DOI:
10.1039/D3QM01020A
(Review Article)
Mater. Chem. Front., 2024,
8, 1195-1211
Research advances and applications of ZIF-90 metal–organic framework nanoparticles in the biomedical field
Received
18th September 2023
, Accepted 23rd October 2023
First published on 27th October 2023
Abstract
In recent years, metal–organic frameworks (MOFs) have been widely used in the field of pharmaceuticals, and their stable structure and chemical properties have shown great potential in applications requiring drug delivery carriers. ZIF-90, as a new type of MOF, has gradually attracted more attention in the field of biological medicine. ZIF-90 has the advantages of a large specific surface area, a uniform pore structure, and good biocompatibility. Importantly, the specific pH/ATP sensitivity and mitochondrial targeting provide an opportunity for it to become an intelligent drug delivery system. But unfortunately, there are few specialized reviews with a focus on ZIF-90. This article not only summarizes the structure, characterization, synthesis and biosecurity of ZIF-90, but also discusses the research progress using it as a therapeutic platform in anticancer therapy, biomedical imaging and other therapies for different diseases, over the past five years. In addition, the remaining challenges and future opportunities have also been evaluated. It is hoped that this review can provide a foundation for broadening the application of ZIF-90 in the clinical treatment, prevention and diagnosis of diseases.
1. Introduction
A nanoscale drug carrier is a drug carrier delivery system whose particle size is generally 1–1000 nm.1 Compared with traditional drug delivery systems, nanoscale drug delivery systems have the advantages of sustained release, controlled release, targeting, reducing drug toxicity and side effects, improving drug dissolution, promoting cell absorption, reducing drug elimination by the body, and improving bioavailability,2 all of which are now widely studied and applied. Some inorganic nanocarriers such as silicon dioxide and gold nanoparticles even have a high drug encapsulation efficiency.3 However, their low biosafety and the difficulty in their degradation in vivo limits their use in the pharmaceutical field.4–7 However, organic nanocarriers can improve these problems to a certain extent, among which metal–organic frameworks (MOFs) are now being considered.8–10
MOFs have emerged as a class of porous crystalline materials centered on metal ions, with the advantages of adjustable porosity, large specific surface area, good drug loading, strong surface modification function,11 and site-specific drug delivery, which make up for the shortcomings of other types of nanocarriers.12,13 Among them, zeolitic imidazolate frameworks (ZIFs) are a subclass of MOFs with a M–IM–M bond angle like the zeolite Si–O–Si bond angle, which is synthesized by the reaction of Zn(II) or Co(II) with an imidazole ligand.14 To date, ZIF-8 is the most remarkable member of this family,15 however, ZIF-8 still has disadvantages with regard to safety and it is difficult to modify, comparatively speaking. ZIF-90 has shown excellent performance in the biomedical field due to its predominant biocompatibility. During recent years, ZIF-90 has been widely used in the fields of catalysis,16–18 separation,19–21 adsorption,22,23 storage24,25 and drug delivery.26 In biomedical applications, ZIF-90 can achieve higher cell uptake and lower side effects without reducing pharmacological action, and most importantly, we can utilize the sensitivity of ZIF-90's skeleton to design more diverse drug delivery platforms.
It is worth noting that in addition to the current application of ZIF-90 in the field of antitumour treatment, there is also new progress in research to treat other diseases. Regrettably, there are few specialized reviews with a focus on a comprehensive report of the use of functionalized ZIF-90 nanocomposites. In this paper, we summarize the research advances of ZIF-90 as a nanocarrier in anticancer therapy, biomedical imaging and other therapy for different diseases (Fig. 1) over the last five years and evaluate the opportunities and challenges that may be encountered in future clinical applications.
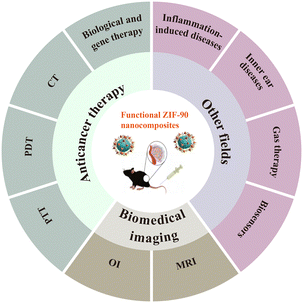 |
| Fig. 1 The schematic illustration of ZIF-90 nanocomposites applied in anticancer therapy, biomedical imaging and other therapies for different diseases. The biomedical imaging approach includes optical imaging (OI), and magnetic resonance imaging (MRI). The anticancer therapies include chemotherapy (CT), photodynamic therapy (PDT), photothermal therapy (PTT), and biological and gene therapy. Other therapies include inflammatory diseases, inner ear diseases, biosensors and gas therapy. | |
2. ZIF-90 nanoparticles
2.1. Structure
As a subclass of ZIFs, ZIF-90 is formed by combining Zn2+ and imidazole-2-carboxaldehyde(ICA) (Fig. 2a). Each N atom on the imidazole ligand is combined with a Zn atom to form a ZnN4 unit structure, which is connected by the imidazole ring to form a regular hexahedral cage structure (Fig. 2b). ZIF-90 with a superoxide dismutase (SOD) topology combines the advantages of natural zeolite and MOFs, and has a pore size of 3.5 A and a specific surface area of 1270 m2 g−1; moreover, the pore size can also be regulated.27 ICA is different from other ZIFs in that it has an aldehyde group and does not participate in the reaction during the synthesis of the ZIF-90 structure, which means that ZIF-90 contains many independently active aldehyde groups on the surface, and this will not affect its crystal structure when reacting with these aldehyde groups.28
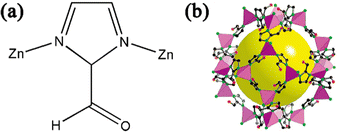 |
| Fig. 2 (a) ZIF-90 unit structure. (b) Crystal structure of ZIF-90. Reprinted with permission from ref. 28. Copyright 2008 American Chemical Society. | |
2.2. Characterization
Adsorptivity.
ZIF-90 has a very large specific surface area to adsorb drugs or gases. Some studies have found that triethylamine (TEA) could increase the area to 1900 m2 g−1 by simple addition.29 Meanwhile, its rich pore structure means that small drug molecules can be fixed in the pore by van der Waals forces, electrostatic adsorption and other modes of action, which improves the drug loading capacity.
Stability.
The zeolite-like structure gives ZIF-90 better thermal stability, which can improve the harsh storage conditions of some drugs. Compared to ZIF-8, the strong hydrogen bonds and covalent bonds mean that ZIF-90 maintains good stability under hydrothermal conditions, indicating that ZIF-90 can better maintain its structural integrity at high temperatures.
Structural modifiability.
The aldehyde group present in ZIF-90 offers the possibility of multifunctional modification. Firstly, ZIF-90 can carry drugs on the surface through simple chemical reactions, for example, doxorubicin (DOX) can be immobilized on the surface of the carrier by a Schiff reaction to achieve multi-drug loading. Secondly, new functional groups (such as carboxyl groups, amino groups and thiol groups) can be introduced onto the organic ligands of ZIF-90 by covalent modification to increase its polarity, hydrophilicity and reactivity.30
Skeleton sensitivity.
In addition to being pH-sensitive like most other ZIFs, ZIF-90 can also collapse under high concentrations of adenosine triphosphate (ATP) because of the competition between ATP and Zn2+.31,32 It is known that ATP, which is found in the mitochondria, is the most basic energy source in organisms.33–35 Therefore, ZIF-90 can be a good carrier for mitochondrial targeting and drug delivery.
2.3. Synthesis
ZIF-90 is prepared from Zn2+ and ICA. Nowadays, the commonly used preparation methods include solvothermal methods,36 liquid phase diffusion methods,37 template methods, etc. Meanwhile, the ideal particle size of ZIF-90 can be obtained by changing the reaction conditions (e.g., temperature, reaction time, solvent ratio, etc.), which can adjust the particle size and pore size. Furthermore, reaction materials are constantly being optimized, and greener and more environmentally friendly methods are constantly being explored.38,39 Among the preparation methods, solvothermal synthesis is the preferred method, which refers to a reaction method using organic matter as solvent under high temperature and pressure conditions in a closed space. Usually, ICA and Zn2+ are placed in DMF or DMSO to react under high temperature and high pressure. Li et al.40 used methanol as a solvent and mixed it with sodium in a reactor at 85 °C for one day to obtain nanosized ZIF-90 nanoparticles, and the size of the particles could be adjusted by changing the mechanical stirring time and mechanical speed.
Since organic reagents such as DMF pollute the environment and need a long time to react, researchers have found a simpler and greener method, a liquid phase diffusion method, which uses a liquid material as a solvent and reacts under normal temperature and pressure.41 Zhang et al.42 used tert-butanol and water as solvents to prepare nanoparticles (NPs) of different particle sizes by varying the ratio of Zn2+ to ICA. When the ratio of ICA to Zn2+ was 60
:
1, the particle size was 200–300 nm. Jones et al.43 reported a general method for the preparation of ZIF-90. The group investigated the effect of using different types of tertiary amines, and temperature on the size of the ZIF-90, and concluded that ZIF-90 nanoparticles with a particle size of 30 nm could be prepared under the conditions of TEA and room temperature, which provided a complete idea for preparing ZIF-90 with different preparation requirements.
2.4. Biosecurity
High biosecurity is one of the keys to making ZIF-90 a promising candidate for biomedical applications. Imidazole is one of the components of amino acids in the human body, and Zn2+ is one of the microelements in the human body. When the drug is released into blood, its material components can be used by the human body. Toxicity in the human body is minimized, so it can be widely used in the field of biomedicine. Jiang et al.44 designed a comparison experiment between ZIF-90 and ZIF-8, and the results indicated that at a nanoparticle concentration of 140 μg mL−1, the survival rate of triple-negative breast cancer cells with ZIF-8 was only 22.1%. In contrast, the cell viability of ZIF-90 was still 89.7%. For the mammary epithelial cells, the cell viability of ZIF-8 was only about 1.5%. When the concentration of nanoparticles was 100 mg kg−1, the survival rate of mice in the ZIF-8 experimental group was 0%, whereas the survival rate of mice in the ZIF-90 group was still 100%. It can be seen, that compared with ZIF-8, ZIF-90 has higher safety and better advantages as a drug carrier. Meanwhile, the team conducted systematic in vivo toxicity experiments on ZIF-90, and found that ZIF-90 had no effect on other aspects of the blood of mice, and Zn2+ did not accumulate in the body.
There have been no specific reports on the mechanism of the biosecurity difference so far, however, our analysis is based on the following two reasons. Firstly, ICA carries aldehyde groups, but its toxicity is lower than that of imidazole-2-methyl (2-MIM), the synthetic material of ZIF-8, so the toxicity of the final synthesized materials will also show differences. Secondly, ZIF-90 is hydrophilic and more likely to form hydrogen bonds with water molecules, so has better compatibility with biological tissues. However, ZIF-8 is hydrophobic and absorption in the organism may be incomplete.
3. Applications in anticancer therapy
Compared with normal tissues, the tumour microenvironment (TME) has some unique properties, such as an acidic pH, a higher ATP concentration, intracellular hypoxia, glutathione (GSH) overexpression, etc. Based on this, a variety of therapy methods have been designed to improve the effect of the treatment of tumours.45,46 Here, ZIF-90 has proven to be a promising delivery carrier, because the triggered release of the metal skeleton in the TME improves the targeting of drugs at the tumour site. Therefore, ZIF-90 can be used as a pH/ATP-responsive drug carrier in intelligent drug delivery systems for the treatment of a wide range of diseases, which are mainly focused on the field of tumour therapy (Table 1).
Table 1 Examples of ZIF-90 for drug delivery
ZIF-90 as drug nanocarriers |
Name of functionalized ZIF-90 |
Drug |
Targeted cell lines |
Ref. |
AP-ZIF-90@DOX |
DOX |
MDA-MB-231 |
44
|
PLB@ZIF-90 |
PLB |
Gastric cancer cell lines |
48
|
RGD/PTX@ZIF-90 |
PTB |
MCF-7 |
49
|
ZIF-90@ZnO |
5-FU |
L929 |
50
|
2-ME/TK-CPT@ZIF-90 |
CPT, 2-ME |
4T1 cells |
54
|
ZIF-90@DDP |
DDP |
A2780, A2780/DDP |
55
|
5-FU@ZIF-90-DOX |
5-FU, DOX |
— |
56
|
IR780/DOX@ZIF-DH |
IR780, DOX |
HeLa cells |
63
|
ZIF-90@(Ce6-21 + Ce6-155)@HA |
Ce6-21 + Ce6-155 |
MDA-MB-231, MDA-MB-436, HEK293 |
64
|
IR@ZIF-RGD |
IR |
4T1 |
68
|
ZIF-90 |
GFP, CAS9 |
HeLa cells |
72
|
3.1. Chemotherapy (CT)
Chemotherapy (CT) generally refers to a type of therapy where chemical drugs are used to kill tumours. ZIF-90 is mainly used as a carrier to enhance the effects of some chemotherapy drugs. The mechanism action of broad-spectrum antineoplastic drugs usually involves interference with cellular nucleic acid replication or transcription, protein synthesis, and damage to the chemical structure of DNA, which have destructive effects on tumour cells. However, these drugs do not distinguish between tumour cells and normal cells, which can lead to tissue damage and serious adverse effects.47 Therefore, nanocarriers have become the preferred carriers of chemotherapeutic drugs because of their excellent targeting, fewer adverse reactions, and drug resistance, as well as their higher drug efficacy.
The pH sensitivity of ZIF-90 is often used to achieve tumour targeting. Because the pH of the internal TME is about 5.5, the skeleton can disintegrate and release the drug in this range. Li et al.48 designed a PLB@ZIF-90 carrier platform, which could inhibit the YAP1 signalling pathways to treat gastric cancer by delivering plumbagin (PLB) after entering the cells (Fig. 3a). As shown in Fig. 3b, the PLB was released from the PLB@ZIF-90 very slowly at pH 7.4 and pH 8, whereas PLB was rapidly released from PLB@ZIF-90 at pH 5.5, indicating that the nanoparticles had pH release properties and could deliver the drug specifically to the tumour. To investigate the influence of the YAP1 signalling pathway on the effect produced by the formulation, PLB@ZIF-90 was used to explore the inhibitory effect on all models with high and low YAP1 expression. The experimental results showed that the formulation group inhibited the tumour growth more significantly in the high-YAP1-expression cancer group compared to the free group (Fig. 3c). In examining the protective effect of ZIF-90 on PLB, free PLB could be detected in the plasma of mice in the treated group after 72 h, whereas free PLB could not be detected in the control group after only 12 h. This showed that encapsulation with the carrier can significantly prolong the half-life of the drug in vivo. In a mouse model of gastric cancer, the antitumour effect of the formulation group was superior to that of the free-PLB treatment group (Fig. 3d). It can be seen that ZIF-90 can effectively load PLB, which can enhance the targeted aggregation of drugs, improve the half-life of drugs in plasma, and achieve precise targeted therapy for gastric cancer cells. Furthermore, the importance of inhibiting the development of gastric cancer by suppressing the YAP1 signalling pathway was elucidated, which provided new ideas and potential therapeutic targets for the treatment of gastric cancer.
 |
| Fig. 3 (a) A schematic diagram of the preparation and curative mechanism of PLB@ZIF-90 nanoparticles. (b) PLB release from PLB@ZIF-90 at 37 °C under different pH conditions. (c) Inhibition rate of tumour growth by PLB@ZIF-90 treatment. (d) Tumour images and weight. Reprinted with permission from ref. 48. Copyright 2022 Elsevier. | |
Similarly, Ge et al.49 prepared a targeted nano-delivery system for paclitaxel (PTX) based on RGD/PTX@ZIF-90, which could release up to 84% of PTX at pH 5.5. By modifying the specific peptide RGD on the surface of the carrier, they enhanced the localization of the carrier drug to tumour cells. Xiao et al.50 also developed a pH-responsive system via a core–shell structure that combined 5-fluorouracil (5-FU) and zinc oxide, which enabled the drug to achieve pH-controlled delivery in acidic organisms and also stop premature leakage of drugs under physiological conditions. These experimental results demonstrated that ZIF-90 is an effective drug carrier that can enhance the drug loading capacity and safety, prevent premature release by modifying the surface, and enable the drugs to reach and attack the tumour cells.
In addition to pH responsiveness, pH and ATP dual responsiveness could enhance the therapeutic effect on tumours. Jiang et al.44 developed an AP-ZIF-90@DOX nanoparticle to reduce the toxicity of DOX and increase the targeting ability of nanoparticle aggregation in triple-negative breast tumours. The team prepared particles with a size of 100 nm and observed that the particles could be structurally disintegrated under the dual conditions of pH 5.0 and 0.5 mM ATP. In the control experiments, the release rate was 0% for a pH of 7.4, which indicated that the particles could be well released in the TME. After co-incubation with MDA-MB-231 cells, fluorescence of DOX could be observed in the cell plasma and nucleus, confirming the good uptake of the nanoparticles by the cells. The relative tumour volume of the AP-ZIF-90@DOX experimental group was much smaller than that of the control group at day 10 after intravenous injection of triple-negative breast cancer tumour-bearing mice. This strategy provided a new direction for improving the treatment effect of triple-negative breast cancer.
Due to the fact that many potent antitumour drugs become resistant soon after treatment, organelle-targeted therapy has been put forward in order to further improve the therapeutic effect on tumours. Mitochondria are the main source of ATP, and the mitochondrial metabolism plays a crucial role in the origin, proliferation, metastasis and drug resistance. Therefore, targeting mitochondria is used as an effective technique to induce cancer cell death and bypass the drug resistance mechanisms.51–53
Based on the above background, Pan et al.54 reported a 2-ME/TK-CPT@ZIF-90@C nanocarrier platform for mitochondrial drug release of 2-methoxyestradiol (2-ME), which could trigger the release of sulfhydryl-modified camptothecin (CPT) by increasing the ambient ROS levels in the mitochondria (Fig. 4a). In this experiment, the 100 nm nanoparticles were completely disintegrated within 2 minutes in a 2 mM ATP medium (Fig. 4b). To verify the targeting of the drug carrier, a mitochondrial tracking dye was used in the cellular experiments in vitro. After incubating the 4T1 cells with the ME/TK-CPT@ZIF-90@C, the red fluorescence of the 4T1 tumour cells was observed to increase gradually from the confocal laser scanning microscopy (CLSM) imaging results (Fig. 4c), which indicated that the accumulation of the nanoparticles in the mitochondria was gradually increased, suggesting that the nanoparticles could be targeted to the cellular mitochondria, thus increasing the killing of the tumour cells (Fig. 4d). Pharmacodynamic studies were performed on 4T1 hormonal mice injected intravenously, in which the tumours in the MTZ@C treatment group were significantly smaller than those in the other control groups (Fig. 4e). The results of this experiment indicated that the carrier-modified drug enhanced the targeting property, induced persistent apoptosis of the cancer cells after the mitochondrial aggregation, and inhibited the proliferation and growth of breast cancer cells.
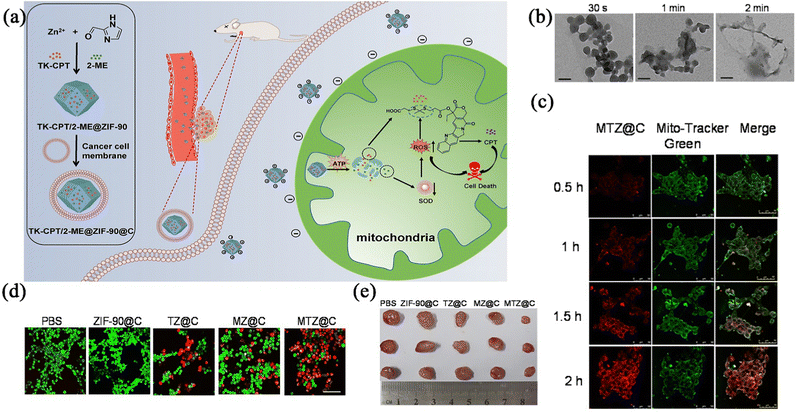 |
| Fig. 4 (a) Schematic illustration of the preparation of the ZIF-90 based platform and cancer treatment processes. (b) TEM images of MTZ at different intervals. (c) Mitochondrial co-location images of MTZ@C nanoparticles incubated with 4T1 cells. (d) Confocal images of calcium AM and PI stained 4T1 cells after different treatment. (e) Photographs of dissected tumours after the mice were treated with different materials. Reprinted with permission from ref. 54. Copyright 2021, The author(s). | |
In another study, Xing et al.55 also developed self-assembled ZIF-90@DDP nanoparticles synthesized at room temperature, which were used to improve the resistance of cisplatin to ovarian cancer by mitochondrial targeting. It was also verified that ZIF-90 could bypass the multiple resistance mechanism of tumours through the mitochondrial targeting and reverse the drug resistance of tumours. From the previous studies, it can be seen that a ZIF-90 drug-loaded nanosystem can destroy the function of organelles and affect the normal growth of tumours by mitochondrial targeting promoted by ATP sensitivity. ZIF-90 can not only enhance the killing effect of the drug, but also has a strong therapeutic effect on drug-resistant cells, which will provide new experimental solutions for the design of an organelle-targeted therapeutic platform for the induction of apoptosis.
A combination of two or more drugs is often used to combat cellular resistance, and Zhang et al.56 first reported the use of ZIF-90 loaded with two antitumour drugs, including 5-fluorouracil (5-FU) and DOX, as a combination therapeutic platform for cancer drug-targeted therapy. DOX with amino groups was loaded on the surface of the carrier by a Schiff base reaction, and the 5-FU was embedded in the carrier, with 300 nm 5-FU@ZIF-90-DOX nanoparticles being formed. The drug loadings of the 5-FU and DOX were up to 36.35 wt% and 11–13.5 wt%, respectively. In vitro experiments showed that the release amount of the two drugs could reach more than 90% at pH 5.5, which indicated that the drugs could be released faster and more efficiently into the environment of the tumour cells than in normal cells. ZIF-90 has excellent physical properties and a surface that is easy to functionalize, which can improve the efficiency of multi-drug delivery, and increase the targeting characteristics, and combination therapy with more new drug delivery platform designs provides new treatment strategies.
Overall, traditional targeted delivery systems are mainly delivered through pathological tissue to achieve cell membrane targeting. The delivery system can first reach the tumour tissue by the enhanced permeability and retention (EPR) effect and then crosses the biological barrier to gather in a specific organelle. However, only a small percentage of therapeutic agents successfully reach specific organelle targets. Direct delivery to sub-organelles is therefore key to solve the systemic toxicity. ZIF-90, as a carrier of anticancer drugs, can specifically increase the targeting of the mitochondria by pH, ATP or pH/ATP dual response modes, which provide a safety guarantee and a therapeutic pathway for improving the therapeutic effect of anticancer drugs.
3.2. Photodynamic therapy (PDT)
Photodynamic therapy (PDT) is a technique that is different from traditional cancer treatments, as it uses a photosensitizer (PS) and specific wavelengths of light to irradiate the tumour site, triggering a photochemical reaction to produce reactive oxygen species (ROS) and thus destroy tumour cells.57,58 Compared with traditional CT, PDT can not only directly kill tumour cells, but also activate the host's immune system, such as specific T cells, and enhance the killing power of such cells themselves to induce immunogenic death of tumour cells.59,60 However, among the three elements of PDT (oxygen, light source, and photosensitizer), the hypoxic environment in the tumour environment reduces the effect of the PDT; the light source, as the external condition of treatment, often makes the light reaction impossible or difficult to occur due to the insufficient penetration of the light source; and lastly, the type of photosensitizer can also lead to ineffectiveness of the PDT. Nowadays, in order to overcome the above difficulties, the application of nano-agents in PDT treatment has been widely studied. Advanced nano technology can not only solve the hypoxia problem of the tumour environment by combining oxygen supply substances,61 but can also be loaded with a photosensitizer to reduce the premature release and self-quenching of luminescent substances in the body.62
Shen et al.63 used a microfluidic nanoprecipitation method to prepare an IR780/DOX@ZIF-DH nanoparticle, where DOX was coordinated to the ZIF-90 framework, and hydrophobic photosensitizer IR780 was loaded into a spermine-modified acetylated dextran (SAD) shell, enabling the combination therapy of photodynamic treatment with CT (Fig. 5a). As shown in Fig. 5b, both DOX@ZIF and IR780/DOX@ZIF-DH exhibited an obvious structural collapse and a decrease in hydrodynamic size after 4 h in PBS at pH 5.5. To investigate if the photosensitizer encapsulated in the carrier could produce the same effect in biological organisms, the preparation was incubated in HeLa cells and then fluorescence measurements were used to determine the levels of ROS production with laser irradiation. As shown in Fig. 5c, the intensity of the strong green fluorescence of the HeLa cells after IR780/DOX@ZIF-DH combined 808 nm treatment showed that the preparation could generate ROS when the laser irradiation photochemical reaction occurred. IR780/DOX@ZIF-DH combined with CT and PDT resulted in a much greater therapeutic effect than other single-modality treatments in HeLa tumour-bearing mice treated with both intravenous injection and laser irradiation (Fig. 5d). In summary, Shen's group prepared a carrier nanoplatform for multi-drug combination therapy. The experiment provided a powerful strategy combining smart polymer design and controlled microfluidic production, which can simultaneously solve multiple problems, and can also be combined with a photodynamic force to improve the anti-tumour effect.
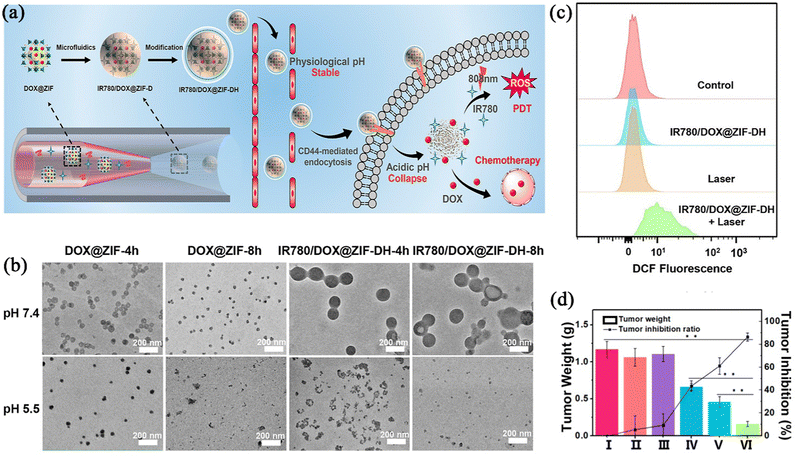 |
| Fig. 5 (a) Schematic illustration of the synthesis and structure of IR780/DOX@ZIF-DH. (b) TEM images of DOX@ZIF and IR780/DOX@ZIF-DH after immersion in PBS at pH 7.4 and 5.5 for different durations (4 and 8 h). (c) Quantitative analysis of intracellular ROS levels in HeLa cells by employing the flow cytometric technique. (d) Average weight of excised tumours and tumour inhibition ratios of mice after different treatments. Reprinted with permission from ref. 63. Copyright 2020 American Chemical Society. | |
In a study of synergistic therapy, Shang et al.64 prepared a ZIF-90@(Ce6-21 + Ce6-155)@HA nano-platform. Due to the pH responsive drug release of ZIF-90, antisense oligonucleotides (anti-miRNAs) and photosensitizers were able to be efficiently released inside the tumour microenvironment. The nano-delivery system captured overexpressed oncogenic miRNAs while the photosensitizer Ce6 generates ROS under light irradiation to effectively induce the apoptosis of tumour cells. The results confirmed this combinatorial effect, suggesting that the therapeutic approach used can effectively inhibit tumour cell proliferation and metastasis, and that the concept of combining gene therapy with PDT has great potential in cancer treatment or adjuvant therapy.
3.3. Photothermal therapy (PTT)
Photothermal therapy (PTT) refers to a treatment method that uses materials with a high photothermal conversion efficiency to convert light energy into heat energy under infrared illumination to kill cancer cells.65,66 The gathering of heat at the tumour site for continuous warming can directly denature proteins to kill tumour cells directly, and this process is now in wide use in clinical practice.67 Although the photothermal method can inhibit primary tumours, the laser effect was limited by different heat distributions and locations in clinical experiments, which makes it impossible to eradicate the cancer, and even causes thermal damage to normal tissues around the tumours.
Based on this, Yu et al.68 developed a nanocomplex (IR@ZIF-RGD) delivery system that can be used to intervene in the ATP energy metabolism, which could lead to mitochondrial oxidative stress and dysfunction and therefore disrupt the tumour growth environment via ZIF-90-loaded siRNAs. Also, by using Indocyanine Green (ICG)-triggered PTT, it could provoke potent immunogenic cell death (ICD) to enhance antitumour immunogenicity (Fig. 6a). Since mature dendritic cells (DCs) play a key role in activating the adaptive immune system to kill tumour cells, the results of in vitro immunoreactivity experiments showed that the nanocomplexes had the potential to induce the maturation of ICD-activated DCs, and the proportion of mature DCs could be increased from 14.2%, 20.1%, 34.7%, and 51.7% of the control group after treatment with the experimental group, suggesting that the PTT could induce a higher level of oxidative stress than ATP depletion-mediated oxidative stress for stronger anti-tumour immunity. In order to confirm the anti-tumour effect, after 808 nm near-infrared irradiation, it was found that the degree of tumour inhibition in the group treated with light was significantly higher than that in the group not treated with light (Fig. 6b). At the same time, the temperature of the tumour area was shown to have increased by using near-infrared thermal imaging, indicating that ICG was released and produced thermal energy inside the tumour. The highest ratio of CD4+ and CD8+ T cells in the treated and executed mice existed in the combined treatment irradiation group, and the immunosuppressive T cells and interleukin-10 (IL-10) were down-regulated in the combined treatment irradiation group (Fig. 6c), indicating that this nano-formulation delivery system could effectively remodel the tumour immune microenvironment and reverse its immunosuppressive effects. Taken together, this nanocomplex has been shown to elicit a protective anticancer immune response in vivo, thereby inhibiting tumour growth. Such a drug delivery system not only reduces the side effects on the body compared to the traditional tumour treatment, but also provides a superior therapeutic strategy to improve the clinical effect of the tumour treatment by a combination of PTT and immune therapy.
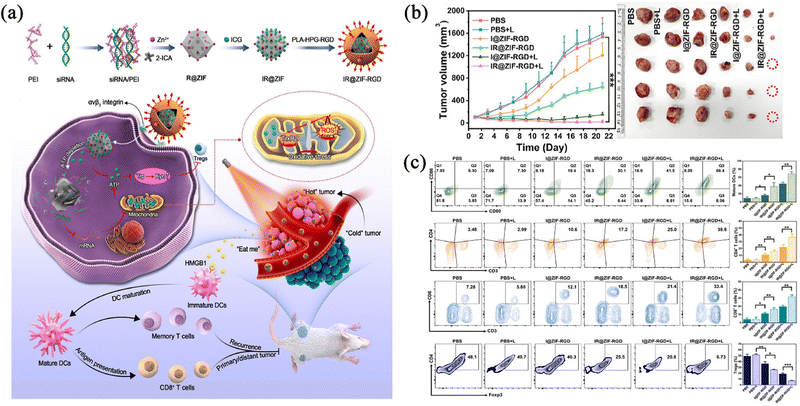 |
| Fig. 6 (a) Schematic illustration of intratumoural metabolic intervention and immune response mediated by IR@ZIF-RGD with ATP-exhausted properties. (b) Tumour growth curves of the mice bearing 4T1 tumour after different treatments. (c) Flow cytometry. Reprinted with permission from ref. 68. Copyright 2022 Elsevier. | |
3.4. Biological and gene therapy
In recent years, the biology and gene treatment of tumours has become a new trend, and this mainly includes use of proteins, peptides, enzymes, antibodies, and nucleic acid drugs, etc., which are derived from substances in living organisms using modern biotechnological methods. Compared to traditional anticancer drugs, these drugs have weaker side effects. However, due to their fragility, immunogenicity, low cell permeability and shorter half-life,69,70 they often require the addition of different excipients or external protection techniques, which makes the production cost significantly higher. Searching for a suitable and innocuous drug carrier is still an urgent problem. Using ZIF-90 as a metal–organic carrier, can protect the nucleic acid or protein structure, improve the stability and reduce storage difficulties. Research in the past five years has also turned its attention to using ZIF-90 as a carrier to improve the effect of biological and nucleic acid drugs in vivo. Composites based on ZIF-90 offer promising delivery systems for biological and gene therapies.71
Yang et al.72 used ZIF-90 as a general platform for cytosolic protein delivery and CRISPR/Cas9 genome editing. Using a one-pot method, five proteins with different molecular weights, such as GFP and BSA, could be successfully loaded and ZIF-90/protein nanoparticles were obtained, which released the drugs into the high ATP environment of the tumour (Fig. 7a). In the in vitro release experiment, it was observed that the ZIF-90/protein nanoparticles collapsed in a short time in the 2 mM ATP environment (Fig. 7b), and the protein release rate reached 75% within 8 h (Fig. 7c). Interestingly, the drug release experiments showed only 5% protein release within 15 h when using PBS with a low pH of 5 (Fig. 7d), implying that the necessary conditions for possible release of ZIF-90 when loaded with macromolecules is a high content of ATP rather than a high pH. To explore the protein uptake efficiency, as shown in Fig. 7e, the protein uptake in HeLa cells using the prepared nanoparticles was higher than that of pure protein, which meant that the cells increased the protein delivery after using ZIF-90. To study the use of ZIF-90 nanoparticles for cytosolic delivery, RNase A-NBC (a chemically modified protein that is toxic to tumour cells) delivery assays on cancer cells were carried out, and the results are shown as Fig. 7f. Unlike the free drug, the ZIF-90 formulation group was highly cytotoxic to HeLa cells at all protein concentrations, indicating the high efficiency of ZIF-90 nanoparticles in delivering cytotoxic proteins for cancer therapy. These favourable results demonstrated that ZIF-90 could protect the protein activity and improve the absorption and delivery of biological macromolecules in the organism. The protein-loaded ZIF-90 nano-platform can deliver proteins to the cytoplasm regardless of molecular weight and achieve targeted release at the tumour site under the ATP conditions, which is a new idea for the clinical treatment of tumours with biological macromolecules.
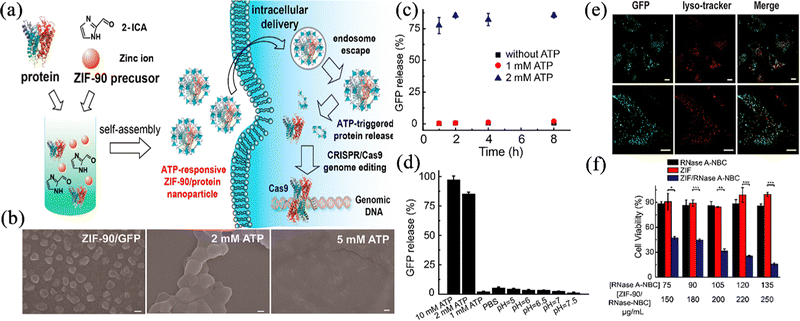 |
| Fig. 7 (a) Schematic illustration of the ATP-triggered protein release from ZIF-90 nanoparticle inside cells. (b) SEM images of ZIF-90/GFP nanoparticles without ATP and with ATP. (c) ZIF-90/GFP nanoparticles were treated with ATP for protein release kinetics study. (d) Selectivity of ATP triggered protein release from ZIF-90/GFP nanoparticles. (e) CLSM images of HeLa cells treated with ZIF-90/GFP nanoparticle. (f) RNase A-NBC delivery efficiently prohibited tumour cell growth. Reprinted with permission from ref. 72. Copyright 2019 American Chemical Society. | |
Chen et al.73 prepared PTB@ZIF-90/MB nanoparticles, loading modified photothermal bacteria (PTB) into ZIF-90 for tumour targeted PTT. Methylene blue (MB) could cause mitochondrial dysfunction by producing singlet oxygen (1O2) under light illumination (Fig. 8a). In all PTB@ZIF-90/MB-treated groups, the tumours were suppressed to a certain extent in the 660 nm light (660) or 808 nm laser (808)-treated groups compared to the untreated groups, and this could be attributed to the photodynamic or photothermal effects (Fig. 8b). To investigate the mechanism of enhanced PTT efficacy in the 660 + 808 group, the expression of two typical heat shock-resistant proteins (HSP70 and HSP90) was assessed. Compared with other groups, immunofluorescence staining results showed that to the 660 + 808 group, expression of heat shock proteins (HSPs) was significantly lower, suggesting that the enhanced effect of tumour ablation meight be due to the inhibition of heat tolerance induced by heat shock proteins (Fig. 8c). On the one hand, this strategy confirms the ability of ZIF-90 to act as an organism carrier and retain its original chemical properties. On the other hand, PTB@ZIF-90/MB as a combined therapeutic platform of PTT and biological therapy has a preferential tumour targeting ability and enhanced photothermal tumour ablation ability, and confirms that HSPs affect tumour resistance. This provides a strong experimental basis for solving the problems of PTT.
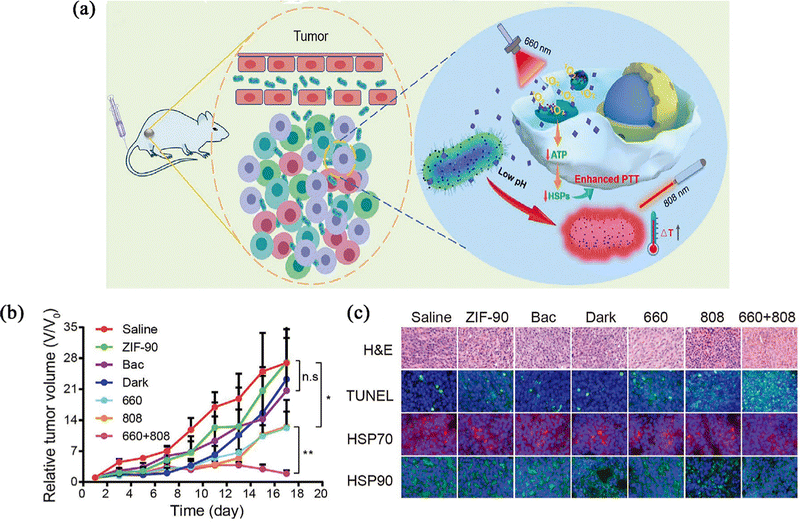 |
| Fig. 8 (a) Schematic illustration of mechanism of tumour targeting and photothermal tumour ablation of PTB@ZIF-90/MB upon laser irradiation. (b) Tumour growth profiles of different treatments within 17 days. (c) Tumour H&E staining, TUNEL assay, and immunofluorescence staining of HSP70 and HSP90 in different treatment groups. Reprinted with permission from ref. 73. Copyright 2020 Wiley. | |
In conclusion, ZIF-90 guarantees the activity of these types of drugs after drug loading, which can ensure that their activity and action are stable in normal temperatures or in the circulation environment in vivo. Moreover, it also improves the efficiency of macromolecular drugs in entering the cells of the organism, and enlarges the variety of therapeutic drugs.
4. Applications in biomedical imaging
With the continuous development of biomedicine, visualization of disease in the organism has become the new way to promote the diagnosis, treatment, and interventional imaging element in drug delivery systems, tracking the process of drug delivery in the organism to provide information about the lesions of diseases, with the techniques used including positron emission tomography (PET), optical imaging (OI), magnetic resonance imaging (MRI), and computed tomography (CT). Nanomaterials have been widely used as a new type of imaging probe in biomedical imaging research. Most MOFs have great potential as biological imaging probes.74,75 As a type of MOF, ZIF-90 can also be loaded with some fluorescent compounds, allowing them to be used to accurately locate the location of tumours.76
4.1. Optical imaging (OI)
Optical imaging (OI) has been widely used in the detection of cells, tissues and even organisms. Usually, fluorescent dyes are exposed to light of a certain excitation wavelength, causing the dyes to produce fluorescence that can be observed by the naked eye for the diagnosis of diseases. However, due to the poor stability and short emissivity of fluorescent dyes, the results are often unclear, or the dyes are unable to emit fluorescence during imaging. The use of ZIF-90 can stabilize and release fluorescent dyes in different response modes, which allows an expansion of the variety of OI.
Hou et al.77 reported ATP-responsive the use of near-infrared (NIR) fluorescent nanoprobes where the NIR fluorescent dyes are encapsulated in the pores of ZIF-90 (Fig. 9a). The probes could differentiate normal cells from tumour cells by their ATP concentration. As shown in Fig. 9b, it was observed that the fluorescence intensity is enhanced by increasing ATP concentration, and this could clearly distinguish tumour cells from normal cells (Fig. 9c). For the mice treated with the prepared nanoparticles, the fluorescence accumulated at the tumour site and showed strong fluorescence compared with the normal mice, which verified that the fluorescent nanoprobes could be successfully used for real-time fluorescence imaging of endogenous and exogenous ATP levels in living cells (Fig. 9d). Overall, ZIF-90 has a good protective effect, and the prepared fluorescent probe can be accurately released at the position of high ATP concentration, and give rise to a highly accurate imaging effect, which can be used in the clinical application of monitoring the ATP level to diagnose the occurrence of diseases.
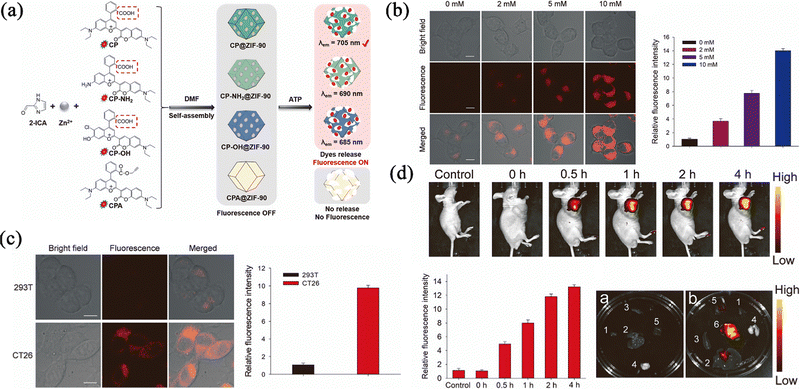 |
| Fig. 9 (a) Schematic diagram of the construction of four nanoprobes and their response to ATP. (b) Fluorescence imaging of exogenous ATP in 293 T cells. (c) Fluorescence imaging of endogenous ATP in 293 T cells and CT26 cells. (d) Fluorescence imaging of endogenous ATP in mice, main organs and tumour. Reprinted with permission from ref. 77. Copyright 2022 Elsevier. | |
Due to the rapid growth of tumours, the resulting hypoxic environment also becomes a responsive condition. Nitroreductase (NTR) is a flavin-containing enzyme that is often overexpressed in hypoxic areas of solid tumors. It can be combined with the enzyme probe to produce fluorescence, and so it could be used for fluorescence imaging to detect hypoxic area of solid tumors. Thus, Zou et al.78 fabricated ZIF-90 as a carrier which was loaded with enzyme-sensitive organic small molecule probes and this was used as a new fluorescence imaging system to detect the unique hypoxic environment of tumours by fluorescence. In the extracellular fluorescence test, the fluorescence imaging of A549 cells showed that the nanoprobe could detect fluorescent signals in an environment with a high expression of NTR under hypoxia. After injecting the small molecule nanocarrier probe into the tail vein of a tumour-bearing mouse, the fluorescence intensity of the tumour site of the mice was 5.8 times higher than that of the mice injected with PBS. The highly expressed NTR in the tumour environment was different from ordinary cells and this could be captured by the fluorescent probe. In summary, Zou's group successfully applied ZIF-90 to fluorescence imaging in response to hypoxia without affecting the enzyme recognition of the probe, opening up a promising future for enzyme reactive probes.
In summary, in terms of overcoming the shortage of fluorescent dyes, ZIF-90 can reduce the self-quenching phenomenon of dyes during circulation in the body, which expands the use of fluorescent dyes. Furthermore, ZIF-90 can carry small molecules to bind to enzymes in the body environment, which can improve the sensitivity of OI by more sensitive signal sensing.
4.2. Magnetic resonance imaging (MRI)
Magnetic resonance imaging (MRI) is one of the most commonly used molecular imaging techniques in clinical methods. It is a type of imaging based on nuclear spin orientation in a magnetic field, which can detect changes in body tissue and provide accurate information about cell interaction dynamics. Usually, a contrast agent (CA) is used to distinguish between normal and abnormal tissues of the body.79 With high spatial resolution, contrast and infinite advantages of penetration, but low sensitivity, MRI cannot distinguish between abnormal tissue and normal tissue, and so it is important to make a more sensitive carrier application.
Jiang et al.80 reported a magnetic resonance imaging contrast agent designed with active targeting and a pH-responsive drug delivery system APT (a ligand)-Mn-ZIF-90 bound to a novel neuropeptide Y1 receptor (Y1R) to achieve in vivo drug delivery monitoring of the intended site (Fig. 10a). In vitro cell targeting experiments confirmed that the mean fluorescence intensity (MFI) of APT-Mn-ZIF-90 was about 1.5 times greater than that of Mn-ZIF-90, and the relative endocytosis rate of cells increased with increasing nanoparticle concentration (Fig. 10b). At 24 h after intravenous injection of APT-Mn-ZIF-90 and Mn-ZIF-90 in tumour-bearing mice, the average gray value of APT-Mn-ZIF-90 was higher than that of Mn-ZIF-90, indicating that more NPs had accumulated in the tumour after APT modification. Meanwhile, a T1-weighted MR signal could be observed at the tumour, but this signal was not seen with the PBS-treated group (Fig. 10c). On the one hand, DNA-damaging drugs loaded in this nanosystem can be released into mitochondria by post-synthetic modification of ZIF-90 with Mn2+, resulting in better therapeutic effects. On the other hand, the nanosystem itself can generate high-resolution T1-weighted MR signals at the tumour, which can be used for in vivo drug location tracking. This strategy can improve the accumulation of drug-loaded particles to destroy tumour cells, and improve their use for more accurate and personalized imaging guided treatment.
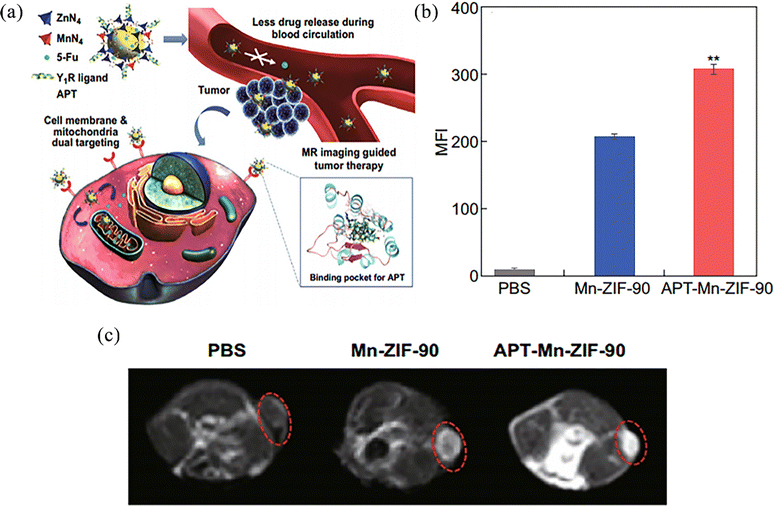 |
| Fig. 10 (a) Schematic of bio-application and potential mechanism of APT-Mn-ZIF-90/5-FU for tumour therapy. (b) Mean fluorescence intensity of MCF-7 cells incubated with NPs for 8 h. (c) T1-weighted MR images of MCF-7 tumour-bearing nude mice after intravenous injection. Reprinted with permission from ref. 80. Copyright 2019, The Author(s). | |
5. Applications in other fields
5.1. Inflammation-induced diseases
Besides the targeted treatment of cancer, ZIF-90 can also be used as a carrier to package some drugs with a short half-life, in order to increase the therapeutic effect of the drugs in the body and reduce the toxicity to normal cells. Lin et al.81 established a pH-responsive drug delivery system, MI@ZIF-90, which was used to treat paediatric sepsis by encapsulating antibacterial metronidazole (MI) in hydrophobic ZIF-90. This enhanced the targeted release of drugs in an acidic environment and increased the drug loading capacity (23.65%), effectively controlled microbial infection and enhanced immunity. In the static biofilm model test, the inhibitory effect of the drug-loaded particles on the bacterial biofilm was shown by fluorescent images. In vitro, MI@ZIF-90 showed the inhibitory effect on the growth of Escherichia coli and Staphylococcus aureus strains, which may be due to the antibacterial ability of zinc and metronidazole to produce ROS-induced bacterial death. The nanodrug delivery system prepared by Lin's group had a high drug loading and synergistic antibacterial effect on metronidazole, indicating that ZIF-90 is effective for antimicrobial therapy.
5.2. Inner ear diseases
In another study, Xu et al.82 prepared MP@ZIF-90 nanoparticles for the treatment of noise-induced hearing loss (NIHL) in order to reduce the side effects of the steroid hormone methylprednisolone (MP). The nanoparticles with a fluorescent effect (FITC@ZIF) were injected intraperitoneally into the mouse cochlea, and strong fluorescent signals were shown in the image of the mouse cochlea, which confirmed that the nanosystem could be targeted into the inner ear. In the study of mice treated via the inner ear, using the auditory brainstem response (ABR) test using free MP, MP@ZIF-90 and MP@ZIF-8 contrast experiments, the results showed that the mice in the MP@ZIF-90 group still showed significant protection after high-frequency noise treatment. This study provides a basis for research on using ZIF-90 as a carrier for steroid hormones, and provide insights into novel delivery systems for inner ear therapy. Most importantly, it also contributes a strong experimental basis for improving the effect of other hormone drugs.
5.3. Biosensors
In fact, disease diagnosis is not limited to detection in vivo. ZIF-90 has great potential for use as a biosensor to detect glucose, urea, protein and other biomolecules in the body in vitro, which could be used for disease diagnosis with external detection. Li et al.83 used ZIF-90 combined with PVP to prepare a photoacoustic sensor with a high photoacoustic signal and stability. The sensor could realize the simultaneous detection of glucose and urea with good anti-interference ability and reproducibility, and detect glucose and urea in human blood samples.
Wu et al.84 reported a horse radish peroxidase (HRP)–ZIF-90 nanocomposite for the detection of secreted protein acidic and rich in cysteine (SPARC). HRP molecules can be quickly released from nanocomposites under acidic conditions to catalyze a chromogenic reaction, enabling the ultrasensitive detection of SPARC with a low detection limit. In addition, the detection of SPARC in colon cancer tissues can also be used to further diagnose the stage of the disease (Fig. 11a). To investigate the sensitivity of the preparation to proteins, the nanoparticles were tested separately for different proteins, and the results showed the highest SPARC signal response, which indicated that the nanoplatform could be used as a nanoprobe to detect the SPARC protein level in organisms (Fig. 11b). To investigate the ability of the nanoprobe to distinguish between tumours at different stages, clinical human colon cancer samples were used and different depths of colour were detected (Fig. 11c). It was observed that the colour on the microplate gradually became darker with the decrease of the degree of differentiation. After further statistical analysis, it was found that there was a significant difference between the cancerous group and the normal group, and the differentiated group and the normal group. So ZIF-90 can be used as a nano-enzyme to act on proteins or DNA in the tumour environment, which provides a new platform for biological detection. It has the advantages of high efficiency, low cost, and easy operation, and can be of service in disease diagnosis, drug screening, biosafety monitoring and other fields, thus contributing to the development of human health and life science.
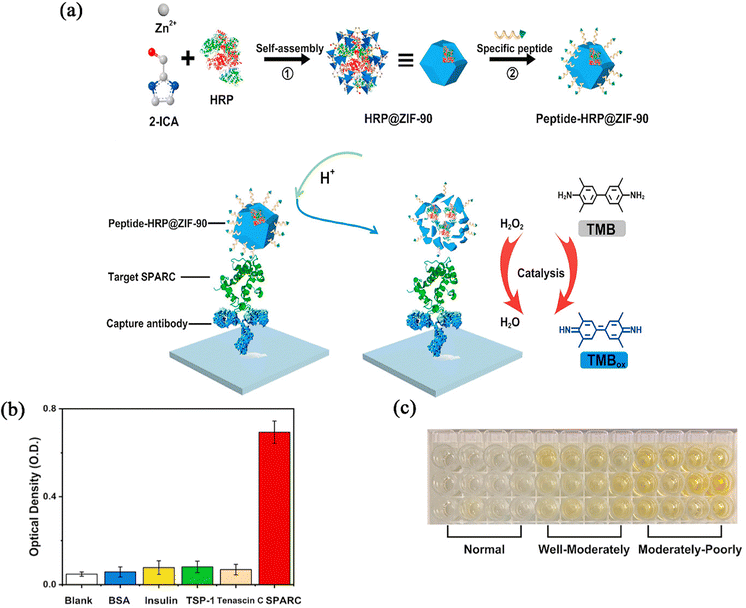 |
| Fig. 11 (a) Schematic diagram for the fabrication process of peptide-HRP@ZIF-90 and colorimetric detection of target SPARC. (b) The selectivity of peptide-HRP@ZIF-90. (c) The photograph of colorimetric responses toward three groups of tissue samples using peptide-HRP@ZIF-90. Reprinted with permission from ref. 84. Copyright 2020 Elsevier. | |
5.4. Gas therapy
The superior pore structure of ZIF-90 can also adsorb gas, showing great potential in gas therapy. Xie et al.85 prepared a novel multifunctional nanodrug carrier UC@mSiO2-RB@ZIF-O2-DOX-PEGFA that combined oxygen (O2)-enhanced PDT with pH-responsive CT. Among them, a mesoporous silica shell (mSiO2) was used as a photosensitive carrier, and ZIF-90 was coated in silica as an O2 reservoir to rapidly release O2 alleviating tumour hypoxia, which enhances the therapeutic effect of PDT. This experiment showed that ZIF-90 could adsorb gas and release it in the TME, which gives us some insight that some inherently therapeutic gases, such as carbon monoxide and nitric oxide,86 can also be directly adsorbed onto ZIF-90, and this strategy will provide a new development direction for ZIF-90 gas therapy.
From the above research progress, it can be seen that although ZIF-90 is less widely used in areas without tumours, it still has the potential to be used as a carrier for some hormone drugs or antibacterial drugs to reduce the dose and toxicity in therapy. In the field of biosensors, it provides another convenient and sensitive method for the detection of cell or tissue components. In addition, ZIF-90 has a powerful gas adsorption capacity, which may provide a new therapeutic approach for cancer treatment.
6. Conclusions and perspectives
In summary, the preparation of ZIF-90 nanocomposites and their applications in biomedical fields have been discussed in detail. Firstly, its structural characteristics determine the advantages of drug loading, and this can be amplified by different syntheses. Secondly, the superior biocompatibility and passive targeting of ZIF-90 increase the flexibility of the response to the tumour environment and so decrease the side-effect of the drug; meanwhile, its unique mitochondrial targeting can significantly improve the therapeutic effect. Thirdly, the aldehyde group on the surface of ZIF-90 can be subjected to various reactions, which increases the modification ability of the carrier and can be processed according to the drug requirements to change the performance. In conclusion, compared with other MOFs, ZIF-90 has better biocompatibility and can deliver drugs to tissues and organs in a targeted and controlled manner, showing great potential for clinical use.
However, there are still many challenges to be solved before the clinical application of ZIF-90 nanocomposites, and these mainly include the following aspects:
(a) ZIF-90 synthesis methods mainly include solvothermal and hydrothermal methods, but these methods have certain disadvantages, such as long reaction times, high temperature, low yield, etc. Therefore, it is necessary to explore more efficient, environmentally friendly and economical synthesis methods, such as microwave-assisted methods, and ultrasound-assisted methods.
(b) The structure of ZIF-90 is regulated by factors such as the ratio of metal ions and organic ligands. Different structures and modification methods have different effects on its biological safety and therapeutic methods, so the diversification of its structure and properties still needs to be explored.
(c) At present, most of the pharmacodynamic experiments on ZIF-90 are at the cellular level research stage in vitro. Therefore, the pharmacokinetics and pharmacodynamic behaviour in vivo need to be further explored, which is of great significance for guiding drug therapy, evaluating drug efficacy, adjusting treatment regimens and exploring drug safety.
As a member of the ZIF family with great potential, ZIF-90 also shows better application prospects in other new medical fields. ZIF-90 can be coated with a transition metal oxide for tumour treatment by chemodynamic therapy. It can also be used as a carrier of cells for enhancing cell adhesion and proliferation, regulating cell differentiation and function, in order to repair tissue and promote wound healing. In the future, we believe that more innovative and multifunctional ZIF-90-based nanocarriers will continue to be further developed and applied.
Conflicts of interest
There are no conflicts to declare.
Acknowledgements
This work was supported by Science Research Project of The Educational Department of Liaoning Province (JYTMS20231358).
Notes and references
- R. N. Saha, S. Vasanthakumar, G. Bende and M. Snehalatha, Nanoparticulate drug delivery systems for cancer chemotherapy, Mol. Membr. Biol., 2010, 27, 215–231 CrossRef CAS PubMed.
- S. Dufort, L. Sancey and J. L. Coll, Physico-chemical parameters that govern nanoparticles fate also dictate rules for their molecular evolution, Adv. Drug Delivery Rev., 2012, 64, 179–189 CrossRef CAS PubMed.
- M. Twana, W. Keng, M. L. Wing and V. K. Vitaliy, Silica nanoparticles in transmucosal drug delivery, Pharmaceutics, 2020, 12, 751 CrossRef PubMed.
- P. P. Yang, S. L. Gai and J. Lin, Functionalized mesoporous silica materials for controlled drug delivery, Chem. Soc. Rev., 2012, 41, 36793698 Search PubMed.
- A. A. Nayl, A. I. Abd-Elhamid, A. A. Aly and S. Bräse, Recent progress in the applications of silica-based nanoparticles, RSC Adv., 2022, 12, 13706–13726 RSC.
- N. Singh, S. Shi and S. Goel, Ultrasmall silica nanoparticles in translational biomedical research: Overview and outlook, Adv. Drug Delivery Rev., 2023, 192, 114638 CrossRef CAS PubMed.
- M. Tannous, F. Caldera, G. Hoti, U. Dianzani, R. Cavalli and F. Trotta, Drug-Encapsulated Cyclodextrin Nanosponges, Methods Mol. Biol., 2021, 2207, 247–283 CrossRef CAS PubMed.
- A. Jash, A. Ubeyitogullari and S. S. H. Rizvi, Liposomes for oral delivery of protein and peptide-based therapeutics: challenges, formulation strategies, and advances, J. Mater. Chem. B, 2021, 9, 4773–4792 RSC.
- A. E. Brooks, B. D. Brooks, S. N. Davidoff, P. C. Hogrebe, M. A. Fisher and D. W. Grainger, Polymer-controlled release of tobramycin from bone graft void filler, Drug Delivery Transl. Res., 2013, 3, 518–530 CrossRef CAS PubMed.
- H. Namazi and M. Adeli, Dendrimers of citric acid and poly (ethylene glycol) as the new drug-delivery agents, Biomaterials, 2005, 26, 1175–1183 CrossRef CAS PubMed.
- A. Bieniek, A. P. Terzyk, M. Wiśniewski, K. Roszek, P. Kowalczyk, L. Sarkisov, S. Keskin and K. Kaneko, MOF materials as therapeutic agents, drug carriers, imaging agents and biosensors in cancer biomedicine: Recent advances and perspectives, Prog. Mater. Sci., 2021, 117, 100743 CrossRef CAS.
- B. Maranescu and A. Visa, Applications of Metal-Organic Frameworks as Drug Delivery Systems, Int. J. Mol. Sci., 2022, 23, 4458 CrossRef CAS PubMed.
- M. Ding, W. Liu and R. Gref, Nanoscale MOFs: From synthesis to drug delivery and theranostics applications, Adv. Drug Delivery Rev., 2022, 190, 114496 CrossRef CAS PubMed.
- A. Phan, C. J. Doonan, F. J. Uribe-Romo, C. B. Knobler, M. O’Keeffe and O. M. Yaghi, Synthesis, structure, and carbon dioxide capture properties of zeolitic imidazolate frameworks, Acc. Chem. Res., 2009, 42, 58–67 Search PubMed.
- H. N. Abdelhamid, Zeolitic Imidazolate Frameworks (ZIF-8) for Biomedical Applications: A Review, Curr. Med. Chem., 2021, 28, 7023–7075 CAS.
- T. Jose, Y. Hwang, D. W. Kim, M. I. Kim and D. W. Park, Functionalized zeolitic imidazolate framework F-ZIF-90 as efficient catalyst for the cycloaddition of carbon dioxide to allyl glycidyl ether, Catal. Today, 2015, 245, 61–67 CrossRef CAS.
- Y. Zou, X. Liu and H. Zhang, A dual enzyme-containing microreactor for consecutive digestion based on hydrophilic ZIF-90 with size-selective sheltering, Colloids Surf., B, 2021, 197, 111422 CrossRef CAS PubMed.
- X. Yang, J. Zhao, A. Cavaco-Paulo, J. Su and H. Wang, Encapsulated laccase in bimetallic Cu/Zn ZIFs as stable and reusable biocatalyst for decolorization of dye wastewater, Int. J. Biol. Macromol., 2023, 233, 123410 CrossRef CAS PubMed.
- D. Hua, Y. K. Ong, Y. Wang, T. X. Yang and T. S. Chung, ZIF-90/P84 mixed matrix membranes for pervaporation dehydration of isopropanol, J. Membr.
Sci., 2014, 453, 155–167 CrossRef CAS.
- A. Huang, W. Dou and J. Caro, Steam-stable zeolitic imidazolate framework ZIF-90 membrane with hydrogen selectivity through covalent functionalization, J. Am. Chem. Soc., 2010, 132, 15562–15564 CrossRef CAS PubMed.
- A. Huang, N. Wang, C. Kong and J. Caro, Organosilica-functionalized zeolitic imidazolate framework ZIF-90 membrane with high gas-separation performance, Angew. Chem., Int. Ed., 2012, 51, 10551–10555 CrossRef PubMed.
- J. A. Gee, J. Chung, S. Nair and D. S. Sholl, Adsorption and Diffusion of Small Alcohols in Zeolitic Imidazolate Frameworks ZIF-8 and ZIF-90, J. Phys. Chem. C, 2013, 117, 3169–3176 CrossRef CAS.
- E. I. Nosike, Z. Jiang, L. Miao, O. U. Akakuru, B. Yuan, S. Wu, Y. Zhang, Y. Zhang and A. Wu, A novel hybrid nanoadsorbent for effective Hg2+ adsorption based on zeolitic imidazolate framework (ZIF-90) assembled onto poly acrylic acid capped Fe3O4 nanoparticles and cysteine, J. Hazard. Mater., 2020, 392, 122288 CrossRef CAS PubMed.
- N. Y. Tan, M. T. Ruggiero, C. Orellana-Tavra, T. Tian, A. D. Bond, T. M. Korter, D. Fairen-Jimenez and J. A. Zeitler, Investigation of the terahertz vibrational modes of ZIF-8 and ZIF-90 with terahertz time-domain spectroscopy, Chem. Commun., 2015, 51, 16037–16040 RSC.
- C. Duan, H. Zhang, F. Li, J. Xiao, S. Luo and H. Xi, Hierarchically porous metal-organic frameworks: rapid synthesis and enhanced gas storage, Soft Matter, 2018, 14, 9589–9598 RSC.
- J. Hao, I. S. Milašin, Z. B. Eken, M. Mravak-Stipetic, K. Pavelić and F. Ozer, Effects of Zeolite as a Drug Delivery System on Cancer Therapy: A Systematic Review, Molecules, 2021, 26, 6196 CrossRef CAS PubMed.
- C. Byrne, A. Ristić, S. Mal, M. Opresnik and N. L. Zabukovec, Evaluation of ZIF-8 and ZIF-90 as Heat Storage Materials by Using Water, Methanol and Ethanol as Working Fluids, Crystals, 2021, 11, 1422 CrossRef CAS.
- W. Morris, C. J. Doonan, H. Furukawa, R. Banerjee and O. M. Yaghi, Crystals as molecules: postsynthesis covalent functionalization of zeolitic imidazolate frameworks, J. Am. Chem. Soc., 2008, 130, 12626–12627 CrossRef CAS PubMed.
- P. F. Liguori, B. Russo, A. Melicchio and G. Golemme, Synthesis and gas sorption behavior of ZIF-90 with large pore volume, New J. Chem., 2017, 41, 13235–13239 RSC.
- C. I. Yen, S. M. Liu, W. S. Lo, J. W. Wu, Y. H. Liu, R. J. Chein, R. Yang, K. C. Wu, J. R. Hwu, N. Ma and F. K. Shieh, Cytotoxicity of Postmodified Zeolitic Imidazolate Framework-90 (ZIF-90) Nanocrystals: Correlation between Functionality and Toxicity, Chemistry, 2016, 22, 2925–2929 CrossRef CAS PubMed.
- J. Deng, K. Wang, M. Wang, P. Yua and L. Mao, Mitochondria Targeted Nanoscale Zeolitic Imidazole Framework-90 for ATP Imaging in Live Cells, J. Am. Chem. Soc., 2017, 139, 5877–5882 CrossRef CAS PubMed.
- Y. Wang, D. Zhang, Y. Zeng, Y. Sun and P. Qi, Simultaneous ultrasensitive ADP and ATP quantification based on CRISPR/Cas12a integrated ZIF-90@Ag3AuS2@Fe3O4 nanocomposites, Biosens. Bioelectron., 2022, 218, 114784 CrossRef CAS PubMed.
- A. Patel, L. Malinovska and S. Saha, ATP as a biological hydrotrope, Science, 2017, 356, 753–756 CrossRef CAS PubMed.
- M. I. Sweeney, Neuroprotective effects of adenosine in cerebral ischemia: window of opportunity, Neurosci. Biobehav. Rev., 1997, 21, 207–217 CrossRef CAS PubMed.
- P. E. Porporato, N. Filigheddu, J. M. B. Pedro, G. Kroemer and L. Galluzzi, Mitochondrial metabolism and cancer, Cell Res., 2018, 28, 265–280 CrossRef CAS PubMed.
- T. Taghizadeh, A. Ameri, A. Talebian-Kiakalaieh, S. Mojtabavi, A. Ameri, H. Forootanfar, S. Tarighi and M. A. Faramarz, Lipase@zeolitic imidazolate framework ZIF-90: A highly stable and recyclable biocatalyst for the synthesis of fruity banana flavour, Int. J. Biol. Macromol., 2021, 166, 1301–1311 CrossRef CAS PubMed.
- W. S. Lo, S. M. Liu, S. Wang, H. P. Lin, N. H. Ma, H. Y. Huang and F. K. Shieh, A green and facile approach to obtain 100nm zeolitic imidazolate framework-90 (ZIF-90) particles via leveraging viscosity effects, RSC Adv., 2014, 4, 52883–52886 RSC.
- A. Škrjanc, C. Byrne and N. Z. Logar, Green Solvents as an Alternative to DMF in ZIF-90 Synthesis, Molecules, 2021, 26, 1573 CrossRef PubMed.
- F. K. Shieh, S. C. Wang, S. Y. Leo and K. C. Wu, Water-based synthesis of zeolitic imidazolate framework-90 (ZIF-90) with a controllable particle size, Chemistry, 2013, 19, 11139–11142 CrossRef CAS PubMed.
- X. Li, B. Tang, W. Huang and H. Yu, Effect from Mechanical Stirring Time and Speed on Adsorption Performance of ZIF-90 for n-Hexane, Z. Anorg. Allg. Chem., 2019, 645, 73–78 CrossRef CAS.
- H. Li, A. Kang, B. An, L. Y. Chou, F. K. Shieh, C. K. Tsung and C. Zhong, Encapsulation of bacterial cells in cytoprotective ZIF-90 crystals as living composites, Mater. Today Bio, 2021, 10, 100097 CrossRef CAS PubMed.
- F. M. Zhang, H. Dong, X. Zhang, X. J. Sun, M. Liu, D. D. Yang, X. Liu and J. Z. Wei, Postsynthetic Modification of ZIF-90 for Potential Targeted Codelivery of Two Anticancer Drugs, ACS Appl. Mater. Interfaces, 2017, 9, 27332–27337 CrossRef CAS PubMed.
- C. G. Jones, V. Stavila, M. A. Conroy, P. Feng, B. V. Slaughter, C. E. Ashley and M. D. Allendorf, Versatile synthesis and fluorescent labeling of ZIF-90 nanoparticles for biomedical application, ACS Appl. Mater. Interfaces, 2016, 8, 7623–7630 CrossRef CAS PubMed.
- Z. Jiang, Y. Wang, L. Sun, B. Yuan, Y. Tian, L. Xiang, Y. Li, Y. Li, J. Li and A. Wu, Dual ATP and pH responsive ZIF-90 nanosystem with favorable biocompatibility and facile post-modification improves therapeutic outcomes of triple negative breast cancer in vivo, Biomaterials, 2019, 197, 41–50 CrossRef CAS PubMed.
- J. E. Bader, K. Vossn and J. C. Rathmell, Targeting Metabolism to Improve the Tumour Microenvironment for Cancer Immunotherapy, Mol. Cell, 2020, 78, 1019–1033 CrossRef CAS PubMed.
- Y. Xiao and D. Yu, Tumour microenvironment as a therapeutic target in cancer, Pharmacol. Ther., 2021, 221, 107753 CrossRef CAS PubMed.
- C. E. DeSantis, C. C. Lin, A. B. Mariotto, R. L. Siegel, K. D. Stein, J. L. Kramer, R. Alteri, A. S. Robbins and A. Jemal, Cancer Treatment and Survivorship Statistics, Ca-Cancer J. Clin., 2014, 64, 252–271 CrossRef PubMed.
- Q. Li, H. Luo, Y. Y. Luo, W. Zhang, H. M. Hong, M. S. Deng, Y. Wang, B. Xu, G. B. Song and C. X. Xu, Plumbagin-loaded ZIF-90 nanoparticles suppress gastric cancer progression by targeting the YAP1 signaling, Chem. Eng. J., 2022, 437, 135369 CrossRef CAS.
- F. Ge, R. Qiao, P. Song, Y. Tao, L. Zhu, W. Zhang, W. Li and L. Gui, Construction of the targeted and pH-sensitive paclitaxel drug delivery system RGD/PTX@ZIF-90 and anti-tumour activity research, Mater. Res. Express, 2021, 8, 045012 CrossRef CAS.
- X. Xiao, S. Liang, Y. Zhao, D. Huang, B. Xing, Z. Cheng and J. Lin, Core-shell structured 5-FU@ZIF-90@ZnO as a biodegradable nanoplatform for synergistic cancer therapy, Nanoscale, 2020, 12, 3846–3854 RSC.
- M. D. Yaqoob, L. Xu, C. Li, M. M. L. Leong and D. D. Xu, Targeting mitochondria for cancer photodynamic therapy, Photodiagn. Photodyn. Ther., 2022, 38, 102830 CrossRef CAS PubMed.
- F. D. Virgilio, A. C. Sarti and S. Falzoni, Extracellular ATP and P2 purinergic signalling in the tumour microenvironment, Nat. Rev. Cancer, 2018, 18, 601–618 CrossRef PubMed.
- Q. Guan, L. L. Zhou, Y. A. Li and Y. B. Dong, Diiodo-Bodipy-Encapsulated Nanoscale Metal-Organic Framework for pH-Driven Selective and Mitochondria Targeted Photodynamic Therapy, Inorg. Chem., 2018, 57, 10137–10145 CrossRef CAS PubMed.
- W. Pan, B. Cui, K. Wang, M. Shi, F. Lu, N. Li and B. Tang, ATP-triggered mitochondrial cascade reactions for cancer therapy with nanoscale zeolitic imidazole framework-90, Theranostics, 2021, 11, 7869–7878 CrossRef CAS PubMed.
- Y. Xing, Z. Jiang, O. U. Akakuru, Y. He, A. Li, J. Li and A. Wu, Mitochondria-targeting zeolitic imidazole frameworks to overcome platinum-resistant ovarian cancer, Colloids Surf., B, 2020, 189, 110837 CrossRef CAS PubMed.
- F. M. Zhang, H. Dong, X. Zhang, X. J. Sun, M. Liu, D. D. Yang, X. Liu and J. Z. Wei, Postsynthetic Modification of ZIF-90 for Potential Targeted Codelivery of Two Anticancer Drugs, ACS Appl. Mater. Interfaces, 2017, 9, 27332–27337 CrossRef CAS PubMed.
- S. Kwiatkowski, B. Knap, D. Przystupski, J. Saczko, E. Kędzierska, K. Knap-Czop, J. Kotlińska, O. Michel, K. Kotowski and J. Kulbacka, Photodynamic therapy-mechanisms, photosensitizers and combinations, Biomed. Pharmacother., 2018, 106, 1098–1107 CrossRef PubMed.
- M. Yang, J. Li, P. Gu and X. Fan, The application of nanoparticles in cancer immunotherapy: Targeting tumour microenvironment, Bioact Mater., 2020, 6, 1973–1987 Search PubMed.
- B. Ji, M. Wei and B. Yang, Recent advances in nanomedicines for photodynamic therapy (PDT)-driven cancer immunotherapy, Theranostics, 2022, 12, 434–458 CrossRef CAS PubMed.
- D. Ziental, D. T. Mlynarczyk, B. Czarczynska-Goslinska, K. Lewandowski and L. Sobotta, Photosensitizers Mediated Photodynamic Inactivation against Fungi, Nanomaterials, 2021, 11, 2883 CrossRef CAS PubMed.
- J. Zhang, J. Yang, X. Qin, J. Zhuang, D. Jing, Y. Ding, B. Lu, Y. Wang, T. Chen and Y. Yao, Glucose Oxidase Integrated Porphyrinic Covalent Organic Polymers for Combined Photodynamic/Chemodynamic/Starvation Therapy in Cancer Treatment, ACS Biomater. Sci. Eng., 2022, 8, 1956–1963 CrossRef CAS PubMed.
- Z. H. Bao, K. X. Li, P. P. Hou, R. Xiao, Y. Yuan and Z. H. Sun, Nanoscale metal–organic framework composites for phototherapy and synergistic therapy of cancer, Mater. Chem. Front., 2021, 5, 1632–1654 RSC.
- J. Shen, M. Ma, H. Zhang, H. Yu, F. Xue, N. Hao and H. Chen, Microfluidics-Assisted Surface Trifunctionalization of a Zeolitic Imidazolate Framework Nanocarrier for Targeted and Controllable Multitherapies of Tumours, ACS Appl. Mater. Interfaces, 2020, 12, 45838–45849 CrossRef CAS PubMed.
- M. Shang, Y. Wu, Y. Wang, Y. Cai, J. Jin and Z. Yang, Dual antisense oligonucleotide targeting miR-21/miR-155 synergize photodynamic therapy to treat triple-negative breast cancer and inhibit metastasis, Biomed. Pharmacother., 2022, 146, 112564 CrossRef CAS PubMed.
- X. Huang, Y. Lu, M. Guo, S. Du and N. Han, Recent strategies for nano-based PTT combined with immunotherapy: from a biomaterial point of view, Theranostics, 2021, 11, 7546–7569 CrossRef CAS PubMed.
- W. Feng, X. Han, R. Wang, X. Gao, P. Hu, W. Yue, Y. Chen and J. Shi, Nanocatalysts-augmented and photothermalenhanced tumour-specific sequential nanocatalytic therapy in both NIR-I and NIR-II biowindows, Adv. Mater., 2019, 31, 1805919 CrossRef PubMed.
- Y. Ma, C. Wang, L. Zhu, C. Yu, B. Lu, Y. Wang, Y. Ding, C. M. Dong and Y. Yao, Polydopamine-drug conjugate nanocomposites based on ZIF-8 for targeted cancer photothermal-chemotherapy, J. Biomed. Mater. Res., 2022, 110, 954–963 CrossRef CAS PubMed.
- M. Yu, W. Zeng, Y. Ouyang, S. Liang, Y. Yi, H. Hao, J. Yu, Y. Liu, Y. Nie, T. Wang, Y. Deng and M. Wu, ATP-exhausted nanocomplexes for intratumoural metabolic intervention and photoimmunotherapy, Biomaterials, 2022, 284, 121503 CrossRef CAS PubMed.
- M. Yu, J. Wu, J. Shi and O. C. Farokhzad, Nanotechnology for protein delivery: Overview and perspectives, J. Controlled Release, 2016, 240, 24–37 CrossRef CAS PubMed.
- G. Chen, W. Kang, W. Li, S. Chen and Y. Gao, Oral delivery of protein and peptide drugs: from non-specific formulation approaches to intestinal cell targeting strategies, Theranostics, 2022, 12, 1419–1439 CrossRef CAS PubMed.
- Y. Guo, Y. Li, S. Zhou, Q. Ye, X. Zan and Y. He, Metal-Organic Framework-Based Composites for Protein Delivery and Therapeutics, ACS Biomater. Sci. Eng., 2022, 8, 4028–4038 CrossRef CAS PubMed.
- X. Yang, Q. Tang, Y. Jiang, M. Zhang, M. Wang and L. Mao, Nanoscale ATP-Responsive Zeolitic Imidazole Framework-90 as a General Platform for Cytosolic Protein Delivery and Genome Editing, J. Am. Chem. Soc., 2019, 141, 3782–3786 CrossRef CAS PubMed.
- Q. W. Chen, X. H. Liu, J. X. Fan, S. Y. Peng, J. W. Wang, X. N. Wang, C. Zhang, C. J. Liu and X. Z. Zhang, Self-Mineralized Photothermal Bacteria Hybridizing with Mitochondria-Targeted Metal-Organic Frameworks for Augmenting Photothermal Tumour Therapy, Adv. Funct. Mater., 2020, 30, 1909806 CrossRef CAS.
- H. Guo, L. Liu, Q. Hu and H. Dou, Monodisperse ZIF-8@dextran nanoparticles co-loaded with hydrophilic and hydrophobic functional cargos for combined near-infrared fluorescence imaging and photothermal therapy, Acta Biomater., 2022, 137, 290–304 CrossRef CAS PubMed.
- K. Tabatabaeian, M. Simayee, A. Fallah-Shojaie and F. Mashayekhi, N-doped carbon nanodots@UiO-66-NH2 as novel nanoparticles for releasing of the bioactive drug, rosmarinic acid and fluorescence imaging, Daru, J. Pharm. Sci., 2019, 27, 307–315 CrossRef CAS PubMed.
- Y. Xin, D. Zhang, Y. Zeng, Y. Wang and P. Qi, A dual-emission ratiometric fluorescent sensor based on copper nanoclusters encapsulated in zeolitic imidazolate framework-90 for rapid detection and imaging of adenosine triphosphate, Anal. Methods, 2023, 15, 788–796 RSC.
- M. J. Hou, J. T. Chen, W. L. Jiang, G. F. Zeng, J. Zhan, G. J. Mao and C. Y. Li, ATP fluorescent nanoprobe based on ZIF-90 and near-infrared dyes for imaging in tumour mice, Sens. Actuators, B, 2022, 369, 132286 CrossRef CAS.
- R. Zou, Q. Gong, Z. Shi, J. Zheng, J. Xing, C. Liu, Z. Jiang and A. Wu, A ZIF-90 nanoplatform loaded with an enzyme-responsive organic small-molecule probe for imaging the hypoxia status of tumour cells, Nanoscale, 2020, 12, 14870–14881 RSC.
- Y. I. Won, Y. Choi, W. T. Yuh, S. W. Kwon, C. H. Kim, S. H. Yang and C. K. Chung, Validity of magnetic resonance imaging (MRI) in the primary spinal cord tumours in routine clinical setting, Sci. Rep., 2022, 12, 1–8 CrossRef PubMed.
- Z. Jiang, B. Yuan, N. Qiu, Y. Wang, L. Sun, Z. Wei, Y. Li, J. Zheng, Y. Jin, Y. Li, S. Du, J. Li and A. Wu, Manganese-Zeolitic Imidazolate Frameworks-90 with High Blood Circulation Stability for MRI-Guided Tumour Therapy, Nano-Micro Lett., 2019, 11, 61 CrossRef CAS PubMed.
- T. Lin, T. Qin, S. Jiang, C. Zhang and L. Wang, Anti-inflammatory and anti-biotic drug metronidazole loaded ZIF-90 nanoparticles as a pH responsive drug delivery system for improved pediatric sepsis management, Microb. Pathog., 2022, 176, 105941 CrossRef PubMed.
- X. Xu, K. Lin, Y. Wang, K. Xu, Y. Sun, X. Yang, M. Yang, Z. He, Y. Zhang, H. Zheng and X. Chen, A metal-organic framework based inner ear delivery system for the treatment of noise-induced hearing loss, Nanoscale, 2020, 12, 16359–16365 RSC.
- W. Li, S. Chen, Y. Yang, Y. Song, C. Ma, X. Qiao and C. Hong, Ultrasensitive electrochemical immunosensor based on the signal amplification strategy of the competitive reaction of Zn2+ and ATP ions to construct a “signal on” mode GOx-HRP enzyme cascade reaction, Mikrochim. Acta, 2021, 188, 61 CrossRef CAS PubMed.
- S. Wu, Z. Sun, Y. Peng, Y. Han, J. Li, S. Zhu, Y. Yin and G. Li, Peptide-functionalized metal-organic framework nanocomposite for ultrasensitive detection of secreted protein acidic and rich in cysteine with practical application, Biosens. Bioelectron., 2020, 169, 112613 CrossRef CAS PubMed.
- Z. Xie, X. Cai, C. Sun, S. Liang, S. Shao, S. Huang, Z. Cheng, M. Pang, B. Xing and A. A. A. Kheraif, and Jun Lin, O2-Loaded pH-Responsive Multifunctional Nanodrug Carrier for Overcoming Hypoxia and Highly Efficient Chemo-Photodynamic Cancer Therapy, Chem. Mater., 2019, 31, 483–490 CrossRef CAS.
- Y. Wang, D. Jing, J. Yang, S. Zhu, J. Shi, X. Qin, W. Yin, J. Wang, Y. Ding, T. Chen, B. Lu and Y. Yao, Glucose oxidase-amplified CO generation for synergistic anticancer therapy via manganese carbonyl-caged MOFs, Acta Biomater., 2022, 154, 467–477 CrossRef CAS PubMed.
|
This journal is © the Partner Organisations 2024 |
Click here to see how this site uses Cookies. View our privacy policy here.