DOI:
10.1039/D2RA07098G
(Review Article)
RSC Adv., 2023,
13, 7468-7489
Atmospheric microplastics: exposure, toxicity, and detrimental health effects
Received
8th November 2022
, Accepted 28th February 2023
First published on 8th March 2023
Abstract
Microplastics (MPs) are micro-particulate pollutants present in all environments whose ubiquity leads humans to unavoidable exposure. Due to low density, MPs also accumulate in the atmosphere, where they are easily transported worldwide and come into direct contact with the human body by inhalation or ingestion, causing detrimental health effects. This literature review presents the sources of atmospheric MPs pollution, transport routes, physicochemical characteristics, and environmental interactions. The document also explains the implications for human health and analyzes the risk of exposure based on the potential toxicity and the concentration in the atmosphere. MPs' toxicity lies in their physical characteristics, chemical composition, environmental interactions, and degree of aging. The abundance and concentration of these microparticles are associated with nearby production sources and their displacement in the atmosphere. The above elements are presented in an integrated way to facilitate a better understanding of the associated risk. The investigation results encourage the development of future research that delves into the health implications of exposure to airborne MPs and raises awareness of the risks of current plastic pollution to promote the establishment of relevant mitigation policies and procedures.
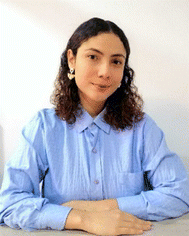 Angela Patricia Abad López | Angela Patricia Abad López is a Chemical Engineer doing her master's degree in Chemical Sciences, exploring and investigating the presence of microplastics in the atmospheric environment as part of her thesis project. She has five years of experience in the academic sector, directed the chair of Scientific and Environmental Thought, and is currently a member of a research group in Photochemistry and Photobiology. |
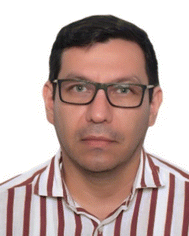 Jorge Trilleras | Dr. Jorge Trilleras was born in Palmira (Valle del Cauca, Colombia), and after graduating in Chemistry, he received his PhD in Chemical Sciences in 2009 from Universidad del Valle (Cali, Colombia). He has 13 years of research experience and holds over 50 original research papers published in journals. His research focuses on the synthesis of heterocyclic compounds. In the last five years, he has participated in identifying and characterizing microplastic particles and emerging pollutants in different environmental matrices. Currently, he is working as a Titular Professor in the Department of Chemistry at Universidad del Atlántico. |
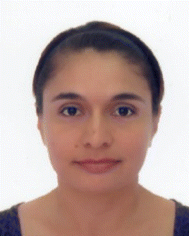 Victoria A. Arana | Victoria Andrea Arana Rengifo graduated from Universidad del Valle (Cali, Colombia) with a degree in Chemistry, a master's degree in Sanitary and Environmental Engineering (2007), and a PhD in Chemical Sciences (2015). She is a professor at the Basic Sciences Faculty of the Universidad del Atlántico, and since 2016 has been the coordinator of a master's degree in Chemical Sciences at the same University. She does research in environmental pollution, water treatment, and spectroscopy-based metabolomics applied to food and health. |
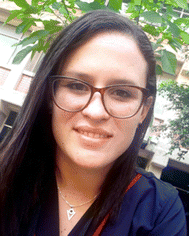 Luz Stella Garcia-Alzate | Luz Stella García Alzate is a Chemist with a Master's in Chemistry from the Universidad de Quindío in 2016. She is a professor at the Faculty of Basic Sciences of the Universidad del Atlántico. She is currently doing doctoral studies in Chemical Sciences at the Universidad del Atlántico in microplastics present in the environment. She has researched feed formulation and bioremediation alternatives. |
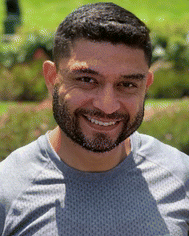 Carlos David Grande-Tovar | Carlos David Grande Tovar is an Associate Professor in the Basic Sciences faculty at the Universidad del Atlántico. He received his BS (2004) and PhD (2010) in Chemistry from the Universidad del Valle (Cali, Colombia). He joined the Basic Sciences faculty at Universidad del Atlántico after his postdoctoral training at the University of Houston, Case Western Reserve University, and Universidad del Valle. Professor Grande works with fungi for plastic recycling, and sustainable green solutions for the increased pollution worldwide. His group is working in biopolymers and nanocomposites for tissue engineering and the extension of the shelf life of food. |
Introduction
Plastic is a material present in several articles of daily use due to its low cost, versatility, ductility, and durability.1,2 However, only 9% of plastic material is recycled, which, combined with the outstanding resistance, generates a high accumulation.3 Moreover, plastics are quickly released into the environment, and are fragmented due to the exposure to solar radiation, wind, and other abiotic or biotic factors, generating smaller particles, even plastic microparticles.4
In general, the definition of Frias & Nash5 is used to define a “microplastic” as “any synthetic solid particle or polymeric matrix, with a regular or irregular shape, with a size between 1 μm and 5 mm and that is insoluble in water.” Besides, to better study and categorize these microparticles, Hartmann et al.6 have proposed a framework with descriptors like (a) chemical composition, e.g., polyethylene (PE), polypropylene (PP), polyethylene terephthalate (PET), polystyrene (PS), among others; (b) color, e.g., blue, yellow, green, black; and (c) shape, e.g., spheres, pellets, foams, fibers, fragments, films. This last form of classification represents the primary means to quantify MPs and also facilitates subsequent analysis since a part of these microparticles is identified, and the others are evaluated based on similarities.7
Another way to classify MPs is according to their origin: “primary” and “secondary” MPs.8 Those MPs that are synthesized at the micrometric scale for multiple applications (such as components of personal care and cleaning products) are called primary MPs.9 These MPs end up in wastewater or industrial water once their life cycle is over, penetrate aquatic ecosystems due to their incomplete elimination in water treatment plants, and even penetrate soils when sediments from these plants are used for agricultural activities.10
Otherwise, MPs that arise from the degradation of macroplastics are called secondary MPs and contribute to a greater extent to global microplastic pollution.11 From a regulatory point of view, this classification is relevant for risk management. It can allow the identification of the main parties responsible for the release of MPs and assign commitments aimed at improving their processes so that MPs' emissions or their sources are reduced through regulatory, economic, and persuasive instruments.6
Although most of the literature published is based on the categories mentioned above, these are not the only proposals. Another alternative was proposed by Kooi and Koelmans,12 who expose that discrete classifications for sizes, shapes, and densities are unsatisfactory as they do not represent the continuous nature of environmental MPs. They propose a full definition of MPs through a three-dimensional (3D) probability distribution, with size, shape, and density as dimensions. Here we can see the first challenge, reaching a consensus on the classification of the MPs.
Due to microparticle size being an essential factor in defining their interaction with biota and environmental fate, the easy MPs migration to other spaces, spreading pollution, and complicating control and management have been studied.13
Since their discovery, the study of MPs has focused on aquatic10,14 and terrestrial ecosystems.15,16 However, from 2015, when MPs were detected for the first time in atmospheric precipitation,17 studies on airborne MPs have dramatically increased,18–20 as shown in Fig. 1, because the atmosphere is a reservoir and a superhighway through which large amounts of suspended materials are transported.21–23 The research on airborne MPs is divided into three categories: suspended atmospheric microplastics (SAMPs), atmospheric fallout, and dust (indoors or outdoors),24 where the density of the MPs is one of the essential factors for the classification since a higher particle density leads to faster deposition.23 Each category has a specific sampling process; the first two categories are based on passive measurements, and the last one is on active sampling, which more accurately reflects airborne MP exposure intensity.25 MPs' presence in the air causes a particular concern since, in contrast to those in other environmental compartments, they can enter the human body by inhalation and ingestion.26,27 MPs in biological systems can cause inflammatory lesions, oxidative stress, and cytotoxicity. Once inside, they can remain intact for a long time, translocate to other tissues, and even accumulate in different organs.9 In addition, the inability of the immune system to eliminate MPs can lead to chronic inflammation and increase the risk of neoplasia.28
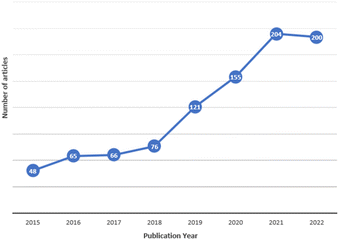 |
| Fig. 1 Number of publications about atmospheric MPs per year, since 2015 to May 2022. Source: Scopus database. | |
Therefore, this investigation aims to recognize the attributes that confer toxic potential to MPs, associated with their physicochemical characteristics and interactions in the environment. The research also presents the main routes of exposure to these atmospheric pollutants, their abundance, sources, mechanisms of transport, and recent findings on their implications for animals and human health.
Methodology
The investigation was performed for articles published between 2015 and May 2022 using the Scopus and Google Scholar databases. The literature was obtained from a systematic search according to the PRISMA methodology – Preferred Reporting Items for Systematic Reviews and Meta-Analyses.29 Publications related to the study of atmospheric MPs and their effects on human health were obtained using the following search key: (microplastic* OR micro-plastic* OR microfiber* OR fiber*) AND (air OR atmosphere OR atmospheric* OR airborne) AND (health OR risk OR inhalation OR toxic* OR exposure).
This search yielded 832 articles filtered by title, abstract, and general content (Fig. 2). In addition, the search was limited to documents included in the “Environmental Sciences”; category and the “English”; and “Spanish”; languages in the Scopus database. The total number of publications included in this review was 143, and 81% were published in the last three years, demonstrating the relevance of the MPs' problem and its ubiquitous presence in the atmosphere.
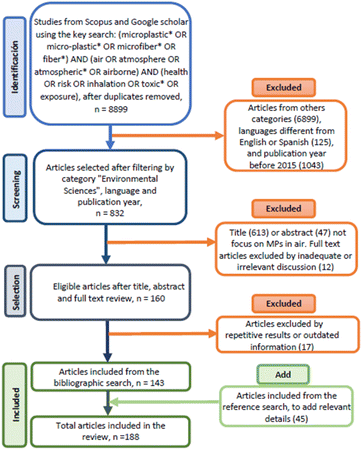 |
| Fig. 2 Schematic representation of this review's literature search, screen, and selection process. | |
Likewise, additional articles were included directly from the reference search to expand or complement relevant details to understand better the possible detrimental health effects caused by MPs.
Abundance of atmospheric MPs
Estimating their abundance in the air is the first step to analyzing the exposure and risk associated with atmospheric MPs. MPs' abundance is generally related to their sources and transport.
Regarding sources of atmospheric MPs, these can be studied according to the environment, outdoors or indoors, and all are associated with anthropogenic activities, like industrial, agricultural, or domestic activities.21
Sources of airborne MPs – outdoor environments
The sources of airborne MPs in outdoor environments are diverse. For example, synthetic clothing has been mentioned as a predominant source of microplastic fibers.30 Likewise, abrasion of synthetic textiles,31 incomplete incineration of plastic waste,32 municipal solid waste,33 dust storms,34 abrasion from synthetic rubber tires,35 scaffolding mesh on construction sites, and synthetic turf for ground cover36 are recognized as potential sources of MPs suspended in outdoor air.
Sources of airborne MPs – indoor environments
In general, site furniture represents an essential source of MPs in the indoor air because the tiny fibers of furniture, curtains, carpets and other objects can be released into the air.30 Also, the use of air conditioners,37,38 paint coatings, and the type of floor39 influence the concentration of MPs suspended indoors.
Likewise, the levels of MPs in the air depend on the activities that take place indoors, the number of people present,40 and the plastic contained in the objects used41 since it has been found that MPs are generated just by opening and handling plastics, such as containers or wrappers.42
In addition to these local sources, distant sources of MPs are also important, especially for remote areas with less anthropogenic disturbance,8 since atmospheric transport allows the migration of MPs to sites far from their starting point.23,43 The MPs' presence in air samples from remote oceanic regions43,44 and even glacial territories45 indicates that it is not a local problem.
Effects of atmospheric processes on MPs abundance
Although MPs are ubiquitous in atmospheric environments, their abundance varies from one place to another depending on the altitude, latitude, environmental conditions, and season of the year, among others.44 For instance, a higher concentration of MPs has been found near the ground than at high altitudes due to the influence of atmospheric pressure and gravity (vertical concentration gradient).45 On the other hand, the vertical temperature gradient helps the ascending movement of MPs because, in the lower part, the air is warmer and, therefore, less dense, tending to rise. Still, it may also block MPs in the soft layers of the atmosphere in case of temperature inversion since the cold air located at a lower altitude is heavier and cannot rise, preventing the dispersion of atmospheric pollution and leaving the pollutants trapped near the surface.21
In addition to these thermodynamic aspects, it is necessary to consider chemical processes since, in the atmosphere, MPs can change their size distributions due to the photodegradation, influencing their abundance, transport mechanisms, diffusion, reactivity, and interactions with other contaminants and microorganisms.46 Free radicals mediate these photooxidation reactions, and they are initiated by solar radiation, altering the mechanical, physicochemical, thermal, and surface properties (e.g., such as hydrophobicity, surface charge, surface area, and porosity) of MPs, especially for those with the presence of chromophores.4
Subsequent reactions in photodegradation are associated with chain scission reactions, which generate lower molecular weight microparticles that are easier to move in air,47 as well as gaseous products and soluble organic compounds that can promote or inhibit microbial growth.46 The molecules of O3, SO2 (in singlet or triplet reactive state), and NO2 present in the atmosphere are responsible for the chain scission of polymers after the reaction with the carbon–carbon double bonds.4 Fig. 3 illustrates these atmospheric processes and their impact on MPs' transport.
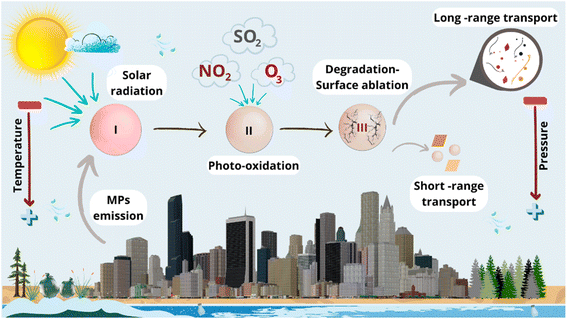 |
| Fig. 3 Atmospheric processes on MPs. (I) Airborne pristine MP. (II) Photodegradation of MP by solar radiation and interactions with atmospheric compounds. (III) MP degradation, lower weight particles achieve long-range transport, heavier ones achieve short transport distances ©[Abad] via https://www.canva.com/. | |
To address these heterogeneous processes on solid particle surfaces in the atmosphere, the IUPAC Subcommittee on Evaluation of Gas Kinetic Data for Atmospheric Chemistry48 determines absorption coefficients and adsorption parameters to calculate rates of heterogeneous trace gas reactions. So far, the interactions between gases and solid surfaces such as ice, acid hydrates, and mineral dust have been studied. However, due to the complexity of these heterogeneous atmospheric processes, current knowledge is insufficient to allow plastics to be incorporated into chemical and atmospheric transport models.
Factors associated with the abundance of airborne MPs
For outdoor environments, meteorological phenomena such as dust storms34 and monsoons promote transboundary migrations of MPs.49,50 Climatic factors such as wind and rainfalls (significant for tropical countries with high intensity and volume precipitation) positively correlate with MPs abundance, influencing the transport and settlement of MPs suspended in the air.50 In coastal regions, air mass trajectory can affect MPs deposition; when the inflowing air mass is terrestrial (predominantly from local sources), there is a higher MPs deposit than when the inflowing air mass is marine. Concerning wet and dry periods, MPs deposition is higher during wet periods. In this period, the amount of MPs will depend on the intensity of precipitations that may occur (rainfall, snowfall). In dry periods, MPs deposition may increase when the mean wind speed and relative humidity increase. MP particles may increase in size due to hygroscopic growth, increasing the deposition rate.51 Fibers represent the type of MPs with the highest abundance percentage found in different atmospheric environments. These particles are more transportable due to the low density of plastic particles (0.65 to 1.8 g cm−3) concerning soil particles (∼2.65 g cm−3).52
Also, just as atmospheric MPs can be deposited, those present on land or water can be resuspended in the air, leading to a cyclical exchange of MPs via air, water, and land.24 Even terrestrial plants could temporarily store MPs in their leaves.53 In this exchange dynamic, airborne MPs contribute significantly to pollution levels in marine and terrestrial ecosystems by falling onto their surfaces.22,54,55 Some studies reported that MPs atmospheric deposition is the main contributor to MPs' presence in the ocean.23,56
Regarding indoor environments, the movement of MPs is influenced by temperature and humidity.57 In addition, the presence of air conditioners is relevant because they can resuspend sedimented particles or fibers and control their distribution through indoor air.37 Diverse studies have shown that the concentration of MPs in the indoor air environment is much higher than that in the outdoor within the same area,44,58 which may be attributed to conditions of high population density and mainly to the poor particle dispersion.41
For the above, the composition of indoor MPs varies significantly compared to outdoor. Still, in ventilated spaces, similar patterns are observed59 since an exchange of indoor and outdoor MPs can occur due to an airflow caused by the ventilation of a door or window.58
It is worth mentioning that other factors that affect the abundance, transport, dispersion, and settlement of MPs in the air are the shape and length of the particles (associated with their buoyancy), so these physical characteristics play an essential role in their movement.54,60
Transport of airborne MPs
Some investigations estimate the influence of distant MPs sources on the local abundance of these microparticles in a particular area. For instance, Wright et al.61 studied the long-range transport of MPs from the time they are suspended in the air, and the trajectory traveled based on their concentrations, wind speed, and direction data. The suspension time was calculated based on the average height of the study site's boundary layer and the MPs' sedimentation speeds, which were obtained according to each morphology and the average wind speed. In addition, they studied the local sources of MPs through bivariate polar diagrams, which also allowed them to determine the mean value of the concentration of an environmental pollutant against the direction and speed of the wind.61
To explore the relationship between weather conditions and atmospheric MPs abundance and also to determine the inner connection between environmental factors and MPs distribution, Liu K. et al.62 calculated a rough estimation of the horizontal transport flux of SAMPs as follows:
where
Nδ,
w and
z represent the MPs abundance (n m
−3) (items per cubic meter of air), the horizontal wind speed (m s
−1) in the
θ direction, and the approximate sampling height (40 m), respectively.
To track the areas through which the air masses passed before reaching the reception points and to analyze the dominant airflow directions and possible sources of MPs that affect the sampling area, Ding et al.56 used backward trajectories. They used the National Oceanic and Atmospheric Administration database (in addition to the Global Data Assimilation System meteorological archives) and the Hybrid Single Particle Lagrangian Trajectory Integrated (HYSPLIT) model. This computer model is widely used to calculate the backward trajectories of airborne MPs to determine how far and in which direction these microparticles will move.22,49,63 Using backward trajectories allows entering multiple input meteorological fields, physical processes, and emission sources to estimate transport, dispersion, and deposition patterns.56
To assess MP total deposition flux (DF), Kernchen et al.64 used the following equation:
where DF: number (
nDF, N m
−2 d
−1) or mass (mDF, kg ha
−1 a
−1) deposition flux, respectively;
χ: blank subtracted count (N) or mass (kg) of plastic particles in a field subsample found by μFT-IR;
γ: subsample size;
a: exposed surface area of the collecting funnel (0.05187 m
2/5.187 × 10
−6 ha);
t: duration of sampling (days per year).
Exposure routes to MPs
Human exposure to airborne MPs occurs along three routes: inhalation, ingestion, and dermal contact,9,26–28 as shown in Fig. 4.
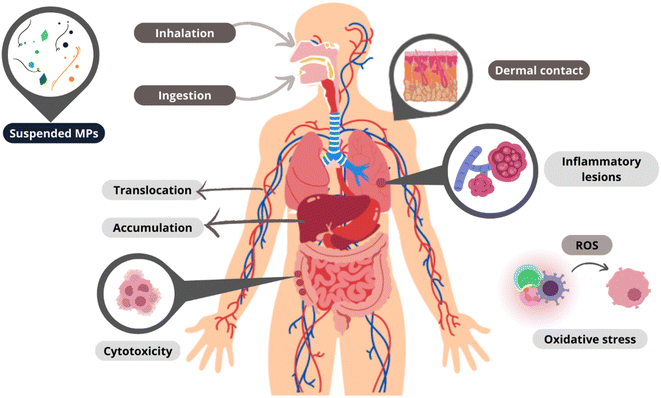 |
| Fig. 4 Exposure routes and potential effects of MPs in humans ©[Abad] via https://www.canva.com/. | |
Inhalation
Inhalation is the most significant route of human exposure to MPs.65 It has been estimated that, on average, a person inhales between 26 and 130 MPs per day,18 and even with light physical activity, up to 272 MPs per day can be inhaled.66 Indoors, an input between 1.9 × 10 3 to 1.0 × 10 5 MPs per year is estimated, while a range of 0–3.0 × 107 MPs per year for outdoor environments.67
Akhbarizadeh et al.68 carried out an estimation of MPs intake via inhalation on average and dusty days using the following equation:
Where ADI is the average daily inhale (items per person per day),
C is the MPs number (items per
m3) and IR is the inhalation rate (
m3 per day).
The possible inhalation of MPs depends on their size, which determines whether they will reach the respiratory system.28 Thus, MPs can be inhalable (that is, capable of being deposited in the upper respiratory tract after entering through the nostrils or the mouth) or breathable (capable of reaching and being deposited deep in the lung).69 Particles <10 μm are toxicologically relevant due to their greater probability of crossing epithelial barriers and increased reactivity with cells and tissues.18
Similarly, the MPs' shape affects exposure, as there is evidence that MPs in the form of fibers can penetrate deep into the human lung.70 The size and shape of the microplastic particles are determined by visual or optical microscopy techniques.71 For example, the fluorescent microscope, binocular microscopy, and polarized light microscopy are options for use.60,72
Ingestion
MPs intake occurs because their settlement on the food we eat is more significant than that due to the bioaccumulation of MPs in the food chain.73
Approximately 33% of domestic dust fallout corresponds to MPs.74 In places to eat and drink, up to 105 MPs m −2 d −1 have been found with higher exposure rates indoors than outdoors.75
Based on average daily dust exposure (100 mg d−1), adult intake ranges from 17.7 to 75.4 MPs per day76 and varies from 1 × 10 2 to 1.9 × 10 4 MPs per year.67
Zhang et al.77 calculated the Estimated Daily Intake (EDI) of MPs via ingestion using the:
where
mingestion Is the soil and dust ingestion rate (g d
−1) recommended by the United States Environmental Protection Agency (USEPA); BW is the body weight (kg);
c is the concentration of MPs (mg g
−1) in soil; AMIMP is the average mass of individual MP particle.
For their part, C. Liu et al.78 calculated the EDI of MPs via indoor and outdoor dust ingestion according to the:
where
f1 and
f2 are the indoor and outdoor exposure fractions, respectively;
c1 and
c2 are concentrations of the target MPs in indoor and outdoor dust, respectively;
mdi,1 and
mdi,2 are the indoor and outdoor dust ingestion rates (mg d
−1), and BW is the average body weight (kg).
The EDI values of dust are inversely proportional to body weight,78 so EDI values in adults will be an order of magnitude lower in contrast to children.60,79,80 Furthermore, due to pulmonary mucociliary clearance, microparticles can enter the digestive tract after inhalation.65
Dermal contact
Dermal contact can occur through the penetration of MPs into the skin pores, mainly through contact with water contaminated with MPs or through cosmetics and other body products incorporating them.81
This dermal contact with MPs is associated with skin damage due to local inflammation and cytotoxicity.82 However, this exposure is a function of individual susceptibility since the pores of human skin can vary according to each individual.83
It should be noted that physical characteristics such as size (associated with accumulation and translocation in tissues), color (visibility and preference for ingestion by animals), and shape (density and adherence capacity) affect the risks of exposure to MPs.84
Toxicity of MPs
The potential toxicity of MPs, from which the magnitude of their health implications can be measured (in addition to their mere presence), is described by their chemical nature1 and is related to the possible release of chemical constituents (polymer raw materials and additives).85 The release of microorganisms,86–88 heavy metals, and other substances that have been adsorbed during their residence in the environment is also a concerning factor.89,90 The adverse effects of this release will also depend on the translocation of MPs to other cell tissues.26,44,91
Chemical identity
The original chemical identity of plastics can be made up of a mixture of approximately 40
000 substances.92 Within this mixture, polymers are found as the main constituents. Still, various additives such as plasticizers, stabilizers, flame retardants, flow modifiers, processing aids, impact modifiers, antioxidants, pigments, biocides, fragrances, and even residues of monomers or by-products formed during production can be found.6
Polymeric matrix
The characterization of MPs based on their constituent polymers can be carried out through various techniques. For example, pyrolysis with gas chromatography and mass spectrometry is a technique that produces pyrograms of the polymers under study after their thermal degradation, which are then compared with pyrograms of polymers of known origin to achieve identification.93 Similarly, by exposing MPs to infrared radiation at different wavelengths, Fourier transform infrared spectroscopy allows the polymers present to be identified by comparing the infrared spectra obtained from the sample with the infrared spectra of known polymers.39,71,94 On the other hand, through a high-frequency laser that excites the surfaces of materials until reaching the emission of photons, Raman spectroscopy identifies the chemical composition of a sample in which 1 of every 7–10 photons are emitted at a right angle, forming what is called Raman scattering. In contrast, the rest of the photons are cast in line with the laser.59,77,95
The most common polymers found in atmospheric MPs are polystyrene (PS), polyethylene (PE), polyester (PES), polyamide (PA), polypropylene (PP), polyethylene terephthalate (PET), polyacrylonitrile (PAN), polyurethane (PUR), polycarbonate (PC), epoxy resins (EP), polyvinyl chloride (PVC), and polyvinyl acetate (PVA).8,19 Table 1 shows the polymers characterized in some studies of atmospheric MPs (indoor and outdoor ambient) and the concentrations and physicochemical characteristics reported.
Table 1 Examples of reported physicochemical characteristics of MPs in an indoor and outdoor environment
|
Type of sample |
Concentration |
Size |
Identification and characterization |
Main polymers |
Shapes |
Colora |
Reference |
Color: B: blue; BK: black; BW: brown; G: green; GY: gray; O: orange; P: purple; PK: pink; R: red; T: transparent; W: white; Y: yellow. |
Indoors |
Suspended dust |
3.24–27.13 [MPs per m3 ] |
0.45–2800 μm |
Stereomicroscope |
PES, PA |
Fibers (91%), fragments (9%) |
B, T, BK, R, GY |
38 |
Fluorescent + Nile red + stereomicroscope |
UV using hot needle technique + μRaman |
Suspended dust |
1583 ± 1181 [MPs per m3] |
5–4665 μm range |
Nile red staining + μFTIR |
PES, PA, PP |
Fragments (89.6%), fibers (10.4%) |
— |
96 |
< 30 μm: 60.4% |
30–100 μm: 28.5% |
>100 μm: 11% |
Indoor air |
22–6169 |
50–2000 μm |
Stereomicroscope + fluorescent microscope + μFTIR |
PE, PES, PET, PA, PVC, PS |
Fibers, fragments, and films |
BK, G, B, R, GY, BW, T |
39 |
Mean: 3095 [MPs/m2 per day] |
Suspended dust |
1–60 [MPs per m3] |
50–3250 μm |
Stereo microscope + ATR – FTIR |
PP, PA |
Fibers |
— |
58 |
Indoor air |
Bedroom: 9,9 × 103 |
50–2000 μm (80%) |
Stereomicroscope + μFTIR |
PES, PUR, PP, PS, PA |
Fibers, fragments |
T, B, R, Y, BK, P, G |
57 |
Office: 1,8 × 103 |
Hall: 1,5 × 103 [MPs per m2 per day] |
Outdoors |
Suspended dust and atmospheric fallout |
0.3–1.1 [MPs per m3] |
50–100 μm (75%) |
Binocular microscopy, polarized light microscopy, and fluorescence microscopy + SEM/EDS |
— |
Microspheres, films, fragments, and fibers |
W-T, Y-O, R-PK, B-G, BK-GY |
60 |
>1000 μm |
Suspended dust (Pacific ocean) |
0–1.37 |
16.14–2086.09 μm |
Stereomicroscope + μFTIR |
PET, PE, PES, EP, PA, PAN, PP, PS, PVA, PVC |
Fibers (60%), fragments (31%), Granules (8%), and microspheres (1%) |
BK, B, BW, G, O, PK, P, R, W, Y, GY |
43 |
Mean: 0.01 [MPs per m3] |
Average: 318.53 μm |
Suspended dust |
0–2 [MPs per m3] |
Fibers: 428.81 μm |
Stereo microscope + μFTIR |
PET, EP, PE, PP, PA, PS |
Fibers, fragments, microspheres |
W, PK, B, Y, BK, R, G, GY, P, BW |
62 |
Fragments: 121.4 μm |
Microspheres: 36.59 μm |
Atmospheric fallout |
275 [MPs per m2 per day] |
Groups |
Fluorescent microscope + μRaman |
PE, PVA, PET |
Fragments (95%), fibers (5%) |
— |
97 |
<63 μm |
63–300 μm |
300–5000 μm |
Suspended dust (south China sea) |
0–3.1 [MPs per 100 m3] |
286–1862 μm |
Stereo microscope + μFTIR |
PP, PET, PVA |
Fibers (75%), fragments (25%) |
BK, Y, R |
63 |
Additives
The most common additives intentionally added to give plastic qualities and improve specific mechanical properties are toxic plasticizers, dyes, and flame retardants.98 For example, Bisphenol A (BPA), a common plasticizer, is considered estrogenic due to its hormone-disrupting properties in the human body, which are associated with obesity, cardiovascular disease, reproductive disorders, and breast cancer.1
Likewise, heavy metals frequently found in pigments and plastic stabilizers can cause harm to the human body. It has been estimated that cadmium promotes cell apoptosis and DNA methylation, inducing oxidative stress, causing DNA damage, bone and lung damage, alterations in calcium metabolism, and kidney stone formation.99 Lead, another common metal in plastics, can cause muscle weakness, brain damage, kidney damage, and even death.100 The potential human health effects of other common additives found in MPs are shown in Table 2.
Table 2 Some additives in the manufacture of plastics and their toxic potential for health
Category |
Composite/metal |
Associated polymers |
Potential effects |
References |
Flame retardants |
Polybrominated Diphenyl Ethers (PBDEs) |
PP, PE, PS, PVC, PA |
Diabetes, neurobehavioral and developmental disorders, cancer, reproductive health effects, and impaired thyroid function |
107 and 108 |
Antimony (Sb) |
PVC, PU, PET |
Associated with carcinogenic effects |
83, 109 and 110 |
Pigments |
Cadmium (Cd) |
PVC, PP, PE, PC |
Promotion of cell apoptosis and DNA methylation, oxidative stress, DNA damage, bone and lung damage, alterations in calcium metabolism, and kidney stone formation |
99, 111 and 112 |
Titanium (ti) |
PP, PS, PE |
Decreased cell viability and increased intracellular reactive oxygen species (ROS) cytotoxicity in human lung and colon epithelial cells |
113 and 114 |
Biocides |
Arsenic (As) |
PVC |
Cell proliferation, apoptosis, and neoplastic transformation. Cellular damage occurs through the generation of ROS with oxidative stress, alteration of the DNA repair mechanism, and mitochondrial and cytoskeletal damage. They are associated with different types of cancer (skin, kidney, liver, liver, prostate, lung, etc.), cardiovascular disorders, diabetes, and hypertension |
115 and 116 |
Mercury (Hg) |
PU |
Mutagenicity/carcinogenicity through the generation of free radicals; alteration of the molecular structure of DNA, alterations in brain functions; damage to the kidney, brain, and developing fetus |
99 and 115 |
UV stabilizers |
Lead (Pb) |
PVC |
Carcinogenesis, DNA damage, alteration of chromosomal structure and sequence, toxicity related to the gastrointestinal tract and the central nervous system, alterations in the functioning of the central nervous system, and decreased neurotransmission |
99 and 109 |
Cobalt (Co) |
PET |
Formation of reactive oxygen species, neurological, cardiovascular, and endocrine deficits |
1 and 117 |
Plasticizers |
Phthalates (DBP, DEHP) |
PVC, PET, PE |
Endocrine disruption, associated with reproductive toxicity and carcinogenicity |
118–120 |
Bisphenol A (BPA) |
PC, PE, PP, PVC |
Endocrine disruptors associated with obesity, fertility problems, cardiovascular disease, reproductive disorders, and breast and prostate cancer |
121–123 |
The mechanism of adsorption or desorption of these substances in MPs is not yet completely understood; however, possible interactions include hydrophobic interactions, polar interactions favored by pH variations, particle aging (photo-oxidation), and polymer decomposition.1
Interactions of MPs in the environment
MPs can interact during their residence in the environment and serve as vectors for various contaminants adhered to their surface.68 Their adsorption rate will depend on the type of MPs, their physical and chemical properties, surface area (affecting diffusivity), and hydrophobicity (affecting polarity).90 MPs mainly adsorb chemicals from the surrounding medium through hydrophobic interactions, π–π type interactions, and electrostatic interactions. Pollutant-loaded MPs exhibit a high toxicity potential.101
Once suspended in the air, MPs become a part of atmospheric particulate matter (PM), and their possible interactions with other PM are being studied.23,35 For instance, in urban environments, owing to atmospheric MPs co-occur with traffic emissions, they may carry Polycyclic Aromatic Hydrocarbons (PAHs), transition metals, or other chemicals.69
According to Akhbarizadeh et al.,68 MPs and PAHs are the critical chemicals found in urban deposited/suspended dust that has attracted global attention in recent years. They carried out the Pearson correlation analysis to clarify the relationship between total PAHs, MPs, and PM2.5 (PM with a diameter of 2.5 micrometers or less), and the results revealed that there was a moderately significant relationship between PAHs and PM2.5 (r = 0.45, p < 0.05). There was also a meaningful positive relationship between MPs and PM2.5 (r = 0.76, p < 0.05), demonstrating the co-occurrence of MPs and PM2.5, which means that airborne MPs could act as a vector for PAHs and their presence in the atmosphere could increase the toxic effects of chemical contaminants.
Regarding trace elements, Yao et al.102 found that Ca, Al, Na, Cl, P, S, O, and C are common elements that could be attached to the airborne MPs and may accelerate the degradation processes from airborne MPs to nanoplastics. Besides, the presence of components such as ozone (O3), sulfur dioxide (SO2), nitrogen dioxide (NO2) and volatile organic compounds (VOCs) can directly attack MPs and catalyze the formation of radicals by photochemical reactions, which can also lead to their degradation.4
Besides, MPs can also serve as a vector for microorganisms (MOs), due to these can become a microhabitat where MOs easily adhere to their hydrophobic surfaces, organizing themselves into biofilms.103 As a barrier, the biofilm creates a stable internal environment for the vital activities of cells, acting as a protective ecological niche called the “plastisphere”.87 This biofilm structure is adopted when aggregates of MOs irreversibly adhere to the MPs' surface and reproduce and secrete a polysaccharide matrix surrounding the colony.101
In aquatic environments, various microorganisms have been detected adhering to MPs. For example, pathogenic Vibrio species have been found in marine MPs,88 as well as Pseudomonas monteilii, Pseudomonas mendocina,104 and Escherichia coli strains.105
For terrestrial environments, Gkoutselis et al.87 conducted studies on the fungal colonization of MPs deposited in the soil in terrestrial ecosystems. They observed a fast reproduction and propagation of fungi within the plastisphere (the majority of these opportunistic human pathogens responsible for a wide range of human mycoses), where the hyphae adhere to the plastic surface through small protrusions of the peripheral cell wall.87
MPs' primary interaction/accumulation pathways with microorganisms might include bioadsorption, biouptake (cellular uptake), and biodegradation.106 From these interactions, the possible development of antibiotic-resistant microbial communities within biofilms is of concern, either as a result of horizontal gene transfer by environments exposed to antibiotics104 or by co-selection from exposure to metals adsorbed on MPs.106
On the other hand, in addition to acting as pathogens carriers, plastic surfaces protect them from the surrounding environment, favoring their survival in the atmosphere.124 For example, it has been found that (under certain conditions) SARS-CoV-2 can survive approximately 72 hours on plastic surfaces.125 A study confirmed a positive correlation between the severe acute respiratory syndrome coronavirus (SARS-CoV-2) and the levels of MPs in the air.126 Other examples of MOs and pathogens found in MPs, along with their potential health effects, are shown in Table 3.
Table 3 Potential health risks of some studies on MPs as vectors of MOs and pathogens
Category |
Species |
Polymer constituent of MPs |
Health effects |
Reference |
Bacteria |
Vibrio spp. |
PET, PE, PS, PP |
Cholera, gastrointestinal diseases, wound infections, and sepsis |
88, 127–129 |
Bacteria |
Pseudomonas monteilii, Pseudomonas mendocina |
PVC |
Hypersensitivity pneumonitis and bronchiectasis due to exacerbation, nosocomial infections, central nervous system infections, and skin and soft tissue infections |
104 and 130 |
Bacteria |
Vibrio spp. Escherichia coli, Tenacibaculum discolor, Acinetobacter oleivorans |
PET, PE, PP |
Hemolytic uremic syndrome and hemorrhagic colitis lead to acute renal failure and death. They can also cause extraintestinal diseases and sepsis |
86, 105, 129 and 131 |
Fungi |
Fusarium oxysporum alternaria alternata, Didymella glomerata, Cladosporium, Phoma and Curvularia lunata, N. diffluens, Rh. mucilaginosa, R. anthropophila, P. herbarum |
PE, PA, PP, |
Fusariosis, eumycetoma and phaeohyphomycosis, bronchopulmonary infections, cutaneous, subcutaneous, and systemic |
87 and 132 |
Bacteria |
Enterococcus faecalis, Acinetobacter baumanii complex |
PET, PVC, PS, PE, PP |
Nosocomial bloodstream infections, pneumonia, and infective endocarditis can cause bacteremia by moving through the intestine and accessing the lymphatic vessels and the bloodstream |
133–135 |
Bacteria |
Ochrobactrum anthropi, Acinetobacter lwoffii, Afipia broomeae, Acinetobacter baumannii, Pseudomonas aeruginosa, and Escherichia coli |
PS |
Nosocomial infections include bacteremia, secondary meningitis, urinary tract infection and pneumonia, skin and wound infections, and infective endocarditis |
136 and 137 |
Bacteria |
Raoultella ornithinolytica, Stenotrophomonas maltophilia |
PE, PS |
Infections of the bloodstream, respiratory tract, and pneumonia; urinary tract infections; gastrointestinal disorders; wound and skin infections; and bacteremia, osteomyelitis, meningitis, brain abscess, mediastinitis, pericarditis, conjunctivitis, and otitis |
138–140 |
Virus |
SARS-CoV-2 |
PS, PAN, PA, PVC, PU |
Fever, cough, dyspnea, pneumonia, acute respiratory distress syndrome, systemic inflammatory response syndrome and multiple organ failure, gastrointestinal tract infections such as diarrhea and dysgeusia, hypoxia, and pulmonary short circuit due to vasodilation |
126, 141 and 142 |
Bacteria |
Mycobacterium, Legionella, Arcobacter |
PA, PVC, PE, PET, PP |
They invade eukaryotic cells, induce an immune response, and produce toxins that damage host cells, cause enteritis, bacteremia, ear infections, etc. |
143–146 |
MOs in MPs must remain investigated in the following years due to the urgent need for possible health risk prevention. In addition, the mechanism of growth and dispersion of pathogenic MOs in MPs is still not clearly understood, so research must continue in this field to provide greater clarity and generate risk prevention strategies.
Effect of aging on the toxicity of MPs
When exposed to the environment, the MPs grow old, altering some of their properties in contrast to pristine versions.89 The degree of aging will depend on the exposure time, the environment, and the properties of the polymer constituents.147
Solar radiation is the main factor that ages suspended MPs in the atmosphere.148 Photo-oxidation reactions driven by ultraviolet radiation are cataloged as the most important environmental cause of polymer deterioration since they cause breaks at the molecular level in polymer chains, thus reducing the cohesion of the material.149 Unlike in terrestrial or aquatic environments, more significant deterioration is observed in the air because the associated photo-oxidation has higher temperatures, higher levels of available oxygen, and ultraviolet radiation.150
Among the changes caused by aging is the increase in the adsorption capacity of MPs.151,152 This response to changes in its hydrophobicity90 and the appearance of rough surfaces contribute to increasing the effective surface area,89,147,153 offering more adsorption sites for contaminants.150 This adsorption capacity is also favored when the organic matter has been bound to the MPs154 since the adsorption facilitates the subsequent adsorption and mobility of trace elements.155
In addition, as aging is prolonged, a substantial increment in the cytotoxicity of MPs is observed due to an increase in their oxidative potential and reactive oxygen species (ROS).156 ROS have essential biological functions for normal cell development. However, an increase above the basal level can lead to impaired homeostasis, reflected in cell metabolism alterations and regulation, in which biological molecules, DNA, membrane proteins, and lipids can be irreversibly affected.157 In addition, it has been found that aged MPs can be internalized more efficiently into cells, the key route by which MPs can be transferred to tissues and where they can cause toxicological effects with severe implications for human health.158
MPs will continue to age throughout their environmental migration,150 with color as a strong indicator of chemical changes due to photodegradation. Discoloration occurs with a continuous exposure, where extremely weathered particles can show a loss of color at the edges and surfaces.25
Health effects due to exposure to atmospheric MPs
MPs have been demonstrated to have negative health consequences on animals. Experiments with mice revealed that molecular or higher-level alterations arise after prolonged exposure to MPs.159 The damage that occurs in organs and tissues depends on the dose exposed,160 and plastic particles detected in the lungs not only comprise the range of MPs since smaller plastic particles (100 nm–1 μm) have been observed.161 Likewise, MPs' harmful effects on the respiratory system are exacerbated in mice with pre-existing respiratory diseases.162 It was even found that MPs can affect the reproductive system since, in a study with polystyrene MPs, it was shown that they caused fibrosis and apoptosis of ovarian granulosa cells through oxidative stress, which resulted in a decrease in ovarian reserve capacity.163 This cell toxicity has also been observed on the eye's surface in the exposed mucosal tissue, leading to ocular dysfunction.164 The possible appearance of cellular lesions by MPs could cause mitochondrial dysfunction, where the ATP produced by the mitochondria could be released in the extracellular spaces and induce proinflammatory responses in the surrounding tissue.165 An impairment in energy metabolism and altered lipid metabolism could result from a significant reduction in ATP concentration.166 In fishes, the accumulation of MPs in the intestinal tissue exerts functional damage, such as reducing calcium levels.167 Similarly, MPs can cause neurotoxicity and lipid peroxidation in the brain and muscles (oxidative damage) and change the activity of energy-related enzymes.168 Détrée & Gallardo-Escárate169 detected in mussels that, after exposure to MPs, for the regulation of stress proteins and the preservation of homeostasis, when oxidative stress is induced, metabolic and energetic costs are required that force a reallocation of the energy assigned to growth to achieve the maintenance of homeostasis.
Several studies have confirmed the presence of MPs inside the human body.170,171 Once inside, MPs can migrate to various tissues by translocation, move to the lymphatic system, accumulate in organs, or affect the immune system.172 MPs have been found even in the human placenta.173 MPs in the body can act as endocrine disruptors based on additives on their surface that interfere with the endocrine system's development and affect the functioning of the organs that respond to hormonal signals.1
The presence of MPs in the human respiratory system has been documented. Lung tissue obtained from autopsies, polyethylene (PE), and polypropylene (PP) microfibers (MF) were the most frequent.70 Verifying inhalation as the main route of exposure, in an analysis of human lung tissue samples, 39 MPs were detected in 11 of 13 pieces examined. Polypropylene, polyethylene terephthalate, and resin were the most abundant in lung tissue obtained from surgical resections. Compared to the superior and media/lingular lobes, the lower lobe had the highest levels of MPs.174 The amount of MF gradually increased as the patients' ages increased in the lower airways using bronchoalveolar lavage fluid (polyester MF).175 The development of pulmonary ground glass nodules (GGNs) is predisposed by a high risk of exposure to microfibers and MPs.176 Long fiber length may cause frustrated phagocytosis and increased inflammatory biomolecule production.177
Few studies assess the toxicity of MP particles in humans. Normal human lung epithelial cells showed adverse effects after exposure to polystyrene microplastics (PS-MP), causing inflammatory and oxidative stress and inducing alterations in the pulmonary barrier. The risk of chronic obstructive pulmonary disease (COPD) risk can occur with exposure to high and low levels of PS-MP. When inhaled MPs enter the respiratory system, the airway epithelium secretes numerous biochemical mediators, including ROS and cytokines, to recruit inflammatory cells, which can cause tissue damage without effective repair and alterations in airway structures.178 Lung carcinoma cells (A549 cells) had a toxic effect on nano-PET exposure, with a decrease in mitochondrial membrane potential induced by increased reactive oxygen species (ROS) caused by oxidative stress as the nano-PET dose increased. However, cell viability may occur under low nano-PET dose conditions but increasing the nano-PET dose inhibits cell viability.179 In lung epithelial cells, two sizes of polystyrene nanoparticles (PS-NP 25 nm and 70 nm) affected cell viability at high concentrations and altered gene expression, causing inflammatory responses and inducing apoptosis, with the smaller particle size exhibiting more toxicity. Therefore, the size, exposure time, and concentration of PS-NPs are factors that may cause functional alterations in the human respiratory system.180 High concentrations (100 μg mL−1) and prolonged exposure times (48 h) to waste-derived MP (mixture mainly PE and PP < 50 μm) induce inflammation and genotoxicity in alveolar lung cells (A549).181 Likewise, the activity of A549 cells decreases with a combined exposure of PS-NPs with phthalate esters (PAEs). The toxicity of PAEs depends on the concentration of PS-NPs; at lower PS-NPs concentrations, the toxicity of PAEs is reduced, but at higher PS-NPs concentrations, the toxicity is dominated by NPs.182 In addition, NPs, after prolonged exposure, can cause tissue damage, reducing the repair capacity of the lungs.183
The toxic effects of PS-NPs with a smaller size and a superficially positive charge are more potent.184 Since the size is related to translocation in tissues, studies have shown that PS-NPs have a higher absorption potential to enter the human colon and small intestine epithelial cells than PS-MPs.185 Depending on their size, MPs can enter cells through endocytosis, significantly decreasing the potential of the mitochondrial membrane. NPs can adsorb Bisphenol A (BPA). NPs can absorb more BPA due to their substantial specific surface area and cause a significant increase in toxicity to cells.186
Cellular oxidative stress is one of the mechanisms of cytotoxicity by MPs in the human brain and epithelial cells.82 Studies that simulated the early development of the human cerebral cortex discovered that exposure to PS-MPs, depending on their size and concentration, may have adverse effects.187 NPs (PET) and NPs-contaminant complexes (glyphosate, levofloxacin, and Hg2+ ions) have also been shown to impact heterogeneous human epithelial colorectal adenocarcinoma cells. NPs (PET) can adsorb pollutants. Cell physiology is significantly altered in the presence of NPs (PET) and NPs-contaminant complexes.188
Given the above, it is worthwhile further to explore the presence of MPs in the atmosphere and determine their incidence on human health since there are many risks associated with their accumulation in the body that deserve greater attention from regulatory government entities and the scientific community in general.
Discussion
This article provides integrated knowledge that explains MPs as emerging and biopersistent environmental pollutants, which are becoming a potential problem for human and animal health, focusing on their presence in the air. The concern that airborne MPs have generated in the scientific community is reflected in the fact that, although the research is at a relatively early stage, there is a clear upward trend and rapid increase in the number of publications in recent years.
In addition, although contamination by MPs has been evidenced in the different environmental compartments, the atmosphere has played a fundamental role in connecting them, favoring the source-pathway-sink network and influencing the flow and accumulation of MPs in the environmental matrices. Therefore, it is essential that future studies address this contamination globally and not consider the environments independently because they are closely related.
Primary and secondary MPs contribute to the total amount of MPs in the environment. In this task, analyzing the morphology and composition and observing the degradation of the MPs can help trace their origin. As discussed, atmospheric transport makes it possible for MPs to reach remote areas without the need for local sources of plastics, which represents a challenge in source attribution given the variability and geographic distribution of potential sources.
The tracking of atmospheric sources of MPs and the study of their transport is new in investigating MPs in the air. To describe the dynamics of the transportation of atmospheric MPs, the use of Lagrangian atmospheric models such as HYSPLIT represents valuable tools. Those models allow us to carry out a deep and detailed analysis of the movement of MPs, being also helpful for the identification of possible sources of origin and the atmospheric trajectory traveled. However, studies on the fate and transport mechanisms of microplastics in the air are still limited, and further research is required regarding the contribution of resuspension of MPs from aquatic or terrestrial environments on the abundance of airborne MPs, as well as the contribution of dry and wet atmospheric precipitation of atmospheric MPs to these environments.
On the other hand, it should be noted that the circulation of MPs in the air can be amplified by meteorological events that favor the transport of large amounts of pollutants along their path and that without the presence of significant winds, low temperatures, and excellent atmospheric stability, there would be no dispersion or dilution processes of pollutants.
Additionally, the transport of MPs also involves the transportation of contaminants that adhered to their surface during their stay in the environment, increasing the potential threat they represent to health since these contaminants can cause genotoxicity when desorbed, added to the fact that the MPs themselves and their additives lead to reproductive damage, carcinogenic effects, and mutagenicity. Knowledge about the interactions between MPs and other particles in the atmosphere and their combined influence and impact on human health is still limited; future research is expected to broaden these aspects.
Regarding the evidence collected on the possible effects of the entry of MPs into the body, given that MPs are a mixture of compounds and most toxicological tests differ in nature in contrast to environmental microparticles (in terms of surface, adsorbed compounds, and microorganisms), results obtained may be different or inaccurate with reality, so they should be considered as approximations under certain simulated conditions.
In addition, the current knowledge of the health risks due to the presence of MPs comes from mathematical models, animal studies, and in vitro tests that, although essential to identify possible adverse effects, do not replace findings obtained through studies in humans. It is necessary to advance with this focus for greater clarity regarding the real risks in the human body and, even more, to understand if the fact of presenting comorbidities that cause a decrease in epithelial barriers significantly implies an increase in permeability for MPs and, therefore, whether the people who give them would be considered as the most vulnerable population to this exposure.
Other challenges in future studies are identifying the routes of elimination of MPs from the human body and determining if a particular path of entry causes more significant effects than another. Likewise, research needs to be expanded to understand all possible access routes and their implications. Although it is well known that MPs that enter the body through inhalation and ingestion represent a significant amount, these are not the only routes of entry. Given the evidence, the possible dermal penetration and the potential exposure to MPs via the transplacental route to fetuses should be studied in depth.
Finally, it should be noted that the concern for plastic pollution does not end with MPs since these microparticles can continue to fragment to nanoplastics, whose research is much more recent, and consequently, their transport processes and interactions with the environment are unknown; due to their smaller size, the sampling and analysis protocols are more complex, and its penetration into cell barriers is further facilitated. All of the above should motivate the establishment regulatory policies for MPs, just as they exist for other air pollutants.
Conclusions
The atmosphere plays a crucial role in the global challenge posed by microplastic pollution. Understanding the source of airborne MPs is essential to reduce plastic pollution and to explore the potential source routes and transport pathways of atmospheric MPs, for what some models have been proposed.
In the atmosphere, transport, dispersion, and deposition mechanisms are the driver for MPs movement, which is influenced by their size, shape, and length. MPs can be transported to distant areas, reaching ubiquity and causing inevitable exposure. Inhalation and ingestion are the main routes by which MPs suspended in air can enter the body's interior. Once inside, they can translocate and accumulate in different organs and tissues. The toxic effects of MPs can be attributed to their physical characteristics (size, shape, and length), chemical constitution (presence of additives and type of polymer), concentration level, and the possible presence of microbial biofilms. The health detrimental lies in the ubiquitous presence, the constituents releasing and aging during permanence in the environment. Besides, due to the direct photo-irradiation, MPs aging in the atmosphere generates changes in their surface (such as roughness and hydrophobicity), which exacerbate their cytotoxicity, adsorption of contaminants, and transport of microorganisms.
MPs-induced cytotoxicity stimulates oxidative stress by generating free radicals that originate from ROS and whose overproduction can alter cell homeostasis. Inflammatory lesions, metabolic alterations, and increased risk of cancer are some of the health implications caused by MPs when they encounter the interior of the body.
Future perspectives
Significant advances have been made in understanding the sources, transport, contact routes, and factors that influence the toxicity of MPs to analyze the potential risks associated with exposure to MPs. However, there is scarce information about MPs' implications for human health. Some elements to consider advancing in this field are:
• Delve into the scope and impact of MPs by inhalation and ingestion exposure and investigate the possible dermal exposure.
• Study and propose mechanisms that describe how substances and MOs' adsorption/desorption processes occur on the surface of MPs.
• Analyze the possible toxic effects generated by the different additives and contaminants adsorbed by MPs in greater detail.
• Advance in quantifying the adsorption of atmospheric pollutants by MPs in air.
• Advances in models allow simulating of the transport of MPs, including the possible resuspension of MPs as a variable since strong winds can remobilize the deposited ones.
• Continue exploring potential interactions between airborne MPs and other air pollutants, their combined effects, and implications for ecosystems and health.
• Examine more human tissue samples for the possible presence of MPs.
Author contributions
Project conceptualization, administration, funding acquisition and supervision, C. D. G.-T., J. T., and V. A. A.; methodology, investigation, data analysis, writing—original draft preparation, A. P. A. L., and L. E. G.-A.; writing—review and editing, C. D. G.-T., J. T., and V. A. A. All authors contributed to the discussion, reviews, and approval of the manuscript for publication.
Conflicts of interest
The authors declare that they have no known conflict of interest that could have appeared to influence the work reported in this paper.
Acknowledgements
This research was funded by FONDO DE CIENCIA, TECNOLOGÍA E INNOVACIÓN DEL SISTEMA GENERAL DE REGALÍAS – Colombia (FCTeI-SGR), grant BPIN 2020000100065, Ministerio de Ciencia Tecnología e Innovación (Minciencias) – Colombia, grant 1116-852-72530 (agreement 80740-485-2020) and the Universidad del Atlántico.
References
- C. Campanale, C. Massarelli, I. Savino, V. Locaputo and V. F. Uricchio, A detailed review study on potential effects of microplastics and additives of concern on human health, Int. J. Environ. Res. Public Health, 2020, 17(4), 1212 CrossRef CAS PubMed
. - G. Croxatto Vega, A. Gross and M. Birkved, The impacts of plastic products on air pollution – A simulation study for advanced life cycle inventories of plastics covering secondary microplastic production, Sustain. Prod. Consum., 2021, 28, 848–865, DOI:10.1016/j.spc.2021.07.008
. - OECD Global, Plastics Outlook: Economic Drivers, Environmental Impacts and Policy Options, Glob Plast Outlook, 2022, available from: https://www.oecd-ilibrary.org/environment/data/global-plastic-outlook_c0821f81-en Search PubMed
. - K. Zhang, A. H. Hamidian, A. Tubić, Y. Zhang, J. K. H. Fang and C. Wu, et al., Understanding plastic degradation and microplastic formation in the environment: A review, Environ. Pollut., 2021, 274, 116554 CrossRef CAS PubMed
. - J. P. G. L. Frias and R. Nash, Microplastics: Finding a consensus on the definition, Mar. Pollut. Bull., 2019, 138, 145–147 CrossRef CAS PubMed
. - N. B. Hartmann, T. Hüffer, R. C. Thompson, M. Hassellöv, A. Verschoor and A. E. Daugaard, et al., Are We Speaking the Same Language? Recommendations for a Definition and Categorization Framework for Plastic Debris, Environ. Sci. Technol., 2019, 53(3), 1039–1047 CrossRef CAS PubMed
. - K. Jarosz, R. Janus, M. Wądrzyk, W. Wilczyńska-Michalik, P. Natkański and M. Michalik, Airborne Microplastic in the Atmospheric Deposition and How to Identify and Quantify the Threat: Semi-Quantitative Approach Based on Kraków Case Study, Int. J. Environ. Res. Public Health, 2022, 19(19), 12252 CrossRef CAS PubMed
. - A. Xu, M. Shi, X. Xing, Y. Su, X. Li and W. Liu, et al., Status and prospects of atmospheric microplastics: A review of methods, occurrence, composition, source and health risks, Environ. Pollut., 2022, 303, 119173 CrossRef CAS PubMed
. - A. Rahman, A. Sarkar, O. P. Yadav, G. Achari and J. Slobodnik, Potential human health risks due to environmental exposure to nano- and microplastics and knowledge gaps: A scoping review, Sci. Total Environ., 2021, 757, 143872, DOI:10.1016/j.scitotenv.2020.143872
. - M. B. Ahmed, M. S. Rahman, J. Alom, M. S. Hasan, M. A. H. Johir and M. I. H. Mondal, et al., Microplastic particles in the aquatic environment: A systematic review, Sci. Total Environ., 2021, 775, 145793, DOI:10.1016/j.scitotenv.2021.145793
. - B. R. Kiran, H. Kopperi and S. Venkata Mohan, Micro/nano-plastics occurrence, identification, risk analysis and mitigation: challenges and perspectives, Reviews in Environmental Science and Biotechnology, Springer Netherlands, 2022, vol. 21, pp. 169–203, DOI:10.1007/s11157-021-09609-6
. - M. Kooi and A. A. Koelmans, Simplifying Microplastic via Continuous Probability Distributions for Size, Shape,and Density, Environ. Sci. Technol. Lett., 2019, 6(9), 551–557 CrossRef CAS
. - J. Gong and P. Xie, Research progress in sources, analytical methods, eco-environmental effects, and control measures of microplastics, Chemosphere, 2020, 254, 126790, DOI:10.1016/j.chemosphere.2020.126790
. - O. S. Alimi, J. Farner Budarz, L. M. Hernandez and N. Tufenkji, Microplastics and Nanoplastics in Aquatic Environments: Aggregation, Deposition, and Enhanced Contaminant Transport, Environ. Sci. Technol., 2018, 52(4), 1704–1724 CrossRef CAS PubMed
. - B. Xu, F. Liu, Z. Cryder, D. Huang, Z. Lu and Y. He, et al., Microplastics in the soil environment: Occurrence, risks, interactions and fate–A review, Crit. Rev. Environ. Sci. Technol., 2020, 50(21), 2175–2222, DOI:10.1080/10643389.2019.1694822
. - R. Qi, D. L. Jones, Z. Li, Q. Liu and C. Yan, Behavior of microplastics and plastic film residues in the soil environment: A critical review, Sci. Total Environ., 2020, 703, 134722, DOI:10.1016/j.scitotenv.2019.134722
. - R. Dris, J. Gasperi, V. Rocher, M. Saad, N. Renault and B. Tassin, Microplastic contamination in an urban area: A case study in Greater Paris, Environ. Chem., 2015, 12(5), 592–599 CrossRef CAS
. - J. C. Prata, Airborne microplastics: Consequences to human health?, Environ. Pollut., 2018, 234, 115–126, DOI:10.1016/j.envpol.2017.11.043
. - C. E. Enyoh, A. W. Verla, E. N. Verla, F. C. Ibe and C. E. Amaobi, Airborne microplastics: a review study on method for analysis, occurrence, movement and risks, Environ. Monit. Assess., 2019, 191(11), 1–17 CrossRef PubMed
. - G. Chen, Q. Feng and J. Wang, Mini-review of microplastics in the atmosphere and their risks to humans, Sci. Total Environ., 2020, 703, 135504, DOI:10.1016/j.scitotenv.2019.135504
. - O. Mbachu, G. Jenkins, C. Pratt and P. Kaparaju, A New Contaminant Superhighway? A Review of Sources, Measurement Techniques and Fate of Atmospheric Microplastics, Water, Air, Soil Pollut., 2020, 231(2), 1–27 CrossRef
. - S. Allen, D. Allen, V. R. Phoenix, G. Le Roux, P. Durántez Jiménez and A. Simonneau, et al., Atmospheric transport and deposition of microplastics in a remote mountain catchment, Nat. Geosci., 2019, 12(5), 339–344 CrossRef CAS
. - N. Evangeliou, H. Grythe, Z. Klimont, C. Heyes, S. Eckhardt and S. Lopez-Aparicio, et al., Atmospheric transport is a major pathway of microplastics to remote regions, Nat. Commun., 2020, 11(1), 3381, DOI:10.1038/s41467-020-17201-9
. - D. Huang, J. Tao, M. Cheng, R. Deng, S. Chen and L. Yin, et al., Microplastics and nanoplastics in the environment: Macroscopic transport and effects on creatures, J. Hazard. Mater., 2021, 407, 124399, DOI:10.1016/j.jhazmat.2020.124399
. - Y. Zhang, S. Kang, S. Allen, D. Allen, T. Gao and M. Sillanpää, Atmospheric microplastics: A review on current status and perspectives, Earth-Sci. Rev., 2020, 203, 103118, DOI:10.1016/j.earscirev.2020.103118
. - P. A. Stapleton, Microplastic and nanoplastic transfer, accumulation, and toxicity in humans, Curr. Opin. Toxicol., 2021, 28, 62–69, DOI:10.1016/j.cotox.2021.10.001
. - S. Abbasi, Routes of human exposure to micro(nano)plastics, Curr. Opin. Toxicol., 2021, 27, 41–46, DOI:10.1016/j.cotox.2021.08.004
. - J. C. Prata, J. P. da Costa, I. Lopes, A. C. Duarte and T. Rocha-Santos, Environmental exposure to microplastics: An overview on possible human health effects, Sci. Total Environ., 2020, 702, 134455, DOI:10.1016/j.scitotenv.2019.134455
. - M. J. Page, J. E. McKenzie, P. M. Bossuyt, I. Boutron, T. C. Hoffmann and C. D. Mulrow, et al., The PRISMA 2020 statement: An updated guideline for reporting systematic reviews, BMJ, 2021, 372, 71 CrossRef PubMed
. - R. Dris, J. Gasperi, M. Saad, C. Mirande and B. Tassin, Synthetic fibers in atmospheric fallout: A source of microplastics in the environment?, Mar. Pollut. Bull., 2016, 104(1–2), 290–293, DOI:10.1016/j.marpolbul.2016.01.006
. - B. Nowack, Y. Cai, D. M. Mitrano and R. Hufenus, Formation of fiber fragments during abrasion of polyester textiles, Environ. Sci. Technol., 2021, 55(12), 8001–8009 CrossRef PubMed
. - L. Hu, J. Fu, S. Wang, Y. Xiang and X. Pan, Microplastics generated under simulated fire scenarios: Characteristics, antimony leaching, and toxicity, Environ. Pollut., 2021, 269, 115905, DOI:10.1016/j.envpol.2020.115905
. - T. Hu, P. He, Z. Yang, W. Wang, H. Zhang and L. Shao, et al., Emission of airborne microplastics from municipal solid waste transfer stations in downtown, Sci. Total Environ., 2022, 828, 154400, DOI:10.1016/j.scitotenv.2022.154400
. - S. Abbasi, M. Rezaei, F. Ahmadi and A. Turner, Atmospheric transport of microplastics during a dust storm, Chemosphere, 2022, 292, 133456, DOI:10.1016/j.chemosphere.2021.133456
. - J. Sun, S. S. H. Ho, X. Niu, H. Xu, L. Qu and Z. Shen, et al., Explorations of tire and road wear microplastics in road dust PM2.5 at eight megacities in China, Sci. Total Environ., 2022, 823, 153717, DOI:10.1016/j.scitotenv.2022.153717
. - T. Mehmood and L. Peng, Polyethylene scaffold net and synthetic grass fragmentation: a source of microplastics in the atmosphere?, J. Hazard. Mater., 2022, 429, 128391, DOI:10.1016/j.jhazmat.2022.128391
. - Y. Chen, X. Li, X. Zhang, Y. Zhang, W. Gao and R. Wang, et al., Air conditioner filters become sinks and sources of indoor microplastics fibers, Environ. Pollut., 2022, 292(PB), 118465, DOI:10.1016/j.envpol.2021.118465
. - S. Uddin, S. W. Fowler, N. Habibi, S. Sajid, S. Dupont and M. Behbehani, Indoor Aerosol—Kuwait ’ s Baseline, Toxics, 2022, 2–17 Search PubMed
. - N. S. Soltani, M. P. Taylor and S. P. Wilson, Quantification and exposure assessment of microplastics in Australian indoor house dust, Environ. Pollut., 2021, 283 Search PubMed
. - I. Bahrina, A. D. Syafei, R. Satoto, J. J. Jiang, N. R. Nurasrin and A. F. Assomadi, et al., An Occupant-Based Overview of Microplastics in Indoor Environments in the City of Surabaya, Indonesia, J. Ecol. Eng., 2020, 21(8), 236–242 CrossRef
. - E. Y. Chen, K. T. Lin, C. C. Jung, C. L. Chang and C. Y. Chen, Characteristics and influencing factors of airborne microplastics in nail salons, Sci. Total Environ., 2022, 151472, DOI:10.1016/j.scitotenv.2021.151472
. - Z. Sobhani, Y. Lei, Y. Tang, L. Wu, X. Zhang and R. Naidu, et al., Microplastics generated when opening plastic packaging, Sci. Rep., 2020, 10(1), 1–7, DOI:10.1038/s41598-020-61146-4
. - K. Liu, T. Wu, X. Wang, Z. Song, C. Zong and N. Wei, et al., Consistent Transport of Terrestrial Microplastics to the Ocean through Atmosphere, Environ. Sci. Technol., 2019, 53(18), 10612–10619 CrossRef CAS PubMed
. - H. Yang, Y. He, Y. Yan, M. Junaid and J. Wang, Characteristics, toxic effects, and analytical methods of microplastics in the atmosphere, Nanomaterials, 2021, 11(10), 1–21 Search PubMed
. - Y. Li, L. Shao, W. Wang, M. Zhang, X. Feng and W. Li, et al., Airborne fiber particles: Types, size and concentration observed in Beijing, Sci. Total Environ., 2020, 705, 135967, DOI:10.1016/j.scitotenv.2019.135967
. - A. Stubbins, K. L. Law, S. E. Muñoz, T. S. Bianchi and L. Zhu, Plastics in the Earth system, Science, 2021, 373(6550), 51–55 CrossRef CAS PubMed
. - A. L. Andrady, P. W. Barnes, J. F. Bornman, T. Gouin, S. Madronich and C. C. White, et al., Oxidation and fragmentation of plastics in a changing environment; from UV-radiation to biological degradation, Sci. Total Environ., 2022, 851, 158022, DOI:10.1016/j.scitotenv.2022.158022
. - J. N. Crowley, M. Ammann, R. A. Cox, R. G. Hynes, M. E. Jenkin and A. Mellouki, et al., Evaluated kinetic and photochemical data for atmospheric chemistry: Volume v -heterogeneous reactions on solid substrates, Atmos. Chem. Phys., 2010, 10(18), 9059–9223 CrossRef CAS
. - X. Wang, K. Liu, L. Zhu, C. Li, Z. Song and D. Li, Efficient transport
of atmospheric microplastics onto the continent via the East Asian summer monsoon, J. Hazard. Mater., 2021, 414, 125477, DOI:10.1016/j.jhazmat.2021.125477
. - M. C. Jong, X. Tong, J. Li, Z. Xu, S. H. Q. Chng and Y. He, et al., Microplastics in equatorial coasts: Pollution hotspots and spatiotemporal variations associated with tropical monsoons, J. Hazard. Mater., 2022, 424(PC), 127626, DOI:10.1016/j.jhazmat.2021.127626
. - K. Szewc, B. Graca and A. Dołęga, Atmospheric deposition of microplastics in the coastal zone: Characteristics and relationship with meteorological factors, Sci. Total Environ., 2021, 761, 143272 CrossRef CAS PubMed
. - J. Brahney, M. Hallerud, E. Heim, M. Hahnenberger and S. Sukumaran, Plastic rain in protected areas of the United States, Science, 2020, 368(6496), 1257–1260 CrossRef CAS PubMed
. - K. Liu, X. Wang, Z. Song, N. Wei and D. Li, Terrestrial plants as a potential temporary sink of atmospheric microplastics during transport, Sci. Total Environ., 2020, 742, 140523, DOI:10.1016/j.scitotenv.2020.140523
. - K. Liu, X. Wang, T. Fang, P. Xu, L. Zhu and D. Li, Source and potential risk assessment of suspended atmospheric microplastics in Shanghai, Sci. Total Environ., 2019, 675, 462–471, DOI:10.1016/j.scitotenv.2019.04.110
. - M. Rezaei, M. J. P. M. Riksen, E. Sirjani, A. Sameni and V. Geissen, Wind erosion as a driver for transport of light density microplastics, Sci. Total Environ., 2019, 669, 273–281, DOI:10.1016/j.scitotenv.2019.02.382
. - Y. Ding, X. Zou, C. Wang, Z. Feng, Y. Wang and Q. Fan, et al., The abundance and characteristics of atmospheric microplastic deposition in the northwestern South China Sea in the fall, Atmos. Environ., 2021, 253, 118389, DOI:10.1016/j.atmosenv.2021.118389
. - Q. Zhang, Y. Zhao, F. Du, H. Cai, G. Wang and H. Shi, Microplastic Fallout in Different Indoor Environments, Environ. Sci. Technol., 2020, 54(11), 6530–6539 CrossRef CAS PubMed
. - R. Dris, J. Gasperi, C. Mirande, C. Mandin, M. Guerrouache and V. Langlois, et al., A first overview of textile fibers, including microplastics, in indoor and outdoor environments, Environ. Pollut., 2017, 221, 453–458 CrossRef CAS PubMed
. - Y. Xie, Y. Li, Y. Feng, W. Cheng and Y. Wang, Inhalable microplastics prevails in air: Exploring the size detection limit, Environ. Int., 2022, 162, 107151, DOI:10.1016/j.envint.2022.107151
. - S. Abbasi, B. Keshavarzi, F. Moore, A. Turner, F. J. Kelly and A. O. Dominguez, et al., Distribution and potential health impacts of microplastics and microrubbers in air and street dusts from Asaluyeh County, Iran, Environ. Pollut., 2019, 244, 153–164, DOI:10.1016/j.envpol.2018.10.039
. - S. L. Wright, J. Ulke, A. Font, K. L. A. Chan and F. J. Kelly, Atmospheric microplastic deposition in an urban environment and an evaluation of transport, Environ. Int., 2020, 136, 105411, DOI:10.1016/j.envint.2019.105411
. - K. Liu, X. Wang, N. Wei, Z. Song and D. Li, Accurate quantification and transport estimation of suspended atmospheric microplastics in megacities: Implications for human health, Environ. Int., 2019, 132, 105127, DOI:10.1016/j.envint.2019.105127
. - X. Wang, C. Li, K. Liu, L. Zhu, Z. Song and D. Li, Atmospheric microplastic over the South China Sea and East Indian Ocean: abundance, distribution and source, J. Hazard. Mater., 2020, 389, 121846, DOI:10.1016/j.jhazmat.2019.121846
. - S. Kernchen, M. G. J. Löder, F. Fischer, D. Fischer, S. R. Moses and C. Georgi, et al., Airborne microplastic concentrations and deposition
across the Weser River catchment, Sci. Total Environ., 2022, 818 Search PubMed
. - K. D. Cox, G. A. Covernton, H. L. Davies, J. F. Dower, F. Juanes and S. E. Dudas, Human Consumption of Microplastics, Environ. Sci. Technol., 2019, 53(12), 7068–7074 CrossRef CAS PubMed
. - A. Vianello, R. L. Jensen, L. Liu and J. Vollertsen, Simulating human exposure to indoor airborne microplastics using a Breathing Thermal Manikin, Sci. Rep., 2019, 9(1), 1–11, DOI:10.1038/s41598-019-45054-w
. - Q. Zhang, E. G. Xu, J. Li, Q. Chen, L. Ma and E. Y. Zeng, et al., A Review of Microplastics in Table Salt, Drinking Water, and Air: Direct Human Exposure, Environ. Sci. Technol., 2020, 54(7), 3740–3751 CrossRef CAS PubMed
. - R. Akhbarizadeh, S. Dobaradaran, M. Amouei Torkmahalleh, R. Saeedi, R. Aibaghi and F. Faraji Ghasemi, Suspended fine particulate matter (PM2.5), microplastics (MPs), and polycyclic aromatic hydrocarbons (PAHs) in air: Their possible relationships and health implications, Environ. Res., 2021, 192, 110339, DOI:10.1016/j.envres.2020.110339
. - J. Gasperi, S. L. Wright, R. Dris, F. Collard, C. Mandin and M. Guerrouache, et al., Microplastics in air: Are we breathing it in?, Curr. Opin. Environ. Sci. Heal., 2018, 1, 1–5, DOI:10.1016/j.coesh.2017.10.002
. - L. F. Amato-Lourenço, R. Carvalho-Oliveira, G. R. Júnior, L. dos Santos Galvão, R. A. Ando and T. Mauad, Presence of airborne microplastics in human lung tissue, J. Hazard. Mater., 2021, 416, 126124 CrossRef PubMed
. - L. Mai, L. J. Bao, L. Shi, C. S. Wong and E. Y. Zeng, A review of methods for measuring microplastics in aquatic environments, Environ. Sci. Pollut. Res., 2018, 25(12), 11319–11332 CrossRef CAS PubMed
. - S. Dehghani, F. Moore and R. Akhbarizadeh, Microplastic pollution in deposited urban dust, Tehran metropolis, Iran, Environ. Sci. Pollut. Res., 2017, 24(25), 20360–20371 CrossRef CAS PubMed
. - A. I. Catarino, V. Macchia, W. G. Sanderson, R. C. Thompson and T. B. Henry, Low levels of microplastics (MP) in wild mussels indicate that MP ingestion by humans is minimal compared to exposure via household fibres fallout during a meal, Environ. Pollut., 2018, 237, 675–684, DOI:10.1016/j.envpol.2018.02.069
. - R. Dris, First assessment of sources and fate of macro and micro plastics in urban hydrosystems : Case of Paris megacity, 2017, vol. 248 Search PubMed
. - M. Fang, Z. Liao, X. Ji, X. Zhu, Z. Wang and C. Lu, et al., Microplastic ingestion from atmospheric deposition during dining/drinking activities, J. Hazard. Mater., 2022, 432, 128674, DOI:10.1016/j.jhazmat.2022.128674
. - Q. Zhang, F. Du, W. Liang, Q. Chen, J. Meng and H. Shi, Microfiber fallout during dining and potential human intake, J. Hazard. Mater., 2022, 430, 128477, DOI:10.1016/j.jhazmat.2022.128477
. - M. Zhang, L. Liu, D. Xu, B. Zhang, J. Li and B. Gao, Small-sized microplastics (< 500 μm) in roadside soils of Beijing, China: Accumulation, stability, and human exposure risk, Environ. Pollut., 2022, 304, 119121, DOI:10.1016/j.envpol.2022.119121
. - C. Liu, J. Li, Y. Zhang, L. Wang, J. Deng and Y. Gao, et al., Widespread distribution of PET and PC microplastics in dust in urban China and their estimated human exposure, Environ. Int., 2019, 128, 116–124 CrossRef CAS PubMed
. - M. Javad, F. Zarei, B. Keshavarzi, M. Zarei, F. Moore and R. Busquets, et al., Science of the Total Environment Microplastic occurrence in settled indoor dust in schools, Sci. Total Environ., 2022, 807, 150984, DOI:10.1016/j.scitotenv.2021.150984
. - J. Zhang, L. Wang and K. Kannan, Microplastics in house dust from 12 countries and associated human exposure, Environ. Int., 2020, 134, 105314, DOI:10.1016/j.envint.2019.105314
. - C. Lassen, S. F. Hansen, K. Magnusson, N. B. Hartmann, P. Rehne Jensen, T. G. Nielsen and A. Brinch, Microplastics Occurrence, Effects and Sources of Releases, Environmental Project No. 1793, 2015, Danish Environmental Protection Agency, Copenh. 2016 Search PubMed
. - G. F. Schirinzi, I. Pérez-Pomeda, J. Sanchís, C. Rossini, M. Farré and D. Barceló, Cytotoxic effects of commonly used nanomaterials and microplastics on cerebral and epithelial human cells, Environ. Res., 2017, 159, 579–587, DOI:10.1016/j.envres.2017.08.043
. - M. Revel, A. Châtel and C. Mouneyrac, Micro(nano)plastics: A threat to human health?, Curr. Opin. Environ. Sci. Heal., 2018, 1, 17–23, DOI:10.1016/j.coesh.2017.10.003
. - P. Ebrahimi, S. Abbasi, R. Pashaei, A. Bogusz and P. Oleszczuk, Investigating impact of physicochemical properties of microplastics on human health: A short bibliometric analysis and review, Chemosphere, 2022, 289, 133146, DOI:10.1016/j.chemosphere.2021.133146
. - M. Kumar, H. Chen, S. Sarsaiya, S. Qin, H. Liu and M. K. Awasthi, et al., Current research trends on micro- and nano-plastics as an emerging threat to global environment: A review, J. Hazard. Mater., 2021, 409, 124967, DOI:10.1016/j.jhazmat.2020.124967
. - D. Hou, M. Hong, Y. Wang, P. Dong, H. Cheng and H. Yan, et al., Assessing the risks of potential bacterial pathogens attaching to different microplastics during the summer–autumn period in a mariculture cage, Microorganisms, 2021, 9(9), 1909 CrossRef CAS PubMed
. - G. Gkoutselis, S. Rohrbach, J. Harjes, M. Obst, A. Brachmann and M. A. Horn, et al., Microplastics accumulate fungal pathogens in terrestrial ecosystems, Sci. Rep., 2021, 11(1), 1–14, DOI:10.1038/s41598-021-92405-7
. - I. V. Kirstein, S. Kirmizi, A. Wichels, A. Garin-Fernandez, R. Erler and M. Löder, et al., Dangerous hitchhikers? Evidence for potentially pathogenic Vibrio spp. on microplastic particles, Mar. Environ. Res., 2016, 120, 1–8, DOI:10.1016/j.marenvres.2016.07.004
. - Y. Wang, X. Wang, Y. Li, J. Li, Y. Liu and S. Xia, et al., Effects of exposure of polyethylene microplastics to air, water and soil on their adsorption behaviors for copper and tetracycline, Chem. Eng. J., 2021, 404, 126412, DOI:10.1016/j.cej.2020.126412
. - L. Bradney, H. Wijesekara, K. N. Palansooriya, N. Obadamudalige, N. S. Bolan and Y. S. Ok, et al., Particulate plastics as a vector for toxic trace-element uptake by aquatic and terrestrial organisms and human health risk, Environ. Int., 2019, 131, 104937, DOI:10.1016/j.envint.2019.104937
. - S. B. Fournier, J. N. D'Errico, D. S. Adler, S. Kollontzi, M. J. Goedken and L. Fabris, et al., Nanopolystyrene translocation and fetal deposition after acute lung exposure during late-stage pregnancy, Part. Fibre Toxicol., 2020, 17(1), 1–12 CrossRef PubMed
. - L. Zimmermann, A. Dombrowski, C. Völker and M. Wagner, Are bioplastics and plant-based materials safer than conventional plastics? In vitro toxicity and chemical composition, Environ. Int., 2020, 145, 106066, DOI:10.1016/j.envint.2020.106066
. - S. O'Brien, E. D. Okoffo, C. Rauert, J. W. O'Brien, F. Ribeiro and S. D. Burrows, et al., Quantification of selected microplastics in Australian urban road dust, J. Hazard. Mater., 2021, 416, 125811, DOI:10.1016/j.jhazmat.2021.125811
. - Z. Ouyang, R. Mao, E. Hu, C. Xiao, C. Yang and X. Guo, The indoor exposure of microplastics in different environments, Gondwana Res., 2022, 108, 193–199, DOI:10.1016/j.gr.2021.10.023
. - A. Patchaiyappan, K. Dowarah, S. Zaki Ahmed, M. Prabakaran, S. Jayakumar and C. Thirunavukkarasu, et al., Prevalence and characteristics of microplastics present in the street dust collected from Chennai metropolitan city, India, Chemosphere, 2021, 269, 128757, DOI:10.1016/j.chemosphere.2020.128757
. - Z. Liao, X. Ji, Y. Ma, B. Lv, W. Huang and X. Zhu, et al., Airborne microplastics in indoor and outdoor environments of a coastal city in Eastern China, J. Hazard. Mater., 2021, 417, 126007, DOI:10.1016/j.jhazmat.2021.126007
. - M. Klein and E. K. Fischer, Microplastic abundance in atmospheric deposition within the Metropolitan area of Hamburg, Germany, Sci. Total Environ., 2019, 685, 96–103, DOI:10.1016/j.scitotenv.2019.05.405
. - J. N. Hahladakis, C. A. Velis, R. Weber, E. Iacovidou and P. Purnell, An overview of chemical additives present in plastics: Migration, release, fate and environmental impact during their use, disposal and recycling, J. Hazard. Mater., 2018, 344, 179–199, DOI:10.1016/j.jhazmat.2017.10.014
. - E. A. Godwill, P. U. Ferdinand and M. Nwalo NFUnachukwu, Mechanism and Health Effects of Heavy Metal Toxicity in Humans, 2019, pp. 1–23 Search PubMed
. - A. T. Jan, M. Azam, K. Siddiqui, A. Ali, I. Choi and Q. M. R. Haq, Heavy metals and human health: Mechanistic insight into toxicity and counter defense system of antioxidants, Int. J. Mol. Sci., 2015, 16(12), 29592–29630 CrossRef CAS PubMed
. - H. Luo, C. Liu, D. He, J. Xu, J. Sun and J. Li, et al., Environmental behaviors of microplastics in aquatic systems: A systematic review on degradation, adsorption, toxicity and biofilm under aging conditions, J. Hazard. Mater., 2022, 423(PA), 126915, DOI:10.1016/j.jhazmat.2021.126915
. - Y. Yao, M. Glamoclija, A. Murphy and Y. Gao, Characterization of microplastics in indoor and ambient air in northern New Jersey, Environ. Res., 2022, 207, 112142, DOI:10.1016/j.envres.2021.112142
. - S. Oberbeckmann, C. M. G. J. Lo and M. Labrenz, Marine microplastic-associated biofilms – a review, Environ. Chem., 2015, 12, 551–562 CrossRef CAS
. - X. Wu, J. Pan, M. Li, Y. Li, M. Bartlam and Y. Wang, Selective enrichment of bacterial pathogens by microplastic biofilm, Water Res., 2019, 165, 114979, DOI:10.1016/j.watres.2019.114979
. - M. M. Silva, G. C. Maldonado, R. O. Castro, J. de Sá Felizardo, R. P. Cardoso and R. M. dos Anjos, et al., Dispersal of potentially pathogenic bacteria by plastic debris in Guanabara Bay, RJ, Brazil, Mar. Pollut. Bull., 2019, 141, 561–568, DOI:10.1016/j.marpolbul.2019.02.064
. - F. K. Mammo, I. D. Amoah, K. M. Gani, L. Pillay, S. K. Ratha and F. Bux, et al., Microplastics in the environment: Interactions with microbes and chemical contaminants, Sci. Total Environ., 2020, 743, 140518, DOI:10.1016/j.scitotenv.2020.140518
. - Y. R. Kim, F. A. Harden, L. M. L. Toms and R. E. Norman, Health consequences of exposure to brominated flame retardants: A systematic review, Chemosphere, 2014, 106, 1–19, DOI:10.1016/j.chemosphere.2013.12.064
. - A. Turner, PBDEs in the marine environment: Sources, pathways and the role of microplastics, Environ. Pollut., 2022, 301, 118943, DOI:10.1016/j.envpol.2022.118943
. - A. Turner and M. Filella, Hazardous metal additives in plastics and their environmental impacts, Environ. Int., 2021, 156, 106622, DOI:10.1016/j.envint.2021.106622
. - M. Filella, P. Hennebert, G. Okkenhaug and A. Turner, Occurrence and fate of antimony in plastics, J. Hazard. Mater., 2020, 390, 121764, DOI:10.1016/j.jhazmat.2019.121764
. - A. Turner, Cadmium pigments in consumer products and their health risks, Sci. Total Environ., 2019, 657, 1409–1418, DOI:10.1016/j.scitotenv.2018.12.096
. - A. Massos and A. Turner, Cadmium, lead and bromine in beached microplastics, Environ. Pollut., 2017, 227, 139–145, DOI:10.1016/j.envpol.2017.04.034
. - H. K. Imhof, C. Laforsch, A. C. Wiesheu, J. Schmid, P. M. Anger and R. Niessner, et al., Pigments and plastic in limnetic ecosystems: A qualitative and quantitative study on microparticles of different size classes, Water Res., 2016, 98, 64–74, DOI:10.1016/j.watres.2016.03.015
. - D. Gandamalla, H. Lingabathula and N. Yellu, Nano titanium exposure induces dose- and size-dependent cytotoxicity on human epithelial lung and colon cells, Drug Chem. Toxicol., 2019, 42(1), 24–34 CrossRef CAS PubMed
. - M. Sendra, P. Pereiro, A. Figueras and B. Novoa, An integrative toxicogenomic analysis of plastic additives, J. Hazard. Mater., 2021, 409, 124975, DOI:10.1016/j.jhazmat.2020.124975
. - N. Medda, S. K. De and S. Maiti, Different mechanisms of arsenic related signaling in cellular proliferation, apoptosis and neo-plastic transformation, Ecotoxicol. Environ. Saf., 2021, 208, 111752, DOI:10.1016/j.ecoenv.2020.111752
. - L. Leyssens, B. Vinck, C. Van Der Straeten, F. Wuyts and L. Maes, Cobalt toxicity in humans—A review of the potential sources and systemic health effects, Toxicology, 2017, 387, 43–56, DOI:10.1016/j.tox.2017.05.015
. - Z. M. Zhang, L. Y. Wang, Y. Y. Gu, A. L. Sun, J. J. You and X. Z. Shi, et al., Probing the contamination characteristics, mobility, and risk assessments of typical plastic additive–phthalate esters from a typical coastal aquaculture area, China, J. Hazard. Mater., 2021, 416, 125931, DOI:10.1016/j.jhazmat.2021.125931
. - Z. Xu, X. Xiong, Y. Zhao, W. Xiang and C. Wu, Pollutants delivered every day: Phthalates in plastic express packaging bags and their leaching potential, J. Hazard. Mater., 2020, 384, 121282, DOI:10.1016/j.jhazmat.2019.121282
. - H. C. Erythropel, M. Maric, J. A. Nicell, R. L. Leask and V. Yargeau, Leaching of the plasticizer di(2-ethylhexyl)phthalate (DEHP) from plastic containers and the question of human exposure, Appl. Microbiol. Biotechnol., 2014, 98(24), 9967–9981 CrossRef CAS PubMed
. - W. Kouidhi, L. Thannimalay, C. S. Soon and M. A. Mohd, Occupational exposure to bisphenol a (BPA) in a plastic injection molding factory in Malaysia, Int. J. Occup. Med. Environ. Health, 2017, 30(5), 743–750 Search PubMed
. - H. Hirai, H. Takada, Y. Ogata, R. Yamashita, K. Mizukawa and M. Saha, et al., Organic micropollutants in marine plastics debris from the open ocean and remote and urban beaches, Mar. Pollut. Bull., 2011, 62(8), 1683–1692, DOI:10.1016/j.marpolbul.2011.06.004
. - W. Wei, Q. S. Huang, J. Sun, J. Y. Wang, S. L. Wu and B. J. Ni, Polyvinyl Chloride Microplastics Affect Methane Production from the Anaerobic Digestion of Waste Activated Sludge through Leaching Toxic Bisphenol-A, Environ. Sci. Technol., 2019, 53(5), 2509–2517 CrossRef CAS PubMed
. - R. Han, C. Yu, X. Tang, S. Yu, M. Song and F. Shen, et al., Release of inhalable particles and viable microbes to the air during packaging peeling: Emission profiles and mechanisms, Environ. Pollut., 2021, 285, 117338, DOI:10.1016/j.envpol.2021.117338
. - N. van Doremalen, T. Bushmaker and D. Morris, Aerosol and Surface Stability of SARS-CoV-2 as Compared with SARS-CoV-1, N. Engl. J. Med., 2020, 382(16), 1564–1567 CrossRef PubMed
. - L. F. Amato-Lourenço, N. de Souza Xavier Costa, K. C. Dantas, L. dos Santos Galvão, F. N. Moralles and S. C. F. S. Lombardi, et al., Airborne microplastics and SARS-CoV-2 in total suspended particles in the area surrounding the largest medical centre in Latin America, Environ. Pollut., 2022, 292, 118299 CrossRef PubMed
. - S. Oberbeckmann, A. M. Osborn and M. B. Duhaime, Microbes on a bottle: Substrate, season and geography influence community composition of microbes colonizing marine plastic debris, PLoS One, 2016, 11(8), 1–25, DOI:10.1371/journal.pone.0159289
. - Y. Zhang, J. Lu, J. Wu, J. Wang and Y. Luo, Potential risks of microplastics combined with superbugs: Enrichment of antibiotic resistant bacteria on the surface of microplastics in mariculture system, Ecotoxicol. Environ. Saf., 2020, 187, 109852, DOI:10.1016/j.ecoenv.2019.109852
. - C. Baker-Austin, J. D. Oliver, M. Alam, A. Ali, M. K. Waldor and F. Qadri, et al., Vibrio spp. infections, Nat. Rev. Dis. Primers, 2018, 4(1), 1–19, DOI:10.1038/s41572-018-0005-8
. - P. Ioannou and G. Vougiouklakis, A Systematic Review of Human Infections by Pseudomonas mendocina, Trop. Med. Infect Dis., 2020, 5(2), 1–9 Search PubMed
. - J. Jang, H. G. Hur, M. J. Sadowsky, M. N. Byappanahalli, T. Yan and S. Ishii, Environmental Escherichia coli: ecology and public health implications—a review, J. Appl. Microbiol., 2017, 123(3), 570–581 CrossRef CAS PubMed
. - C. Gostinčar, J. Zajc, M. Lenassi, A. Plemenitaš, S. de Hoog and A. M. S. Al-Hatmi, et al., Fungi between extremotolerance and opportunistic pathogenicity on humans, Fungal Diversity, 2018, 93(1), 195–213 CrossRef
. - Ü. N. Tavşanoğlu, G. Başaran Kankılıç, G. Akca, T. Çırak and Ş. Erdoğan, Microplastics in a dam lake in Turkey: type, mesh size effect, and bacterial biofilm communities, Environ. Sci. Pollut. Res., 2020, 27(36), 45688–45698 CrossRef PubMed
. - J. Garnacho-Montero and J. F. Timsit, Managing Acinetobacter baumannii infections, Curr. Opin. Infect. Dis., 2019, 32(1), 69–76 CrossRef PubMed
. - N. Fernández-Hidalgo, L. Escolà-Vergé and J. M. Pericàs, Enterococcus faecalis endocarditis: What's next?, Future Microbiol., 2020, 15(5), 349–364 CrossRef PubMed
. - J. Shi, D. Wu, Y. Su and B. Xie, Selective enrichment of antibiotic resistance genes and pathogens on polystyrene microplastics in landfill leachate, Sci. Total Environ., 2021, 765, 142775, DOI:10.1016/j.scitotenv.2020.142775
. - P. Ioannou, V. Mavrikaki and D. P. Kofteridis, Infective endocarditis by Acinetobacter species: a systematic review, J. Chemother., 2021, 33(4), 203–215, DOI:10.1080/1120009X.2020.1812804
. - D. N. Pham, L. Clark and M. Li, Microplastics as hubs enriching antibiotic-resistant bacteria and pathogens in municipal activated sludge, J. Hazard. Mater. Lett., 2021, 2, 100014, DOI:10.1016/j.hazl.2021.100014
. - W. J. Looney, M. Narita and K. Mühlemann, Stenotrophomonas maltophilia: an emerging opportunist human pathogen, Lancet Infect. Dis., 2009, 9(5), 312–323, DOI:10.1016/S1473-3099(09)70083-0
. - P. Seng, B. M. Boushab, F. Romain, F. Gouriet, N. Bruder and C. Martin, et al., Emerging role of Raoultella ornithinolytica in human infections: A series of cases and review of the literature, Int. J. Infect. Dis., 2016, 45, 65–71 CrossRef PubMed
. - A. Brito-Azevedo, E. C. Pinto, G. A. de Cata Preta Corrêa and E. Bouskela, SARS-CoV-2 infection causes pulmonary shunt by vasodilatation, J. Med. Virol., 2021, 93(1), 573–575 CrossRef CAS PubMed
. - G. Marasco, M. V. Lenti, C. Cremon, M. R. Barbaro, V. Stanghellini and A. Di Sabatino, et al., Implications of SARS-CoV-2 infection for neurogastroenterology, Neurogastroenterol. Motil., 2021, 33(3), 1–16 CrossRef PubMed
. - Z. Wang, J. Gao, Y. Zhao, H. Dai, J. Jia and D. Zhang, Plastisphere enrich antibiotic resistance genes and potential pathogenic bacteria in sewage with pharmaceuticals, Sci. Total Environ., 2021, 768, 144663, DOI:10.1016/j.scitotenv.2020.144663
. - L. Mughini-Gras, R. Q. J. van der Plaats, P. W. J. J. van der Wielen, P. S. Bauerlein and A. M. de Roda Husman, Riverine microplastic and microbial community compositions: A field study in the netherlands, Water Res., 2021, 192, 116852, DOI:10.1016/j.watres.2021.116852
. - Y. Zhao, J. Gao, Z. Wang, H. Dai and Y. Wang, Responses of bacterial communities and resistance genes on microplastics to antibiotics and heavy metals in sewage environment, J. Hazard. Mater., 2021, 402, 123550, DOI:10.1016/j.jhazmat.2020.123550
. - S. Ferreira, J. A. Queiroz, M. Oleastro and F. C. Domingues, Insights in the pathogenesis and resistance of Arcobacter: A review, Crit. Rev. Microbiol., 2016, 42(3), 364–383 CAS
. - L. Gao, D. Fu, J. Zhao, W. Wu, Z. Wang and Y. Su, et al., Microplastics aged in various environmental media exhibited strong sorption to heavy metals in seawater, Mar. Pollut. Bull., 2021, 169, 112480, DOI:10.1016/j.marpolbul.2021.112480
. - K. Zhu, H. Jia, W. Jiang, Y. Sun, C. Zhang and Z. Liu, et al., The First Observation of the Formation of Persistent Aminoxyl Radicals and Reactive Nitrogen Species on Photoirradiated Nitrogen-Containing Microplastics, Environ. Sci. Technol., 2022, 56(2), 779–789 CrossRef CAS PubMed
. - N. F. A. Biber, A. Foggo and R. C. Thompson, Characterising the deterioration of different plastics in air and seawater, Mar. Pollut. Bull., 2019, 141, 595–602 CrossRef CAS PubMed
. - R. Mao, M. Lang, X. Yu, R. Wu, X. Yang and X. Guo, Aging mechanism of microplastics with UV irradiation and its effects on the adsorption of heavy metals, J. Hazard. Mater., 2020, 393, 122515, DOI:10.1016/j.jhazmat.2020.122515
. - I. Acosta-Coley, D. Mendez-Cuadro, E. Rodriguez-Cavallo, J. de la Rosa and J. Olivero-Verbel, Trace elements in microplastics in Cartagena: A hotspot for plastic pollution at the Caribbean, Mar. Pollut. Bull., 2019, 139, 402–411, DOI:10.1016/j.marpolbul.2018.12.016
. - L. Cai, J. Wang, J. Peng, Z. Wu and X. Tan, Observation of the degradation of three types of plastic pellets exposed to UV irradiation in three different environments, Sci. Total Environ., 2018, 628–629, 740–747, DOI:10.1016/j.scitotenv.2018.02.079
. - S. Wieland, A. Balmes, J. Bender, J. Kitzinger, F. Meyer and A. F. Ramsperger, et al., From properties to toxicity: Comparing microplastics to other airborne microparticles, J. Hazard. Mater., 2022, 428, 128151, DOI:10.1016/j.jhazmat.2021.128151
. - A. Turner and L. A. Holmes, Adsorption of trace metals by microplastic pellets in fresh water, Environ. Chem., 2015, 12(5), 600–610 CrossRef CAS
. - H. Wijesekara, N. S. Bolan, L. Bradney, N. Obadamudalige, B. Seshadri and A. Kunhikrishnan, et al., Trace element dynamics of biosolids-derived microbeads, Chemosphere, 2018, 199, 331–339 CrossRef CAS PubMed
. - K. Zhu, H. Jia, Y. Sun, Y. Dai, C. Zhang and X. Guo, et al., Enhanced cytotoxicity of photoaged phenol-formaldehyde resins microplastics: Combined effects of environmentally persistent free radicals, reactive oxygen species, and conjugated carbonyls, Environ. Int., 2020, 145, 106137, DOI:10.1016/j.envint.2020.106137
. - J. Zhu, L. Kovacs, W. Han, G. Liu, Y. Huo and R. Lucas, et al., Reactive Oxygen Species-Dependent Calpain Activation Contributes to Airway and Pulmonary Vascular Remodeling in Chronic Obstructive Pulmonary Disease, Antioxid. Redox Signaling, 2019, 31(12), 804–818 CrossRef CAS PubMed
. - A. F. R. M. Ramsperger, V. K. B. Narayana, W. Gross, J. Mohanraj, M. Thelakkat and A. Greiner, et al., Environmental exposure enhances the internalization of microplastic particles into cells, Sci. Adv., 2020, 6(50), 1–10 Search PubMed
. - D. Lim, J. Jeong, K. S. Song, J. H. Sung, S. M. Oh and J. Choi, Inhalation toxicity of polystyrene micro(nano)plastics using modified OECD TG 412, Chemosphere, 2021, 262, 128330, DOI:10.1016/j.chemosphere.2020.128330
. - X. Li, T. Zhang, W. Lv, H. Wang, H. Chen and Q. Xu, et al., Intratracheal administration of polystyrene microplastics induces pulmonary fibrosis by activating oxidative stress and Wnt/β-catenin signaling pathway in mice, Ecotoxicol. Environ. Saf., 2022, 232, 113238, DOI:10.1016/j.ecoenv.2022.113238
. - Z. Fan, T. Xiao, H. Luo, D. Chen, K. Lu and W. Shi, et al., A study on the roles of long non-coding RNA and circular RNA in the pulmonary injuries induced by polystyrene microplastics, Environ. Int., 2022, 163, 107223, DOI:10.1016/j.envint.2022.107223
. - K. Lu, K. P. Lai, T. Stoeger, S. Ji, Z. Lin and X. Lin, et al., Detrimental effects of microplastic exposure on normal and asthmatic pulmonary physiology, J. Hazard. Mater., 2021, 416, 126069, DOI:10.1016/j.jhazmat.2021.126069
. - R. An, X. Wang, L. Yang, J. Zhang, N. Wang and F. Xu, et al., Polystyrene microplastics cause granulosa cells apoptosis and fibrosis in ovary through oxidative stress in rats, Toxicology, 2021, 449 Search PubMed
. - Y. Xue, Polystyrene microplastic particles : In vivo and in vitro ocular surface toxicity assessment, Environ. Pollut., 2022, 303, 119126, DOI:10.1016/j.envpol.2022.119126
. - B. W. Lewis, M. L. Ford, L. K. Rogers and R. D. Britt, Oxidative Stress Promotes Corticosteroid Insensitivity in Asthma and COPD, Antioxidants, 2021, 10(9), 1335 CrossRef CAS PubMed
. - Y. Deng, Y. Zhang, B. Lemos and H. Ren, Tissue accumulation of microplastics in mice and biomarker responses suggest widespread health risks of exposure, Sci. Rep., 2017, 7, 1–10 CrossRef PubMed
. - L. Lei, S. Wu, S. Lu, M. Liu, Y. Song and Z. Fu, et al., Microplastic particles cause intestinal damage and other adverse effects in zebrafish Danio rerio and nematode Caenorhabditis elegans, Sci. Total Environ., 2018, 619–620, 1–8, DOI:10.1016/j.scitotenv.2017.11.103
. - L. G. A. Barboza, L. R. Vieira, V. Branco, N. Figueiredo, F. Carvalho and C. Carvalho, et al., Microplastics cause neurotoxicity, oxidative damage and energy-related changes and interact with the bioaccumulation of mercury in the European seabass, Dicentrarchus labrax (Linnaeus, 1758), Aquat. Toxicol., 2018, 195, 49–57, DOI:10.1016/j.aquatox.2017.12.008
. - C. Détrée and C. Gallardo-Escárate, Single and repetitive microplastics exposures induce immune system modulation and homeostasis alteration in the edible mussel Mytilus galloprovincialis, Fish Shellfish Immunol., 2018, 83, 52–60, DOI:10.1016/j.fsi.2018.09.018
. - H. A. Leslie, M. J. M. van Velzen, S. H. Brandsma, A. D. Vethaak, J. J. Garcia-Vallejo and M. H. Lamoree, Discovery and quantification of plastic particle pollution in human blood, Environ. Int., 2022, 163, 107199, DOI:10.1016/j.envint.2022.107199
. - P. Schwabl, S. Koppel, P. Konigshofer, T. Bucsics, M. Trauner and T. Reiberger, et al., Detection of various microplastics in human stool: A prospective case series, Ann. Intern. Med., 2019, 171(7), 453–457 CrossRef PubMed
. - S. L. Wright and F. J. Kelly, Plastic and Human Health: A Micro Issue?, Environ. Sci. Technol., 2017, 51(12), 6634–6647 CrossRef CAS PubMed
. - A. Ragusa, A. Svelato, C. Santacroce, P. Catalano, V. Notarstefano and O. Carnevali, et al., Plasticenta: First evidence of microplastics in human placenta, Environ. Int., 2021, 146, 106274, DOI:10.1016/j.envint.2020.106274
. - L. C. Jenner, J. M. Rotchell, R. T. Bennett, M. Cowen, V. Tentzeris and L. R. Sadofsky, Detection of microplastics in human lung tissue using μFTIR spectroscopy, Sci. Total Environ., 2022, 831, 154907, DOI:10.1016/j.scitotenv.2022.154907
. - C. Baeza-Martínez, S. Olmos, M. González-Pleiter, J. López-Castellanos, E. García-Pachón and M. Masiá-Canuto, et al., First evidence of microplastics isolated in European citizens’ lower airway, J. Hazard. Mater., 2022, 438, 129439 CrossRef PubMed
. - Q. Chen, J. Gao, H. Yu, H. Su, Y. Yang and Y. Cao, et al., An emerging role of microplastics in the etiology of lung ground glass nodules, Environ. Sci. Eur., 2022, 34(1), 25, DOI:10.1186/s12302-022-00605-3
. - T. Padmore, C. Stark, L. A. Turkevich and J. A. Champion, Quantitative analysis of the role of fiber length on phagocytosis and inflammatory response by alveolar macrophages, Biochim. Biophys. Acta, Gen. Subj., 2017, 1861(2), 58–67, DOI:10.1016/j.bbagen.2016.09.031
. - C. D. Dong, C. W. Chen, Y. C. Chen, H. H. Chen, J. S. Lee and C. H. Lin, Polystyrene microplastic particles: In vitro pulmonary toxicity assessment, J. Hazard. Mater., 2020, 385, 121575, DOI:10.1016/j.jhazmat.2019.121575
. - H. Zhang, S. Zhang, Z. Duan and L. Wang, Pulmonary toxicology assessment of polyethylene terephthalate nanoplastic particles in vitro, Environ. Int., 2022, 162, 107177, DOI:10.1016/j.envint.2022.107177
. - M. Xu, G. Halimu, Q. Zhang, Y. Song, X. Fu and Y. Li, et al., Internalization and toxicity: A preliminary study of effects of nanoplastic particles on human lung epithelial cell, Sci. Total Environ., 2019, 694, 133794, DOI:10.1016/j.scitotenv.2019.133794
. - R. Bengalli, A. Zerboni, P. Bonfanti, M. Saibene, D. Mehn and C. Cella, et al., Characterization of microparticles derived from waste plastics and their bio-interaction with human lung A549 cells, J. Appl. Toxicol., 2022, 2030–2044 CrossRef CAS PubMed
. - Q. Shi, J. Tang, L. Wang, R. Liu and J. P. Giesy, Combined cytotoxicity of polystyrene nanoplastics and phthalate esters on human lung epithelial A549 cells and its mechanism, Ecotoxicol. Environ. Saf., 2021, 213, 112041, DOI:10.1016/j.ecoenv.2021.112041
. - S. Yang, Y. Cheng, Z. Chen, T. Liu, L. Yin and Y. Pu, et al., In vitro evaluation of nanoplastics using human lung epithelial cells, microarray analysis and co-culture model, Ecotoxicol. Environ. Saf., 2021, 226, 112837, DOI:10.1016/j.ecoenv.2021.112837
. - G. Halimu, Q. Zhang, L. Liu, Z. Zhang, X. Wang and W. Gu, et al., Toxic effects of nanoplastics with different sizes and surface charges on epithelial-to-mesenchymal transition in A549 cells and the potential toxicological mechanism, J. Hazard. Mater., 2022, 430, 128485 CrossRef CAS PubMed
. - Y. Zhang, S. Wang, V. Olga, Y. Xue, S. Lv and X. Diao, et al., The potential effects of microplastic pollution on human digestive tract cells, Chemosphere, 2022, 291(P1), 132714, DOI:10.1016/j.chemosphere.2021.132714
. - Q. Wang, J. Bai, B. Ning, L. Fan, T. Sun and Y. Fang, et al., Effects of bisphenol A and nanoscale and microscale polystyrene plastic exposure on particle uptake and toxicity in human Caco-2 cells, Chemosphere, 2020, 254, 126788, DOI:10.1016/j.chemosphere.2020.126788
. - T. Hua, S. Kiran, Y. Li and Q. X. A. Sang, Microplastics exposure affects neural development of human pluripotent stem cell-derived cortical spheroids, J. Hazard. Mater., 2022, 435, 128884, DOI:10.1016/j.jhazmat.2022.128884
. - D. Magrì, M. Veronesi, P. Sánchez-Moreno, V. Tolardo, T. Bandiera and P. P. Pompa, et al., PET nanoplastics interactions with water contaminants and their impact on human cells, Environ. Pollut., 2021, 271 Search PubMed
.
|
This journal is © The Royal Society of Chemistry 2023 |
Click here to see how this site uses Cookies. View our privacy policy here.