DOI:
10.1039/C9NR01678C
(Paper)
Nanoscale, 2019,
11, 8458-8463
Lysosome-targeted carbon dots for ratiometric imaging of formaldehyde in living cells†
Received
24th February 2019
, Accepted 3rd April 2019
First published on 4th April 2019
Abstract
Formaldehyde (FA) is involved in many biological processes and is closely connected with many diseases including Alzheimer's disease and cancer. Therefore, methods for sensitive and selective detection of FA in living cells are highly demanded. As a new class of carbon nanomaterials, carbon dots (CDs) have attracted great attention owing to their robust photostability, good biocompatibility and environmental friendliness. In this manuscript, the first lysosome-targeted CDs for ratiometric fluorescence detection of FA were efficiently prepared from dexamethasone and 1,2,4,5-tetraaminobenzene through the microwave-assisted hydrothermal method. These CDs show highly selective and sensitive sensing ability towards FA with fast response and great changes of ratio values. The CDs exhibit robust photostability and good biocompatibility and were successfully employed in ratiometric fluorescence bioimaging of FA fluctuations in lysosomes of living cells, which demonstrates their great practicability in FA-related bioanalysis and biological studies.
Introduction
Formaldehyde (FA) is a notorious environmental toxin and carcinogen.1 Overloading of FA can severely damage the central nervous system and increase the risk of leukemia and brain cancer.2 At the same time, FA is an endogenously generated-reactive carbonyl species (RCS) by demethylase and oxidase enzymes, which is involved in many biological processes, such as one carbon metabolism and epigenetics, etc.3,4 The normal level of FA in the brains of healthy individuals is reported to be in the range of 0.2–0.4 mM and it plays important roles in human memory storage as well as retrieval through DNA demethylation cycles.5 Abnormal levels of FA are closely connected with many diseases, such as Alzheimer's disease,6 cancer,7 diabetes, heart disorder and chronic liver disease, etc.8,9 Therefore, assays for sensitive and selective detection of FA are highly demanded.
In recent years, many efforts have been devoted to the development of new assays for FA detection.10–23 Among them, fluorescence microscopy and fluorescence imaging attract more and more attention due to their inherent benefits including high sensitivity and selectivity non-invasive nature and spatiotemporal resolution. To date, a number of small molecular fluorescent probes for detection of FA have been reported based on FA-induced specific reactions, such as aza-Cope rearrangement and formimine and aminal forming reactions.24,25 Besides, imaging of FA in living animals has also been realized via positron emission tomography (PET)15 and chemiluminescence imaging.22 However, there still exist some limits, for example, small molecular fluorescent probes usually have poor photostability, and PET probes have a relatively high cost and radiation damage.26 Therefore, it is important to develop new methods to monitor FA fluctuations in living cells.
Carbon dots (CDs), as a new class of carbon nanomaterials, have attracted great attention in the area of photocatalysis, optoelectronics, drug delivery, biosensing and bioimaging owing to their robust photostability, good biocompatibility, low cost, and environmental friendliness.27–36 For instance, Ma et al. reported fluorescein and rhodamine modified CDs as a ratiometric fluorescent pH probe for intracellular pH imaging.27 Lin's group developed three types of CDs with red, green and blue fluorescence for multicolor cellular imaging.28 Yang et al. reported near-infrared emissive CDs and explored their applications in bioimaging of mice.29 Xu et al. reported selenium-doped CDs with reactive oxygen species-dependent reversible fluorescence and investigated their applications in intracellular free radical scavenging and imaging.33 After ten years of development, CDs have become one of the most powerful fluorescent materials in the detection and imaging of various biologically important species. Although FA plays many important roles in the human body, to the best of our knowledge, CD-based fluorescence imaging and monitoring of FA in living cells have not been realized yet.37 In this manuscript, we disclosed the rational design of a novel CD-based ratiometric fluorescent probe for the rapid detection of FA fluctuations in living cells. Interestingly, the CDs show excellent intrinsic lysosome targeting ability. As the dysmetabolism of FA in lysosomes is closely related to age-related cognitive impairment such as Alzheimer's disease, the lysosome targeting FA-response CDs reported here show great potential to be used in FA-related bioanalysis and biological studies.
Results and discussion
Rational design
Dexamethasone and 1,2,4,5-tetraaminobenzene (TAB) were selected as precursors due to their abundant carbonyl, alcohol, fluoro and amino groups which can undergo condensation and carbonization to afford CDs. The residue o-diamino group in the as-prepared CDs was expected to react quickly and selectively towards FA, leading to ratiometric fluorescence response to FA through altering the intramolecular charge transfer (ICT) process from the electron donating amino group to the electron withdrawing carbonyl group of CDs (Scheme 1).26 Thereby, the selective ratiometric detection of FA can be realized.
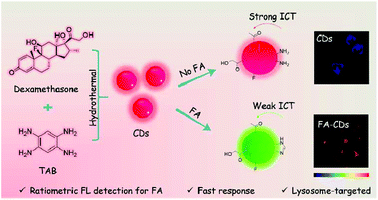 |
| Scheme 1 The first CD-based ratiometric fluorescent probe for intracellular imaging of FA was developed. The CDs can selectively and sensitively sense FA in lysosomes of living cells with fast response and great ratio values changes. | |
Synthesis and characterization of CDs
The synthesis of CDs was carried out via a microwave assisted hydrothermal method as shown in Scheme 1. After ultrafiltration, the CD solid powder was obtained through freeze-drying. The CDs were well characterized by TEM, FTIR and XPS.
The morphologies of CDs were investigated by TEM. The TEM image shows that the diameters of CDs mainly distribute from 1.5 to 3.8 nm with an average size of 2.7 nm (Fig. 1A). The surface group and chemical state of the as-prepared CDs were examined by FTIR and XPS, respectively. The absorption peak at 3401 and 3147 cm−1 in the FTIR spectrum (Fig. 1B) can be attributed to –NH2 and –OH vibrations. The absorption peaks at 1648 and 1399 cm−1 are assigned to C
O and C–O vibrations, respectively. The XPS measurement of CDs shows three obvious peaks at 284.1, 399.9, 530.7 and 684.4 eV, which are attributed to C 1s, N 1s, O 1s and F 1s (Fig. 1C). Fig. 1D shows the high-resolution XPS spectra of N 1s. The peaks at 399.6 eV for amide N and 401.3 eV for graphene N are observed clearly. These results demonstrated that the N atoms were doped in the CDs. The results fit well with the FTIR data and illustrate that the CD surface is equipped with –COOH, –OH and –NH2 as functional groups, which is consistent with our initial design.
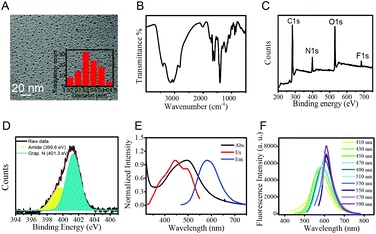 |
| Fig. 1 Characterization of CDs. (A) TEM image of the as-prepared CDs. Inset: Size distribution of CDs. (B) The FTIR spectrum of CDs. (C) The broad XPS spectrum of CDs. (D) High-resolution N 1s peaks of CDs. (E) UV-Vis absorption (black line), excitation (red line), and emission (blue line) spectra of CDs in aqueous solutions (50 μg mL−1). (F) Emission spectra of CDs with different excitation wavelengths (starting from 410 to 590 nm with a 20 nm increment). | |
Next, the optical properties of the as-prepared CDs were examined by UV-vis absorption and fluorescence spectroscopy. The CDs show a main absorption at 496 nm and red fluorescence at 585 nm with a broad excitation wavelength from 400 to 500 nm (Fig. 1E). The emission wavelength of CDs was observed to be dependent on the excitation wavelength (Fig. 1F). With the excitation wavelength increasing from 410 nm to 590 nm, the main fluorescence peaks red shift from 568 nm to 610 nm. The excitation-dependent emission properties of CDs could be attributed to the different energy levels resulting from the different conjugation degrees and distinct functional groups of CDs.38,39
Ratiometric fluorescence detection of FA
The fluorescence response of CDs to FA was examined by using time-dependent fluorescence and UV-vis spectra. The CDs (50 μg mL−1) showed strong fluorescence at 580 nm (QY = 2.01%, using rhodamine as a reference) in PBS buffer when excited at 405 nm. After being treated with 1 mM FA, the main fluorescence of CDs gradually faded away within 5 min and blue shifted to 500 nm (QY = 0.15%, using quinine sulfate as a reference) with a 5-fold enhancement of the ratio value of fluorescence intensity at 500 nm and 580 nm (Fig. 2A and B). The response time of CDs is notably fast (5 min), whereas the reported small molecule-based fluorescent probes usually required 0.5–3 hours to achieve a remarkable signal output. The fluorescence life-time before and after the reaction with FA is determined to be 1.99 and 2.27 ns, respectively (Fig. S1†). The response of CDs toward FA was also examined by time dependent UV-vis spectra. As shown in Fig. S2,† after the addition of 1 mM FA, the main absorption of CDs at 496 nm gradually decreases with time and blue shifts to 450 nm. Two well-defined isosbestic points were observed at 400 nm and 530 nm, which indicated the formation of new species. The hypsochromic shifts in both fluorescence and the UV-vis spectra indicate that the aforementioned ICT process from the electron-donating amino group to the electron-withdrawing carbonyl is weaker in the FA-CDs compared to the as-prepared CDs.
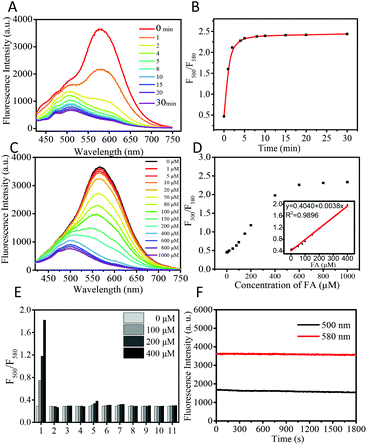 |
| Fig. 2 (A) The time-dependent fluorescence spectra changes of CDs (50 μg mL−1) toward 1 mM FA excited at 405 nm in PBS buffer (pH 7.4, 10 mM). (B) Fluorescence ratio changes of CDs in the presence of FA over time. (C) Fluorescence spectra of CDs in the presence of various amounts of FA (0, 1, 5, 10, 20, 50, 80, 100, 150, 200, 400, 600, 800 and 1000 μM), and spectra were recorded after the addition of FA for 5 min. (D) The relationship between I500/I580 and FA from 0 μM to 1000 μM. Inset: A linear plot was obtained in the concentration range of FA from 0 to 400 μM. (E) Fluorescence response of CDs (50 μg mL−1) upon addition of various biological reactive species (0, 100, 200, 400 μM), including (1) FA, (2) acrolein, (3) 4-hydroxynonenal, (4) acetaldehyde, (5) methylglyoxal, (6) pyruvate, (7) DHA, (8) H2O2, (9) Cys, (10) Hcy, and (11) GSH. (F) Fluorescence intensity of CDs at 580 nm and the reaction product from FA detection at 500 nm under the irradiation of 405 nm for 30 min. | |
Meanwhile, we examined the dynamic range of CDs toward FA. Upon addition of the incremental concentration of FA, the ratio value (fluorescence intensity at 500 nm/fluorescence intensity at 580 nm) of CDs gradually increased and showed a good linear relationship in the range of 0–400 μM (Fig. 2C and D). The detection limit was calculated to be 0.9 μM based on S/N = 3. These results indicate that CDs can detect FA in the biological range with great sensitivity.
As selectivity is an important feature, the selectivity experiments of CDs for FA were then conducted. As shown in Fig. 2E and Fig. S3,† CDs show no obvious fluorescence changes after the addition of various biological species, including amino acids (Lys, Arg, Tyr, Trp, Thr, Ser, Leu, Ile, His, Pro, Met, Gln, Glu, Asn, Asp, Phe, Val, Ala, and Gly), cations (Zn2+, Fe3+, Fe2+, Cu2+, K+, Ca2+, Na+, and Mg2+), reactive oxygen species (H2O2), small molecule biothiols (Cys, Hcy, and GSH), and RCS (acrolein, 4-hydroxynonenal, acetaldehyde, methylglyoxal, pyruvate and DHA). Only FA induced remarkable ratiometric fluorescence responses, demonstrating the high selectivity of the as-prepared CDs for FA over common biological species.
Furthermore, the effect of pH on the CDs was determined. The fluorescence of CDs is stable in the pH range of 4–8, and the CDs show remarkable ratiometric fluorescence response for FA in the pH range of 4–8 (Fig. S4†). Both CDs and the reaction product FA-CDs show great photostability. Under the irradiation of 405 nm for 30 min using a Xe lamp as the light source, the fluorescence intensity of CDs and FA-CDs at 580 nm and 500 nm remained at 98% and 97%, respectively (Fig. 2F). These results suggest that the CDs have great potential in monitoring FA in a complex environment and living systems.
Sensing mechanism
To study the sensing mechanism, the product of CDs with FA was separated and well characterized by FTIR (Fig. S5†). FA-CDs exhibited a clear signal of the imidazole group in IR spectra (1115 cm−1), whereas the CDs themselves show an intense signal of the amino group (1600 cm−1). These results suggested that after the reaction with FA, the amino group in CDs transformed into imidazole heterocycles. The average hydrodynamic diameter of CDs and FA-CDs was determined to be in the range of 10.5 ± 101.2 to 17.1 ± 299.6 (Fig. S10†) by dynamic light scattering. The zeta potential of the CDs changed from +1.17 mV to +0.24 mV after the reaction with FA (Fig. S11†), which might be attributed to the weaker basic effect of the imidazole group compared to the o-diamino group. Due to the weaker electron donating ability of the imidazole heterocycle as compared to that of the amino group, the ICT process in the FA-CDs is weaker than that in CDs, leading to the blue shift in the fluorescence and UV-vis spectra, which is in good agreement with our design concept.40,41
Intrinsic lysosome targeting ability of the CDs
Encouraged by the above results, we employed the CDs to monitor FA in living cells. The cytotoxicity of CDs was first examined by MTT assay, and the results suggested that CDs have minimal cytotoxicity to HeLa cells (Fig. S6†). Then, the subcellular imaging ability of CDs was investigated through colocalization experiments. HeLa cells were treated with CDs (30 μg mL−1, Fig. S7†) and a commercial lysosome indicator, Lyso-Traker Deep Red, for 15 min. Strong green and orange red fluorescence from CDs and purple (pseudo-color) fluorescence from Lyso-Traker Deep Red were clearly observed (Fig. 3). Pearson's colocalization coefficient was calculated to be 0.90. The fine merged image and high colocalization coefficient clearly showed that CDs could be specifically distributed in lysosomes. Lysosomes are important organelles and play vital roles in cellular degradation, autophagy, immunological stress and FA metabolism. A recent research study revealed that the dysmetabolism of FA in lysosomes is closely related to age-related cognitive impairment such as Alzheimer's disease.42 Thus, the detection of FA in lysosomes is very important to disclose more biological roles of FA. On the other hand, there are only a few CDs that can specifically image lysosomes, and most of them require post modification with lysosome targeting morpholine ligands. The intrinsic lysosome targeting CDs reported here without any post modification might be helpful to design more lysosome targeting CDs. The pH value is about 4.5–5.5 in lysosomes, which is lower than that of other organelles. Due to the acidotropic effect, lipophilic amines can acquire about 100-fold enrichment in the lysosomes.43,44 Small molecular probes,45,46 organosilica nanodots47 and carbon dots48 equipped with amine groups were reported to show intrinsic lysosome targeting ability. Thus, the intrinsic lysosome targeting ability should be attributed to the acidotropic effect of the amino group of the CDs. Moreover, CDs show great photostability as shown in Fig. 4, and no obvious difference of the fluorescence intensity of CD stained HeLa cells is observed for 10 min.
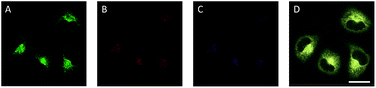 |
| Fig. 3 Fluorescence images of HeLa cells incubated with CDs (30 μg mL−1) (A, B) and Lyso-Traker Deep Red (0.1 μM) (C) at 37 °C for 15 min. The images were collected in the range of 450–550 nm (A) and 550–650 nm (B) with the excitation wavelength at 405 nm. The images were collected in the range of 650–740 nm (C) with the excitation wavelength at 638 nm. (D) Image of the merge channel (A, B, C, and bright field channel). Scale bar: 25 μm. The three emission channels were displayed with pseudo-color. | |
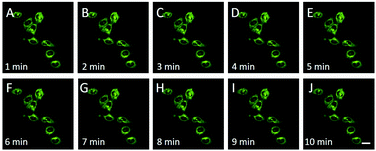 |
| Fig. 4 The overlay fluorescence images of HeLa cells after incubation in the medium containing CDs (30 μg mL−1) and then with a continuous scan of 10 min. A–J: 1–10 min. | |
Ratiometric fluorescence imaging of FA in living cells
The selective imaging of FA in living HeLa cells was achieved by CDs (Fig. 5 and Fig. S8†). After incubation with CDs for 10 min, HeLa cells showed strong fluorescence in both green and orange red channels. When HeLa cells were pretreated with 200 μM and 400 μM FA, the ratio value (green channel/orange red channel) increases with the concentration of FA. By contrast, when HeLa cells were pre-incubated with the FA scavenger, 200 μM sodium bisulfite (NaHSO3), the fluorescence ratio value of HeLa cells decreased obviously. The above results demonstrated that CDs could image both exogenous and endogenous FA in living cells.
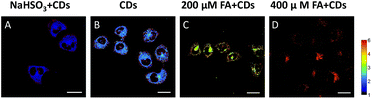 |
| Fig. 5 Ratio channel images of HeLa cells treated with CDs (30 μg mL−1) at 37 °C for 10 min in the presence of NaHSO3 (200 μM) or FA (200 μM or 400 μM). (A) HeLa cells were incubated with 200 μM NaHSO3 for 30 min and then CDs (30 μg mL−1) for 10 min. (B) HeLa cells were incubated with CDs (30 μg mL−1) for 10 min. (C) HeLa cells were pretreated with 200 μM FA for 20 min and then incubated with CDs (30 μg mL−1) for 10 min. (D) HeLa cells were incubated with 400 μM FA for 20 min and then incubated with CDs (30 μg mL−1) for 10 min. Images were acquired by Image J software. The color strip on the right shows the pseudo-color change. Scale bar: 20 μm. | |
Conclusions
In conclusion, lysosome-targeted CDs for ratiometric fluorescence detection of FA were developed based on the ICT sensing mechanism and FA-induced aminal heterocycle formation reaction. The CDs show great photostability and good biocompatibility. The CDs can selectively and sensitively sense FA with fast response and great ratio values changes. Furthermore, bioimaging studies demonstrated that these CDs can be employed in ratiometric fluorescence monitoring of FA fluctuations in lysosomes of living cells. The rationally designed concept introduced in this work is expected to be widely adopted to construct various CDs for FA or other important biological species detection.
Experimental section
Materials and apparatus
Formaldehyde (FA) was purchased from Sinoreagent. Acrolein, 4-hydroxynonenal, methylglyoxal, and pyruvate were bought from J&K chemical. DHA, glutathione (GSH), cysteine (Cys), homocysteine (Hcy), lysine (Lys), arginine (Arg), tyrosine (Tyr), tryptophan (Trp), threonine (Thr), serine (Ser), leucine (Leu), isoleucine (Ile), histidine (His), proline (Pro), methionine (Met), glutamine (Gln), glutamic acid (Glu), asparagine (Asn), aspartic acid (Asp), phenylalanine (Phe), valine (Val), alanine (Ala) and glycine (Gly) were obtained from Sigma-Aldrich. Acetaldehyde, H2O2, ascorbic acid (AA), glucose, and metal ions (Zn2+, Fe3+, Fe2+, Cu2+, K+, Ca2+, Na+, and Mg2+) were purchased from Aladdin. The PBS buffer (10 mM, pH 7.4) was used for sensing of FA. All chemicals were used without further purification.
UV-vis absorption spectroscopy was carried out with an Agilent 8453 spectrometer. Fluorescence spectroscopy was performed using a Hitachi F-4600 spectrometer. Fourier transform infrared (FT-IR) spectroscopy was performed using a Bruker Tensor 27 spectrophotometer. Transmission electron microscopy (TEM) was used for determining the morphological details using an FEI-Tecnai G2. The X-ray photoelectron spectroscopy (XPS) spectra were taken on a Thermo Fisher Scientific ESCALAB 250 photoelectron spectrometer. Confocal imaging of HeLa cells was performed using a Leica TCS SP8 confocal microscopy system.
Synthesis of CDs
The synthesis of CDs was carried out via a microwave assisted hydrothermal method. Briefly, 10.0 mg of dexamethasone and 10.0 mg of 1,2,4,5-tetraaminobenzene (TAB) were dissolved in 5 mL of deionized water followed by ultrasonic treatment for 10 min to obtain a homogeneous solution. The mixture was transferred into a 10 mL glass microwave tube and heated to 160 °C for 15 min. After being cooled down, the product was centrifuged at 10
278g for 10 min. The supernatant was collected and then free precursors were removed using ultrafiltration devices (Millipore, 3 K MW cutoff) to obtain the CDs. Then the product was lyophilized and stored at room temperature for further use.
Cell viability
The cell viability of HeLa cells was evaluated by performing MTT assay. Firstly, HeLa cells were seeded in 96-well plates at a density of 3000 cells per well and maintained at 37 °C for 24 h with 5% CO2. Then, the medium was discarded and fresh DMEM (100 μL) containing varied concentrations of CDs (1–150 μg mL−1) was added for incubation. Twenty-four hours later, the media were discarded and treated with MTT solution (20 μL, 0.5 mg mL−1) for incubating for another 4 h, followed by abandoning the supernatant. DMSO (100 μL) was used to break cellular membranes for releasing formazan crystals. The absorbance at 490 nm was measured and was employed to calculate the cell viability.
Cellular imaging
Cells were plated on confocal dishes and incubated at 37 °C with 5% CO2 using DMEM with 10% fetal bovine serum (FBS), 100 μg mL−1 streptomycin, and 100 units per mL penicillin. After growing to approximately 70% confluence, the medium was replaced with fresh DMEM which contains CDs at 30 μg mL−1. For imaging of endogenous FA, the confocal dish was placed in an incubator for 10 min followed by washing thrice using 0.1 M PBS solution (pH = 7.4) for use. Upon pretreatment with NaHSO3 for the control group, HeLa cells were incubated in the presence of NaHSO3 (200 μM) for 30 min, and subsequently incubated with CDs (30 μg mL−1) for another 10 min, followed by washing thrice with PBS before imaging. For sensing exogenous FA, before recording the fluorescence images, HeLa cells were first incubated with FA (200 μM, 400 μM) for 20 min, washed thrice with PBS, and then incubated with CDs (30 μg mL−1) for 10 min. Cell imaging was performed on a confocal microscope which was equipped with a TCS-SP8 confocal laser scanning microscope (Ex = 405 nm, Em = 450–550 nm for the green channel and 550–650 nm for the orange red channel).
Conflicts of interest
There are no conflicts to declare.
Acknowledgements
Z. Li and L. Qu would like to acknowledge the financial support of the National Natural Science Foundation of China (21708035), China Postdoctoral Science Foundation (2017M620302, 2018T110736), the Foundation for University Key Teacher by Henan Province (2017GGJS007), and the Key Scientific Research Project in Universities of Henan Province (19A150048). We would like to thank the Academy of Medical Sciences of Zhengzhou University Translational Medicine platform and the software Image J for data analysis.
Notes and references
-
WHO Chlorine Dioxide (Gas), Concice International Chemical Assessment Document, World Health Organization, 2002, Document No. 37 Search PubMed
.
- H. Heck and M. Casanova, Regul. Toxicol. Pharmacol., 2004, 40, 92–106 CrossRef CAS PubMed
.
- A. S. Tibbetts and D. R. Appling, Annu. Rev. Nutr., 2010, 30, 57–81 CrossRef CAS PubMed
.
- P. M. Tedeschi, E. K. Markert, M. Gounder, H. Lin, D. Dvorzhinski, S. C. Dolfi, L. Y. Chan, J. Qiu, R. S. DiPaola, K. M. Hirshfield, L. G. Boros, J. R. Bertino, Z. N. Oltvai and A. Vazquez, Cell Death Dis., 2013, 4, e877 CrossRef CAS PubMed
.
- Z. Tong, C. Han, W. Luo, X. Wang, H. Li, H. Luo, J. Zhou, J. Qi and R. He, Age, 2013, 35, 583–596 CrossRef PubMed
.
- M. Unzeta, M. Sole, M. Boada and M. Hernandez, J. Neural Transm., 2007, 114, 857–862 CrossRef CAS PubMed
.
- Z. Tong, W. Luo, Y. Wang, F. Yang, Y. Han, H. Li, H. Luo, B. Duan, T. Xu, Q. Maoying, H. Tan, J. Wang, H. Zhao, F. Liu and Y. Wan, PLoS One, 2010, 5, e10234 CrossRef PubMed
.
- K. Tulpule and R. Dringen, J. Neurochem., 2013, 127, 7–21 CAS
.
-
J. Miao and R. He, Chronic Formaldehyde-Mediated Impairments and Age-Related Dementia, ed. L. M. Martins, InTech, Shanghai, 2012, pp. 59–77 Search PubMed
.
- T. F. Brewer and C. J. Chang, J. Am. Chem. Soc., 2015, 137, 10886–10889 CrossRef CAS PubMed
.
- Y. Tang, X. Kong, Z. R. Liu, A. Xu and W. Lin, Anal. Chem., 2016, 88, 9359–9363 CrossRef CAS PubMed
.
- Y. Tang, X. Kong, A. Xu, B. Dong and W. Lin, Angew. Chem., Int. Ed., 2016, 55, 3356–3359 CrossRef CAS PubMed
.
- K. J. Bruemmer, R. R. Walvoord, T. F. Brewer, G. Burgos- Barragan, N. Wit, L. B. Pontel, K. J. Patel and C. J. Chang, J. Am. Chem. Soc., 2017, 139, 5338–5350 CrossRef CAS PubMed
.
- Y. H. Lee, Y. Tang, P. Verwilst, W. Lin and J. S. Kim, Chem. Commun., 2016, 52, 11247–11250 RSC
.
- W. Liu, C. Truillet, R. R. Flavell, T. F. Brewer, M. J. Evans, D. M. Wilson and C. J. Chang, Chem. Sci., 2016, 7, 5503–5507 RSC
.
- A. Roth, H. Li, C. Anorma and J. Chan, J. Am. Chem. Soc., 2015, 137, 10890–10893 CrossRef CAS PubMed
.
- L. He, X. Yang, M. Ren, X. Kong, Y. Liu and W. Lin, Chem. Commun., 2016, 52, 9582–9585 RSC
.
- L. He, X. Yang, Y. Liu, X. Kong and W. Lin, Chem. Commun., 2016, 52, 4029–4032 RSC
.
- Z. Li, Y. Xu, H. Zhu and Y. Qian, Chem. Sci., 2017, 8, 5616–5621 RSC
.
- T. F. Brewer, G. Burgos-Barragan, N. Wit, K. J. Patel and C. J. Chang, Chem. Sci., 2017, 8, 4073–4081 RSC
.
- K. J. Bruemmer, T. F. Brewer and C. J. Chang, Curr. Opin. Chem. Biol., 2017, 39, 17–23 CrossRef CAS PubMed
.
- K. J. Bruemmer, O. Green, T. A. Su, D. Shabat and C. J. Chang, Angew. Chem., 2018, 130, 7630–7634 CrossRef
.
- H. Yang, G. Fang, M. Guo, P. Ning, Y. Feng, H. Yu and X. Meng, Sens. Actuators, B, 2018, 270, 318–326 CrossRef CAS
.
- F. Wu, Y. Zhang, L. Huang, D. Xu and H. Wang, Anal. Methods, 2017, 9, 5472–5477 RSC
.
- X. Song, X. Han, F. Yu, J. Zhang, L. Chen and C. Lv, Analyst, 2018, 143, 429–439 RSC
.
- M. H. Lee, J. S. Kim and J. L. Sessler, Chem. Soc. Rev., 2015, 44, 4185–4191 RSC
.
- W. Shi, X. Li and H. Ma, Angew. Chem., Int. Ed., 2012, 51, 6432–6435 CrossRef CAS PubMed
.
- K. Jiang, S. Sun, L. Zhang, Y. Lu, A. Wu, C. Cai and H. Lin, Angew. Chem., Int. Ed., 2015, 54, 5360–5363 CrossRef CAS PubMed
.
- S. Lu, L. Sui, J. Liu, S. Zhu, A. Chen, M. Jin and B. Yang, Adv. Mater., 2017, 29, 1603443–1603448 CrossRef PubMed
.
- Y. Song, C. Zhu, J. Song, H. Li, D. Du and Y. Lin, ACS Appl. Mater. Interfaces, 2017, 9, 7399–7405 CrossRef CAS PubMed
.
- Q. Y. Cai, J. Li, J. Ge, L. Zhang, Y. L. Hu, Z. H. Li and L. B. Qu, Biosens. Bioelectron., 2015, 72, 31–36 CrossRef CAS PubMed
.
- Y. Hu, X. Geng, L. Zhang, Z. Huang, J. Ge and Z. Li, Sci. Rep., 2017, 7, 5849 CrossRef PubMed
.
- F. Li, T. Li, C. Sun, J. Xia, Y. Jiao and H. Xu, Angew. Chem., 2017, 129, 10042–10046 CrossRef
.
- J. Xia, S. Chen, G. Y. Zou, Y. L. Yu and J. H. Wang, Nanoscale, 2018, 10, 22484–22492 RSC
.
- L. L. Feng, Y. X. Wu, D. L. Zhang, X. X. Hu, J. Zhang, P. Wang, Z. L. Song, X. B. Zhang and W. Tan, Anal. Chem., 2017, 89, 4077–4084 CrossRef CAS PubMed
.
- X. Geng, Z. Li, Y. Hu, H. Liu, Y. Sun, H. Meng, Y. Wang, L. Qu and Y. Lin, ACS Appl. Mater. Interfaces, 2018, 10, 27979–27986 CrossRef CAS PubMed
.
- H. J. Li, X. Sun, F. F. Xue, N. Ou, B. W. Sun, D. J. Qian, M. Chen, D. Wang, J. H. Yang and X. Y. Wang, ACS Sustainable Chem. Eng., 2018, 6, 1708–1716 CrossRef CAS
.
- H. Liu, Y. Sun, J. Yang, Y. Hu, R. Yang, Z. Li, L. Qu and Y. Lin, Sens. Actuators, B, 2019, 280, 62–68 CrossRef CAS
.
- X. Li, M. Rui, J. Song, Z. Shen and H. Zeng, Adv. Funct. Mater., 2015, 25, 4929–4947 CrossRef CAS
.
- X. Li, X. Gao, W. Shi and H. Ma, Chem. Rev., 2014, 114, 590–659 CrossRef CAS PubMed
.
- Z. Liu, W. He and Z. Guo, Chem. Soc. Rev., 2013, 42, 1568–1600 RSC
.
- X. X. Chen, T. Su, Y. G. He and R. Q. He, Prog. Biochem. Biophys., 2017, 44, 486–494 Search PubMed
.
- W. Xu, Z. Zeng, J. H. Jiang, Y. T. Chang and L. Yuan, Angew. Chem., Int. Ed., 2016, 55, 13658–13699 CrossRef CAS PubMed
.
- A. M. Kaufmann and J. P. Krise, J. Pharm. Sci., 2007, 96, 729–746 CrossRef CAS PubMed
.
- C. Ji, Y. Zheng, J. Li, J. Shen, W. Yang and M. Yin, J. Mater. Chem. B, 2015, 3, 7494–7498 RSC
.
- C. Ji, L. Ma, H. Chen, Y. Cai, X. Zhao and M. Yin, ACS Appl. Bio Mater., 2019, 2, 555–561 CrossRef CAS
.
- X. Chen, X. Zhang, L. Y. Xia, H. Y. Wang, Z. Chen and F. G. Wu, Nano Lett., 2018, 18, 1159–1167 CrossRef CAS PubMed
.
- Q. Q. Zhang, T. Yang, R. S. Li, H. Y. Zou, Y. F. Li, J. Guo, X. D. Liu and C. Z. Huang, Nanoscale, 2018, 10, 14705–14711 RSC
.
Footnotes |
† Electronic supplementary information (ESI) available. See DOI: 10.1039/c9nr01678c |
‡ These authors contributed equally to this work. |
|
This journal is © The Royal Society of Chemistry 2019 |
Click here to see how this site uses Cookies. View our privacy policy here.