping from a mixture of solutions of cationic and anionic dyes is much more challenging and important as an ion-adsorbent. We sought to perform such an experiment with our material to check the efficiency of charge selective dye capture from a mixture of dyes. In a typical experiment, we took a 1
:
1 mixture of 0.02 mM of methylene blue and methyl orange dye solution. To the mixture of dye solutions, we added 1 mg of HCP-91@Na and monitored with UV-Vis spectroscopy. As anticipated, with time the peak corresponding to MB (λ = 670 nm) decreased sharply, whereas the peak for MO (λ = 460 nm) remained almost unaltered (Fig. 3b). In addition to the UV-Vis spectra, we observed the change visually. The color of the mixture solution was green initially (the color of MB is blue and MO is orange), but with time the color of the solution turned orange, as only methyl orange was left in the solution (Fig. S26, ESI†). For further validation, we performed the same experiment with a mixture of methyl orange and the largest dye used, i.e., rhodamine B. As per our expectation, here also we have found a similar result as in the case of the MB and MO mixture. Both the UV-Vis spectra and visual change validated our speculation as only RB got selectively adsorbed by HCP-91@Na and MO was left in the solution (Fig. 3c and Fig. S27, ESI†). Conclusively, our adsorbent material adsorbed selectively cationic dye molecules; on the other hand depending on the size of the cationic dyes the time of adsorption changed from lower to higher.
Conclusions
In conclusion, we have synthesized a robust and cost-effective microporous hyper-cross-linked polymer (HCP) and later carried out post-synthetic incorporation of cations inside the network (HCP-91). On the basis of cation exchange, efficient cationic dye capture has been performed with HCP-91@Na. Furthermore, we have shown selective cationic dye (methylene blue, crystal violet and rhodamine B) capture over anionic dyes (methyl orange) by virtue of the ionic charge selectivity. To the best our knowledge, selective cationic dye capture in the regime of a microporous organic framework has not been reported to date. We believe that this work will propel research in the area of pollutant trapping with such robust and cost-effective materials.
Acknowledgements
P. S. is thankful to UGC for research fellowships. A. V. D. and P. C. are thankful to IISER Pune for research fellowships. We are grateful to IISER Pune for research facilities. DST (Project No. 3116248) is acknowledged for the financial support.
Notes and references
-
(a) Y. Xu, S. Jin, H. Xu, A. Nagai and D. Jiang, Chem. Soc. Rev., 2013, 42, 8012 RSC;
(b) A. G. Slater and A. I. Cooper, Science, 2015, 348 DOI:10.1126/science.aaa8075;
(c) P. J. Waller, F. Gándara and O. M. Yaghi, Acc. Chem. Res., 2015, 48, 3053 CrossRef CAS PubMed;
(d) D. Yuan, W. Lu, D. Zhao and H.-C. Zhou, Adv. Mater., 2011, 23, 3723 CrossRef CAS PubMed;
(e) R. Dawson, E. Stöckel, J. R. Holst, D. J. Adams and A. I. Cooper, Energy Environ. Sci., 2011, 4, 4239 RSC;
(f) C. R. DeBlase, K. E. Silberstein, T.-T. Truong, H. D. Abruña and W. R. Dichtel, J. Am. Chem. Soc., 2013, 135, 16821 CrossRef CAS PubMed.
-
(a) M. G. Rabbani and H. M. El-Kaderi, Chem. Mater., 2011, 23, 1650 CrossRef CAS;
(b) B. Li, Y. Zhang, D. Ma, Z. Shi and S. Ma, Nat. Commun., 2014 DOI:10.1038/ncomms6537;
(c) W. Lu, D. Yuan, D. Zhao, C. I. Schilling, O. Plietzsch, T. Muller, S. Bräse, J. Guenther, J. Blümel, R. Krishna, Z. Li and H.-C. Zhou, Chem. Mater., 2010, 22, 5964 CrossRef CAS;
(d) A. Karmakar, A. Kumar, A. K. Chaudhari, P. Samanta, A. V. Desai, R. Krishna and S. K. Ghosh, Chem. – Eur. J., 2016, 22, 4931 CrossRef CAS PubMed;
(e) T. Ben, H. Ren, S. Q. Ma, D. P. Cao, J. H. Lan, X. F. Jing, W. C. Wang, J. Xu, F. Deng, J. M. Simmons, S. L. Qiu and G. S. Zhu, Angew. Chem., Int. Ed., 2009, 48, 9457 CrossRef CAS PubMed;
(f) H. A. Patel, S. H. Je, J. Park, D. P. Chen, Y. Jung, C. T. Yavuz and A. Coskun, Nat. Commun., 2013 DOI:10.1038/ncomms2359;
(g) S. Dalapati, E. Jin, M. Addicoat, T. Heine and D. Jiang, J. Am. Chem. Soc., 2016, 138, 5797 CrossRef CAS PubMed;
(h) A. Karmakar, R. Illathvalappil, B. Anothumakkool, A. Sen, P. Samanta, A. V. Desai, S. Kurungot and S. K. Ghosh, Angew. Chem., Int. Ed., 2016, 55, 10667 CrossRef CAS PubMed.
-
(a) Y. Luo, B. Li, W. Wang, K. Wu and B. Tan, Adv. Mater., 2012, 24, 5703 CrossRef CAS PubMed;
(b) R. Dawson, L. A. Stevens, T. C. Drage, C. E. Snape, M. W. Smith, D. J. Adams and A. I. Cooper, J. Am. Chem. Soc., 2012, 134, 10741 CrossRef CAS PubMed.
-
(a) B. Li, R. Gong, W. Wang, X. Huang, W. Zhang, H. Li, C. Hu and B. Tan, Macromolecules, 2011, 44, 2410 CrossRef CAS;
(b) R. Dawson, T. Ratvijitvech, M. Corker, A. Laybourn, Y. Z. Khimyak, A. I. Cooper and D. J. Adams, Polym. Chem., 2012, 3, 2034 RSC.
-
(a) S. Bhunia, B. Banerjee and A. Bhaumik, Chem. Commun., 2015, 51, 5020 RSC;
(b) P. Samanta, P. Chandra and S. K. Ghosh, Beilstein J. Org. Chem., 2016, 12, 1981 CrossRef CAS PubMed.
-
(a) R. T. Woodward, L. A. Stevens, R. Dawson, M. Vijayaraghavan, T. Hasell, I. P. Silverwood, A. V. Ewing, T. Ratvijitvech, J. D. Exley, S. Y. Chong, F. Blanc, D. J. Adams, S. G. Kazarian, C. E. Snape, T. C. Drage and A. I. Cooper, J. Am. Chem. Soc., 2014, 136, 9028 CrossRef CAS PubMed;
(b) S. Mondal, J. Mondal and A. Bhaumik, ChemCatChem, 2015, 7, 3570 CrossRef CAS;
(c) X. Jing, D. Zou, P. Cui, H. Ren and G. Zhu, J. Mater. Chem. A, 2013, 1, 13926 RSC;
(d) J. Wang, W. Sng, G. Yi and Y. Zhang, Chem. Commun., 2015, 51, 12076 RSC.
-
(a) S. J. Allen and B. Koumanova, J. Univ. Chem. Technol. Metall., 2005, 40, 175 CAS;
(b) C.-C. Wang, J.-R. Li, X.-L. Lv, Y.-Q. Zhang and G. Guo, Energy Environ. Sci., 2014, 7, 2831 RSC.
- X. Liu, W. Gong, J. Luo, C. Zou, Y. Yang and S. Yang, Appl. Surf. Sci., 2016, 362, 517 CrossRef CAS.
-
(a) A. Ayati, M. N. Shahrak, B. Tanhaei and M. Sillanpää, Chemosphere, 2016, 160, 30 CrossRef CAS PubMed;
(b) S.-R. Zhang, J. Li, D.-Y. Du, J.-S. Qin, S.-L. Li, W.-W. He, Z.-M. Su and Y.-Q. Lan, J. Mater. Chem. A, 2015, 3, 23426 RSC;
(c) S. Chen, J. Zhang, C. Zhang, Q. Yue, Y. Li and C. Li, Desalination, 2010, 252, 149 CrossRef CAS;
(d) A. Mittal, A. Malviya, D. Kaur, J. Mittal and L. Kurup, J. Hazard. Mater., 2007, 148, 229 CrossRef CAS PubMed.
-
(a) E. Haque, V. Lo, A. I. Minett, A. T. Harris and T. L. Church, J. Mater. Chem. A, 2014, 2, 193 RSC;
(b) E. Haque, J. E. Lee, I. T. Jang, Y. K. Hwang, J.-S. Chang, J. Jegal and S. H. Jhung, J. Hazard. Mater., 2010, 181, 535 CrossRef CAS PubMed.
-
(a) G. Crini, Non-conventional low-cost adsorbents for dye removal: a review, Bioresour. Technol., 2006, 97, 1061 CrossRef CAS PubMed;
(b) K. K. H. Choy, G. McKay and J. F. Porter, Resour., Conserv. Recycl., 1999, 27, 57 CrossRef.
-
(a) C. Zou, Z. Zhang, X. Xu, Q. Gong, J. Li and C.-D. Wu, J. Am. Chem. Soc., 2012, 134, 87 CrossRef CAS PubMed;
(b) X. Zhao, X. Bu, T. Wu, S.-T. Zheng, L. Wang and P. Feng, Nat. Commun., 2013 DOI:10.1038/ncomms3344;
(c) M.-L. Ma, J.-H. Qin, C. Ji, H. Xu, R. Wang, B.-J. Li, S.-Q. Zang, H.-W. Hou and S. R. Batten, J. Mater. Chem. C, 2014, 2, 1085 RSC;
(d) Z. Zhu, Y.-L. Bai, L. Zhang, D. Sun, J. Fang and S. Zhu, Chem. Commun., 2014, 50, 14674 RSC;
(e) Y.-Q. Lan, H.-L. Jiang, S.-L. Li and Q. Xu, Adv. Mater., 2011, 23, 5015 CrossRef CAS PubMed;
(f) J.-S. Qin, S.-R. Zhang, D.-Y. Du, P. Shen, S.-J. Bao, Y.-Q. Lan and Z.-M. Su, Chem. – Eur. J., 2014, 20, 5625 CrossRef CAS PubMed;
(g) P. Li, N. A. Vermeulen, X. Gong, C. D. Malliakas, J. F. Stoddart, J. T. Hupp and O. K. Farha, Angew. Chem., Int. Ed., 2016, 128, 10514 CrossRef;
(h) S. Kumar, G. Verma, W.-Y. Gao, Z. Niu, L. Wojtas and S. Ma, Eur. J. Inorg. Chem., 2016, 4373 CrossRef CAS;
(i) P. Yu, Q. Li, Y. Hu, N. Liu, L. Zhang, K. Su, J. Qian, S. Huang and M. Hong, Chem. Commun., 2016, 52, 7978 RSC;
(j) X. Liu, Z. Xiao, J. Xu, W. Xu, P. Sang, L. Zhao, H. Zhu, D. Sun and W. Guo, J. Mater. Chem. A, 2016, 4, 13844 RSC;
(k) D. Chen, W. Shi and P. Cheng, Chem. Commun., 2015, 51, 370 RSC;
(l) Y. Han, S. Sheng, F. Yang, Y. Xie, M. Zhaoa and J.-R. Li, J. Mater. Chem. A, 2015, 3, 12804 RSC;
(m) Z. Hasan and S. H. Jhung, J. Hazard. Mater., 2015, 283, 329 CrossRef CAS PubMed;
(n) H. Hahm, S. Kim, H. Ha, S. Jung, Y. Kim, M. Yoon and M. Kim, CrystEngComm, 2015, 17, 8418 RSC;
(o) Y. Zhu, Y.-M. Wang, S.-Y. Zhao, P. Liu, C. Wei, Y.-L. Wu, C.-K. Xia and J.-M. Xie, Inorg. Chem., 2014, 53, 7692 CrossRef CAS PubMed;
(p) A. V. Desai, B. Manna, A. Karmakar, A. Sahu and S. K. Ghosh, Angew. Chem., Int. Ed., 2016, 55, 7811 CrossRef CAS PubMed;
(q) Y.-Y. Jia, Y.-H. Zhang, J. Xu, R. Feng, M.-S. Zhang and X.-H. Bu, Chem. Commun., 2015, 51, 17439 RSC;
(r) A. Karmakar, B. Joarder, A. Mallick, P. Samanta, A. V. Desai, S. Basu and S. K. Ghosh, Chem. Commun., 2017, 53, 1253 RSC.
-
(a) D. M. D'Alessandro, B. Smit and J. R. Long, Angew. Chem., Int. Ed., 2010, 49, 6058 CrossRef PubMed;
(b) W. Lu, D. Yuan, J. Sculley, D. Zhao, R. Krishna and H.-C. Zhou, J. Am. Chem. Soc., 2011, 133, 18126 CrossRef CAS PubMed.
- S.-B. Yu, H. Lyu, J. Tian, H. Wang, D.-W. Zhang, Y. Liu and Z.-T. Li, Polym. Chem., 2016, 7, 3392 RSC.
Footnote |
† Electronic supplementary information (ESI) available. See DOI: 10.1039/c6qm00362a |
|
This journal is © the Partner Organisations 2017 |
Click here to see how this site uses Cookies. View our privacy policy here.