DOI:
10.1039/D1QO00478F
(Research Article)
Org. Chem. Front., 2021,
8, 3668-3674
Cucurbitimines – imine cages with concave walls†
Received
30th March 2021
, Accepted 29th April 2021
First published on 17th May 2021
Abstract
The variety of shape-persistent organic cages by imine bond formation has tremendously enlarged in recent years by using different building blocks (aldehydes and amines) in the condensation reactions. Here, we describe the use of a kinked tetraldehyde to generate pumpkin-shaped cages with concave walls, similar to cucurbiturils.
Introduction
The synthesis of shape-persistent organic cage compounds, applying dynamic covalent chemistry (DCC) has developed in recent years tremendously fast and enabled the access of a large variety of defined molecular structures of different sizes, geometries and functions.1–6 Although a few examples of boronic ester cages, or those based on disulphide formation or other DCC reactions are known,7–18,19–21,22–26 no doubt, the vast majority of shape-persistent organic cages is relying on the use of multiple imine condensation reactions,1 which bear the advantage to be transformed further to convert these to chemically robust cages,27–31 which is for the other type of DCC reactions difficult or even impossible.32 Among those imine cages, shapes, such as trigonal prisms,33–35 tetrahedra,36–39 truncated tetrahedra,40,41 cubes,42–46 tetrapods,47 adamantoids48–50 and even more complex structures51–57 have been realized as well as tubular structures, based on linear tetraaldehydes.58–61,62
Here we introduce [3 + 6] imine cages based on the condensation of triptycene tetraaldehydes with 1,2-cyclohexyldiamines. Due to the inherent 120° angle of the triptycene blades, the resulting imine cage contains concave π-walls giving the overall structure the shape comparable to those of cucurbiturils,63–67 thus we call the compounds cucurbitimines (Fig. 1).
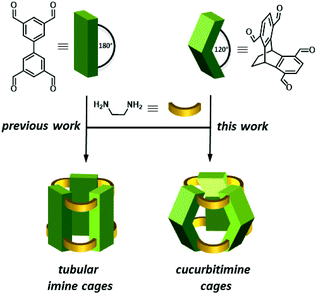 |
| Fig. 1 Schematic representation of literature-known tubular [3 + 6] imine cages (left)58–61 and the cucurbitimines presented herein. Please note that any further functional groups or substituents present in the molecular structures have been omitted for clarity. | |
Results and discussion
A key-step in the achievement of cucurbitimines is the synthesis of a triptycene-based tetraaldehyde precursor, having on two aromatic blades two aldehyde units each and at the third one none. Therefore, we developed a route where electron density of one blade was diminished by turning it into a phenazine unit and in addition allowed us to install long solubilizing hexyl-sidechains (Scheme 1). To achieve this first synthetic goal, hexamethoxytriptycene 1 was partially oxidized to the quinone 268–70 and condensed with phenylene diamine 371 to give phenazine 4 in 79% yield.72 The next step is the introduction of the aldehyde groups. Finally, the tetraaldehyde precursor was achieved by a three-step-method, which was based on the fourfold chloromethylation to 5 (60% yield), followed by basic substitution of chlorine by hydroxyl groups to give tetra benzylic alcohol 6 in 77% yield, which was then oxidized with pyridinium chloro chromate (PCC) to give tetraaldehyde 7 in 85% yield. All compounds have been fully characterized, including single crystal structure analysis by X-ray diffraction for 4 and 5 (for details, see ESI†).
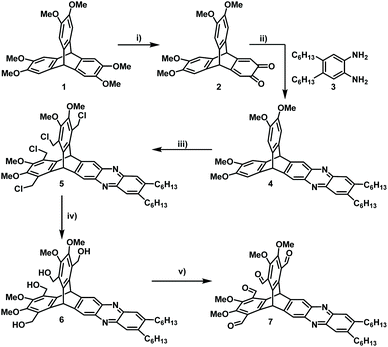 |
| Scheme 1 Synthesis of tetraaldehyde 7. (i) conc. HNO3, DCM, AcOH, 5 min, 91%; (ii) CHCl3, AcOH, 80 °C, 7 h, 79%; (iii) ClCH2OCH3, CH3SO3H, 80 °C, 16 h, 60%; (iv) Cs2CO3, dioxane/water (1 : 1 v/v), 140 °C, 3d, 77%; (v) PCC, Celite, DCM, r.t., 6 h, 85%. | |
With tetraaldehyde 7 in hand, condensation reactions with (R,R)-1,2-cyclohexyldiamine 8 were investigated under various conditions (Scheme 2). Solvents, such as MeOH, MeCN, DCM, THF, toluene or CHCl3 were used and the reactants with the addition of a small amount of TFA (2 mol%) were stirred at room temperature or under reflux. Although in all cases the [3 + 6] cucurbitimine cage was formed, as detected by MALDI-TOF mass spectrometry (m/z = 2739), the corresponding 1H NMR spectra revealed that only in DCM the purity of the crude material was high enough to allow isolation and purification of the cage 9 in 10% yield. However, the best results were achieved by layering solutions of the two reactants and let them diffuse into each other, which has been widely used by Cooper et al.37,73,74 Although in contrast to their cages, which usually crystallized during formation, here the reaction mixture still kept a clear solution. Nevertheless, by this method we were able to isolate cucurbitimine cage (R,R)-9 in 49% yield by applying separation with recycling-GPC (gel permeation chromatography,) besides 5% of the larger [4 + 8] cage (R,R)-10 (m/z = 3653). Using the enantiomeric (S,S)-1,2-cyclohexyldiamine gave basically the same results and here the cages (S,S)-9 and (S,S)-10 have been isolated in 54% and 5% yield (Fig. 2). It is worth to mention, that the calculated heats of formation (PM3) for the trimeric cage 9 is ΔHf = 751 kJ mol−1 (250.3 kJ mol per unit) and for tetrameric cage 10 ΔHf = 975 kJ mol−1 (243.3 kJ mol per unit) are very similar, if compared per unit (ΔΔHf = 7 kJ mol−1). Thus a more equal distribution would be excepted, if the cage products are in thermodynamically equilibrium. Since it is not the case in combination with all the observations made under various reaction conditions, it is concluded, that the cages 9 are rather kinetically controlled products than thermodynamically ones.
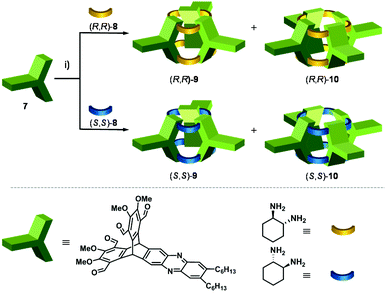 |
| Scheme 2 Synthesis of cucurbitimines. (i) DCM, 2 mol% TFA, r.t., 7d, yields: (R,R)-9 (49%); (R,R)-10 (5%); (S,S)-9 (54%); (S,S)-10 (5%). | |
 |
| Fig. 2 Recycling-GPC chromatogram of the reaction of 7 with (S,S)-8. For experimental details, see ESI.† Please note that in between the peak at 150 min and 160 min, the compounds responsible for the broad peak were removed. | |
In the 1H NMR spectra of enantiopure cages 9, the most characteristic peaks are found in the aromatic region (Fig. 3). Whereas triptycene bridgehead protons overlap with the outer phenazine protons (7.84 ppm), the inner ones resonate at 7.67 ppm. The imine protons show a large difference in chemical shifts. The peak at 9.26 ppm are assigned to the imine protons closer to the triptycene bridgeheads, the peak at 8.70 ppm corresponds to the imine protons pointing towards the outer rim of the cage. The larger cages 10 are unfortunately not stable enough to be fully purified by GPC or other means and still contain some minor impurities, as detected by 1H NMR spectroscopy (see ESI†). By DOSY experiments, diffusion coefficients of D = 4.3 × 10−10 m2 s−1 for the cages 9 were determined, which corresponds to a solvodynamic radius of rs = 1.1 nm and fits well to the outer diameter of the molecule found by single-crystal X-ray diffraction (vide infra). For [4 + 8] cage 10 a diffusion coefficient of D = 3.4 × 10−10 m2 s−1 was measured, thus the solvodynamic radius was a bit larger (rs = 1.4 nm) and fits to the radius of the molecular model (see below).
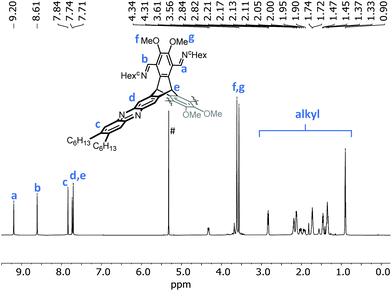 |
| Fig. 3
1H NMR spectrum (700 MHz, CD2Cl2) of cage (R,R)-9. # residual CH2Cl2. | |
For cage (R,R)-9 single-crystals for X-ray diffraction were grown from diffusion of n-pentane into a saturated solution of the cage in DCM (Fig. 4). The compound crystallizes in the orthorhombic space group P21212 and contained one enclathrated DCM molecule inside the cavity and disordered electron density (according to 8 DCM molecules per asymmetric unit) inside the formed extrinsic channels (Fig. 4d and e). The cages pack window-to-window with a closest distance of two methoxy units of d(C⋯OMe) = 3.47 Å (Fig. 4b), which is a hint for some week dipole–dipole interactions (Keesom interactions) as found before for methoxy-substituted π-extended heteroarenes.75 Due to the relative small window, pores are isolated, e.g. for gases with kinetic diameters >2.2 Å, if one assumes a static structure in the crystals.76 By the second dominant packing motif (CH⋯π interactions) between the cyclohexyl moieties and the aromatic phenazine backbones (Fig. 4c) extrinsic one-dimensional channels are formed containing disordered solvate (DCM) molecules (Fig. 4d and e). Up to now, these have not been tried to be activated for gas sorption, because we are more interested in the molecular structure as defined host for supramolecular host-guest chemistry.
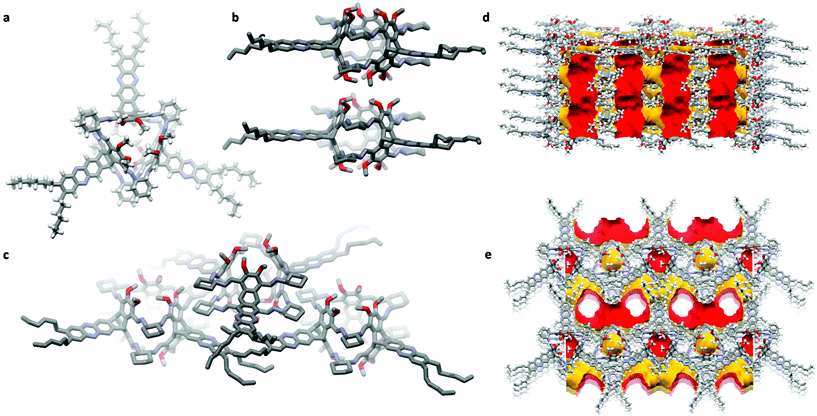 |
| Fig. 4 Single crystal X-ray structure of cage (R,R)-9. (a) single molecule from top; (b) window-to-window packing; (c) packing by CH-π interactions of aromatic phenazine units and aliphatic cyclohexyl ring; (d) and (e) calculated pores with a probe radius of 1.82 Å. For (b) and (c) hydrogen atoms have been omitted for clarity. In (d) an (e) the red surface is the solvent accessible surface and yellow the inaccessible surface. | |
It is worth to compare the molecular dimensions of the cavity of the cucurbitimines 9 with the cucurbiturils (Fig. 5). The distance between two oxygens of adjacent veratrole units is between 3.8 and 4.6 Å. The distance of two adjacent triptycene bridgehead carbons is 7.6 Å. The height of the molecule (distance between two eclipsed oxygens of the upper and the lower rim) is between 9.0 and 9.2 Å. By applying the SwissPDB viewer77 a pore volume of 177 Å3 was calculated. Most interestingly, these dimensions are somewhat larger than the 115 Å3 we calculated for cucurbit[6]uril (CB6) with the same program, but comparable to those reported in literature (164 Å3).65 In CB6 the diameter of two opposite oxygens at the rims is 3.9 Å, the inner distance of two opposite carbons is with 5.8 Å smaller than in cage 9 and the height is very similar (9.1 Å).
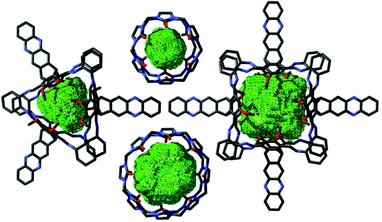 |
| Fig. 5 Comparison of molecular pore volumes of cucurbitimines (R,R)-9 (left) and (R,R)-10 (right) with those of CB6 (top middle) and CB8 (bottom middle)., generated with SwissPDBviewer.77 For details, see ESI.† | |
For the [4 + 8] cages 10 we were not successful to grow suitable crystals for a detailed analysis by X-ray diffraction. However, cage 9 is very rigid and thus shape-persistent and the experimental structure determined by X-ray diffraction fits nearly perfectly to the calculated model (PM6). Thus we take the PM6 model of the larger cage 10 for comparison with cucurbiturils. As in cucurbiturils the height is the same for all members of the family, the height is here also the same for cage 10 and cage 9 (9.1 Å). Due to the higher symmetry, the distance between two opposite oxygens at one rim can be measured. With 9.0 Å it is significantly larger than e.g. for cucurbituril[8] CB8 (6.9 Å). The inner diameter (distance between two opposite triptycene bridgehead carbons) is 12.0 Å. The calculated void volume is with 368 Å3 similar to what we estimated for CB8 with the PDB viewer (351 Å3) but substantially smaller than reported in literature (479 Å3).65 Furthermore we calculated the electron density distribution, which is similar to curcurbiturils, but less pronounced (see ESI†), so the curcubitimines may show a similar host–guest behaviour as the cucurbiturils.65,66,78,79 Whereas all initial tests to incorporate ammonium compounds into the cavity did not show any uptake, it was realized that neutral molecules are found in the molecular void. As described above, by X-ray crystallography one DCM molecule was found in the interior of cage 9, and as soon as it is purified by recycling-GPC with THF as eluent, one molecule of THF is bound inside, when analysed by 1H NMR in toluene-d8 (Fig. 6). The peak at −0.84 ppm is clearly assigned to the eight THF protons of THF inside the cavity. The corresponding peaks of (R,R)-9 of the host–guest complex are highlighted in blue. In Fig. 6 (top spectrum) already a second set of cage protons are detected (magenta colored peaks), that are shifted all slightly upfield. By time these increase in intensity (Fig. 6, bottom, whereas those of the THF@(R,R)-9 complex decrease. Besides that, free THF is found (1.45 and 3.56 ppm), thus we conclude that THF most likely got exchanged by toluene-d8. The exchange is very slow at room temperature (more than 2 d) but can be accelerated to less than 1 h at 100 °C.
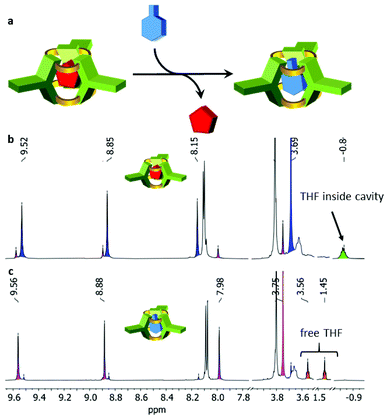 |
| Fig. 6 (a) Exchange of host molecules from THF@(R,R)-9 to toluene-d8@(R,R)-9. (b) 1H NMR spectrum (700 MHz) of freshly prepared THF@(R,R)-9 in toluene-d8. (c) same sample after exchange of THF guest by toluene-d8. Note, that in the upper spectrum some toluene-d8@(R,R)-9 has formed. | |
Conclusions
Based on a triptycene tetraaldehyde a new type of imine cages has been introduced that have a similar shape than cucurbiturils. Therefore, these imine cages are named cucurbitimines. Initial studies demonstrate, that small neutral guests are bound inside the cavity and that their exchange (here THF by toluene) is kinetically suppressed due to the small window sizes of the opening. By increasing the temperature, the exchange of guest molecules can be accelerated. This behaviour is quite similar to that of the cucurbiturils. In contrast to those, the here discussed cucurbitimines are much better soluble in organic solvents and, which for cucubiturils is quite rare,80 are chiral, opening up new possibilities for classical host–guest chemistry, but also for separation of volatile chiral compounds.81 Furthermore, we will look for possibilities to get the larger members of the family ([5 + 10] and higher) to open up new vistas in supramolecular chemistry simply by enlarging the windows – as has been done before for cucurbiturils.
Conflicts of interest
There are no conflicts to declare.
Acknowledgements
We thank the European Research Council ERC in the frame of the consolidators grant CaTs n DOCs (grant no. 725765). Jochen C. Lauer and Tobias Kirschbaum (both Ruprecht-Karls-Universität Heidelberg) are acknowledged for their helpful discussions.
References
- N. M. Rue, J. Sun and R. Warmuth, Polyimine Container Molecules and Nanocapsules, Isr. J. Chem., 2011, 51, 743–768 Search PubMed.
- V. Santolini, M. Miklitz, E. Berardo and K. E. Jelfs, Topological landscapes of porous organic cages, Nanoscale, 2017, 9, 5280–5298 Search PubMed.
- M. Mastalerz, Porous Shape-Persistent Organic Cage Compounds of Different Size, Geometry, and Function, ACC. Chem. Res., 2018, 51, 2411–2422 Search PubMed.
- K. Acharyya and P. S. Mukherjee, Organic Imine Cages: Molecular Marriage and Applications, Angew. Chem., Int. Ed., 2019, 58, 8640–8653 Search PubMed.
- M. A. Little and A. I. Cooper, The Chemistry of Porous Organic Molecular Materials, Adv. Funct. Mater., 2020, 30, 1909842 Search PubMed.
- F. Beuerle and B. Gole, Covalent Organic Frameworks and Cage Compounds: Design and Applications of Polymeric and Discrete Organic Scaffolds, Angew. Chem., Int. Ed., 2018, 57, 4850–4878 Search PubMed.
- M. Mastalerz, Shape-Persistent Organic Cage Compounds by Dynamic Covalent Bond Formation, Angew. Chem., Int. Ed., 2010, 49, 5042–5053 Search PubMed.
- N. Christinat, R. Scopelliti and K. Severin, Multicomponent Assembly of Boronic Acid Based Macrocycles and Cages, Angew. Chem., 2008, 120, 1874–1878 Search PubMed.
- M. Hutin, G. Bernardinelli and J. R. Nitschke, An Iminoboronate Construction Set for Subcomponent Self-Assembly, Chem. – Eur. J., 2008, 14, 4585–4593 Search PubMed.
- N. Nishimura and K. Kobayashi, Self-Assembly of a Cavitand-Based Capsule by Dynamic Boronic Ester Formation, Angew. Chem., Int. Ed., 2008, 47, 6255–6258 Search PubMed.
- A. Granzhan, C. Schouwey, T. Riis-Johannessen, R. Scopelliti and K. Severin, Connection of Metallamacrocycles via Dynamic Covalent Chemistry: A Versatile Method for the Synthesis of Molecular Cages, J. Am. Chem. Soc., 2011, 133, 7106–7115 Search PubMed.
- J. Tönnemann, R. Scopelliti, K. O. Zhurov, L. Menin, S. Dehnen and K. Severin, Borophosphonate Cages: Easily Accessible and Constitutionally Dynamic Heterocubane Scaffolds, Chem. – Eur. J., 2012, 18, 9939–9945 Search PubMed.
- K. Ono, K. Johmoto, N. Yasuda, H. Uekusa, S. Fujii, M. Kiguchi and N. Iwasawa, Self-Assembly of Nanometer-Sized Boroxine Cages from Diboronic Acids, J. Am. Chem. Soc., 2015, 137, 7015–7018 Search PubMed.
- S. Klotzbach and F. Beuerle, Shape-Controlled Synthesis and Self-Sorting of Covalent Organic Cage Compounds, Angew. Chem., Int. Ed., 2015, 54, 10356–10360 Search PubMed.
- N. Schäfer, M. Bühler, L. Heyer, M. I. S. Röhr and F. Beuerle, Endohedral Hydrogen Bonding Templates the Formation of a Highly Strained Covalent Organic Cage Compound, Chem. – Eur. J., 2021, 27, 6077–6085 Search PubMed.
- S. Klotzbach, T. Scherpf and F. Beuerle, Dynamic covalent assembly of tribenzotriquinacenes into molecular cubes, Chem. Commun., 2014, 50, 12454–12457 Search PubMed.
- G. Zhang, O. Presly, F. White, I. M. Oppel and M. Mastalerz, A Permanent Mesoporous Organic Cage with an Exceptionally High Surface Area, Angew. Chem., Int. Ed., 2014, 53, 1516–1520 Search PubMed.
- S. M. Elbert, N. I. Regenauer, D. Schindler, W.-S. Zhang, F. Rominger, R. R. Schröder and M. Mastalerz, Shape-Persistent Tetrahedral [4+6] Boronic Ester Cages with Different Degrees of Fluoride Substitution, Chem. – Eur. J., 2018, 24, 11438–11443 Search PubMed.
- S.-W. Tam-Chang, J. S. Stehouwer and J. Hao, Formation of a Macrobicyclic Tris(disulfide) by Molecular Self-Assembly, J. Org. Chem., 1999, 64, 334–335 Search PubMed.
- K. R. West, K. D. Bake and S. Otto, Dynamic Combinatorial Libraries of Disulfide Cages in Water, Org. Lett., 2005, 7, 2615–2618 Search PubMed.
- J. Shin and K. Paek, Bull. Korean Chem. Soc., 2014, 35, 2205–2206 Search PubMed.
- C. Zhang, Q. Wang, H. Long and W. Zhang, A Highly C70 Selective Shape-Persistent Rectangular Prism Constructed through One-Step Alkyne Metathesis, J. Am. Chem. Soc., 2011, 133, 20995–21001 Search PubMed.
- Q. Wang, C. Zhang, B. C. Noll, H. Long, Y. Jin and W. Zhang, A Tetrameric Cage with D2 h Symmetry through Alkyne Metathesis, Angew. Chem., Int. Ed., 2014, 53, 10663–10667 Search PubMed.
- S. Lee, A. Yang, T. P. Moneypenny and J. S. Moore, Kinetically Trapped Tetrahedral Cages via Alkyne Metathesis, J. Am. Chem. Soc., 2016, 138, 2182–2185 Search PubMed.
- R.-C. Brachvogel, F. Hampel and M. von Delius, Self-assembly of dynamic orthoester cryptates, Nat. Commun., 2015, 6, 7129 Search PubMed.
- O. Shyshov, R.-C. Brachvogel, T. Bachmann, R. Srikantharajah, D. Segets, F. Hampel, R. Puchta and M. von Delius, Adaptive Behavior of Dynamic Orthoester Cryptands, Angew. Chem., Int. Ed., 2017, 56, 776–781 Search PubMed.
- M. Liu, M. A. Little, K. E. Jelfs, J. T. A. Jones, M. Schmidtmann, S. Y. Chong, T. Hasell and A. I. Cooper, Acid- and Base-Stable Porous Organic Cages: Shape Persistence and pH Stability via Post-synthetic “Tying” of a Flexible Amine Cage, J. Am. Chem. Soc., 2014, 136, 7583–7586 Search PubMed.
- X.-Y. Hu, W.-S. Zhang, F. Rominger, I. Wacker, R. R. Schröder and M. Mastalerz, Transforming a chemically labile [2+3] imine cage into a robust carbamate cage, Chem. Commun., 2017, 53, 8616–8619 Search PubMed.
- A. S. Bhat, S. M. Elbert, W.-S. Zhang, F. Rominger, M. Dieckmann, R. R. Schröder and M. Mastalerz, Transformation of a [4+6] Salicylbisimine Cage to Chemically Robust Amide Cages, Angew. Chem., Int. Ed., 2019, 58, 8819–8823 Search PubMed.
- T. H. G. Schick, J. C. Lauer, F. Rominger and M. Mastalerz, Transformation of Imine Cages into Hydrocarbon Cages, Angew. Chem., Int. Ed., 2019, 58, 1768–1773 Search PubMed.
- P.-E. Alexandre, W.-S. Zhang, F. Rominger, S. M. Elbert, R. R. Schröder and M. Mastalerz, A Robust Porous Quinoline Cage: Transformation of a [4+6] Salicylimine Cage by Povarov Cyclization, Angew. Chem., Int. Ed., 2020, 59, 19675–19679 Search PubMed.
- M. Hähsler and M. Mastalerz, A Giant [8+12] Boronic Ester Cage with 48 Terminal Alkene Units in the Periphery for Postsynthetic Alkene Metathesis, Chem. – Eur. J., 2021, 27, 233–237 Search PubMed.
- S. Jiang, J. Bacsa, X. Wu, J. T. A. Jones, R. Dawson, A. Trewin, D. J. Adams and A. I. Cooper, Selective gas sorption in a [2+3] ‘propeller’ cage crystal, Chem. Commun., 2011, 47, 8919–8921 Search PubMed.
- M. W. Schneider, I. M. Oppel and M. Mastalerz, Exo-Functionalized Shape-Persistent [2+3] Cage Compounds: Influence of Molecular Rigidity on Formation and Permanent Porosity, Chem. – Eur. J., 2012, 18, 4156–4160 Search PubMed.
- K. Acharyya and P. S. Mukherjee, Hydrogen-Bond-Driven Controlled Molecular Marriage in Covalent Cages, Chem. – Eur. J., 2014, 20, 1646–1657 Search PubMed.
- P. Skowronek and J. Gawronski, Chiral Iminospherand of a Tetrahedral Symmetry Spontaneously Assembled in a [6+4] Cyclocondensation, Org. Lett., 2008, 10, 4755–4758 Search PubMed.
- T. Tozawa, J. T. A. Jones, S. I. Swamy, S. Jiang, D. J. Adams, S. Shakespeare, R. Clowes, D. Bradshaw, T. Hasell, S. Y. Chong, C. Tang, S. Thompson, J. Parker, A. Trewin, J. Bacsa, A. M. Z. Slawin, A. Steiner and A. I. Cooper, Porous organic cages, Nat. Mater., 2009, 8, 973–978 Search PubMed.
- N. Cao, Y. Wang, X. Zheng, T. Jiao and H. Li, Controllable Self-Assembly of Pills and Cages via Imine Condensation for Silver Cation Detection, Org. Lett., 2018, 20, 7447–7450 Search PubMed.
- T. Jiao, L. Chen, D. Yang, X. Li, G. Wu, P. Zeng, A. Zhou, Q. Yin, Y. Pan, B. Wu, X. Hong, X. Kong, V. M. Lynch, J. L. Sessler and H. Li, Trapping White Phosphorus within a Purely Organic Molecular Container Produced by Imine Condensation, Angew. Chem., Int. Ed., 2017, 56, 14545–14550 Search PubMed.
- J. C. Lauer, W.-S. Zhang, F. Rominger, R. R. Schröder and M. Mastalerz, Shape-Persistent [4+4] Imine Cages with a Truncated Tetrahedral Geometry, Chem. – Eur. J., 2018, 24, 1816–1820 Search PubMed.
- T. Kunde, E. Nieland, H. V. Schröder, C. A. Schalley and B. M. Schmidt, A porous fluorinated organic [4+4] imine cage showing CO2 and H2 adsorption, Chem. Commun., 2020, 56, 4761–4764 Search PubMed.
- S. Hong, M. R. Rohman, J. Jia, Y. Kim, D. Moon, Y. Kim, Y. H. Ko, E. Lee and K. Kim, Porphyrin Boxes: Rationally Designed Porous Organic Cages, Angew. Chem., Int. Ed., 2015, 54, 13241–13244 Search PubMed.
- R. D. Mukhopadhyay, Y. Kim, J. Koo and K. Kim, Porphyrin Boxes, Acc. Chem. Res., 2018, 51, 2730–2738 Search PubMed.
- P. Wagner, F. Rominger, W.-S. Zhang, J. H. Gross, S. M. Elbert, R. R. Schröder and M. Mastalerz, Chiral Self-sorting of Giant Cubic [8+12] Salicylimine Cage Compounds, Angew. Chem., Int. Ed., 2021, 60, 8896–8904 Search PubMed.
- D. Xu and R. Warmuth, Edge-Directed Dynamic Covalent Synthesis of a Chiral Nanocube, J. Am. Chem. Soc., 2008, 130, 7520–7521 Search PubMed.
- H. Qu, Y. Wang, Z. Li, X. Wang, H. Fang, Z. Tian and X. Cao, Molecular Face-Rotating Cube with Emergent Chiral and Fluorescence Properties, J. Am. Chem. Soc., 2017, 139, 18142–18145 Search PubMed.
- M. E. Briggs, K. E. Jelfs, S. Y. Chong, C. Lester, M. Schmidtmann, D. J. Adams and A. I. Cooper, Shape Prediction for Supramolecular Organic Nanostructures: [4+4] Macrocyclic Tetrapods, Cryt. Growth Des., 2013, 13, 4993–5000 Search PubMed.
- M. Mastalerz, One-pot synthesis of a shape-persistent endo-functionalised nano-sized adamantoid compound, Chem. Commun., 2008, 4756–4758, 10.1039/B808990F.
- M. W. Schneider, I. M. Oppel, H. Ott, L. G. Lechner, H.-J. S. Hauswald, R. Stoll and M. Mastalerz, Periphery-Substituted [4+6] Salicylbisimine Cage Compounds with Exceptionally High Surface Areas: Influence of the Molecular Structure on Nitrogen Sorption Properties, Chem. – Eur. J., 2012, 18, 836–847 Search PubMed.
- M. W. Schneider, H.-J. S. Hauswald, R. Stoll and M. Mastalerz, A shape-persistent exo-functionalized [4+6] imine cage compound with a very high specific surface area, Chem. Commun., 2012, 48, 9861–9863 Search PubMed.
- Y. Liu, X. Liu and R. Warmuth, Multicomponent Dynamic Covalent Assembly of a Rhombicuboctahedral Nanocapsule, Chem. – Eur. J., 2007, 13, 8953–8959 Search PubMed.
- X. Liu and R. Warmuth, Solvent Effects in Thermodynamically Controlled Multicomponent Nanocage Syntheses, J. Am. Chem. Soc., 2006, 128, 14120–14127 Search PubMed.
- X. Liu, Y. Liu, G. Li and R. Warmuth, One-Pot, 18-Component Synthesis of an Octahedral Nanocontainer Molecule, Angew. Chem., Int. Ed., 2006, 45, 901–904 Search PubMed.
- J. Koo, I. Kim, Y. Kim, D. Cho, I.-C. Hwang, R. D. Mukhopadhyay, H. Song, Y. H. Ko, A. Dhamija, H. Lee, W. Hwang, S. Kim, M.-H. Baik and K. Kim, Gigantic Porphyrinic Cages, Chem, 2020, 6, 3374–3384 Search PubMed.
- K. Su, W. Wang, S. Du, C. Ji, M. Zhou and D. Yuan, Reticular Chemistry in Construction of Porous Organic Cages, J. Am. Chem. Soc., 2020, 142, 18060–18072 Search PubMed.
- X. Wang, Y. Wang, H. Yang, H. Fang, R. Chen, Y. Sun, N. Zheng, K. Tan, X. Lu, Z. Tian and X. Cao, Assembled molecular face-rotating polyhedra to transfer chirality from two to three dimensions, Nat. Commun., 2016, 7, 12469 Search PubMed.
- H. Qu, X. Tang, X. Wang, Z. Li, Z. Huang, H. Zhang, Z. Tian and X. Cao, Chiral molecular face-rotating sandwich structures constructed through restricting the phenyl flipping of tetraphenylethylene, Chem. Sci., 2018, 9, 8814–8818 Search PubMed.
- A. G. Slater, M. A. Little, A. Pulido, S. Y. Chong, D. Holden, L. Chen, C. Morgan, X. Wu, G. Cheng, R. Clowes, M. E. Briggs, T. Hasell, K. E. Jelfs, G. M. Day and A. I. Cooper, Reticular synthesis of porous molecular 1D nanotubes and 3D networks, Nat. Chem., 2017, 9, 17–25 Search PubMed.
- L. Zhang, L. Xiang, C. Hang, W. Liu, W. Huang and Y. Pan, From Discrete Molecular Cages to a Network of Cages Exhibiting Enhanced CO2 Adsorption Capacity, Angew. Chem., Int. Ed., 2017, 56, 7787–7791 Search PubMed.
- C. Liu, K. Liu, C. Wang, H. Liu, H. Wang, H. Su, X. Li, B. Chen and J. Jiang, Elucidating heterogeneous photocatalytic superiority of microporous porphyrin organic cage, Nat. Commun., 2020, 11, 1047 Search PubMed.
- N. Sun, C. Wang, H. Wang, L. Yang, P. Jin, W. Zhang and J. Jiang, Multifunctional Tubular Organic Cage-Supported Ultrafine Palladium Nanoparticles for Sequential Catalysis, Angew. Chem., Int. Ed., 2019, 58, 18011–18016 Search PubMed.
- V. Abet, F. T. Szczypiński, M. A. Little, V. Santolini, C. D. Jones, R. Evans, C. Wilson, X. Wu, M. F. Thorne, M. J. Bennison, P. Cui, A. I. Cooper, K. E. Jelfs and A. G. Slater, Inducing Social Self-Sorting in Organic Cages To Tune The Shape of The Internal Cavity, Angew. Chem., Int. Ed., 2020, 59, 16755–16763 Search PubMed.
- W. A. Freeman, W. L. Mock and N. Y. Shih, Cucurbituril, J. Am. Chem. Soc., 1981, 103, 7367–7368 Search PubMed.
- J. Kim, I.-S. Jung, S.-Y. Kim, E. Lee, J.-K. Kang, S. Sakamoto, K. Yamaguchi and K. Kim, New Cucurbituril Homologues: Syntheses, Isolation, Characterization, and X-ray Crystal Structures of Cucurbit[n]uril (n=5, 7, and 8), J. Am. Chem. Soc., 2000, 122, 540–541 Search PubMed.
- J. W. Lee, S. Samal, N. Selvapalam, H.-J. Kim and K. Kim, Cucurbituril Homologues and Derivatives: New Opportunities in
Supramolecular Chemistry, Acc. Chem. Res., 2003, 36, 621–630 Search PubMed.
- S. J. Barrow, S. Kasera, M. J. Rowland, J. del Barrio and O. A. Scherman, Cucurbituril-Based Molecular Recognition, Chem. Rev., 2015, 115, 12320–12406 Search PubMed.
- E. Masson, X. Ling, R. Joseph, L. Kyeremeh-Mensah and X. Lu, Cucurbituril chemistry: a tale of supramolecular success, RSC Adv., 2012, 2, 1213–1247 Search PubMed.
- X. Sun, B. Liu, Q. Wu and F. Li, Photochemical properties and interfacial fluorescence sensing for homocysteine of triptycene orthoquinone layer-by-layer-assembled multilayers, Thin Solid Films, 2014, 562, 603–607 Search PubMed.
- J.-M. Zhao, H.-Y. Lu, J. Cao, Y. Jiang and C.-F. Chen, Highly selective synthesis of triptycene o-quinone derivatives and their optical and electrochemical properties, Tetrahedron Lett., 2009, 50, 219–222 Search PubMed.
- K. Baumgärtner, F. Rominger and M. Mastalerz, An Oxidative Macrobicyclic Ring Opening of a Triptycene to a Highly Functionalized Fluorene Derivative, J. Org. Chem., 2015, 80, 8881–8886 Search PubMed.
- F. Zhang, S. Bai, G. P. A. Yap, V. Tarwade and J. M. Fox, Abiotic Metallofoldamers as Electrochemically Responsive Molecules, J. Am. Chem. Soc., 2005, 127, 10590–10599 Search PubMed.
- K. Baumgärtner, M. Hoffmann, F. Rominger, S. M. Elbert, A. Dreuw and M. Mastalerz, Homoconjugation and Intramolecular Charge Transfer in Extended Aromatic Triptycenes with Different π-Planes, J. Org. Chem., 2020, 85, 15256–15272 Search PubMed.
- T. Hasell, S. Y. Chong, K. E. Jelfs, D. J. Adams and A. I. Cooper, Porous Organic Cage Nanocrystals by Solution Mixing, J. Am. Chem. Soc., 2012, 134, 588–598 Search PubMed.
- B. Teng, M. A. Little, T. Hasell, S. Y. Chong, K. E. Jelfs, R. Clowes, M. E. Briggs and A. I. Cooper, Synthesis of a Large, Shape-Flexible, Solvatomorphic Porous Organic Cage, Cryt. Growth Des., 2019, 19, 3647–3651 Search PubMed.
- L. Ueberricke, D. Holub, J. Kranz, F. Rominger, M. Elstner and M. Mastalerz, Triptycene End-Capped Quinoxalinophenanthrophenazines (QPPs): Influence of Substituents and Conditions on Aggregation in the Solid State, Chem. – Eur. J., 2019, 25, 11121–11134 Search PubMed.
- D. Holden, K. E. Jelfs, A. Trewin, D. J. Willock, M. Haranczyk and A. I. Cooper, Gas Diffusion in a Porous Organic Cage: Analysis of Dynamic Pore Connectivity Using Molecular Dynamics Simulations, Phys. Chem. C, 2014, 118, 12734–12743 Search PubMed.
- N. Guex and M. C. Peitsch, SWISS-MODEL and the Swiss-Pdb Viewer: An environment for comparative protein modeling, ELECTROPHORESIS, 1997, 18, 2714–2723 Search PubMed.
- W. L. Mock and N. Y. Shih, Host-guest binding capacity of cucurbituril, J. Org. Chem., 1983, 48, 3618–3619 Search PubMed.
- J. Lagona, P. Mukhopadhyay, S. Chakrabarti and L. Isaacs, The Cucurbit[n]uril Family, Angew. Chem., Int. Ed., 2005, 44, 4844–4870 Search PubMed.
- W.-H. Huang, P. Y. Zavalij and L. Isaacs, Chiral Recognition inside a Chiral Cucurbituril, Angew. Chem., Int. Ed., 2007, 46, 7425–7427 Search PubMed.
- T. Mitra, K. E. Jelfs, M. Schmidtmann, A. Ahmed, S. Y. Chong, D. J. Adams and A. I. Cooper, Molecular shape sorting using molecular organic cages, Nat. Chem., 2013, 5, 276 Search PubMed.
Footnotes |
† Electronic supplementary information (ESI) available. CCDC 2072797 (4), 2072798 (5), and 2072799 ((R,R)-9). For ESI and crystallographic data in CIF or other electronic format see DOI: 10.1039/d1qo00478f |
‡ Current address. Institut für Organische Chemie, Julius-Maximilians-Universität Würzburg, Am Hubland, 97074 Würzburg, Germany |
|
This journal is © the Partner Organisations 2021 |
Click here to see how this site uses Cookies. View our privacy policy here.