DOI:
10.1039/D4QI01035C
(Research Article)
Inorg. Chem. Front., 2024,
11, 4611-4618
A polar two-dimensional lead-free hybrid perovskite for self-powered polarization-sensitive photodetection†
Received
26th April 2024
, Accepted 31st May 2024
First published on 3rd June 2024
Abstract
Two-dimensional (2D) lead hybrid perovskites have attracted great attention due to their excellent stability and unique physical and chemical properties. Nevertheless, the toxicity of lead remains an urgent issue. Lead-free homovalent substituents (Sn2+and Ge2+) suffer from poor oxygen-sensitive stability, posing a serious challenge to their device applications. Compared to homovalent substituents, heterovalent substituted perovskites based on the VA group elements, especially bismuth, exhibit excellent stability, making them more competitive. However, 2D Bi-based perovskites with the composition 2D A2BiX4 (A = organic cation; X = Cl−, Br− or I−) cannot be formed considering the charge balance. Here, by reducing the occupancy rate of Bi3+ ions, we successfully obtained the first polar 2D Bi-based perovskite (4BrPEA)3BiI6 (4BrPEA = 4-bromophenethylammonium) with an A2PbX4-like layered structure. (4BrPEA)3BiI6 exhibits a narrow bandgap (2.00 eV) and excellent photoelectric response performance. Specifically, a single crystal device based on (4BrPEA)3BiI6 has been explored for self-powered polarization-sensitive photodetection with a large photocurrent anisotropic ratio ω of 2.16. This work demonstrates the great potential of heterovalent substituted 2D lead-free perovskites and opens up a new way to expand the synthesis of environmentally friendly and high-performance self-powered polar optoelectronic semiconductor materials.
Introduction
Lead-based hybrid perovskites, represented by CH3NH3PbI3, have attracted significant attention in various fields, including photovoltaics,1 light-emitting diodes,2 photoluminescence,3 ferroelectricity,4–6 and photodetection,7,8 on account of their excellent physical and chemical properties.9–11 However, their instability and toxicity greatly hinder further commercial development.12–14 Usually, the stability issues can be effectively addressed by employing dimensional reduction technology to construct 2D hybrid perovskites.15 2D perovskites possess excellent chemical stability compared to typical 3D CH3NH3PbI3 due to their quantum well structure of hydrophobic organic cations and inorganic perovskite layers. The alternant quantum well structure also introduces natural structural anisotropy for 2D perovskites, which facilitates the realization of polarization-sensitive detection.16–19 In particular, polar 2D perovskites exhibit the bulk photovoltaic effect (BPVE), which can produce stable open-circuit voltage and short-circuit current under illumination, making them candidates for highly efficient self-powered polarization-sensitive photodetectors.17 Although considerable progress has been made in lead-based 2D perovskite self-powered polarization-sensitive photodetection, the toxicity of lead remains a pressing issue.
Lead-free is the development trend of green chemistry in perovskites. To obtain 2D lead-free hybrid perovskites, two strategies have been developed: homovalent substitution and heterovalent substitution. Although Group IVA homovalent substituent (Sn2+ and Ge2+) perovskites have produced decent optical and electrical properties, their poorer oxygen-sensitive stability than lead-based perovskites poses a serious challenge to their device applications.12 Compared to homovalent substitution, heterovalent substitution based on the VA group element bismuth is more competitive. Bi3+ has the same 6S26P0 external electron configuration and a similar ionic radius to Pb2+, and its stability and semiconductor properties have been widely demonstrated.14 However, due to the total charge δ of the composition A2BiX4 (A = organic cation; X = Cl−, Br− or I−) greater than 0, the typical (100)-oriented 2D layered perovskites cannot be formed. Thus Bi-based hybrid perovskites tend to form low-dimensional 0D or 1D structures (e.g. 0D A3Bi2X9, 0D A3BiBr6, 1D ABiX4, and 1D A2BiX5).20–24 To satisfy the total charge balance and to form 2D A2PbX4-like structures simultaneously, it is necessary to reduce the occupancy of Bi sites (i.e., by introducing vacancies on the metal sites) on the layer. By reducing the chemical occupancy of Bi sites from 1 to 2/3, stable (100)-oriented 2D perovskites with the composition A3BiX6 can be formed (Scheme 1). Obviously, that is difficult in synthesis because the inherent driving force to form other non-vacancy structures cannot be avoided. At present, a 2D Bi-based perovskite is still a type of under-explored material with only three examples reported, all of which are non-polar structures.25–27 Polar perovskite materials, including ferroelectrics and pyroelectrics, have emerged as competitive self-powered photodetection candidates.7,17 In this context, it is urgent to develop polar Bi-based perovskites for green and high-performance self-powered polarization-sensitive photodetection.
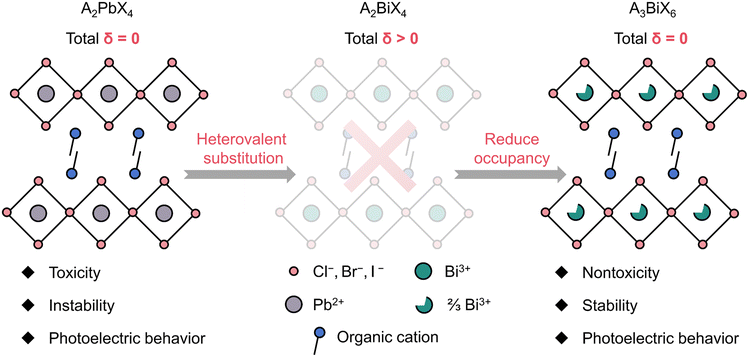 |
| Scheme 1 The strategy of forming the 2D Bi-based perovskite structure. δ represents the total charge. | |
Organic cations were shown to have the potential to template unusual hybrid perovskite structures,15 especially the halogenated organic cations that have the potential to control crystal symmetry breaking.28 Herein, we report the first polar 2D lead-free perovskite (4BrPEA)3BiI6 (4BrPEA = 4-bromophenethylammonium). (4BrPEA)3BiI6 possesses an A2PbX4-like layered structure, where the occupancy rate of Bi atoms on the 2D layer is 2/3. The directional effect of brominated organic cations on polar structures was demonstrated through single crystal structure analysis. (4BrPEA)3BiI6 exhibits excellent semiconductor performance with a narrow bandgap of 2.00 eV. Benefiting from the polar structure, polarization-sensitive detection devices based on (4BrPEA)3BiI6 exhibit a large anisotropy factor ω of 2.16, which is comparable to that of Pb-based perovskites.
Results and discussion
Crystal structure analysis
Dark red single crystals of (4BrPEA)3BiI6 were obtained by the slow temperature cooling method (Fig. S1, ESI†). The powder X-ray diffraction (PXRD) pattern of the powder sample matches well with the simulated results (Fig. S2, ESI†), which verifies the phase purity of the as-synthesized crystal. The PXRD spectrum of the single crystal shows the signals of the (0k0) plane. The thermogravimetric analysis shows that (4BrPEA)3BiI6 has good thermal stability up to 500 K (Fig. S3, ESI†). Single crystal X-ray diffraction (SC-XRD) shows that (4BrPEA)3BiI6 crystallizes in the polar space group Fmm2 (No. 42). Other crystal data and structure refinement details, bond lengths, and bond angles are presented in Tables S1, S2, and S3 in the ESI,† respectively. Crystal structure analysis reveals that the organic cation 4BrPEA separates the inorganic octahedral layer [BiI6]3− along the b-axis to form a typical (110)-oriented 2D quantum well structure (Fig. 1a). The I2A and I2B in the asymmetric unit were refined to be disordered, and their chemical occupancy was freely refined to 0.657 and 0.343 (Fig. S4, ESI†). Specifically, the chemical occupancy of Bi sites was fixed at 2/3 to meet the charge balance. Finally, the formula of (4BrPEA)3BiI6 was adopted, and the total charge δ was kept at 0.
 |
| Fig. 1 (a) 2D polar structure of (4BrPEA)3BiI6 viewed along the a-axis. (b) The second harmonic generation signals of (4BrPEA)3BiI6. | |
In (4BrPEA)3BiI6, the 2D layer is constructed by corner-sharing Bi2/3I6 octahedra, and the 4BrPEA cations and Bi2/3I6 octahedra are ordered along the c-axis, which results in a polar structure. The second harmonic generation signal obtained at room temperature verifies the polar structure (Fig. 1b). It is worth noting that (4BrPEA)3BiI6 is the first polar 2D Bi-based hybrid perovskite. By comparing with the reported 2D centrosymmetric structure of (4FPEA)3BiI6 (its space group is C2/c; 4FPEA = 4-fluorophenethylammonium),26 the origin of electrical polarization of (4BrPEA)3BiI6 was analyzed. (4BrPEA)3BiI6 and (4FPEA)3BiI6 are composed of similar organic cations, with the only difference being the halogen species on the para-position of the benzene ring. In (4FPEA)3BiI6, the 4FPEA cations exhibit an edge-to-face parallel arrangement with C–F⋯π interaction distances ranging from 3.713 to 3.830 Å (Fig. 2a and b). While in (4BrPEA)3BiI6, the orientation of the 4BrPEA cations exhibits vertical arrangement to adapt to strong C–Br⋯π interactions (3.417–3.424 Å) (Fig. 2c and d). In (4BrPEA)3BiI6 and (4FPEA)3BiI6, the main interaction between the [BiI6]3− layer and the organic amine cations is the N–H⋯I hydrogen bond, and the bond length range is roughly the same (Table S4, ESI†). Therefore, in (4BrPEA)3BiI6, the strong C–Br⋯π interactions drive the orientation arrangement of cations within the organic layer, which leads to the separation of positive and negative charges along the c-axis and ultimately results in the polar structure.28
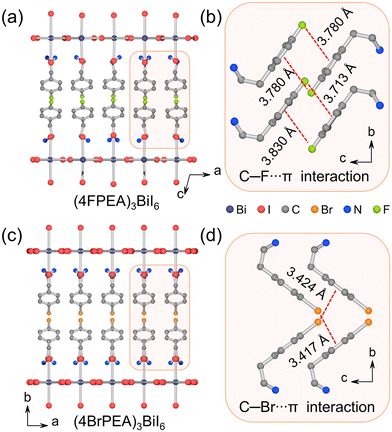 |
| Fig. 2 (a) Crystal structure of (4FPEA)3BiI6 viewed along the b-axis. (b) C–F⋯π interactions in (4FPEA)3BiI6. (c) Crystal structure of (4BrPEA)3BiI6 viewed along the c-axis. (d) C–Br⋯π interactions in (4BrPEA)3BiI6. | |
Semiconductor and optoelectronic properties
2D lead-free hybrid perovskites based on bismuth are expected to exhibit semiconducting-related optoelectronic behavior comparable to those of lead-based compounds. To characterize the semiconducting properties of (4BrPEA)3BiI6, ultraviolet-visible (UV-vis) absorption spectra were recorded. As shown in Fig. 3, (4BrPEA)3BiI6 exhibits an absorption cutoff of around 670 nm and a bandgap of 2.00 eV derived from the Kubelka–Munk function. Compared with the reported 2D Pb-based hybrid perovskites, such as (4BrPEA)2PbI4,29 (4AMP)PbI4 (4AMP = 4-(aminomethyl)piperidinium),30 and (BA)2PbI4 (BA = n-butylammonium),31 2D lead-free Bi-based perovskites exhibit a smaller bandgap.
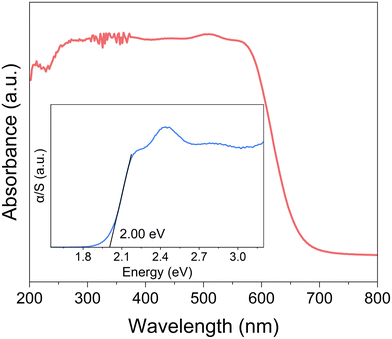 |
| Fig. 3 UV-vis absorption spectra of (4BrPEA)3BiI6. Insert: the optical bandgap. | |
Band gap analysis shows that (4BrPEA)3BiI6 has the potential to be an optoelectronic material.14,32–34 Therefore, we further investigated its photoresponse behavior upon 405 nm LED light irradiation under a 10 V bias. Fig. 4a shows the time-dependent photocurrent (I–t) of the (4BrPEA)3BiI6 single crystal device at different optical power densities. The photocurrent reaches 7.6 pA under an optical power P of 3.19 mW cm−2, and the corresponding Iph/Idark ratio is 102. Even under an optical power of 0.15 mW cm−2, (4BrPEA)3BiI6 still exhibits significant photocurrent. The calculated responsivity R and specific detectivity D* related to optical density are negatively correlated with radiation intensity (Fig. 4b). Under 0.15 mW cm−2 irradiation, the calculated D* and R values are 3.91 × 108 Jones and 0.99 μA W−1, respectively, which were comparable to some reported lead-free perovskites (Table 1).35,36 In addition, the (4BrPEA)3BiI6 device shows a fast rise/decay time of 29/30 ms at 405 nm (Fig. 4c), which is superior to some reported lead-free perovskite photodetectors (Table 1).
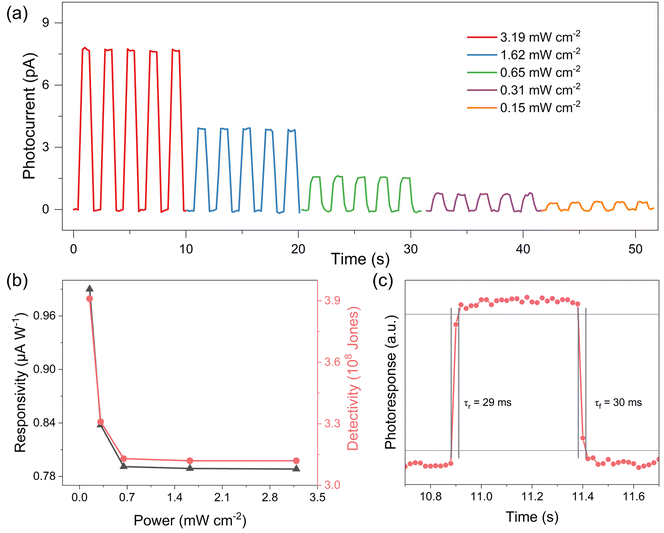 |
| Fig. 4 (a) The I–t curve of the (4BrPEA)3BiI6 single crystal device at 405 nm under 10 V bias. (b) The calculated R and D* related to the optical density. (c) The rise and decay time. | |
Table 1 Summary of the reported lead-free perovskite photodetectors
Compound |
D* (Jones) |
R (A W−1) |
Rise/decay time (ms) |
Ref. |
Cs3Bi2I9 |
1.2 × 1010 |
0.59 μ |
88.66/109.3 |
35
|
MA3Bi2I9 |
9.23 × 1011 |
1.9 m |
26.81/41.98 |
37
|
HDABiI5 |
5.3 × 108 |
6.8 m |
52/35 |
23
|
Cs3Bi2I6Br3 |
4.60 × 1011 |
15 m |
40.7/27.1 |
38
|
AG3Bi2I9 |
8.8 × 1010 |
14.8 m |
0.152/0.187 |
39
|
CsSnI3 |
3.85 × 105 |
54 m |
83.8/243.4 |
36
|
(4BrPEA)3BiI6 |
3.91 × 108 |
0.99 μ |
29/30 |
This work |
Self-powered polarization-sensitive photodetection
Polar hybrid perovskites have also shown great potential in self-powered photodetection based on the BPVE.40,41 The inherent polar structure drives us to further explore the BPVE behavior based on single crystal devices. Fig. 5a shows the current–voltage (I–V) photocurrent curves measured along the c-axis and a-axis (P = 2.94 mW cm−2). The I–V curve on the c-axis shows obvious short-circuit current (Isc = −1.3 pA) and open circuit voltage (Voc = 1.45 V), while the current curve on the a-axis almost passes through the origin. The I–t curve measured along the c-axis under 0 V shows that the photocurrent increases sharply under illumination, and the Iph/Idark ratio is about 50 (Fig. 5b) (P = 4.04 mW cm−2). The value of Voc is larger than many Pb-based hybrid perovskites, such as (BPA)2PbBr4 (Voc = 0.85 V) and (BA)2(FA)Pb2I7 (Voc = 0.3 V).16,17
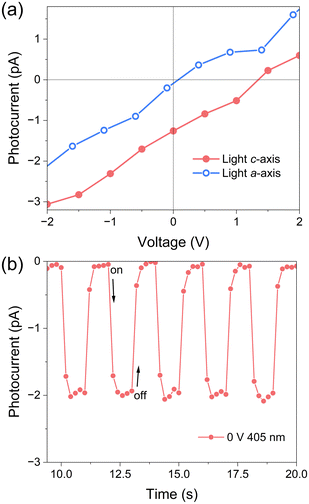 |
| Fig. 5 (a) I–V photocurrent curves measured along the c-axis and a-axis. (b) The I–t curve measured along the c-axis under 0 V bias. | |
The 2D (4BrPEA)3BiI6 perovskite structure has inherent structural anisotropy and can be used for polarization-sensitive photosensitive detection. Therefore, based on excellent BPVE activity, we further investigated the self-powered polarization-sensitive photoresponse of (4BrPEA)3BiI6. A 405 nm LED is used as the light source, ordinary light is converted into linearly polarized light through a polarizer, and the angle of LPL is controlled through a 1/2 wave plate (Fig. 6a) inside the bc-plane. The initial polarization angle (defined as 0°) of LPL is parallel to the b-axis. As shown in Fig. 6a, when the polarization angle of LPL is parallel to the b-axis, the photocurrent reaches its minimum value (Ivalley). The value of photocurrent varies with the polarization angle, and when the LPL angle is parallel to the c direction, the maximum photocurrent value (Ipeak) can be obtained. The polar coordination plot of the photocurrent and the polarization angle shows a clear double-lobed shape, and the calculated photocurrent anisotropy ratio ω (Ipeak/Ivalley) is 2.16. The I–t curve under pulsed light shows a clear polarization angle dependence, which proves the reproducibility and stability of the detector. Fig. 6e summarizes the performance of the reported perovskite and lead-based perovskite polarization-sensitive single crystal detectors.17,23,42–47 The ω value of (4BrPEA)3BiI6 exceeds that of many lead-free perovskites and is even comparable to Pb-based perovskites.
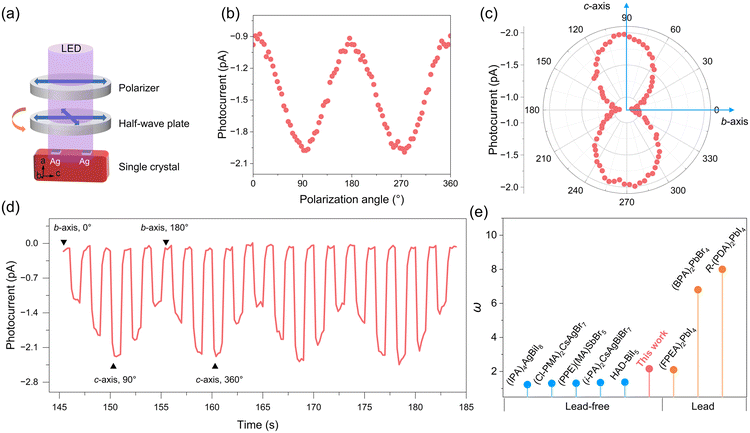 |
| Fig. 6 (a) Schematic diagram of the polarization-sensitive photodetection device. (b) Angle-dependent photocurrent measured under 0 V bias. (c) Polar plots of the angle-dependent photocurrents measured under 0 V bias. (d) Angle-dependent I–t measurements at 405 nm pulsed light. (e) Comparison of the ω values for 2D perovskite photodetectors. | |
The polarization-dependent photocurrent measured at 10 V bias is shown in Fig. S5,† and the photocurrent displays a small ω value of 1.05. This result demonstrates the enormous potential of BPVE-enhanced self-powered LPL detectors. The large ω value of (4BrPEA)3BiI6 at 0 V can be attributed to the angle dependence of the BPVE, which can be expressed as
Jz = Iβ31 sin2(θ) + Iβ33 cos2(θ) |
where
I is the light intensity,
θ is the angle between the LPL polarization plane and the
z axis (polar axis), and
β31 and
β33 are the bulk photovoltaic coefficients.
48–51 Weak coupling occurs when the direction of LPL is perpendicular to the polar axis (
c-axis), resulting in a low
Ivalley. While strong coupling occurs when the LPL is parallel to the polar axis, which promotes carrier separation, resulting in a large
Ipeak and ultimately highly sensitive polarization-sensitive detection.
Conclusions
In summary, by reasonably reducing the occupancy rate of Bi3+ on the 2D layer, we successfully obtained the first 2D polar Bi-based perovskite (4BrPEA)3BiI6 with an A2PbX4-like structure. (4BrPEA)3BiI6 single crystal devices exhibit photocurrent anisotropy ω values (2.16) comparable to Pb-based perovskite devices. This work provides an effective approach for the targeted design of green, lead-free, high-performance, 2D polarization-sensitive perovskites. At the same time, the narrow bandgap, low toxicity, high chemical stability, and excellent self-powered photodetection performance of (4BrPEA)3BiI6 also show its great application potential in solar cells and other photovoltaic conversion devices.
Experimental
Chemicals
Bismuth trioxide (Bi2O3, 99.0%,), 4-bromophenethylamine (4BrPEA, 98%), hypophosphorous acid (H3PO2, AR, 50 wt% in H2O), and hydroiodic acid (HI, AR, 55.0–58%, contains ≤1.5% H3PO2 as a stabilizer) were all commercially obtained without further purification.
Synthesis of (4BrPEA)3BiI6
Bi2O3 (0.233 g, 0.5 mmol) was dissolved in 57% hydroiodic (HI) solution (100 mL) and then H3PO2 (5 mL) and 4BrPEA (0.600 g, 3 mmol) were slowly added dropwise. The mixture was stirred continuously at 373 K for 30 minutes until the precipitate was completely dissolved to give a clear red solution. Slowly cooling the red solution at a rate of 5 K day−1 from 373 K to 298 K resulted in the formation of dark red plate-shaped crystals of (4BrPEA)3BiI6.
Device fabrication
The surfaces of the single crystal were coated with silver paste along the different axes. The effective area of the crystal device was determined to be 1 × 0.3 mm2 by the Phenix MC-D310U high-definition digital camera.
Photocurrent measurement
The photocurrent was measured using a PDA FS380 (Primarius) source meter. The 405 nm LED was obtained from Thorlabs. The polarizer (Glan–Taylor prism) converted the LED laser beam into polarized light, and the polarization angle of the polarized light was then adjusted by a half-wave plate. The state of the polarization state of linearly polarized light was calibrated by a polarimeter system (PAX1000). The light intensity was measured using a digital console with a photodiode sensor (PM120VA, Thorlabs).
Author contributions
C.-D. L. performed most of the experiments and wrote the original manuscript. C.-C. F. and B.-D. L. participated in the writing and revision of the manuscript. W. Z. was responsible for funding acquisition, supervision, writing review, and editing. All authors discussed the results and commented on the manuscript.
Conflicts of interest
There are no conflicts to declare.
Acknowledgements
This work was financially supported by the National Natural Science Foundation of China (Grant 21991144). We thank the Big Data Center of Southeast University for providing the facility support on the numerical calculations.
References
- A. Kojima, K. Teshima, Y. Shirai and T. Miyasaka, Organometal Halide Perovskites as Visible-Light Sensitizers for Photovoltaic Cells, J. Am. Chem. Soc., 2009, 131, 6050–6051 CrossRef CAS PubMed
.
- N. Wang, L. Cheng, R. Ge, S. Zhang, Y. Miao, W. Zou, C. Yi, Y. Sun, Y. Cao, R. Yang, Y. Wei, Q. Guo, Y. Ke, M. Yu, Y. Jin, Y. Liu, Q. Ding, D. Di, L. Yang, G. Xing, H. Tian, C. Jin, F. Gao, R. H. Friend, J. Wang and W. Huang, Perovskite light-emitting diodes based on solution-processed self-organized multiple quantum wells, Nat. Photonics, 2016, 10, 699–704 CrossRef CAS
.
- C. C. Stoumpos, C. D. Malliakas and M. G. Kanatzidis, Semiconducting tin and lead iodide perovskites with organic cations: phase transitions, high mobilities, and near-infrared photoluminescent properties, Inorg. Chem., 2013, 52, 9019–9038 CrossRef CAS PubMed
.
- C.-Q. Jing, C.-Y. Chai, X.-B. Han, C.-D. Liu, W. Wang, T.-Y. Ju, J.-M. Zhang, M.-L. Jin, Q. Ye and W. Zhang, Sensing polarized light via switchable Rashba-Dresselhaus spin splitting in a ferroelectric semiconductor, Matter, 2024, 7, 991–1001 CrossRef CAS
.
- C.-C. Fan, X.-B. Han, B.-D. Liang, C. Shi, L.-P. Miao, C.-Y. Chai, C.-D. Liu, Q. Ye and W. Zhang, Chiral Rashba Ferroelectrics for Circularly Polarized Light Detection, Adv. Mater., 2022, 34, 2204119 CrossRef CAS
.
- W. Q. Liao, Y. Zhang, C. L. Hu, J. G. Mao, H. Y. Ye, P. F. Li, S. D. Huang and R. G. Xiong, A lead-halide perovskite molecular ferroelectric semiconductor, Nat. Commun., 2015, 6, 7338 CrossRef PubMed
.
- I. H. Park, K. C. Kwon, Z. Zhu, X. Wu, R. Li, Q. H. Xu and K. P. Loh, Self-Powered Photodetector Using Two-Dimensional Ferroelectric Dion-Jacobson Hybrid Perovskites, J. Am. Chem. Soc., 2020, 142, 18592–18598 CrossRef CAS
.
- C.-C. Fan, B.-D. Liang, C.-D. Liu, C.-Y. Chai, X.-B. Han and W. Zhang, Stable organic lead iodides with three-dimensional crystallographic and electronic structures showing high photoresponse, Inorg. Chem. Front., 2022, 9, 6404–6411 RSC
.
- D. Shi, V. Adinolfi, R. Comin, M. Yuan, E. Alarousu, A. Buin, Y. Chen, S. Hoogland, A. Rothenberger, K. Katsiev, Y. Losovyj, X. Zhang, P. A. Dowben, O. F. Mohammed, E. H. Sargent and O. M. Bakr, Low trap-state density and long carrier diffusion in organolead trihalide perovskite single crystals, Science, 2015, 347, 519–522 CrossRef CAS PubMed
.
- B. D. Liang, C. C. Fan, C. D. Liu, C. Y. Chai, X. B. Han and W. Zhang, Near-room-temperature martensitic actuation profited from one-dimensional hybrid perovskite structure, Nat. Commun., 2022, 13, 6599 CrossRef CAS PubMed
.
- W. Wang, C. D. Liu, C. C. Fan, X. B. Fu, C. Q. Jing, M. L. Jin, Y. M. You and W. Zhang, Rational Design of 2D Metal Halide Perovskites with Low Congruent Melting Temperature and Large Melt-Processable Window, J. Am. Chem. Soc., 2024, 146, 9272–9284 CrossRef CAS PubMed
.
- B. Vargas, G. Rodríguez-López and D. Solis-Ibarra, The Emergence of Halide Layered Double Perovskites, ACS Energy Lett., 2020, 5, 3591–3608 CrossRef CAS
.
- T. Li, S. Luo, X. Wang and L. Zhang, Alternative Lone-Pair ns2 -Cation-Based Semiconductors beyond Lead Halide Perovskites for Optoelectronic Applications, Adv. Mater., 2021, 33, 2008574 CrossRef CAS
.
- Y. Zhang, Y. Ma, Y. Wang, X. Zhang, C. Zuo, L. Shen and L. Ding, Lead-Free Perovskite Photodetectors: Progress, Challenges, and Opportunities, Adv. Mater., 2021, 33, 2006691 CrossRef CAS PubMed
.
- L. Mao, C. C. Stoumpos and M. G. Kanatzidis, Two-Dimensional Hybrid Halide Perovskites: Principles and Promises, J. Am. Chem. Soc., 2018, 141, 1171–1190 CrossRef PubMed
.
- Z. Xu, X. Dong, L. Wang, H. Wu, Y. Liu, J. Luo, M. Hong and L. Li, Precisely Tailoring a FAPbI3-Derived Ferroelectric for Sensitive Self-Driven Broad-Spectrum Polarized Photodetection, J. Am. Chem. Soc., 2023, 145, 1524–1529 CrossRef CAS PubMed
.
- C. Ji, D. Dey, Y. Peng, X. Liu, L. Li and J. Luo, Ferroelectricity-Driven Self-Powered Ultraviolet Photodetection with Strong Polarization Sensitivity in a Two-Dimensional Halide Hybrid Perovskite, Angew. Chem., Int. Ed., 2020, 59, 18933–18937 CrossRef CAS PubMed
.
- J. Ma, C. Fang, L. Liang, H. Wang and D. Li, Full-Stokes Polarimeter Based on Chiral Perovskites with Chirality and Large Optical Anisotropy, Small, 2021, 17, 2103855 CrossRef CAS PubMed
.
- L. Li, L. Jin, Y. Zhou, J. Li, J. Ma, S. Wang, W. Li and D. Li, Filterless Polarization-Sensitive 2D Perovskite Narrowband Photodetectors, Adv. Opt. Mater., 2019, 7, 1900988 CrossRef CAS
.
- A. J. Dennington and M. T. Weller, Synthesis, structure and optoelectronic properties of hybrid iodobismuthate & iodoantimonate semiconducting materials, Dalton Trans., 2018, 47, 3469–3484 RSC
.
- L. Yao, Z. Zeng, C. Cai, P. Xu, H. Gu, L. Gao, J. Han, X. Zhang, X. Wang, X. Wang, A. Pan, J. Wang, W. Liang, S. Liu, C. Chen and J. Tang, Strong Second- and Third-Harmonic Generation in 1D Chiral Hybrid Bismuth Halides, J. Am. Chem. Soc., 2021, 143, 16095–16104 CrossRef CAS PubMed
.
- R. Jakubas, M. Rok, K. Mencel, G. Bator and A. Piecha-Bisiorek, Correlation between crystal structures and polar (ferroelectric) properties of hybrids of haloantimonates(III) and halobismuthates(III), Inorg. Chem. Front., 2020, 7, 2107–2128 RSC
.
- C.-D. Liu, C.-C. Fan, B.-D. Liang, C.-Y. Chai, C.-Q. Jing, X.-B. Han and W. Zhang, Spectrally Selective Polarization-Sensitive Photodetection Based on a 1D Lead-Free Hybrid Perovskite Ferroelectric, ACS Mater. Lett., 2023, 5, 1974–1981 CrossRef CAS
.
- L. Peedikakkandy, S. Chatterjee and A. J. Pal, Bandgap Engineering and Efficient Conversion of a Ternary Perovskite (Cs3Bi2I9) to a Double Perovskite (Cs2NaBiI6) with the Aid of Alkali Metal Sulfide, J. Phys. Chem. C, 2020, 124, 10878–10886 CrossRef CAS
.
- D. B. Mitzi, Organic−Inorganic Perovskites Containing Trivalent Metal Halide Layers: The Templating Influence of the Organic Cation Layer, Inorg. Chem., 2000, 39, 6107–6113 CrossRef CAS PubMed
.
- M. Li, H. Li, W. Li, B. Li, T. Lu, X. Feng, C. Guo, H. Zhang, H. Wei and B. Yang, Oriented 2D Perovskite Wafers for Anisotropic X-ray Detection through a Fast Tableting Strategy, Adv. Mater., 2022, 34, 2108020 CrossRef CAS
.
- H. Peng, Q. Liu, Y. Z. Lu, S. J. Yang, J. C. Qi, X. G. Chen and W. Q. Liao, A chiral two-dimensional perovskite-like lead-free bismuth(III) iodide hybrid with high phase transition temperature, Chem. Commun., 2023, 59, 10295–10298 RSC
.
- T. Schmitt, S. Bourelle, N. Tye, G. Soavi, A. D. Bond, S. Feldmann, B. Traore, C. Katan, J. Even, S. E. Dutton and F. Deschler, Control of Crystal Symmetry Breaking with Halogen-Substituted Benzylammonium in Layered Hybrid Metal-Halide Perovskites, J. Am. Chem. Soc., 2020, 142, 5060–5067 CrossRef CAS PubMed
.
- D. B. Straus, N. Iotov, M. R. Gau, Q. Zhao, P. J. Carroll and C. R. Kagan, Longer Cations Increase Energetic Disorder in Excitonic 2D Hybrid Perovskites, J. Phys. Chem. Lett., 2019, 10, 1198–1205 CrossRef CAS PubMed
.
- L. Mao, W. Ke, L. Pedesseau, Y. Wu, C. Katan, J. Even, M. R. Wasielewski, C. C. Stoumpos and M. G. Kanatzidis, Hybrid Dion–Jacobson 2D Lead Iodide Perovskites, J. Am. Chem. Soc., 2018, 140, 3775–3783 CrossRef CAS PubMed
.
- C. C. Stoumpos, D. H. Cao, D. J. Clark, J. Young, J. M. Rondinelli, J. I. Jang, J. T. Hupp and M. G. Kanatzidis, Ruddlesden–Popper Hybrid Lead Iodide Perovskite 2D Homologous Semiconductors, Chem. Mater., 2016, 28, 2852–2867 CrossRef CAS
.
- J. Y. Kim, J. W. Lee, H. S. Jung, H. Shin and N. G. Park, High-Efficiency Perovskite Solar Cells, Chem. Rev., 2020, 120, 7867–7918 CrossRef CAS PubMed
.
- H. Y. Zhang, Z. Wei, P. F. Li, Y. Y. Tang, W. Q. Liao, H. Y. Ye, H. Cai and R. G. Xiong, The Narrowest Band Gap Ever Observed in Molecular Ferroelectrics: Hexane-1,6-diammonium Pentaiodobismuth(III), Angew. Chem., Int. Ed., 2018, 57, 526–530 CrossRef CAS PubMed
.
- A. Maiti and A. J. Pal, Effect of Cation Occupancy Ordering in Double Perovskites To Overcome Hurdles in Carrier Transport: Cs2AgBiBr6 as a Case Study, J. Phys. Chem. C, 2021, 125, 16324–16333 CrossRef CAS
.
- A. A. Hussain, Constructing Caesium-Based Lead-Free Perovskite Photodetector Enabling Self-Powered Operation with Extended Spectral Response, ACS Appl. Mater. Interfaces, 2020, 12, 46317–46329 CrossRef CAS
.
- M. Han, J. Sun, M. Peng, N. Han, Z. Chen, D. Liu, Y. Guo, S. Zhao, C. Shan, T. Xu, X. Hao, W. Hu and Z.-X. Yang, Controllable Growth of Lead-Free All-Inorganic Perovskite Nanowire Array with Fast and Stable Near-Infrared Photodetection, J. Phys. Chem. C, 2019, 123, 17566–17573 CrossRef CAS
.
- A. A. Hussain, A. K. Rana and M. Ranjan, Air-stable lead-free hybrid perovskite employing self-powered photodetection with an electron/hole-conductor-free device geometry, Nanoscale, 2019, 11, 1217–1227 RSC
.
- D. Liu, B. B. Yu, M. Liao, Z. Jin, L. Zhou, X. Zhang, F. Wang, H. He, T. Gatti and Z. He, Self-Powered and Broadband Lead-Free Inorganic Perovskite Photodetector with High Stability, ACS Appl. Mater. Interfaces, 2020, 12, 30530–30537 CrossRef CAS PubMed
.
- W. Zhang, Y. Sui, B. Kou, Y. Peng, Z. Wu and J. Luo, Large-Area Exfoliated Lead-Free Perovskite-Derivative Single-Crystalline Membrane for Flexible Low-Defect Photodetectors, ACS Appl. Mater. Interfaces, 2020, 12, 9141–9149 CrossRef CAS PubMed
.
- P.-J. Huang, K. Taniguchi and H. Miyasaka, Bulk Photovoltaic Effect in a Pair of Chiral-Polar Layered Perovskite-Type Lead Iodides Altered by Chirality of Organic Cations, J. Am. Chem. Soc., 2019, 141, 14520–14523 CrossRef CAS PubMed
.
- P.-J. Huang, K. Taniguchi and H. Miyasaka, Crucial Contribution of Polarity for the Bulk Photovoltaic Effect in a Series of Noncentrosymmetric Two-Dimensional Organic–Inorganic Hybrid Perovskites, Chem. Mater., 2022, 34, 4428–4436 CrossRef CAS
.
- Z. Li, Q. Chen, Z. Y. Wang, Y. P. Fan, T. T. Zhu, C. M. Ji, X. J. Kuang and J. H. Luo, Centimeter-size single crystal of a lead-free double perovskite for broad-spectrum polarization-sensitive detection, J. Mater. Chem. C, 2022, 10, 18063–18068 RSC
.
- W. Wu, Z. Xu, Y. Yao, Y. Liu, G. Long, L. Li, M. Hong and J. Luo, Realization of In-Plane Polarized Light Detection Based on Bulk Photovoltaic Effect in A Polar van der Waals Crystal, Small, 2022, 18, 2200011 CrossRef CAS PubMed
.
- W. C. Zhang, M. C. Hong and J. H. Luo, Centimeter-Sized Single Crystal of a One-Dimensional Lead-Free Mixed-Cation Perovskite Ferroelectric for Highly Polarization Sensitive Photodetection, J. Am. Chem. Soc., 2021, 143, 16758–16767 CrossRef CAS PubMed
.
- Y. Li, T. Yang, Z. Xu, X. Liu, X. Huang, S. Han, Y. Liu, M. Li, J. Luo and Z. Sun, Dimensional Reduction of Cs2AgBiBr6: A 2D Hybrid Double Perovskite with Strong Polarization Sensitivity, Angew. Chem., Int. Ed., 2020, 59, 3429–3433 CrossRef CAS PubMed
.
- M. Li, S. Han, B. Teng, Y. Li, Y. Liu, X. Liu, J. Luo, M. Hong and Z. Sun, Minute-Scale Rapid Crystallization of a Highly Dichroic 2D Hybrid Perovskite Crystal toward Efficient Polarization–Sensitive Photodetector, Adv. Opt. Mater., 2020, 8, 2000149 CrossRef CAS
.
- B.-D. Liang, C.-C. Fan, C.-D. Liu, T.-Y. Ju, C.-Y. Chai, X.-B. Han and W. Zhang, Large in-plane anisotropic 2D perovskites toward highly linear polarized light responses, Inorg. Chem. Front., 2023, 10, 5035–5043 RSC
.
- M. M. Yang and M. Alexe, Light-Induced Reversible Control of Ferroelectric Polarization in BiFeO3, Adv. Mater., 2018, 30, 1704908 CrossRef PubMed
.
- W. T. H. Koch, R. Munser, W. Ruppel and P. Würfel, Anomalous photovoltage in BaTiO3, Ferroelectrics, 1976, 13, 305–307 CrossRef CAS
.
- T. Choi, S. Lee, Y. J. Choi, V. Kiryukhin and S.-W. Cheong, Switchable Ferroelectric Diode and Photovoltaic Effect in BiFeO3, Science, 2009, 324, 63–66 CrossRef CAS PubMed
.
- M.-M. Yang, Z.-D. Luo, D. J. Kim and M. Alexe, Bulk photovoltaic effect in monodomain BiFeO3 thin films, Appl. Phys. Lett., 2017, 110, 183902 CrossRef
.
Footnote |
† Electronic supplementary information (ESI) available: Other experimental details, Fig. S1–S5 and Tables S1–S4. CCDC 2343629. For ESI and crystallographic data in CIF or other electronic format see DOI: https://doi.org/10.1039/d4qi01035c |
|
This journal is © the Partner Organisations 2024 |
Click here to see how this site uses Cookies. View our privacy policy here.