DOI:
10.1039/C8QM00147B
(Research Article)
Mater. Chem. Front., 2018,
2, 1508-1514
Porous metal–organic frameworks based on 3,6-bis(4-benzoic acid)-N-(4-benzoic acid)carbazole for HPLC separation of small organic molecules†
Received
4th April 2018
, Accepted 26th May 2018
First published on 29th May 2018
Abstract
Porous metal–organic frameworks (MOFs) with an extensive range of pore sizes have great potential for efficient separation of small organic molecules. Herein, we report a new stable porous metal–organic framework {Cu3L2(4,4′-BPD)[NH(CH3)2]·7DMA·12H2O}n (YCZ-1), which was fabricated from a Cu-paddlewheel metal node and a new linker, namely 3,6-bis(4-benzoic acid)-N-(4-benzoic acid) carbazole (H3L). YCZ-1 possesses a doubly interpenetrated 3,5,6-connected three-dimensional (3D) framework with a Schläfli symbol of {512·83}·{52·8}4·{56·84}2, which is a new topology. The crystalline size of YCZ-1 could be controlled by adding different doses of dimethylamine, which would also be generated from DMA dissociated during the solvothermal reaction. Meanwhile, with excellent structural stability and outstanding surface area, the crystalline material YCZ-1 could be utilized as a stationary phase for high performance liquid chromatography (HPLC) separations during the separation of small organic molecules.
Introduction
Metal–organic frameworks, assembled from organic linkers and metal nodes, have been extensively studied as porous materials for applications in gas storage, separation, catalysis and drug delivery.1–7 The flexibility of metal–organic frameworks provides an opportunity for engineering the structure and absorption properties.8–13 And the well-defined pore structures and high adsorption capacity indicate that MOFs have outstanding separation ability for small-molecules, positional isomers and chiral molecules.14–16
Due to some small organic molecules having similar physical properties or sensitivity to heat, separating them efficiently at room temperature would be an impressive progress for monitoring the self-regulating feedback controlled production of high purity reagents.17,18 The separation characteristics of MOFs could potentially increase the separation performance of high performance liquid chromatography (HPLC) when MOFs are used as stationary phases in the column.14–16,19,20 In 2007, the first example of a MOF-coated HPLC column for separating (R)- and (S)-enantiomers of several sulfoxides was reported by Nuzhdin and co-workers. Since then, plenty of MOFs have been used as stationary phases in HPLC.16,21–24 Meanwhile, some methods have been reported for controlling the particle size of MOF crystalline materials on a macroscale.25–29 With a suitable particle size, MOF crystalline materials would have better separation performance in HPLC.
Carbazole, a star aromatic compound, is commonly used to synthesize stable porous materials.30–32 Owing to its excellent thermal and photochemical stability, large conjugated π system and outstanding hole transporting ability, carbazole-based polymers are excellent candidates for polymers and porous structure materials.30–33 Carbazole can be functionalized at the (3,6-), (2,7-) or N positions.33–35 Although 2,7-carbazole-based polymers have a lower band gap and better fluorescence properties, 3,6-carbazole-based polymers have more outstanding structural extensibility and tend to obtain porous structures.
Therefore, 3,6-bis(4-benzoic acid)-N-(4-benzoic acid)carbazole (H3L) was designed and synthesized for the first time based on the fact that the appended benzoic acid moieties will significantly increase the size of the ligand.33–37 Sizeable ligands contribute to the formation of MOFs with high surface area and large pores, which would be helpful to improve separation characteristics.9,31
Herein, we report a novel porous metal–organic framework, {Cu3L2(4,4′-BPD)[NH(CH3)2]·7DMA·12H2O}n (YCZ-1) (DMA = N,N-dimethylacetamide, 4,4′-BPD = 4,4′-bipyridine, YCZ = MOFs with “Y”-shape carbazole-based linkers), based on 3,6-bis(4-benzoic acid)-N-(4-benzoic acid)carbazole (H3L). YCZ-1 exhibits excellent structural stability and outstanding surface area. Due to the presence of special pores, YCZ-1 could potentially be utilized for the separation of some small organic molecules. In addition, the particle size of YCZ-1 could be designed through synthesis with different initial reactants, which would influence the rate of the rate-determining step of the solvothermal reaction. Moreover, a suitable YCZ-1 particle size, compared with the frits of HPLC, could be utilized as the stationary phase for HPLC separations. As expected, the column, which was packed with YCZ-1 crystalline materials, shows good separation ability for small organic molecules (Scheme 1).
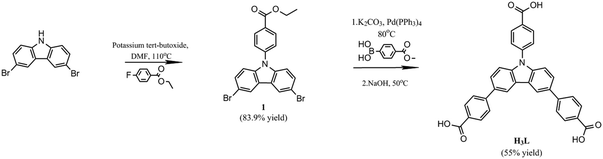 |
| Scheme 1 Synthesis of 3,6-bis(4-benzoic acid)-N-(4-benzoic acid)carbazole (H3L). | |
Experimental
Materials and methods
Reactants and solvents were purchased commercially and used directly. Elemental analyses (C, H and N) were performed with an Elementar Vario EL-Cube Element Analyzer. Powder X-ray diffraction (PXRD) data were collected on a Rigaku MiniFlex 600 diffractometer using Cu Kα radiation (λ = 0.154 nm). Infrared spectra were recorded with KBr pellets in the range 4000–400 cm−1 on a Perkin-Elmer Spectrum One FT-IR spectrometer. Thermogravimetric analysis (TGA) experiments were carried out on a NETZSCH STA 449C Jupiter thermogravimetric analyzer in flowing nitrogen with the sample heated in an Al2O3 crucible from room temperature to 1000 °C at a heating rate of 10 K min−1. Gas sorption isotherms of activated YCZ-1 were measured on a Micromeritics ASAP 2020 surface area analyzer. Particle size distribution analysis was carried out on a Malvern Mastersizer 2000 particle size analyzer.
Synthesis of the ligands
Synthesis of 3,6-dibromo-N-(4-ethyl benzoate)carbazole (1).
Potassium tert-butoxide (3.36 g, 30 mmol) and 3,6-dibromocarbazole (9.78 g, 30 mmol) were dissolved in dry DMF (300 ml) in a two-necked flask fitted with a magnetic stirrer and condenser. Under an atmosphere of nitrogen, the solution was stirred at 110 °C for 60 min and then ethyl 4-fluorobenzoate (6.10 ml, 33 mmol) was added. The system was allowed to react for 24 h and an apricot solution was obtained. The solvents were then removed by vacuum distillation. The crude residue was recrystallized from ethanol (50 ml). A white powder was obtained (11.5 g, 83.9% yield). 1H NMR (400 MHz, CDCl3-d1) δ (ppm) = 8.32 (d, J = 8.5 Hz, 2H), 8.22 (s, 2H), 7.62 (d, J = 8.5 Hz, 2H), 7.54 (d, J = 8.5 Hz, 2H), 7.32 (d, J = 8.5 Hz, 2H), 4.47 (dd, J = 7.0 Hz, 2H), 1.47 (t, J = 7.0 Hz, 3H). 13C NMR (125 MHz, CDCl3-d1) δ (ppm) = 167.33, 147.99, 141.05, 134.19, 130.66, 124.60, 124.18, 123.80, 117.46, 61.28, 14.70. MS (EI): calculated for C21H15Br2NO2m/z: 473.16, found m/z: 473.06.
Synthesis of 3,6-bis(4-benzoic acid)-N-(4-benzoic acid)carbazole (H3L).
A solution of methyl 4-boronobenzoate (2.17 g, 12 mmol) and K2CO3 (38.8 g, 0.28 mol) in 1
:
1 EtOH/H2O (80 ml) was added to a solution of 3,6-dibromo-N-(4-methyl benzoate)carbazole (2.51 g, 5.5 mmol) in toluene (80 ml). The reaction was stirred for 20 min and then tetrakis(triphenylphosphine) palladium(0) (0.300 g, 0.26 mmol) was added as a catalyst. The suspension was refluxed under nitrogen for 5 days. The reaction mixture was allowed to cool to room temperature and the solid in the reaction mixture was removed by filtration. The filtrate was extracted with ethyl acetate and the solvent in the collected organics was removed under reduced pressure. The white solid obtained was added to a solution of NaOH (1.20 g, 30 mmol) in 2
:
1
:
1 H2O/methanol/tetrahydrofuran (30 ml). The mixture was stirred at 50 °C overnight and then it was added to a solution of HCl (300 ml, pH = 2). After stirring for 10 min, the sediment was collected by filtration, washed with tetrahydrofuran and ether several times and then allowed to dry under reduced pressure, and a canary solid was obtained (1.59 g, yield 55%). 1H NMR(400 MHz, DMSO-d6) δ (ppm) = 8.91 (s, 2H), 8.28 (d, J = 8.5 Hz, 2H), 8.08 (d, J = 8.4 Hz, 4H), 7.99 (d, J = 8.4 Hz, 4H), 7.91(d, J = 8.7 Hz, 2H), 7.88 (d, J = 8.4 Hz, 2H), 7.63(d, J = 8.6Hz, 2H). 13C NMR (400 MHz, DMSO-d6) δ (ppm) = 167.70, 167.18, 145.14, 140.96, 140.58, 132.26, 131.85, 130.48, 129.54, 129.41, 127.42, 127.28, 126.74, 124.54, 120.09, 111.05. MS (EI): calculated for C33H21NO6m/z: 526.14, found m/z: 526.13.
Synthesis of {Cu3L2(4,4′-BPD)[NH(CH3)2]·7DMA·12H2O}n (YCZ-1).
Solvothermal reaction of Cu(NO3)2·3H2O (0.024 g, 0.1 mmol), 3,6-bis(4-benzoic acid)-N-(4-benzoic acid) carbazole (H3L) (26.3 mg, 0.05 mmol), 4,4′-bipyridine (7.8 mg, 0.1 mmol) and triethylamine (19 μl) in a mixture of N,N′-dimethylacetamine (DMA) (3 ml) and H2O (1 ml) at 383 K for seven days gave rise to emerald octahedral crystals of YCZ-1 (16.5 mg, yield: 41%). Anal. calcd (%) for C106H138N12O31Cu3: C 56.16, H 6.14, N 7.41, O 21.88. Found: C 56.12, H 6.13, N 7.38, O 21.92.
Synthesis of YCZ-1(0.1N).
Solvothermal reaction of Cu(NO3)2·3H2O (0.024 g, 0.1 mmol), 3,6-bis(4-benzoic acid)-N-(4-benzoic acid)carbazole (H3L) (26.3 mg, 0.05 mmol), 4,4′-bipyridine (7.8 mg, 0.1 mmol) and triethylamine (19 μl) and dimethylamine (0.66 μl, 0.1 N) in a mixture of N,N′-dimethylacetamine (DMA) (3 ml) and H2O (1 ml) at 383 K for six days gave rise to emerald octahedral crystals of YCZ-1(0.1N) (16.9 mg, yield: 42%).
Synthesis of YCZ-1(1N).
Solvothermal reaction of Cu(NO3)2·3H2O (0.024 g, 0.1 mmol), 3,6-bis(4-benzoic acid)-N-(4-benzoic acid)carbazole (H3L) (26.3 mg, 0.05 mmol), 4,4′-bipyridine (7.8 mg, 0.1 mmol) and triethylamine (19 μl) and dimethylamine (6.6 μl, 0.1 N) in a mixture of N,N′-dimethylacetamine (DMA) (3 ml) and H2O (1 ml) at 383 K for three days gave rise to emerald octahedral crystals of YCZ-1(1N) (17.6 mg, yield: 44%).
Single-crystal structure determination
Analysis of YCZ-1 was performed on a SuperNova, Dual, Cu at zero, Atlas diffractometer equipped with graphite-monochromated Cu Ka radiation (λ = 1.54184 Å) at 293(2) K. The structures were solved by direct methods and refined by full matrix least-squares of F2 using the SHELX-2018/1 program.38,39 Hydrogen atoms were added in idealized positions. The SQUEEZE routine of the PLATON software suite was used in removing highly disordered solvent molecules in YCZ-1.40,41 The final formula was calculated according to the SQUEEZE results combined with the results from elemental analyses and thermogravimetric analysis (TGA).42,43 Crystal data for YCZ-1 are presented in Table 1. Selected bond lengths and angles of YCZ-1 are listed in Table S1 of the ESI.†
Table 1 Crystal and X-ray experimental data for YCZ-1
Compound |
YCZ-1
|
R = ∑||Fo| − FC||/∑|Fo|.
wR2 = [P[w(Fo2 − FC2)2]/∑[(Fo2)2]]1/2.
|
Empirical formula |
C54H69N6O15.5Cu1.5 |
Formula weight |
1145.49 |
Crystal system |
Tetragonal |
Space group |
P42/nmc |
a (Å) |
31.8272(3) |
b (Å) |
31.8272(3) |
c (Å) |
27.8945(8) |
α (°) |
90 |
β (°) |
90 |
γ (°) |
90 |
V (Å3) |
28256.3(10) |
Z
|
16 |
Temperature (K) |
293(2) |
D
c (Mg m−3) |
1.077 |
μ (mm−1) |
0.792 |
F(000) |
9640 |
R
1,a wR2b (I > 2σ(I)) |
0.0985, 0.3260 |
θ
ranges (deg) |
3.46 to 74.53 |
h, k, l ranges |
−27 ≤ h ≤ 39 |
−37 ≤ k ≤ 27 |
−33 ≤ l ≤ 23 |
GOF on F2 |
1.098 |
Preparation of a HPLC column using YCZ-1(1N) particles
The separation performance of YCZ-1 toward small organic molecules was also studied. As shown in Fig. S1 (ESI†), a suspension of YCZ-1(1N) (ca. 20 mg) in HPLC-grade hexanes was slurry-loaded into an HPLC column (30 × 2.1 mm) and HPLC-grade hexanes/methanol was chosen as the mobile phase. All of the separation experiments were carried out at room temperature at a flow rate of 0.5 ml min−1.
Results and discussion
Crystal structure of YCZ-1
Solvothermal reaction of H3L and 4,4′-bipytidine in a solution of Cu(NO3)2 at 383 K for seven days gave the emerald crystalline YCZ-1. Single crystal X-ray diffraction of the emerald octahedral YCZ-1 revealed that YCZ-1 crystallizes in the tetragonal P42/nmc space group and in the form of a stable doubly interpenetrated framework.
In each framework unit of the doubly interpenetrated framework, the MOF is composed of two kinds of Cu paddlewheel metal nodes, which are in a different coordination environment, connected together by carbazole-based L3− ligands and 4,4′-bipyridine. The first kind of Cu paddlewheel metal node in YCZ-1 is coordinated to four carboxyl groups of the benzoxy groups which connect with carbazole in the N-position (Cu-pdw-1). And the co-ligand 4,4′-bipyridine coordinates to the axial position of two Cu-pdw-1s, making every Cu-pdw-1 a six-connected node in the framework [Fig. S2(a), ESI†]. The second kind of Cu paddlewheel node in YCZ-1 is coordinated to four carboxyl groups of the benzoxy group which connects with carbazole in the 3,6-position (Cu-pdw-2). One of the axial positions of Cu-pdw-2 is coordinated to 4,4′-bipyridine and the other axial position of Cu-pdw-2 is coordinated to dimethylamine, the product decomposed from DMA [Fig. S2(b), ESI†].
In the ligands, the three benzoxy groups and carbazole plane are twisted; the dihedral angle between the carbazole plane and the benzoxy group connected in the N-position is 53.1° and the dihedral angle between the carbazole plane and the benzoxy group connected in the 3,6-position is 26.5° and 37.8°, respectively.
Topologically, the L3− ligands could be regarded as 3-connected nodes. Cu-pdw-1 nodes could be simplified as 6-connected nodes and Cu-pdw-2 nodes could be simplified as 5-connected nodes. As shown in Fig. 1(c) and (d), the structure of YCZ-1 can be described with a Schläfli symbol of {512·83}·{52·8}4·{56·84}2, which is a new topology.44
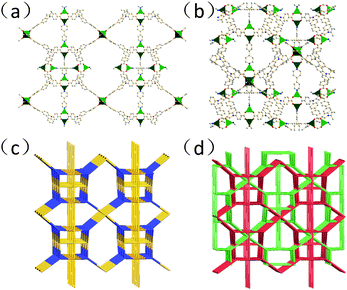 |
| Fig. 1 (a) View of the 3D framework unit; (b) views of the doubly interpenetrated frameworks of YCZ-1; (c) topological representation of the 3D framework unit; (d) topological representation of the doubly interpenetrated frameworks of YCZ-1. | |
A PLATON calculation indicated that YCZ-1 contains both mesopores and micropores and the total potential solvent volume is 17654.8 Å3, which corresponds to 62.9% (28077.0 Å3) cell volume.40,41
The framework structure of YCZ-1 is neutral by calculation of its charge balance. The H2O and DMA molecules could be estimated by elemental analysis (EA), thermogravimetric analysis (TGA) and the result of counting electrons calculated by PLATON.35,42,43 According to the TGA data, a weight loss of 10% from room temperature to 100 °C could be attributed to the loss of H2O in the channels of YCZ-1. Then, a pronounced weight loss of 26% from 150 to 200 °C could be observed because of the loss of DMA molecules. The framework starts to collapse and decompose after a brief period of stability from 200 °C to 250 °C (Fig. S4, ESI†). The EA data are consistent with the calculation of elemental composition according to the TGA data. And the deviation between the results of counting electrons calculated by PLATON and from the TGA data is less than 3%.
X-ray powder diffraction and stability analysis
Powder X-ray diffraction (PXRD) for the bulk samples of YCZ-1 was carried out at different temperatures from 30 °C to 250 °C (Fig. S5, ESI†). The PXRD patterns indicated that the framework was stable under 250 °C. And above that temperature, the framework began to collapse and decompose. The experimental PXRD patterns, for the material in which the disordered solvent molecules in the pores have been removed between 150 and 200 °C, correspond well with the simulated data which were simulated from the single crystal X-ray diffraction data. Meanwhile, the TGA data match with the PXRD patterns in different temperatures very well. In order to confirm the stability of YCZ-1 in different solvents, the crystalline material was also immersed in different solvents at 80 °C for seven days. As shown in Fig. S6 (ESI†), the PXRD patterns indicate that the structures of the doubly interpenetrated framework YCZ-1 are partially different from the original PXRD patterns, which may due to the DMA molecules and the H2O molecules in the pores being replaced by other solvent molecules. However, the YCZ-1 framework still displays partial stability, which indicates the application possibility of YCZ-1 in small organic molecule separations.
Gas sorption properties
The permanent microporosity of the activated sample was confirmed by the N2 sorption experiment at 77 K. The N2 sorption isotherm of the desolvated sample shows a typical Type I isotherm with saturation uptake of 578 cm3 g−1 (Fig. 2). The Brunauer–Emmett–Teller (BET) surface area is estimated to be 1589.9 m2 g−1 and the Langmuir surface area is estimated to be 2314.9 m2 g−1. As shown in the insert figure of Fig. 2, the pore size distribution was evaluated though an Ar-sorption experiment and shows that YCZ-1 has pores with a size between 5 Å and 20 Å.
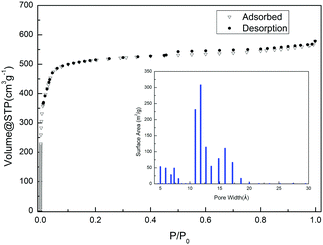 |
| Fig. 2 The N2 sorption isotherms of a YCZ-1 sample at 77 K and 1 bar. Inset figure: Pore size distribution evaluated though an Ar-sorption experiment. | |
Tuning the crystal particle sizes of YCZ-1
Because the dimethylamine, which was generated from DMA dissociation, is an indispensable part of the framework, the speed of fabricating YCZ-1 could be controlled by the speed of the dimethylamine generated. Therefore, the particle size of the YCZ-1 crystal material is controllable by the method of adding different doses of dimethylamine to the original solution. The PXRD patterns of these crystal materials, which were fabricated with various doses of the original dimethylamine, proved that all of them display the same structure (Fig. S7, ESI†). According to the particle size distribution data and optical micrographs of YCZ-1 with various doses of the original dimethylamine, the crystals, which were collected after solvothermal reaction, were smaller but more uniform than the crystals without adding dimethylamine (Fig. 3). Since the porosity of the most common HPLC frits is 2.0 μm, the YCZ-1(1N) crystal particles were the most suitable candidate of the YCZ-1 MOF materials for packing the stationary phase for HPLC separations. Moreover, due to the existence of multisize pores and the adjustability of the crystal size, YCZ-1 could be used as a stationary phase for HPLC separations (Fig. S1, ESI†).
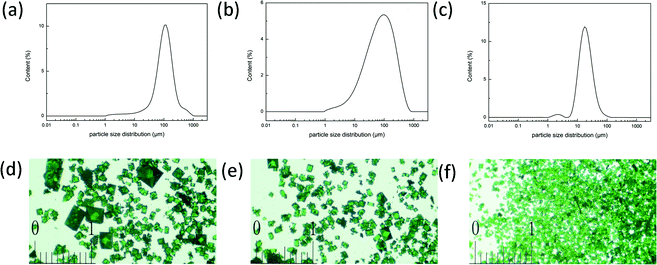 |
| Fig. 3 (a–c) The particle size distribution data of YCZ-1 (a), YCZ-1(0.1N) (b) and YCZ-1(1N) (c); (d–f) the optical micrographs of YCZ-1 (d), YCZ-1(0.1N) (e) and YCZ-1(1N) (f). | |
Small-molecule separations
Dinitrobenzene, an explosive and toxic compound, is accessible by nitration of nitrobenzene.45 Efficient separation of unreacted nitrobenzene from dinitrobenzene at room temperature is crucial and necessary. The separation performance of YCZ-1 towards a mixture of nitrobenzene and dinitrobenzene supplies a new solution to separating them. Liquid-phase separation of a mixture of nitrobenzene and p-dinitrobenzene was performed on the YCZ-1 packed column with hexanes/methanol (96
:
4) as the eluent at a flow rate 0.5 mL min−1. As shown in Fig. 4(a), the retention time for nitrobenzene and p-dinitrobenzene was 1.32 and 7.74 min and the value of resolution (R) is 1.14 (eqn (S1), ESI†). Among the metal–organic framework materials which are utilized in HPLC columns, this is the first reported material that could separate nitrobenzene and dinitrobenzene efficiently with a 30 × 2.1 mm column size.20 The obvious separation may due to the strong size selectivity of the framework.
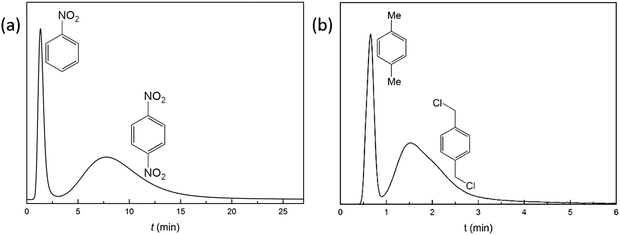 |
| Fig. 4 Liquid-phase separation of (a) a mixture of nitrobenzene and p-dinitrobenzene on the YCZ-1 packed column with hexanes/methanol (96 : 4) as the eluent at a flow rate of 0.5 mL min−1 and (b) a mixture of 1,4-bis(chloromethyl)benzene and para-xylene on the YCZ-1 packed column with hexanes/methanol (98 : 2) as the eluent at a flow rate of 0.5 mL min−1. | |
1,4-Bis(chloromethyl)benzene, an important raw material in the chemical industry, was synthesized by chloridizing para-xylene.44 Efficient separation of unreacted para-xylene from 1,4-bis(chloromethyl)benzene at room temperature would contribute to improving the reaction yield in recycling experiments and reducing the cost of synthesis.46,47 In order to determine the separation ability of YCZ-1 for these compounds, a mixture of 1,4-bis(chloromethyl)benzene and para-xylene was passed through the YCZ-1 packed column with hexanes/methanol (98
:
2) as the eluent. As shown in Fig. 4(b), an obvious baseline separation was obtained. And the retention times for nitrobenzene and toluene are 0.68 and 1.53 min, respectively. Moreover, the resolution (R) between toluene and nitrobenzene is calculated and the value is 0.91. The R value achieved by a 30 × 2.1 mm YCZ-1 packed column reveals the efficient ability of separating 1,4-bis(chloromethyl)benzene and para-xylene by the YCZ-1 material. And this is the first MOF packed column which has been applied in separating 1,4-bis(chloromethyl)benzene and para-xylene.
Selective separation of the small organic molecule isomers is one of the keys to analysis of the synthesis reaction process.15,16,48 Both dinitrotoluene and xylene have ortho-, meta- and para-isomers. Using the same eluent (hexanes/methanol = 95
:
5) and flow rate (0.5 mL min−1), the retention times for meta-, ortho- and para-dinitrotoluene are 3.40 min, 5.67 min and 7.36 min, respectively (Fig. 5). This example demonstrates the potential of the crystalline material YCZ-1 for dinitrotoluene isomer separation. But under the same conditions, the retention times for meta-, ortho- and para-xylene are 0.64 min, 0.67 min and 0.69 min, respectively. The separated ability of YCZ-1 for xylene isomers is limited. The difference in retention times between the isomeric dinitrotoluene and isomeric xylene indicates that, in addition to the size of the molecules, other factors, like the purely electronic nature of the adsorbate and charge distribution, influence the separation of the YCZ-1 packed column.
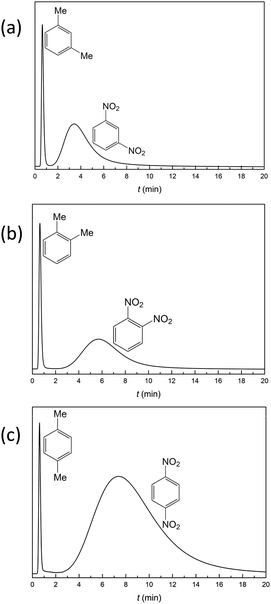 |
| Fig. 5 Liquid-phase separation on the YCZ-1 packed column with hexanes/methanol (95 : 5) as the eluent at a flow rate of 0.5 mL min−1: (a) mixture of m-dimethylbenzene and m-dinitrobenzene; (b) mixture of o-dimethylbenzene and o-dinitrobenzene; (c) mixture of p-dimethylbenzene and p-dinitrobenzene. | |
Conclusions
In conclusion, we report a stable porous metal–organic framework YCZ-1 which was fabricated with a new carbazole-based linker, 3,6-bis(4-benzoic acid)-N-(4-benzoic acid)carbazole. Porous crystalline material YCZ-1 has excellent structural stability, special pore sizes and outstanding surface area. Meanwhile, the particle sizes of YCZ-1 could be controlled to fit the porosity of the frits in HPLC. Therefore, crystalline material YCZ-1 could be utilized as the stationary phase for HPLC separations during the separation of small organic molecules. This work opens a new opportunity to fabricate porous metal–organic frameworks and provides a novel strategy to extend MOFs in separation applications as crystalline materials.
Conflicts of interest
There are no conflicts to declare.
Acknowledgements
The authors gratefully acknowledge financial support from the National Natural Science Foundation of China (21233009 and 21203194), the 973 program (2014CB845603), and the strategic Priority Research Program of the Chinese Academy of Sciences, Grant No. XDB20000000.
References
- L. E. Kreno, K. Leong, O. K. Farha, M. Allendorf, R. P. Van Duyne and J. T. Hupp, Chem. Rev., 2012, 112, 1105 CrossRef PubMed
.
- X. Li, Y. Liu, J. Wang, J. Gascon and J. Li, Chem. Soc. Rev., 2017, 46, 7124 RSC
.
- S. Krause, V. Bon, I. Senkovska, U. Stoeck, D. Wallacher, D. M. Többens, S. Zander, R. S. Pillai, G. Maurin, F.-X. Coudert and S. Kaskel, Nature, 2016, 532, 348 CrossRef PubMed
.
- Y. Inokuma, S. Yoshioka, J. Ariyoshi, T. Arai, Y. Hitora, K. Takada, S. Matsunaga, K. Rissanen and M. Fujita, Nature, 2013, 495, 461 CrossRef PubMed
.
- S. S. Park, Y. Tulchinsky and M. Dinca, J. Am. Chem. Soc., 2017, 139, 13260 CrossRef PubMed
.
- Z. Wang, A. Knebel, S. Grosjean, D. Wagner, S. Brase, C. Woell, J. Caro and L. Heinke, Nat. Commun., 2016, 7, 13872 CrossRef PubMed
.
- Y. Ye, S. Xiong, X. Wu, L. Zhang, Z. Li, L. Wang, X. Ma, Q. H. Chen, Z. Zhang and S. Xiang, Inorg. Chem., 2016, 55, 292 CrossRef PubMed
.
- Z. B. Wang, J. X. Liu, H. K. Arslan, S. Grosjean, T. Hagendorn, H. Gliemann, S. Brase and C. Woell, Langmuir, 2013, 29, 15958 CrossRef PubMed
.
- Y. Guo, X. Feng, T. Han, S. Wang, Z. Lin, Y. Dong and B. Wang, J. Am. Chem. Soc., 2014, 136, 15485 CrossRef PubMed
.
- X. Li, L. Yang, L. Zhao, X.-L. Wang, K.-Z. Shao and Z.-M. Su, Cryst. Growth Des., 2016, 16, 4374 Search PubMed
.
- Y. Ye, L. Zhang, Q. Peng, G. E. Wang, Y. Shen, Z. Li, L. Wang, X. Ma, Q. H. Chen, Z. Zhang and S. Xiang, J. Am. Chem. Soc., 2015, 137, 913 CrossRef PubMed
.
- L. Liang, C. Liu, F. Jiang, Q. Chen, L. Zhang, H. Xue, H. L. Jiang, J. Qian, D. Yuan and M. Hong, Nat. Commun., 2017, 8, 1233 CrossRef PubMed
.
- Y. He, B. Li, M. O'Keeffe and B. Chen, Chem. Soc. Rev., 2014, 43, 5618 RSC
.
- K. J. Hartlieb, J. M. Holcroft, P. Z. Moghadam, N. A. Vermeulen, M. M. Algaradah, M. S. Nassar, Y. Y. Botros, R. Q. Snurr and J. F. Stoddart, J. Am. Chem. Soc., 2016, 138, 2292 CrossRef PubMed
.
- R. El Osta, A. Carlin-Sinclair, N. Guillou, R. I. Walton, F. Vermoortele, M. Maes, D. de Vos and F. Millange, Chem. Mater., 2012, 24, 2781 CrossRef
.
- A. L. Nuzhdin, D. N. Dybtsev, K. P. Bryliakov, E. P. Talsi and V. P. Fedin, J. Am. Chem. Soc., 2007, 129, 12958 CrossRef PubMed
.
- E. Zhang, F. Wang, W. Zhai, K. Scott, X. Wang and G. Diao, Bioresour. Technol., 2017, 229, 111 CrossRef PubMed
.
- M. A. Quiroz, J. L. Sanchez-Salas, S. Reyna, E. R. Bandala, J. M. Peralta-Hernandez and C. A. Martinez-Huitle, J. Hazard. Mater., 2014, 268, 6 CrossRef PubMed
.
- Y. Y. Fu, C. X. Yang and X. P. Yan, Langmuir, 2012, 28, 6794 CrossRef PubMed
.
- L. D. Tran, J. Ma, A. G. Wong-Foy and A. J. Matzger, Chem. – Eur. J., 2016, 22, 5509 CrossRef PubMed
.
- J. H. Zhang, R. Y. Nong, S. M. Xie, B. J. Wang, P. Ai and L. M. Yuan, Electrophoresis, 2017, 38, 2513 CrossRef PubMed
.
- D. D. Zheng, L. Wang, T. Yang, Y. Zhang, Q. Wang, M. Kurmoo and M. H. Zeng, Inorg. Chem., 2017, 56, 11043 CrossRef PubMed
.
- A. Ahmed, K. Skinley, S. Herodotou and H. F. Zhang, J. Sep. Sci., 2018, 41, 99 CrossRef PubMed
.
- L. Xia, L. J. Liu, X. X. Lv, F. Qu, G. L. Li and J. M. You, J. Chromatogr. A, 2017, 1500, 24 CrossRef PubMed
.
- H. Bunzen, M. Grzywa, M. Hambach, S. Spirkl and D. Volkmer, Cryst. Growth Des., 2016, 16, 3190 Search PubMed
.
- Q. L. Zhu, N. Tsumori and Q. Xu, J. Am. Chem. Soc., 2015, 137, 11743 CrossRef PubMed
.
- Q.-L. Zhu, J. Li and Q. Xu, J. Am. Chem. Soc., 2013, 135, 10210 CrossRef PubMed
.
- Y. Pan, D. Heryadi, F. Zhou, L. Zhao, G. Lestari, H. Su and Z. Lai, CrystEngComm, 2011, 13, 6937 RSC
.
- C. H. Kuo, Y. Tang, L. Y. Chou, B. T. Sneed, C. N. Brodsky, Z. Zhao and C. K. Tsung, J. Am. Chem. Soc., 2012, 134, 14345 CrossRef PubMed
.
- X. Duan, C. Wu, S. Xiang, W. Zhou, T. Yildirim, Y. Cui, Y. Yang, B. Chen and G. Qian, Inorg. Chem., 2015, 54, 4377 CrossRef PubMed
.
- P. P. Bag, D. Wang, Z. Chen and R. Cao, Chem. Commun., 2016, 52, 3669 RSC
.
- L. Kong, R. Zou, W. Bi, R. Zhong, W. Mu, J. Liu, R. P. S. Han and R. J. Zou, J. Mater. Chem. A, 2014, 2, 17771 Search PubMed
.
- N. Xie, J. Wang, Z. Guo, G. Chen and Q. Li, Chem. Phys., 2013, 214, 1710 Search PubMed
.
- H. J. Cheng, H. X. Tang, Y. L. Shen, N. N. Xia, W. Y. Yin, W. Zhu, X. Y. Tang, Y. S. Ma and R. X. Yuan, J. Solid State Chem., 2015, 232, 200 CrossRef
.
- D.-H. Chen, L. Lin, T.-L. Sheng, Y.-H. Wen, S.-M. Hu, R.-B. Fu, C. Zhuo, H.-R. Li and X.-T. Wu, CrystEngComm, 2017, 19, 2632 RSC
.
- M. Eddaoudi, Ł. Weseliński and R. Luebke, Synthesis, 2014, 596 CrossRef
.
- D.-H. Chen, L. Lin, T.-L. Sheng, Y.-H. Wen, X.-Q. Zhu, L.-T. Zhang, S.-M. Hu, R.-B. Fu and X.-T. Wu, New J. Chem., 2018, 42, 2830 RSC
.
-
G. M. Shedrick, SHELXL-97, program for Refining Crystal Structure Refinement, University of Göttingen, Germany, 1997 Search PubMed
.
- G. M. Sheldrick, Acta Crystallogr., 2015, 71, 3 CrossRef PubMed
.
-
A. L. Spek, PLATON, Amultipurpose crystallographic tool, Utrecht University, Utrecht, The Netherlands, 2001 Search PubMed
.
- A. L. Spek, Acta Crystallogr., 2009, 65, 148 CrossRef PubMed
.
- Y. Wang, C. Tan, Z. Sun, Z. Xue, Q. Zhu, C. Shen, Y. Wen, S. Hu, Y. Wang, T. Sheng and X. Wu, Chem. – Eur. J., 2014, 20, 1341 CrossRef PubMed
.
- O. H. Bner, A. Glçss, M. Fichtner and W. Klopper, J. Phys. Chem. A, 2004, 108, 3019 CrossRef
.
- V. A. Blatov, Struct. Chem., 2012, 23, 955 CrossRef
.
-
J. Buddrus, Grundlagen der organischen Chemie, de Gruyter, Berlin, 4th edn, 2011, vol. 13, p. 363 Search PubMed
.
- S. H. Combe, A. Hosseini, A. Parra and P. R. Schreiner, J. Org. Chem., 2017, 82, 2407 CrossRef PubMed
.
- G. Xie, Q. Zheng, C. Huang and Y. Chen, Synth. Commun., 2003, 33, 1103 CrossRef
.
- V. Percec, W.-D. Cho, G. Ungar and D. J. P. Yeardley, J. Am. Chem. Soc., 2001, 123, 1302 CrossRef
.
Footnote |
† Electronic supplementary information (ESI) available: Selected bond lengths and angles, PXRD curve, TGA curve, and IR spectra. CCDC 1827217 (YCZ-1). For ESI and crystallographic data in CIF or other electronic format see DOI: 10.1039/c8qm00147b |
|
This journal is © the Partner Organisations 2018 |
Click here to see how this site uses Cookies. View our privacy policy here.