DOI:
10.1039/D5YA00002E
(Paper)
Energy Adv., 2025, Advance Article
Towards green mobility: investigating hydrogen-enriched waste plastic biodiesel blends with n-butanol for sustainable diesel engine applications†
Received
3rd January 2025
, Accepted 29th March 2025
First published on 15th April 2025
Abstract
This study examines the performance of pyrolyzed waste plastic biodiesel (WPO) in a compression ignition engine when combined with n-butanol and enriched hydrogen (H2). Initially, low-density polyethylene (LDPE) plastic waste underwent conversion into waste plastic biodiesel via a pyrolysis thermochemical process. Experiments were conducted to evaluate blends consisting of 30% and 40% waste plastic biodiesel. In order to enhance the physical properties of the WPO, an additive consisting of 5% n-butanol (nBut5) was introduced, with the objective of improving combustion performance and minimizing exhaust emissions. Furthermore, enriched hydrogen was delivered to the combustion chamber via the inlet manifold at flow rates of 8 and 10 liters per minute (lpm). The findings indicated that the 40% WPO combined with 5% n-butanol demonstrated combustion properties that are similar to those of traditional diesel fuel. Moreover, the integration of the 40 WPO + nBut5 blend with 10 lpm enriched hydrogen resulted in a notable reduction in brake specific fuel consumption (BSFC) by 20.89% and an enhancement in brake thermal efficiency (BTE) by 8.22%, alongside a decrease in exhaust emissions, which included a reduction in carbon monoxide (CO) by 43.84%, unburned hydrocarbons (UBHC) by 57.8 ppm, and smoke opacity by 14.70%. Nonetheless, there was a notable increase in nitrogen oxide (NOx) emissions, which went up by 236 ppm when compared to conventional diesel fuel.
1. Introduction
Fossil fuel reserves are finite, and as they become scarcer, the process of extraction grows more difficult and expensive. The combustion of petroleum-based fuels generates large quantities of carbon dioxide (CO2), a major contributor to climate change and the global temperature rise.1 Finding sustainable alternatives to tackle these issues and secure future energy supply is essential. Biodiesel has emerged as a promising option due to its renewable nature, environmental benefits, and minimal environmental impact from its residues.2 The potential of the biofuel market was analyzed in the Biofuels Market Size, Share & Growth Analysis Report, in which the projected market growth was reported to be approximately USD 243.37 billion by 2033, with a compound annual growth rate (CAGR) of 7.02%.3 Current research into biodiesel generation has primarily concentrated on utilizing agricultural byproducts as the main source of feedstock. Nonetheless, this approach is burdened by elevated production expenses and a dispute regarding the choice between food and fuel. To address these challenges, there has been increased worldwide emphasis on second-generation biodiesel in the past decade. This innovative method utilizes biomass materials that are not derived from food, including items such as wood, straw, used cooking oil, and plastic waste.4
The transformation of waste plastic into valuable liquid fuel is of utmost importance due to the alarming annual influx of plastic into the ocean, reaching up to 8 million tons. This has a profound impact on marine animals and, consequently, the food chain.5 Among the two recycling procedures, mechanical and chemical, the chemical recycling method known as pyrolysis is considered the most appropriate choice. This approach achieves a 70% efficiency when heated to temperatures ranging from 350 °C to 450 °C.6 The usage of pure WPO (waste plastic oil) and distilled WPO is not suitable for engines because their higher density and viscosity, lower cetane number, larger sulfur content, and acid value highly affect engine performance and emission.7–10 In order to overcome these issues, the addition of oxygenated additives such as alcohols, ether, esters, or metal-oxide nanoparticles into WPO blends can lessen the aromatic content of WPO.11 Shafferina et al. examined various kinds of plastic waste, including PET, HDPE, PVC, LDPE, PP, and PS. They suggested that the properties of PS (polystyrene) are similar to those of diesel fuel, and that it can be effectively processed through pyrolysis, transforming it into a more valuable oil product instead of it being discarded into landfill forever.12–14
Typically, oxygenated biofuels achieve complete combustion and lower emissions. Butanol has attracted significant interest because it offers several advantages, including a higher energy content on a volumetric basis, compatibility with biodiesel, a higher cetane number, reduced corrosiveness compared to other alcohol fuels, and the ability to be used in engines without requiring modification.15,16 Utilizing alcohol fuels enhances the burning properties of biodiesel and aids in moving towards a more sustainable energy framework by lowering the overall greenhouse gas emissions throughout its lifecycle.17 The experimental investigation of n-butanol as a port fuel injection additive with pure Mahua biodiesel resulted in emissions that were lower than the regulated limits when compared to pure biodiesel.18 An experimental study on the incorporation of butanol into gasoline showed an increase in flame speed, which in turn raised the combustion burn rate, along with the pressure and heat release rate inside the cylinder.19 An experimental investigation into the dual fuel combustion mode of butanol/biodiesel revealed that advancing the direct injection timing of butanol led to a decrease in combustion duration and an enhancement in the indicated thermal efficiency, while also contributing to lower emissions of nitrogen oxides and particulate matter.20,21 An experimental study on butanol blending in a biogas-biodiesel fueled CI engine under dual fuel, reactivity controlled compression ignition (RCCI), and homogeneous charge compression ignition (HCCI) modes demonstrated that dual fuel combustion can fully capitalize on biogas energy, leading to a notable enhancement in efficiency.22 Incorporating alcohols into biodiesel seeks to minimize the ignition delay time and decrease the combustion duration in diesel engines. Research has shown that the addition of 1-butanol results in a stable, homogeneous blend, aiding in the advancement of sustainable and environmentally friendly fuels for a more responsible and energy-efficient future.23,24
The current research examines the utilization of hydrogen-enriched biodiesel to improve the combustion efficiency and decrease emissions. Increasing the quantity of hydrogen reduces the combustion duration and causes a rise in pressure. It achieves a fuel reduction of up to 5.4% and decreased carbon dioxide emissions.25–28 The incorporation of H2 at flow rates between 10 L min−1 and 30 L min−1 has markedly enhanced engine performance and contributed positively to the reduction of exhaust pollutants.29,30 An investigation into the induction and injection techniques for hydrogen delivery into the cylinder demonstrated that a low flow rate is suitable for the induction method, whilst a large flow rate is required for the injection method.31–36 Therefore, this study employed the induction method for hydrogen delivery in conjunction with air. This research aims to employ waste plastic biodiesel in a compression ignition engine, building on prior studies37,38 that explored the use of hydrogen to enhance the performance and combustion characteristics of palm biodiesel. Hence, converting waste products into energy sources is a valuable and essential strategy for government waste management policies.39
The use of hydrogen-enriched waste plastic biodiesel contributes to various sustainable development goals (SDGs). By utilizing waste-to-energy technologies, enhancing fuel efficiency, and reducing emissions, it supports affordable and clean energy (SDG 7). The reduction in dependence on fossil fuels through the use of waste plastic biodiesel, along with hydrogen as a renewable and sustainable fuel, promotes decent work and economic growth (SDG 8). Additionally, the adoption of renewable hydrogen fuel fosters infrastructure development and enhances industrial processes, thereby contributing to industry, innovation, and infrastructure (SDG 9). Furthermore, the reduction of exhaust pollutants leads to improved air quality, directly supporting climate action (SDG 13) and sustainable cities and communities (SDG 11).40,41
1.1. Scope and novelty
Adding 5% butanol to waste plastic oil (WPO) is a new way to improve the physical and combustion properties of waste plastic biodiesel. This is because most previous studies have used higher blends that may lower the fuel's calorific value and energy content, even though they get the best reductions in exhaust emissions. Previous research has indicated that a higher proportion of waste plastic oil (WPO) in fuel blends enhances brake thermal efficiency (BTE) while reducing specific fuel consumption (SFC) as the load increases. Consequently, the author selected WPO blends of 30% and 40% for further investigation.42,43 A study examining the combination of 5% butanol with diesel fuel and biodiesel demonstrated that this blend is a more favorable alternative for diesel engines. This is attributed to its effectiveness in lowering exhaust gas temperatures and reducing NOx and CO emissions. However, it is important to note that higher concentrations of pentanol result in increased HC emissions. While higher butanol concentrations lead to a reduction in energy content, the impact at 5% is minimal, ensuring good engine performance without the need for significant modifications to the engine or fuel system. Therefore, the author selected 5% butanol as an additive.44 Based on our previous work, supplying 8–10 lpm of hydrogen enhances the combustion efficiency, leading to significant improvements in brake thermal efficiency (BTE) and reductions in emissions, including CO, unburned hydrocarbons (UBHC), and smoke opacity.45,46 The effective operation at 40% WPO demonstrates an increased substitution rate for diesel fuel without notable detriment to engine performance, potentially fostering wider acceptance of alternative fuels and facilitating a transition to a more sustainable and circular economy in the automobile industry.
2. Materials and methods
2.1. Production of plastic oil
Waste plastic is first gathered from dump sites and roadside locations, and subsequently pulverized into small particles, and meticulously cleaned. The comminuted polymers are put into reactor, accompanied by zeolites serving as a catalyst. The burner subjected the reactor to a temperature range of 300–500 °C. Fig. 1 illustrates the trajectory of the combustion gas as it traverses a connecting pipe and enters a condenser. The gas pressure within the pipe is diminished due to a cooling process. Consequently, the incineration of 1 kg of plastic yields around 600 to 750 milliliters of biodiesel fuel (Table 1).
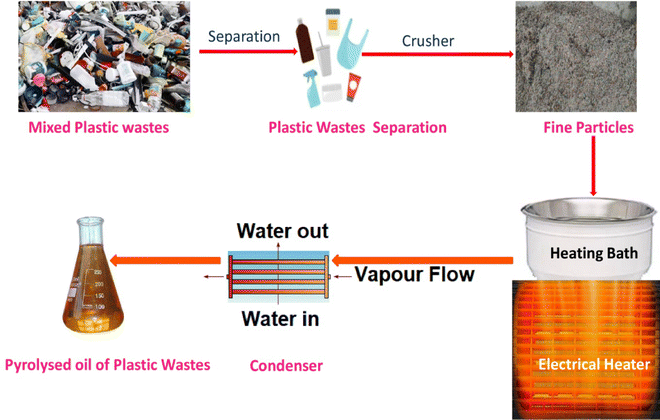 |
| Fig. 1 Preparation of waste plastic oil. | |
Table 1 Properties of the tested fuels
Properties |
Diesel |
WPO 30 |
WPO 40 |
WPO 40+ nBut5 |
ASTM method |
Specific gravity |
0.84 |
0.827 |
0.821 |
0.83 |
D1298 |
Kinematic viscosity (cSt) at 40 °C |
2.15 |
2.4 |
2.52 |
2.37 |
D445 |
Calorific value (kJ kg−1) |
43 500 |
42 834 |
42 501 |
43 167 |
D420 |
Cetane number |
54 |
56 |
56 |
59 |
D613 |
Flash point °C |
45 |
39 |
38 |
40 |
D93 |
2.2. Experimental setup
The experimental study was conducted using a single-cylinder, four-stroke diesel engine manufactured by Kirloskar. The engine is highly durable and is specifically designed for efficiently pumping water in agricultural fields. The engine has a maximum power output of 5.2 kilowatts and operates at a speed of 1500 rpm. The engine is equipped with a water-cooled, direct injection system. It has an 87.5 mm cylinder bore and a 110 mm stroke length. The compression ratio is 17.5, the injection timing is set at 23° bTDC, and the injection pressure is 200 bar. The engine was subjected to testing loads of 0, 4.5 kg, 9.01 kg, 13.5 kg, and 18.01 kg using an eddy current dynamometer. The engine's technical specifications are detailed in Table 2, while the experimental setup is illustrated in a line diagram in Fig. 2. The initial fuel used is diesel, which is subsequently blended with waste plastic oil in proportions of 30%, and 40%. Additionally, alumina nanoparticles are added to the blend of waste plastic oil. Each experiment was conducted under stable-phase settings to ensure precision. Prior to taking the measurements, the engine was operated for a duration of 15 minutes to ensure stability. In order to verify accuracy, every experiment was replicated three times. The output results are fed into the “Engine soft” analysis software to perform real-time assessment and monitoring of combustion pressure and crank angle.
Table 2 Testing engine details
Factors |
Specifications |
Engine make |
Kirloskar |
Model |
TV 1 |
No. of cylinder |
1 |
No. of strokes |
4 |
Fuel |
Diesel |
Rated power |
5.2 kW@1500 rpm |
Cylinder diameter |
87.5 mm |
Stroke length |
110 mm |
Compression ratio |
17.5:1 |
Ignition type |
Compression ignition |
Cooling |
Water-cooled |
Loading type |
Eddy current dynamometer |
Combustion chamber injector nozzle |
Three holes with 0.3 mm diameter each |
Combustion chamber shape |
Hemi spherical shape |
Injection timing |
23° bTDC |
Injection pressure |
200 bar |
 |
| Fig. 2 Photographic view of the engine setup. | |
The accuracy of the experimental results was verified through error analysis using the error propagation method based on Taylor's theorem. The percentage uncertainties for key physical parameters, such as BTE, BSFC, and emission metrics including smoke, NOx, HC, and CO, were calculated based on the percentage uncertainties of the respective instruments. The total uncertainty in the experimental measurements was found to be ±2.38%. The uncertainty details of the instruments used in the experiment are given in Table 3. An estimation of the proportion of experimental uncertainty is obtained using eqn (1), as illustrated below.
|
 | (1) |
Table 3 Uncertainties of measured quantities
S. No. |
Measured quantities |
Uncertainties (%) |
1 |
BP (brake power) |
0.2 |
2 |
SFC (specific fuel consumption) |
1 |
3 |
BTE (brake thermal efficiency) |
1 |
4 |
EGT (exhaust gas temperature) |
0.15 |
5 |
CO (carbon monoxide) |
0.2 |
6 |
HC (hydrocarbon) |
0.2 |
7 |
NO (nitrogen oxide) |
0.2 |
8 |
S (smoke opacity) |
1 |
9 |
CO2 (carbon dioxide) |
1 |
10 |
P (cylinder pressure) |
1 |
11 |
N (speed) |
0.5 |
12 |
L (load) |
0.5 |
3. Results and discussion
3.1. Brake specific fuel consumption
The analysis of Fig. 3 reveals that the specific fuel consumption rate diminishes as the load conditions increase, attributable to the efficient conversion of fuel into brake power output. The elevated density and viscosity of the waste plastic oil blends, specifically those at 30 and 40, resulted in a brake specific fuel consumption (BSFC) that was 5.96% and 9.22% higher, respectively, in comparison to diesel fuel. The combustion properties of a 40% blend of WPO are improved by adding 5% butanol, which has a higher energy content and cetane number.13–15 This modification results in a reduction of brake-specific fuel consumption (BSFC) by 4.69% when compared to conventional diesel fuel. In order to optimize the combustion process and elevate the performance of WPO biodiesel, the introduction of hydrogen in conjunction with air was examined at flow rates of 8 and 10 lpm. The findings further demonstrated that the elevated heating value of hydrogen at flow rates of 8 lpm and 10 lpm, combined with 40% waste plastic oil (WPO) and 5% n-butanol, led to a reduction in brake specific fuel consumption (BSFC) by 9.74% and 20.89%, respectively, when compared to diesel fuel. By supplying hydrogen into the intake manifold, better mixing with air is ensured, while the addition of n-butanol to the fuel enhances spray characteristics. This approach effectively maintains an optimal air–fuel mixture in the combustion chamber, leading to improved combustion performance.
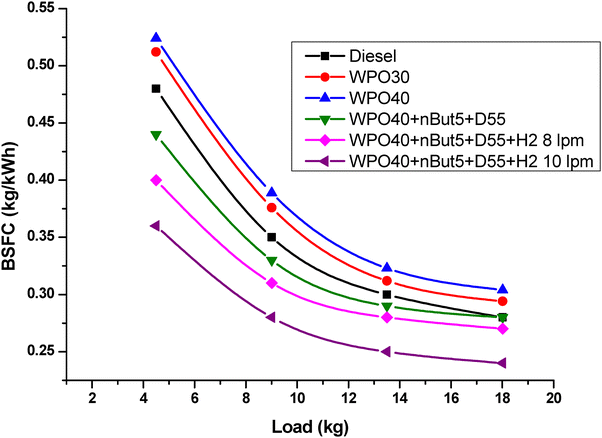 |
| Fig. 3 BSFC at different load conditions. | |
3.2. Brake thermal efficiency
Fig. 4 demonstrates that waste cooking oil biodiesel has a lower BTE than diesel fuel, attributable to its reduced calorific value, elevated viscosity, and resultant increase in SFC.5,7 The incorporation of improved volatility and reduced viscosity in n-butanol blends influences fuel atomization inside the combustion chamber, while their increased oxygen content and absence of aromatic hydrocarbons facilitate complete combustion.47 The incorporation of a 5% butanol mix with biodiesel (WPO40 + nBut5 + D55) resulted in a 3.154% enhancement in efficiency across all loads compared to diesel fuel. The introduction of hydrogen, which possesses four times the calorific value of diesel fuel,30 into the combustion chamber enhances the combustion process in conjunction with a butanol blend, resulting in an enhanced braking thermal efficiency of waste plastic biodiesel compared to diesel fuel. The provision of increased hydrogen at 8 lpm and 10 lpm elevates the brake thermal efficiency by an average of 4.334% and 8.222%, respectively, in comparison to diesel fuel.
 |
| Fig. 4 BTE at different load conditions. | |
3.3. Cylinder pressure
Fig. 5 depicts the fluctuation of cylinder pressure for several tested fuels at maximum load conditions. The findings indicate that the waste plastic biodiesel mixes of 30 and 40, yielding 2.79 and 4.26 bar, respectively, reduced the maximum pressure relative to diesel fuel. The elevated density, reduced calorific value, and poor volatility of waste plastic oil result in decreased combustion efficiency.17 The incorporation of n-butanol rectified this inadequacy by achieving a peak cylinder pressure of 70.77 bar, which is nearer to the maximum pressure of diesel fuel at 70.96 bar. The introduction of enriched hydrogen at flow rates of 8 and 10 lpm resulted in pressure increases of 1.28 bar and 1.66 bar, respectively, compared to diesel fuel. This was due to hydrogen's reduced ignition delay by its higher ignition energy and wide flammability limits; thereby, the combustion starts closer to the top dead center of the combustion chamber, increasing cylinder pressure.
 |
| Fig. 5 Cylinder pressure at maximum load conditions. | |
3.4. Heat release rate
Fig. 6 shows that the heat release rate of the WPO 30 and 40 blends was 8.72 and 11.45 kJ per degree CA lower than that of diesel fuel, primarily due to their higher aromatic content and lower cetane number, which in turn affected the combustion dynamics. The higher oxygen content of the butanol blend breaks the aromatic and long hydrocarbon chain of waste plastic biodiesel.16,18 Therefore, the addition of butanol improved the HRR, bringing its value closer to diesel fuel. Furthermore, to enhance the WPO combustion process, the supply of 8 and 10 lpm-enriched H2 increased HRR by 3.03 and 3.67 kJ per degree CA, respectively, compared to diesel fuel. The oxygen already present in butanol and the faster burn rate of hydrogen synergize to enhance the premixed combustion. The premixed phase burns more of the fuel, resulting in a lower HRR in the diffusion phase.
 |
| Fig. 6 Heat release rate under maximum load conditions. | |
3.5. Carbon monoxide (CO)
Fig. 7 demonstrates that diesel fuel emitted higher levels of carbon monoxide across all load conditions compared to the other tested fuels. The utilization of a blend of waste plastic oil at different concentrations (30%, and 40%) resulted in significant reductions in CO emissions. The reductions in emissions compared to diesel fuel were 21.06% and 28.86%, respectively. This achievement resulted from the elevated oxygen levels and high volatility of plastic oil, which improve fuel atomization and vaporization, leading to more complete combustion of the air-fuel mixture. The incorporation of n-butanol with waste plastic biodiesel decreased CO emissions by an average of 31.51% across all loads in comparison to diesel fuel. The reduced viscosity and lower boiling point facilitate expedited evaporation and air-fuel mixing.16 However, adding n-butanol diminishes the calorific value of the blend, perhaps necessitating a greater fuel supply for the same power output. Consequently, the increased energy content from hydrogen enrichment within the combustion chamber mitigated this issue and facilitated complete combustion, as evidenced by the introduction of 8 and 10 lpm of H2 into the combustion chamber, which resulted in a reduction of CO emissions by 39.47% and 43.84%, respectively, in comparison to diesel fuel.
 |
| Fig. 7 Carbon monoxide emissions at different loads. | |
3.6. Unburned hydrocarbon (UBHC)
Fig. 8 depicts the emission of unburned hydrocarbons from several tested fuels. Subsequent scientific investigation indicated that the diesel fuel demonstrated elevated hydrocarbon emissions relative to the other fuels examined. When compared to diesel fuel, the incorporation of waste plastic oil blends at varied proportions resulted in considerable reductions in UBHC emissions. Specifically, blend concentrations of 30% and 40% lowered the emissions by 6.4 and 14.2 ppm, respectively. This was due to plastic oil's lower volatility nature that led to improved combustion efficiency and reduced fuel evaporations. Adding n-butanol as a catalyst is needed to improve full combustion because it raises the fuel's cetane value by lowering the amount of aromatic and long-chain hydrocarbons that are present.17 The findings demonstrated that a 5% incorporation of n-butanol decreased the UBHC by 27.8 ppm in comparison to diesel fuel. The augmentation of hydrogen enrichment elevates in-cylinder temperatures, facilitating the oxidation of hydrocarbons. This facilitates the combustion of heavier and longer-chain hydrocarbons that may not fully ignite in waste plastic biodiesel and butanol blends. It was proven that the introduction of 8 and 10 lpm of H2 into the combustion chamber resulted in a reduction of UBHC emissions by 45.44 ppm and 57.8 ppm, respectively, in comparison to diesel fuel.
 |
| Fig. 8 Unburned hydrocarbon emissions at different loads. | |
3.7. Nitrogen oxide (NOx)
Fig. 9 illustrates a proportional increase in nitrogen oxide emissions corresponding to the rise in load for all tested fuels. The rise in NOx emissions is attributable to elevated combustion temperatures and adiabatic flame temperatures. The findings demonstrated that the use of waste plastic oil blends at concentrations of 30% and 40% resulted in increased NOx emissions of 102.2 ppm and 153 ppm, respectively, when compared to diesel fuel. Certain factors contribute to the increased presence of oxygen and fuel-bound nitrogen in waste plastic oil. The incorporation of n-butanol additives with WPO results in a 168.8 ppm increase in NOx emissions relative to diesel fuel. The delay in ignition caused by the n-butanol blend is attributed to its heat absorption during vaporization, resulting in rapid fuel combustion and an increase in combustion pressure and temperature.18 The supply of enriched hydrogen increases the combustion temperature due to its high flame propagation speed and energy content, resulting in an improved combustion rate. The addition of 8 and 10 lpm enriched hydrogen led to increases in NOx emissions of 185.6 and 236 ppm, respectively, when compared to diesel fuel.
 |
| Fig. 9 Nitrogen oxide emissions at different loads. | |
3.8. Smoke opacity
Fig. 10 illustrates the smoke opacity emissions associated with different tested fuels. It is evident that increasing cylinder pressure during maximum load conditions leads to a corresponding increase in smoke emission compared to lower loads. In comparison to diesel fuel, the application of various blends, namely WPO 30 and WPO 40, led to markedly reduced smoke opacity emissions. The reductions in smoke opacity were quantified at 5.10% and 7.70% for each respective blend in comparison to diesel fuel. The incorporation of a 5% n-butanol blend with WPO resulted in a 10.20% reduction in smoke opacity emissions. The elevated oxygen content of n-butanol contributed to a decrease in the formation of soot precursors, such as polycyclic aromatic hydrocarbons (PAHs), during combustion, thus facilitating more complete combustion. The incorporation of enriched hydrogen into an n-butanol and WPB blend can markedly decrease smoke opacity emissions by enhancing combustion efficiency, minimizing soot formation, and utilizing the clean-burning characteristics of hydrogen. The findings demonstrated that the incorporation of 8 and 10 lpm-enriched hydrogen resulted in reductions in smoke opacity emissions of 12.80% and 14.70%, respectively, relative to diesel fuel.
 |
| Fig. 10 Smoke opacity emissions at different loads. | |
4. Comparison of results with other previous work
A 20% blend of plastic biodiesel achieved a maximum brake thermal efficiency (BTE) of 34.4% at a compression ratio (CR) of 15.5 under high engine load, whereas neat diesel fuel exhibited the lowest brake-specific fuel consumption (BSFC) of 738.29 g kW−1 h−1 at a CR of 16.5 under similar conditions.48 The BTE of neat plastic oil was 6.90% lower than that of neat diesel. However, plastic oil blends such as PO25, PO50D50, PO75, and PO100 reduced CO2 emissions by 5.60%, 10.87%, 19.06%, and 21.81%, respectively, while also decreasing smoke opacity by 2.05%, 2.79%, 3.11%, and 4.47%.10 The use of plastic oil resulted in a 2% reduction in BTE and an increase in specific fuel consumption (SFC) by 0.281 kg kW−1 h−1, with no significant improvement in pollution reduction.49 A 25% plastic oil blend combined with 20% exhaust gas recirculation (EGR) produced a maximum BTE of 31%, while reducing NOx emissions to 18 g kW−1 h−1 and HC emissions to 0.4 g kW−1 h−1, but increasing smoke opacity to 59% under maximum load conditions.50 The use of neat plastic oil further reduced the engine's thermal efficiency to 27.35%.8 A PO30% blend achieved a maximum BTE of 30.74% and a BSFC of 2.2 kg kW−1 h−1.51 The WPO20 blend with 100 ppm ZnO nanoparticles reduced smoke emissions by 11.86%, CO by 5.7%, unburned hydrocarbons (UHC) by 28%, and NOx by 14.93%, while also enhancing BTE by 2.47% at maximum load.11 The WPO20 + Water 10 + H2 fuel blend achieved the highest BTE of 32.86%, reduced BSFC by up to 46.60%, controlled NOx emissions by 19%, and lowered CO, HC, and smoke opacity by 0.04%, 6 ppm, and 2%, respectively, compared to diesel fuel.52
5. Conclusion
The performance of waste plastic biodiesel blends at concentrations of 30% and 40% was observed to be lower than that of conventional diesel fuel. However, the addition of 5% n-butanol to waste plastic oil (WPO) resulted in improved performance and combustion characteristics compared to diesel. Furthermore, hydrogen enrichment at flow rates of 8 and 10 liters per minute (lpm) further enhanced the performance, combustion, and emission characteristics of waste plastic biodiesel. The results of the optimized blends, 40WPO + nBut5 + H2 8 lpm and 40WPO + nBut5 + H2 10 lpm, were compared with diesel fuel, demonstrating the following improvements:
Brake-specific fuel consumption (BSFC) decreased by 9.74% and 20.89%, respectively.
Brake thermal efficiency (BTE) increased by 4.334% and 8.222%.
At maximum engine load, the peak pressure increased by 1.28 bar and 1.66 bar, while the heat release rate improved by 3.03 kJ per °CA and 3.67 kJ per °CA, respectively.
Carbon monoxide (CO) emissions were reduced by 39.46% and 43.84% by volume.
Unburned hydrocarbon (UHC) emissions decreased by 45.49 ppm and 57.8 ppm.
Nitrogen oxide (NOx) emissions increased by 185.6 ppm and 236 ppm.
Smoke opacity emissions decreased by 12.80% and 14.70%.
These findings highlight the potential of blending waste plastic-derived fuels with renewable additives to enhance engine performance and contribute to sustainable energy solutions. The optimized blend 40WPO + nBut5 + H2 10 lpm exhibited superior results, demonstrating its viability as a sustainable, cost-effective, and environmentally friendly alternative to fossil fuels. The utilization of WPO as a fuel alternative not only reduces plastic waste and associated pollution but also lowers harmful emissions while promoting a circular economy, thereby positioning WPO as a viable waste-to-energy solution.
Despite these promising results, several challenges were encountered, including the optimization of pyrolysis conditions to improve biodiesel yield and quality, variations in low-density polyethylene (LDPE) feedstock, and the availability and cost of n-butanol.
Future research will focus on evaluating additional plastic waste sources, optimizing pyrolysis parameters for broader applicability, and exploring alternative hydrogen delivery methods to enhance fuel-air mixing and combustion efficiency.
Author contributions
Ganesan S – conceptualization, writing – original draft, investigation, formal analysis, supervised the work, validation. Thiruselvam K – formal analysis, methodology, review & editing, software, resources; Sravanth Chandaka – conceptualization, writing – original draft, investigation; Jayavelu S – review & editing, software, resources.
Ethical approval
I (on behalf of the authors) declare that the work is our own original work, which has not been previously published elsewhere. The paper is not currently being considered for publication elsewhere.
Consent to publish
I (on behalf of the authors) give my consent for identifiable details, which can include photograph(s) and/or videos and/or case history and/or details within the text to be published in the above Journal and Article.
Declaration of generative AI and AI-assisted technologies in the writing process statement
During the preparation of this work, the authors used QuillBot as AI-assisted technology in order to improve readability and language. After using this tool/service, the authors reviewed and edited the content as needed and take full responsibility for the content of the publication.
Data availability
The data supporting this article have been included as part of the ESI.†
Conflicts of interest
The authors have no competing interests to declare that are relevant to the content of this article.
Acknowledgements
The authors are thankful to Vel Tech Rangarajan Dr. Sagunthala R & D Institute of Science and Technology for providing financial support in the form of RDF to carry out the research work (Sanctioned Order: VTU RDF (FY23-24)-12).
References
- S. Thiyagarajan, V. Edwin Geo, L. Jesu Martin and B. Nagalingam, Comparative analysis of various methods to reduce CO2 emission in a biodiesel fueled CI engine, Fuel, 2019, 253, 146–158, DOI:10.1016/j.fuel.2019.04.163.
- K. A. Abed, M. S. Gad, A. K. El Morsi, M. M. Sayed and S. Abu Elyazeed, Effect of biodiesel fuels on diesel engine emissions, Egypt. J. Pet., 2019, 28, 183–188, DOI:10.1016/j.ejpe.2019.03.001.
- Biofuels Market Size, Share & Growth Analysis Report for the years 2023 to 2033, https://www.precedenceresearch.com/biofuels-market.
- A. Suhara, Karyadi, S. G. Herawan, A. Tirta, M. Idris, M. F. Roslan, N. R. Putra, A. L. Hananto and I. Veza, Biodiesel Sustainability: Review of Progress and Challenges of Biodiesel as Sustainable Biofuel, Clean Technol., 2024, 6, 886–906, DOI:10.3390/cleantechnol6030045.
- D. Dillikannan, M. V. De Poures, G. Kaliyaperumal, A. P. Sathiyagnanam, R. K. Babu and N. Mukilarasan, Effective utilization of waste plastic oil/n-hexanol in an off-road, unmodified DI diesel engine and evaluating its performance, emission, and combustion characteristics, Energy Sources, Part A, 2020, 42(11), 1375–1390, DOI:10.1080/15567036.2019.1604853.
- C. Mohanraj, T. Senthilkumar and M. Chandrasekar, A review on conversion techniques of liquid fuel from waste plastic materials, Int. J. Energy Res., 2017, 41, 1534–1552, DOI:10.1002/er.3720.
- M. Chandran, S. Tamilkolundu and C. Murugesan, Characterization studies: waste plastic oil and its blends, Energy Sources, Part A, 2019, 1–11, DOI:10.1080/15567036.2019.1587074.
- V. K. Kaimal and P. Vijayabalan, A detailed study of combustion characteristics of a DI diesel engine using waste plastic oil and its blends, Energy Convers. Manage., 2015, 105, 951–956, DOI:10.1016/j.enconman.2015.08.043.
- S. Maithomklang, K. Wathakit, E. Sukjit, B. Sawatmongkhon and J. Srisertpol, Utilizing waste plastic bottle-based pyrolysis oil as an alternative fuel, ACS Omega, 2022, 7, 20542–20555, DOI:10.1021/acsomega.1c07345.
- S. Pal, A. Kumar, M. A. Ali, N. K. Gupta, S. Pandey, P. K. Ghodkhe, S. Bhurat, T. Alam, S. M. Eldin and D. Dobrota, Experimental evaluation of diesel blends mixed with municipal plastic waste pyrolysis oil on performance and emission characteristics of CI engine, Case Stud. Therm. Eng., 2023, 47, DOI:10.1016/j.csite.2023.103074.
- A. Suhel, N. A. Rahim, M. R. Rahman, K. A. Ahmad, U. Khan, Y. H. Teoh and N. Z. Abidin, Impact of ZnO nanoparticles as additive on performance and emission characteristics of a diesel engine fueled with waste plastic oil, Heliyon, 2023, 9, e14782, DOI:10.1016/j.heliyon.2023.e14782.
- S. D. Sharuddin, F. Abnisa, W. M. Daud and M. K. Aroua, A review on pyrolysis of plastic wastes, Energy Convers. Manage., 2016, 115, 308–326, DOI:10.1016/j.enconman.2016.02.037.
- A. K. Singh, H. S. Pali and M. M. Khan, Experimental investigations of CI engine performance using ternary blends of n-butanol/biodiesel/diesel and n-octanol/biodiesel/diesel, Arch. Thermodyn., 2024, 45(1) DOI:10.24425/ather.2024.150443.
- A. G. Mustayen, M. G. Rasul, X. Wang, M. A. Hazrat, M. I. Jahirul and M. Negnevitsky, Plastic-made diesel (PMD) from pyrolysis via vacuum distillation process- A waste recycling fuel to diesel engine performance and emissions improvement, J. Energy Inst., 2023, 107, 101198, DOI:10.1016/j.joei.2023.101198.
- L. Tipanluisa, N. Fonseca-González, N. Flores and A. Ayala, Butanol and selective catalytic reduction of gaseous emissions in a heavy-duty diesel engine, Fuel, 2024, 373, 132236, DOI:10.1016/j.fuel.2024.132236.
- F. Zhou, R. Yan, G. Li and W. Han, Experimental study on the effects of n-butanol and n-propanol on the spray and combustion characteristics of
diesel/biodiesel blends in a constant volume combustion chamber, Renewable Energy, 2024, 235, 121374, DOI:10.1016/j.renene.2024.121374.
- A. Sengupta, S. Biswas, R. Banerjee and N. Biswas, Enhancing Combustion Stability in Biodiesel Engines: The Plausibility of n-Butanol Injection Phasing and Reactivity-Controlled Compression Ignition–An Experimental Endeavour, Fuel, 2024, 371, 131978, DOI:10.1016/j.fuel.2024.131978.
- S. Kumaravel, C. G. Saravanan, V. Raman, M. Vikneswaran, J. Sasikala,J., S. Femilda Josephin, S. Ali Alharbi, A. Pugazhendhi, H. F. Oztop and E. Geo Varuvel, Experimental investigations on in-cylinder flame and emission characteristics of butanol-gasoline blends in SI engine using combustion endoscopic system, Therm. Sci. Eng. Prog., 2024, 49, 102449, DOI:10.1016/j.tsep.2024.102449.
- Q. Zhang, Z. Hu, J. Zhu, P. Zhang and X. Lu, Experimental and numerical study on mixture concentration distribution and ignition characteristics of biodiesel/n-butanol dual-fuel spray impingement in inert and combustion environments, Fuel, 2024, 368, 131592, DOI:10.1016/j.fuel.2024.131592.
- W. Zhao, Y. Zhang, G. Huang, Z. He, Y. Qian and X. Lu, Experimental study of butanol/biodiesel dual-fuel combustion in intelligent charge compression ignition (ICCI) mode: A systematic analysis at low load, Fuel, 2021, 287, 119523, DOI:10.1016/j.fuel.2020.119523.
- M. Feroskhan and N. Gobinath, Experimental study of butanol blending in biogas-biodiesel fueled CI engine under dual fuel, RCCI and HCCI combustion modes, Case Stud. Therm. Eng., 2024, 58, 104349, DOI:10.1016/j.csite.2024.104349.
- B. Waluyo, Y. A. Fatimah, I. Habibi, F. D. Pertiwi, M. Setiyo and E. Marlina, Enhancing Biodiesel-Methanol blends with 1-Butanol for stable and efficient fuels, Fuel, 2024, 361, 130667, DOI:10.1016/j.fuel.2023.130667.
- G. Labeckas, S. Slavinskas and T. Mackevičius, Performance and emissions of a diesel engine operating with renewable binary biodiesel-n-butanol biofuel blends, 2020 DOI:10.22616/ERDev.2020.19.TF342.
- P. Dimitriou and T. Tsujimura, A review of hydrogen as a compression ignition engine fuel, Int. J. Hydrogen Energy, 2017, 42, 24470–24486, DOI:10.1016/j.ijhydene.2017.07.232.
- L. Wang, D. Liu, Z. Yang, H. Li, L. Wei and Q. Li, Effect of H2 addition on combustion and exhaust emissions in a heavy-duty diesel engine with EGR, Int. J. Hydrogen Energy, 2018, 43, 22658–22668, DOI:10.1016/j.ijhydene.2018.10.104.
- T. Anand and S. Debbarma, Influence of Fuel Injection Timing and Hydrogen Enrichment on Waste Plastic Oil: Performance, Combustion, and Emissions Analysis, Defect Diffus. Forum Series, 2024, 433, 85–90, DOI:10.4028/p-px49ib.
- S. Sarıdemir, F. Polat and Ü. Ağbulut, Improvement of worsened diesel and waste biodiesel fuelled-engine characteristics with Hydrogen enrichment: A deep discussion on combustion, performance, and emission analyses, Process Saf. Environ. Prot., 2024, 184, 637–649, DOI:10.1016/j.psep.2024.02.018.
- R. Juknelevičius, A. Rimkus, S. Pukalskas and J. Matijošius, Research of performance and emission indicators of the compression-ignition engine powered by hydrogen - Diesel mixtures, Int. J. Hydrogen Energy, 2019, 44, 10129–10138, DOI:10.1016/j.ijhydene.2018.11.185.
- H. Serin and Ş. Yıldızhan, Hydrogen addition to tea seed oil biodiesel:Performance and emission characteristics, Int. J. Hydrogen Energy, 2018, 43, 18020–18027, DOI:10.1016/j.ijhydene.2017.12.085.
- V. T. Vimalananth, M. S. Panithasan and G. Venkadesan, Investigating the effects of injection and induction modes of hydrogen addition in a CRDI pilot dieselfuel engine with exhaust gas recirculation, Int. J. Hydrogen Energy, 2022, 47, 22559–22573, DOI:10.1016/j.ijhydene.2022.05.043.
- T. Anand and S. Debbarma, Experimental investigation of fuel injection timing effects on a CRDI diesel engine running on hydrogen-enriched waste plastic oil, Int. J. Hydrogen Energy, 2024, 57, 1051–1069, DOI:10.1016/j.ijhydene.2024.01.052.
- K. Muthukumar and G. Kasiraman, Utilization of fuel energy from single-use Low-density polyethylene plastic waste on CI engine with hydrogen enrichment – An experimental study, Energy, 2024, 289, 129926, DOI:10.1016/j.energy.2023.129926.
- A. Sanyal and A. K. Choudhary, Environmental impact of waste plastic oil and hydrogen-enriched diesel engines: A comprehensive review on performance, combustion, and emissions, J. Renewable Sustainable Energy, 2024, 16, 052703, DOI:10.1063/5.0230798.
- D. Barik, et al., Exploration of the dual fuel combustion mode on a direct injection diesel engine powered with hydrogen as gaseous fuel in port injection and diesel-diethyl ether blend as liquid fuel, Int. J. Hydrogen Energy, 2024, 52, 827–840, DOI:10.1016/j.ijhydene.2023.06.083.
- P. Sharma, A. Chhillar, Z. Said, Z. Huang, V. N. Nguyen, P. Q. P. Nguyen and X. P. Nguyen, Experimental investigations on efficiency and instability of combustion process in a diesel engine fueled with ternary blends of hydrogen peroxide additive/biodiesel/diesel, Energy Sources, Part A, 2022, 44(3), 5929–5950, DOI:10.1080/15567036.2022.2091692.
- K. Thiruselvam, S. Senthil and S. Murugapoopathi, et al., Sustainable engine transformation: assessing hydrogen enrichment and CeO2 nanoparticle effects on neat palm biodiesel-fueled CI engines, Environ. Dev. Sustainable, 2024 DOI:10.1007/s10668-024-05051-0.
- K. Thiruselvam, S. Murugapoopathi, T. Ramachandran and K. T. Amesho, Hydrogen-enriched palm biodiesel as a potential alternative fuel for diesel engines: Investigating performance and emission characteristics and mitigation strategies for air pollutants, Int. J. Hydrogen Energy, 2023, 48, 30974–30984, DOI:10.1016/j.ijhydene.2023.04.256.
- T. Sathish, et al., Waste to fuel: Synergetic effect of hybrid nanoparticle usage for the improvement of CI engine characteristics fuelled with waste fish oils, Energy, 2023, 275, 127397, DOI:10.1016/j.energy.2023.127397.
- N. K. Ndiaye, N. S. A. Derkyi and E. Amankwah, Pyrolysis of plastic waste into diesel engine-grade oil, Sci. Afr., 2023, 21, e01836, DOI:10.1016/j.sciaf.2023.e01836.
- R. K. Singh, et al., Waste plastic to pyrolytic oil and its utilization in CI engine: Performance analysis and combustion characteristics, Fuel, 2020, 262, 116539, DOI:10.1016/j.fuel.2019.116539.
- S. Padmanabhan, K. Giridharan and B. Stalin, et al., Energy recovery of waste plastics into diesel fuel with ethanol and ethoxy ethyl acetate additives on circular economy strategy, Sci. Rep., 2022, 12, 5330, DOI:10.1038/s41598-022-09148-2.
- M. Gwalwanshi, et al., A review on butanol properties, production and its application in internal combustion engines, Mater. Today: Proc., 2022, 62(Part 12), 6573–6577, DOI:10.1016/j.matpr.2022.04.573.
- K. Thiruselvam, et al., Hydrogen-enriched palm biodiesel as a potential alternative fuel for diesel engines: Investigating performance and emission characteristics and mitigation strategies for air pollutants, Int. J. Hydrogen Energy, 2023, 48, 30974–30984, DOI:10.1016/j.ijhydene.2023.04.256.
- T. Krishnamoorthi, et al., Synergistic effect of nano additives for combustion and emission mitigation in a hydrogen-enriched CI engine using cocos nucifera oil methyl ester, Int. J. Hydrogen Energy, 2025, 109, 1253–1265, DOI:10.1016/j.ijhydene.2025.02.143.
- M. Nour, A. M. A. Attia and S. A. Nada, Combustion, performance and emission analysis of diesel engine fuelled by higher alcohols (butanol, octanol and heptanol)/diesel blends, Energy Convers. Manage., 2019, 185, 313–329, DOI:10.1016/J.ENCONMAN.2019.01.105.
- A. Sengupta, S. Biswas and R. Banerjee, Stratified injection strategies: Deciphering the emission and combustion synergies of n-butanol/diesel blends in single cylinder engines, Fuel, 2024, 378, 132939, DOI:10.1016/j.fuel.2024.132939.
- A. Mohite, et al., Optimization of the pilot fuel injection and engine load for an algae biodiesel - hydrogen run dual fuel diesel engine using response surface methodology, Fuel, 2024, 357, 129841, DOI:10.1016/j.fuel.2023.129841.
- U. Rajak, et al., Experimental investigation of performance, combustion and emission characteristics of a variable compression ratio engine using low-density plastic pyrolyzed oil and diesel fuel blends, Fuel, 2022, 319, 123720, DOI:10.1016/j.fuel.2022.123720.
- V. Chintala, et al., A comparative assessment of single cylinder diesel engine characteristics with plasto-oils derived from municipal mixed plastic waste, Energy Convers. Manage., 2018, 166, 579–589, DOI:10.1016/j.enconman.2018.04.068.
- J. Jaiganesh, R. Prakash and M. Gowthama Krishnan, Exploring the synergistic potential of prosopis juliflora and waste plastic oil biodiesel through investigation of performance, combustion, and emission in a CRDI engine: A shift towards sustainable fuel, Process Saf. Environ. Prot., 2024, 184, 720–735, DOI:10.1016/j.psep.2024.02.036.
- V. Hariram, S. Seralathan, K. Bhutoria and H. H. Vora, Experimental study on combustion and performance characteristics in a DI CI engine fuelled with blends of waste plastic oil, Alexandria Eng. J., 2018, 57, 2257–2263, DOI:10.1016/j.aej.2017.09.001.
- E. Rama Krishna Reddy and M. K. Pal, Influence of hydrogen induction in a CRDI engine fuelled with water emulsified waste plastic oil blend, Int. J. Hydrogen Energy, 2024, 49, 1282–1293, DOI:10.1016/j.ijhydene.2023.09.025.
|
This journal is © The Royal Society of Chemistry 2025 |
Click here to see how this site uses Cookies. View our privacy policy here.