DOI:
10.1039/C9QO00750D
(Research Article)
Org. Chem. Front., 2019,
6, 2949-2953
n
BuLi-promoted anti-Markovnikov selective hydroboration of unactivated alkenes and internal alkynes†
Received
10th June 2019
, Accepted 28th June 2019
First published on 2nd July 2019
Abstract
An efficient and general nBuLi-promoted anti-Markovnikov selective hydroboration of various α-alkenes, 1,1-disubstituted alkenes and internal alkynes with pinacolborane for the synthesis of alkylboronic esters is described. This protocol features easy accessibility of catalysts and substrates, a broad substrate scope, and simple operation as well as scale-up ability.
Introduction
Alkylboronic esters have been recognized as highly important and versatile synthetic intermediates in organic synthesis and well known due to their unique transformation of C–B bonds and participation in various transition-metal catalysed C–C coupling reactions to access molecules of high value.1 Besides, such compounds also exist in pharmaceutically active molecules or act as bioisosteres in drug design.2 Therefore, a wide array of methods has been developed for the synthesis of these crucial compounds, which mainly includes (1) the classic reactions of organometallic reagents (Grignard or lithium reagents) with suitable boron compounds,3 (2) catalytic hydroboration and borylation of alkenes, (3) catalytic borylation of alkyl halides,4 and (4) catalytic C–H bond borylation.5
Olefins are a class of compounds that can be obtained in large quantities, and are common raw materials and essential intermediates in organic synthesis reactions. Ideally, the catalytic hydroboration of alkenes provides a straightforward, useful, and atom-efficient method to access alkylboronic esters from readily available starting materials. As a result, various transition-metal-catalysed6 and main-group-catalysed7 reactions were advanced to enable highly chemo-, regio- and stereoselective hydroboration of alkenes (Scheme 1A). Along the same line, there is also a research focus on the development of protocols following the principles of green chemistry. For example, it is highly desirable to develop simple base catalysed hydroboration methods8–12 of alkenes with comparable efficiency from a practical point of view due to the economic and environmental benefits of base catalysts. However, the scopes of known chemistries on base-promoted hydroboration are limited mainly to carbonyl compounds,9 pyridine10 or terminal alkyne11 (Scheme 1B). In 2017, Zhao and co-workers12 reported that NaOH powder could efficiently promote the hydroboration of carbonyl groups and styrene type substrates in deuterated benzene. However, the scope of styrene substrates was somewhat limited. Very recently, we disclosed nBuLi-initiated reductive relay hydroboration of allylic alcohol and derivatives to prepare alkylboronic esters in a single operation. Mechanistically, we proposed a one-pot three-step process involving anti-Markovnikov hydroboration of a transiently formed terminal alkene.13 However, to our knowledge, a general base-catalysed direct hydroboration method of unactivated alkenes has not been reported. Herein, as our ongoing research on the hydroboration reaction,13,14 we report a simple base promoted selective hydroboration of unactivated alkenes and 1,1-disubstituted styrenes as well as internal alkynes with HBpin to prepare a wide range of boronic esters.
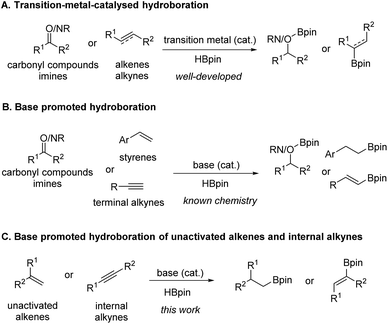 |
| Scheme 1 Transition-metal or base-catalysed hydroboration of unsaturated compounds. | |
Results and discussion
We started the reaction optimization using 4-phenyl-1-butene (1a) as a model substrate in the presence of HBpin (2) and various base catalysts. At the outset, the control experiment using 1a and 2 was conducted at 110 °C without the addition of a base; only trace amounts of the product (3a) were formed (<5% yield, Table 1, entry 1). In contrast, the hydroboration reaction in the presence of 10 mol% of KOBut gave 3a in 75% yield (entry 2), suggesting that a base can accelerate the reaction. We reasoned that a base might weaken the B–H bond to enhance the hydride character which would facilitate this process.15 However, we found that an organic base (entry 3) was not efficient for this transformation. Next, several commercially available and commonly used inorganic bases were extensively evaluated (Table 1, entries 3–9). We were pleased to find that nBuLi was the best catalyst, the use of which led to a full conversion of alkenes after 10 h of reaction to afford 3a in 99% yield (entry 9). Besides, reducing the reaction time to 4 h resulted in only 67% yield of the product (entry 10). It needs to be mentioned that all these reactions delivered linear products as a single isomer, highlighting the excellent anti-Markovnikov regioselectivity of the hydroboration methods.
Table 1 Reaction optimizationa

|
Entry |
Base |
Yield (%) |
Entry |
Base |
Yield (%) |
Yields were determined by NMR analysis using 1,3,5-trimethylbenzene as an internal standard; regioselectivities were determined by GC-MS analysis of the crude reaction mixture, single regioisomers (>99/1) of hydroborated products were obtained for all cases.
Reaction was run in 4h.
|
1 |
— |
<5 |
6 |
Cs2CO3 |
20 |
2 |
t
BuOK |
75 |
7 |
CH3OK |
32 |
3 |
Et3N |
<5 |
8 |
KOH |
25 |
4 |
K3PO4 |
39 |
9 |
n
BuLi |
99 |
5 |
CsF |
40 |
10b |
n
BuLi |
67 |
Under the optimized reaction conditions, we next surveyed the substrate scope of this novel hydroboration method. As depicted in Table 2, a diverse array of α-alkenes was readily transformed into alkylboronic ester products in high to excellent yields. For example, allylic ether (3b), thioether (3c), and silane (3d) were competent substrates affording products in high yield and regioselectivity. Bulkier terminal alkenes (3e and 3f) were efficiently converted into the corresponding product in excellent yields, suggesting that steric effects have no apparent influence on this reaction. Moreover, alkene substrates bearing free alcohol (3g) and a secondary amine (3h) successfully participated in this hydroboration after in situ protection using an additional amount of HBpin. Importantly, this method tolerated a series of functional groups including ethers (3b, 3k–3n), thioethers (3c and 3l), a silane (3d), an alkyl chloride and bromide (3i, 3j), a trifluoromethoxyl group (3k) and a methylthio group (3l). In addition, various heterocycle substituted alkenes, including a furan (3m), a thiophene (3n), a pyrrole (3o), an indole (3p), a carbazole (3q), and a pyrazole (3r), could be applied to this protocol furnished products in high yields.
Table 2 Substrate scope of α-alkenesa
Isolated yields are given; single regioisomers (>99/1) of hydroborated products were obtained as determined by GC-MS analysis.
2.2 equiv. HBpin was used.
|
|
Next, we examined the scope of more challenging 1,1-disubstituted alkene substrates, and the results are depicted in Table 3. We first subjected isopropenylbenzene to the standard hydroboration conditions for α-alkenes, but no reaction was observed. However, when the reaction was performed at a higher temperature (130 °C) and concentration (2.5 M), the desired product (5a) was obtained in 93% yield. Under these conditions, we found that a diverse variety of α-substituted styrenes (5a–4l), and diaryl-substituted (5m), and dialkyl-substituted (5n and 5o) alkenes were compatible, affording various alkylboronic esters in good to excellent yields. Substrates with functional groups such as aryl halides (5d and 5e), and heterocycles including a morpholine (5g), a benzofuran (5i), a pyridine (5j), a thiazole (5k), and a chromane (5l) were all hydroborated without accidents giving products in high yields. A limitation of this method is its inapplicability to internal alkene substrates, likely due to their inert properties and steric effects.
Table 3 Substrate scope of 1,1-disubstituted alkenesa
Isolated yields are given; single regioisomers (>99/1) of hydroborated products were obtained as determined by GC-MS analysis.
|
|
Vinyl boronic esters are versatile coupling partners in organic synthesis and have gained attention increasingly. Over the past few years, the catalytic hydroboration of alkynes has mainly relied on the use of transition metal,16 main group7b,17 or acid18 catalysts. In 2019, Xue and co-workers11 reported that a base efficiently promoted the hydroboration of terminal alkynes to form vinyl boronic esters. However, as indicated by the authors, internal alkynes failed to participate in their reaction conditions and remain an unmet challenge,17e presumably due to the lower reactivity of substrates as well as the difficulty in the control of regioselectivity and stereoselectivity. As an extension of our study, we subjected internal alkynes to our conditions. The results are shown in Table 4. We found that symmetrical diaryl or dialkyl alkynes were readily converted into corresponding vinyl boronic esters (7a, 7b) in high yields, although the E/Z selectivity of 7b is moderate due to easy isomerisation. As for unsymmetrical internal alkynes, both 1,2-alkyl, aryl alkynes (7c) and 1,2-dialkyl alkynes (7d) efficiently participated in the transformation affording products in high yields, albeit in moderate selectivity. Increasing the steric difference of alkyne substituents (7e, 7f) and hydroborated products could result in excellent anti-Markovnikov selectivity (up to 50
:
1).
Table 4 Substrate scope of internal alkynesa
Isolated yields are given.
Determined by 1H NMR analysis of the reaction mixture.
|
|
Next, a gram-scale reaction using 1a was smoothly performed with a lower loading of the nBuLi catalyst (5 mol%) to afford alkylboronic ester 3a in high yield (89%) (Scheme 2). Then, the C–B bonds in 3a, a bench-stable alkylboronic ester, were converted into various carbon–carbon and carbon–heteroatom bonds. For example, 3a can be smoothly oxidized to alcohol 8 in 95% yield. Also, the subjection of 3a to typical Suzuki conditions readily afforded 9 in good yield. Finally, the C–B bond was transformed into a C–N bond (10) in 60% yield through an oxidative amination reaction.19 Given its simple operation, the ready availability of base catalysts, and the rich chemistry of organic boron compounds, the current protocol would constitute a practical means for the transformation of alkenes.
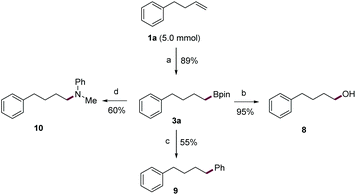 |
| Scheme 2 Gram-scale reaction and transformation of 3a. a 1a (5.0 mmol, 0.66 g), nBuLi (5.0 mol%), HBpin (1.2 equiv.), toluene (5.0 mL), 110 °C, 18 h. b 3a (0.2 mmol), NaBO3·H2O (3.0 equiv.), THF/H2O (1/1, 2.0 mL), r.t., 6 h. c 3a (0.2 mmol), bromobenzene (1.5 equiv.), Pd(OAc)2 (10.0 mol%), rac-BINAP (12.0 mol%), NaOH (15.0 equiv.), THF/H2O (5/1 1.2 ml), 100 °C, 16 h. d 3a (0.2 mmol), N-methylaniline (1.5 equiv.), tBuOOtBu (2.0 equiv.), Cu(OAc)2 (5.0 mol%), toluene (2.0 mL), 80 °C, 24 h. | |
Conclusion
In conclusion, a practical and general base-promoted anti-Markovnikov selective hydroboration of α-alkenes, 1,1-disubstituted alkenes, and internal alkynes with HBpin has been developed. Commercially available nBuLi was employed as a transition-metal-free initiator for this protocol,20 affording a broad array of alkyl or vinyl boronic esters bearing various functional groups and heterocycles in good to excellent yield and selectivity.
Experimental
General procedure for the hydroboration of α-alkenes
In a nitrogen-purged Schlenk tube containing a magnetic stirring bar, toluene (0.5 mL, 1.0 M), HBpin (87.0 μL, 0.6 mmol, 1.2 equiv.) and nBuLi (2.5 M in hexane, 20.0 μL, 0.05 mmol, 10 mol%) were added sequentially. Then the mixture was stirred for 5 min at room temperature. The α-alkene substrate (0.5 mmol) was then added dropwise, and the reaction mixture was stirred at 110 °C for 10 h. After completion of the reaction, the reaction mixture was allowed to cool to r.t. and quenched by using HCl (1.0 M in EtOAc). Then the reaction mixture was directly filtered through a short pad of silica gel, and eluted with EtOAc. The solvent was removed in vacuo. The linear/branched ratios of crude product mixtures were determined at this stage by GC-MS analysis. The product was purified by chromatography on silica gel.
General procedure for the hydroboration of 1,1-disubstituted alkenes and internal alkynes
In a nitrogen-purged Schlenk tube containing a magnetic stirring bar, toluene (0.2 mL, 2.5 M), HBpin (87 μL, 0.6 mmol, 1.2 equiv.) and nBuLi (2.5 M in hexane, 20 μL, 0.05 mmol, 10 mol%) were added sequentially. Then the mixture was stirred for 5 min at room temperature. The alkene or alkyne substrate (0.5 mmol) was then added dropwise, and the reaction mixture was stirred at 130 °C for 12 h. After completion of the reaction, the reaction mixture was allowed to cool to r.t. and quenched by using HCl (1.0 M in EtOAc). Then the reaction mixture was directly filtered through a short pad of silica gel and eluted with EtOAc. The solvent was removed in vacuo. The linear/branched ratios of crude product mixtures were determined at this stage by GC-MS analysis. The product was purified by chromatography on silica gel.
Conflicts of interest
There are no conflicts to declare.
Acknowledgements
We acknowledged the financial support from the Strategic Priority Research Program of the Chinese Academy of Sciences (XDB20000000), “1000-Youth Talents Plan”, the NSF (21690074, 21871288, 91856111, and 21821002), “Innovative Research Team of the Ministry of Education” and “Innovative Research Team in SYPHU by the supporting fund for universities from the Chinese Central Government (51150039)”.
Notes and references
-
(a)
D. S. Matteson, Stereodirected synthesis with organoboranes, Springer Science & Business Media, 2012 Search PubMed
;
(b) H. C. Brown and B. Singaram, Pure Appl. Chem., 1987, 59, 879 CAS
;
(c) A. Suzuki, J. Organomet. Chem., 1999, 576, 147 CrossRef CAS
;
(d) D. Leonori and V. K. Aggarwal, Angew. Chem., Int. Ed., 2015, 54, 1082 CrossRef CAS
.
-
(a) C. Ballatore, D. M. Huryn and A. B. Smith 3rd, ChemMedChem, 2013, 8, 385 CrossRef CAS PubMed
;
(b) C. Li, J. Wang, L. Barton, S. Yu, M. Tian, D. Peters, M. Kumar, A. Yu, K. Johnson, A. Chatterjee, M. Yan and P. Baran, Science, 2017, 356, 1045 CAS
;
(c) B. Das, P. Thapa, R. Karki, C. Schinke, S. Das, S. Kambhampati, S. Banerjee, P. Veldhuizen, A. Verma, L. Weiss and T. Evans, Future Med. Chem., 2013, 5, 653 CrossRef CAS PubMed
.
-
H. C. Brown, G. W. Kramer, A. B. Levy and M. M. Midland, Organic Syntheses via Boranes, Wiley, New York, 1975 Search PubMed
.
- For selected examples on catalytic borylations of alkyl halides:
(a) T. C. Atack and S. P. Cook, J. Am. Chem. Soc., 2016, 138, 6139 CrossRef CAS PubMed
;
(b) S. K. Bose, S. Brand, H. O. Omoregie, M. Haehnel, J. Maier, G. Bringmann and T. B. Marder, ACS Catal., 2016, 6, 8332 CrossRef CAS
;
(c) Y. Cheng, C. Muck-Lichtenfeld and A. Studer, Angew. Chem., Int. Ed., 2018, 57, 16832 CrossRef CAS
.
- For selected examples on catalytic C–H bond borylations:
(a) M. A. Larsen, S. H. Cho and J. Hartwig, J. Am. Chem. Soc., 2016, 138, 762 CrossRef CAS
;
(b) R. L. Reyes, T. Iwai, S. Maeda and M. Sawamura, J. Am. Chem. Soc., 2019, 141, 6817 CrossRef CAS
. For review:
(c) L. Xu, G. Wang, S. Zhang, H. Wang, L. Wang, L. Liu, J. ao and P. Li, Tetrahedron, 2017, 73, 7123 CrossRef CAS
.
- For reviews on transition-metal-catalysed hydroboration of alkenes:
(a) J. V. Obligacion and P. J. Chirik, Nat. Rev. Chem., 2018, 2, 15 CrossRef CAS
;
(b) J. Chen, J. Guo and Z. Lu, Chin. J. Chem., 2018, 36, 1075 CrossRef CAS
;
(c) J. Chen and Z. Lu, Org. Chem. Front., 2018, 5, 260 RSC
;
(d) Z. Huang, Z. Zuo, H. Wen and G. Liu, Synlett, 2018, 29, 1421 CrossRef
;
(e) W. Fan, L. Li and G. Zhang, J. Org. Chem., 2019, 84, 5987 CrossRef CAS
.
- For selected examples on main-group-catalysed hydroboration of alkenes:
(a) Q. Yin, S. Kemper, H. F. Klare and M. Oestreich, Chem. – Eur. J., 2016, 22, 13840 CrossRef CAS
;
(b) N. Ang, C. Buettner, S. Docherty, A. Bismuto, J. R. Carney, J. H. Docherty, M. J. Cowley and S. P. Thomas, Synthesis, 2017, 803 Search PubMed
;
(c) A. Bismuto, M. J. Cowley and S. P. Thomas, ACS Catal., 2018, 8, 2001 CrossRef CAS
.
- For Sc-catalysed hydroboration of alkenes: S. Mandal, P. K. Verma and K. Geetharani, Chem. Commun., 2018, 54, 13690 RSC
.
- For base-promoted hydroboration of relatively reactive compounds (carbonyls):
(a) I. P. Query, P. A. Squier, E. M. Larson, N. A. Isley and T. B. Clark, J. Org. Chem., 2011, 76, 6452 CrossRef CAS
;
(b) D. Mukherjee, H. Osseili, T. P. Spaniol and J. Okuda, J. Am. Chem. Soc., 2016, 138, 10790 CrossRef CAS
;
(c) W. K. Shin, H. Kim, A. K. Jaladi and D. K. An, Tetrahedron, 2018, 74, 6310 CrossRef CAS
;
(d) Z. Zhu, X. Wu, X. Xu, Z. Wu, M. Xue, Y. Yao, Q. Shen and X. Bao, J. Org. Chem., 2018, 83, 10677 CrossRef CAS
.
- E. Jeong, J. Heo, S. Park and S. Chang, Chem. – Eur. J., 2019, 25, 6320 CrossRef CAS PubMed
.
- D. Yan, X. Wu, J. Xiao, Z. Zhu, X. Xu, X. Bao, Y. Yao, Q. Shen and M. Xue, Org. Chem. Front., 2019, 6, 648 RSC
.
- Y. Wu, C. Shan, J. Ying, J. Su, J. Zhu, L. L. Liu and Y. Zhao, Green Chem., 2017, 19, 4169 RSC
.
- Z. C. Wang, D. Shen, J. Gao, X. Jia, Y. J. Xu and S. L. Shi, Chem. Commun., 2019 10.1039/c9cc03459e
.
- Y. Cai, X. T. Yang, S. Q. Zhang, F. Li, Y. Q. Li, L. X. Ruan, X. Hong and S. L. Shi, Angew. Chem., Int. Ed., 2018, 57, 1376 CrossRef CAS
.
-
(a) J. H. Docherty, J. Peng, A. P. Dominey and S. P. Thomas, Nat. Chem., 2017, 9, 595 CrossRef CAS
;
(b) J. M. Farrell, R. T. Posaratnanathana and D. W. Stephan, Chem. Sci., 2015, 6, 2010 RSC
.
- For selected examples on transition-metal-catalysed hydroboration of alkynes:
(a) T. Ohmura, Y. Yamamoto and N. Miyaura, J. Am. Chem. Soc., 2000, 122, 4990 CrossRef CAS
;
(b) C. Gunanathan, M. Holscher, F. Pan and W. Leitner, J. Am. Chem. Soc., 2012, 134, 14349 CrossRef CAS
;
(c) J. V. Obligacion, J. M. Neely, A. N. Yazdani, I. Pappas and P. J. Chirik, J. Am. Chem. Soc., 2015, 137, 5855 CrossRef CAS
;
(d) N. Gorgas, L. G. Alves, B. Stoger, A. M. Martins, L. F. Veiros and K. Kirchner, J. Am. Chem. Soc., 2017, 139, 8130 CrossRef CAS
;
(e) N. Gorgas, B. Stöger, L. F. Veiros and K. Kirchner, ACS Catal., 2018, 8, 7973 CrossRef CAS
.
-
(a) Z. Yang, M. Zhong, X. Ma, K. Nijesh, S. De, P. Parameswaran and H. W. Roesky, J. Am. Chem. Soc., 2016, 138, 2548 CrossRef CAS PubMed
;
(b) J. S. McGough, S. M. Butler, I. A. Cade and M. J. Ingleson, Chem. Sci., 2016, 7, 3384 RSC
;
(c) A. Bismuto, S. P. Thomas and M. J. Cowley, Angew. Chem., Int. Ed., 2016, 55, 15356 CrossRef CAS
;
(d) J. R. Lawson, L. C. Wilkins and R. L. Melen, Chem. – Eur. J., 2017, 23, 10997 CrossRef CAS
;
(e) G. Zhang, J. Wu, H. Zeng, M. C. Neary, M. Devany, S. Zheng and P. A. Dub, ACS Catal., 2018, 9, 874 CrossRef
.
-
(a) H. E. Ho, N. Asao, Y. Yamamoto and T. Jin, Org. Lett., 2014, 16, 4670 CrossRef CAS
;
(b) R. Mamidala, V. K. Pandey and A. Rit, Chem. Commun., 2019, 55, 989 RSC
.
- S. Sueki and Y. Kuninobu, Org. Lett., 2013, 15, 1544 CrossRef CAS
.
- See the ESI for a mechanistic discussion.†.
Footnote |
† Electronic supplementary information (ESI) available. See DOI: 10.1039/c9qo00750d |
|
This journal is © the Partner Organisations 2019 |
Click here to see how this site uses Cookies. View our privacy policy here.