DOI:
10.1039/C9SC04482E
(Edge Article)
Chem. Sci., 2020,
11, 290-294
Synthesis of amino acids and peptides with bulky side chains via ligand-enabled carboxylate-directed γ-C(sp3)–H arylation†
Received
5th September 2019
, Accepted 9th November 2019
First published on 11th November 2019
Abstract
Amino acids and peptides with bulky side chains are of significant importance in organic synthesis and modern medicinal chemistry. The efficient synthesis of these molecules with full enantiocontrol and high diversity remains challenging. Herein we report a Pd-catalyzed ligand-enabled γ-C(sp3)–H arylation of tert-leucine and its derived peptides without using an external directing group (DG) via a less favored six-membered palladacycle. Structurally diverse bulky side chain amino acids and peptides were accessed in a step-economic fashion and the reaction could be conducted on a gram scale with retention of chirality. The resulting amino acids can be used as chiral ligands in Co(III)-catalyzed enantioselective C(sp3)–H amidation. It is worth noting that the weakly coordinating carboxylate DG outcompetes the strongly coordinating bidentate DG of the peptide backbone, providing the products of γ-C(sp3)–H arylation of Tle residue exclusively. This protocol represents the first example of late stage C(sp3)–H functionalization of peptides using a weakly coordinating directing group.
Introduction
Amino acids with bulky side chains are important structural motifs in a broad range of natural products and pharmaceuticals.1 For example, Saxagliptin is a highly potent dipeptidyl peptidase IV Inhibitor for the treatment of type 2 diabetes.1a Asunaprevir is a new generation highly potent hepatitis C virus protease inhibitor in phase III clinical trials (Fig. 1a).1b More recently, amino acids with bulky side chains have been recognized as efficient chiral ligands for enantioselective C–H functionalization via either an outer-sphere (Fig. 1b)2–5 or an inner-sphere pathway (Fig. 1c).6,7 A series of phthalimide protected amino acid derivatives were first realized as efficient ligands for dirhodium(II) complexes for enantioselective C–H insertion of carbenoid by Hashimoto and co-workers (Fig. 1b).3 Although the optimal catalyst might vary depending on the reactions, the sterically bulky tert-leucine (Tle) derived catalyst Rh2(S-PTTL)4 generally gives the best enantiocontrol.3 It has also been realized that apart from the steric bulk of amino acid side chains, the type of phthalimide protecting group also plays crucial roles in asymmetric induction.2–4 Müller and Dauban reported that a 1,8-naphthoyl-protected Tle derived catalyst Rh2(S-NTTL)4 (ref. 4a) was the optimum catalyst that allows highly diastereoselective intermolecular C–H amination.4b The Davies group synthesized sterically bulky adamantly-derived Rh2(S-PTAD)4 and Rh2(S-TCPTAD)4 for asymmetric carbenoid C–H insertion.5 More recently, phthalimide protected bulky side chain amino acids have also been realized as popular chiral ligands in group 9 Cp*M(III)-catalyzed C–H activation.6–8 Cramer and co-workers reported that a strong cooperative effect between the chiral Cpx Ir(III)/Rh(III) and Phth-Tle enabled the enantioselective C–H functionalization of phosphine oxides.6 Bulky side chain amino acids also show remarkable activity in Cp*Co(III)-catalyzed C–H activation. Matsunaga reported the enantioselective C(sp3)–H amidation of thiomides catalyzed by achiral Cp*Co(III) and di-N-protected (S)–H2-BHTL.7 It was found that both of the steric bulk of the side chains and the structure of the protecting groups can significantly influence the asymmetric induction and reactivity. However, the limited availability of sterically bulky amino acids has hampered further development of asymmetric C–H activation reactions. Thus, the development of a novel strategy to diversely access chiral amino acids with bulky side chains would be highly desirable.
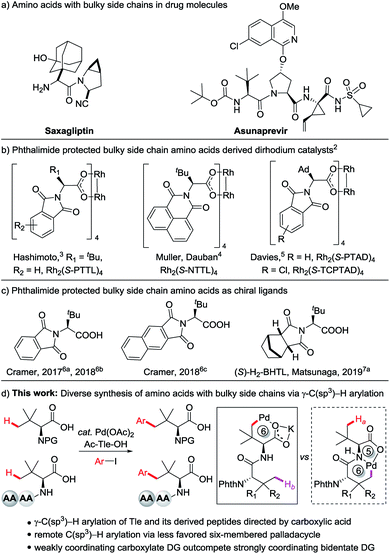 |
| Fig. 1 The importance of amino acids with bulky side chains and our synthetic strategy: (a) amino acids with bulky side chains in drug molecules; (b) phthalimide protected bulky side chain amino acids derived dirhodium catalysts; (c) phthalimide protected bulky side chain amino acids as chiral ligands; (d) this work: diverse synthesis of amino acids with bulky side chains via γ-C(sp3)–H arylation. | |
Undoubtedly, the direct functionalization of commercially available, sterically bulky tert-leucine would offer expeditious access of amino acids with increased steric bulk,9 which might find broad applications in asymmetric reactions. Although the direct functionalization of aliphatic carboxylic acid derivatives using covalently attached external directing groups (DGs) has been well investigated,10 the direct transformation of aliphatic C–H bonds of carboxylic acids without using an exogenous DG remains challenging, largely due to low reactivity resulted from the weak directing ability of carboxyl groups.11 Recently, Pd-catalyzed β-C(sp3)–H functionalization of free carboxylic acids through a kinetically favored five-membered palladacycle has been reported by Yu12 and others.13 Remote C(sp3)–H functionalization using a weakly coordinating carboxylate DG via a six-membered palladacycle remains undeveloped.14 Herein we report a palladium-catalyzed carboxylate-directed γ-C(sp3)–H arylation to synthesize a wide range of large steric hindrance amino acids through a thermodynamically less stable six-membered palladacycle. γ-C(sp3)–H arylation of Tle-derived peptides is also feasible. It is worth noting that the weakly coordinating carboxylate DG outcompetes the strongly coordinating bidentate DG of the peptide backbone,15 providing the products of γ-C(sp3)–H arylation of Tle residue exclusively (Fig. 1d).
Results and discussion
We initiated our studies by exploring the reaction of Phth-Tle-OH 1 and 1-iodo-4-methoxybenzene 2a. After screening a series of mono-N-protected amino acid ligands, we were delighted to find that the use of Ac-Tle-OH as a ligand gave the arylation product 3a in 15% yield (Table S1,† entry 6).16 We hypothesize that the sterically bulky side chain of Ac-Tle-OH plays an important role in promoting the reaction. The subsequent investigation of additives led to an exciting finding that Ag3PO4 could promote the reaction, giving the arylation product 3a in 40% yield (Table S3,† entry 6). Silver phosphate might play a dual role, functioning as both a halide scavenger and a heteronuclear active species to facilitate C–H cleavage.17 Further investigation of reaction temperature revealed that the reaction could be conducted at 70 °C (Table S4,† entry 4, 46%). Monoarylation product 3a could be obtained in 56% yield with 15% diarylation product 3a′ when using 3 equivalents of 2a (Table S5,† entry 3). When D-Ac-Tle-OH was used as the ligand, identical results were obtained (Table S5,† entry 6, 3a, 57%; 3a′, 14%). We finalized the conditions for the γ-selective arylation of 1 with 2a as follows (reaction conditions A): 10 mol% Pd(OAc)2, 30 mol% Ac-Tle-OH, 1.0 equiv. K2CO3, and 1.0 equiv. Ag3PO4, with HFIP as the solvent at 70 °C for 24 h under air.
With the optimized reaction conditions in hand, we first turned to the evaluation of the scope of aryl iodides (Fig. 2). Both electron-withdrawing and electron-donating groups afforded the desired products in good yields under the optimized conditions. Electron-withdrawing groups, such as acetyl (2b and 2f), methoxycarbonyl (2c and 2i), and formyl (2d and 2g), at either the para or the meta position of the aryl iodides, are well tolerated. Multiple substituted aryl iodides (2j–2m) were also compatible with the reaction conditions, giving the desired products in moderate yields. Notably, β-C–H arylation of Phth-Ala-OH also proceeded smoothly under the optimized reaction conditions, giving the β-arylation product in 51% yield (see the ESI†).
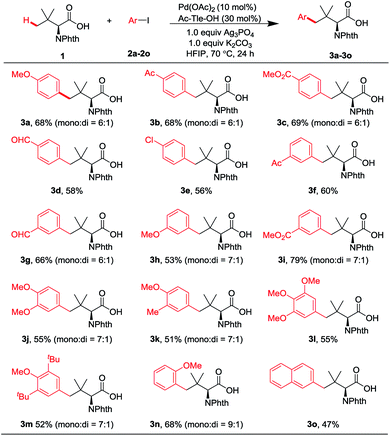 |
| Fig. 2 Scope of aryl iodides for Pd-catalyzed γ-C(sp3)–H arylation. Reaction conditions A: 1 (0.3 mmol), 2 (0.9 mmol), Pd(OAc)2 (0.03 mmol), Ac-Tle-OH (0.09 mmol), Ag3PO4 (0.3 mmol), K2CO3 (0.3 mmol), HFIP (3 mL), 70 °C, air, 24 h, and isolated yield. | |
Recently, transition metal-catalyzed C–H activation has emerged as one of the most powerful strategies for the late-stage modification of structurally complex peptides.18,19 However, C(sp3)–H functionalization of peptides has been largely relied on the use of strong bidentate DGs19 or the biscoordination of the peptide backbone.15 We were pleased to find that this protocol is feasible with peptides under slightly modified conditions (reaction conditions B: Ag2SO4 was used instead of Ag3PO4, Table S2†). Dipeptides containing glycine (4a), cyclohexaneglycine (4b), and leucine (4c) reacted smoothly with 2c to give the arylation products in moderate yields. Yu and coworkers have reported C–H activation of N-terminal amino acids in peptides directed by the N,O- or N,N-biscoordination of the peptide backbone.15 Remarkably, the weakly coordinating carboxylate DG outcompetes the strongly coordinating bidentate DG of the peptide backbone and reacted selectively with the γ-C(sp3)–H bond of Tle residue (Fig. 3b, 5d–5h). Notably, tripeptides were also tolerated, albeit in low yield (5g, 34%; 5h, 23%), which might be due to the competitive coordination of the N,N-biscoordination. The unconventional selectivity might originate from the favorable formation of a six-membered palladacycle over its 5,6-fused bicyclic counterpart (Fig. 3b, Int-A1vs.Int-B1; Int-A2vs.Int-B2). When N-Phth-Ala-Tle-OH (4i), a dipeptide bearing an alanine residue at the N-terminus, was used as the substrate, arylation occurred selectively at β-methyl of the N-terminus. This selectivity is consistent with Yu's report,15a where the formation of a five-membered palladacycle via N,O-bicoordination is favored compared to a six-membered one via a monodentate carboxylate DG (Fig. 3c, Int-B3vs.Int-A3).
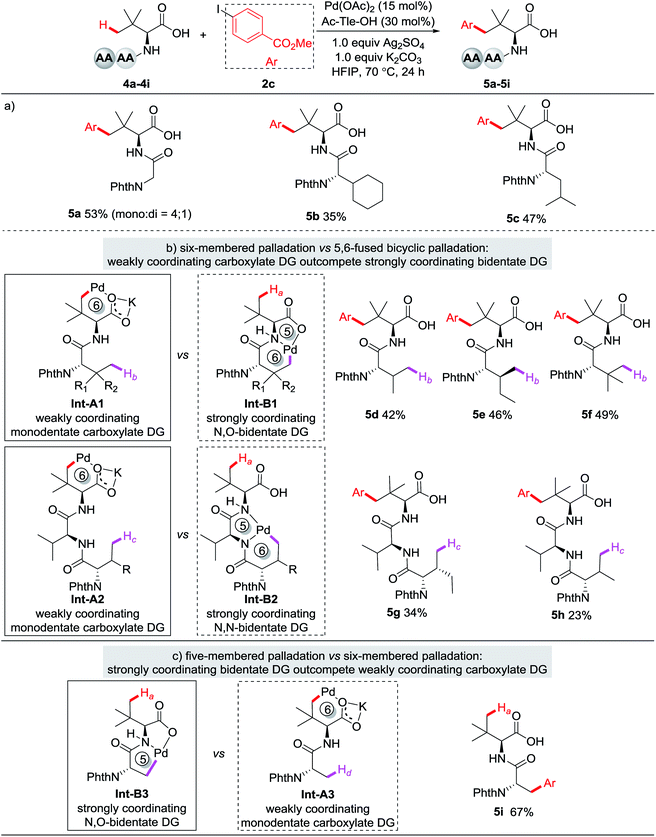 |
| Fig. 3 Scope of different types of peptides (a–c) for Pd-catalyzed γ-C(sp3)–H arylation. Reaction conditions B: 4 (0.1 mmol), 2c (0.3 mmol), Pd(OAc)2 (0.015 mmol), Ac-Tle-OH (0.03 mmol), Ag2SO4 (0.1 mmol), K2CO3 (0.1 mol), HFIP (1 mL), 70 °C, air, 24 h, and isolated yield. | |
Considering that different phthalimide protecting groups have deep influences on asymmetric reactions,3–7 the compatibility of this protocol with diverse phthalimides would be in high demand. Thus, we examined whether other protecting groups could be applicable to carboxylate-directed γ-C(sp3)–H arylation. To our delight, various phthalimides were compatible with this reaction as shown in Fig. 4. The arylation products 7 were obtained in moderate to high yields. In particular, several types of phthalimides that have been used in asymmetric C–H activation,3–7 such as 1,8-naphthoyl (7e), 7g, tetrachlorophthaloyl (7h), and 2,3-naphthoyl (7i), were all tolerated. Succinimide protected Tle (6j) also reacted smoothly to give the desired product 7j in 59% yield. Moreover, Ac-Tle-OH can also react to give the desire product 7kc, albeit in very low yield (22%, mono
:
di = 3
:
1). Unfortunately, the alkoxycarbonyl protecting groups, such as Boc, Fmoc, and Cbz, were incompatible with this protocol.
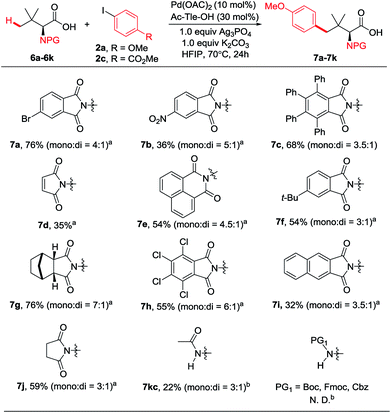 |
| Fig. 4 Scope of protecting groups for Pd-catalyzed C–H arylation. aReaction conditions A: 2a (0.9 mmol) was used as the arylation reagent. bReaction conditions B: 2c (0.9 mmol) was used as the arylation reagent. N. D. = no detected. | |
To demonstrate the practicality of this protocol, gram-scale synthesis of 3a was conducted (Scheme 1a). A 10 mmol-scale reaction of 1 and 2a was performed, delivering 3a in 59% yield (2.18 g) and 3a′ in 12% yield (0.59 g) without erosion of optical purity, which is the prerequisite and necessary condition for application as chiral ligands.
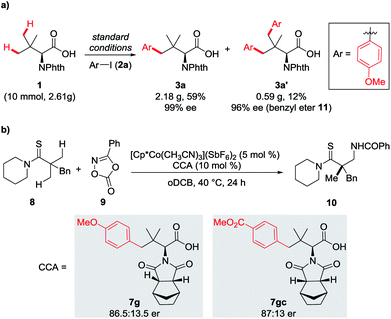 |
| Scheme 1 (a) Gram-scale synthesis of bulky side chain amino acids. (b) Co(III)-catalyzed enantioselective C(sp3)–H amidation of thioamide. | |
The utility of this protocol was further demonstrated by using the resulting bulky side chain amino acids as chiral ligands. Preliminary investigations of Co(III)-catalyzed C(sp3)–H amidation of thioamide 8 with dioxazolone 9 disclosed that 7g and 7gc are highly efficient, giving good enantiocontrol (Scheme 1b, 7g, 86.5:13.5 er; 7gc, 87:13 er; (S)–H2-BHTL, 87:13 er7a). These results indicate that the modified amino acids have high potential in asymmetric reactions, especially considering that two sites, the protecting group motif and the aryl skeleton, could be easily and diversely modified.
Conclusions
In conclusion, a Pd-catalyzed carboxylate-directed γ-C(sp3)–H arylation for the diverse synthesis of bulky side chain amino acids and peptides was developed. Notably, this protocol represents the first example of late stage C(sp3)–H functionalization of peptides using a weakly coordinating directing group. The resulting amino acids with bulky side chains have been successfully applied as chiral ligands in asymmetric C(sp3)–H activation. Studies on further applications of these bulky branched chain amino acids in asymmetric synthesis are currently ongoing.
Conflicts of interest
There are no conflicts to declare.
Acknowledgements
Financial support from the NSFC (21572201 and 21772170), Outstanding Young Talents of Zhejiang Province High-level Personnel of Special Support (ZJWR0108), the National Basic Research Program of China (2015CB856600), the Fundamental Research Funds for the Central Universities (2018XZZX001-02) and the Zhejiang Provincial NSFC (LR17B020001) is gratefully acknowledged.
Notes and references
-
(a) D. J. Augeri, J. A. Robl, D. A. Betebenner, D. R. Magnin, A. Khanna, J. G. Robertson, A. Wang, L. M. Simpkins, P. Taunk, Q. Huang, S.-P. Han, B. Abboa-Offei, M. Cap, L. Xin, L. Tao, E. Tozzo, G. E. Welzel, D. M. Egan, J. Marcinkeviciene, S. Y. Chang, S. A. Biller, M. S. Kirby, R. A. Parker and L. G. Hamann, J. Med. Chem., 2005, 48, 5025–5037 CrossRef CAS
;
(b) P. M. Scola, L.-Q. Sun, A. X. Wang, J. Chen, N. Sin, B. L. Venables, S.-Y. Sit, Y. Chen, A. Cocuzza, D. M. Bilder, S. V. D'Andrea, B. Zheng, P. Hewawasam, Y. Tu, J. Friborg, P. Falk, D. Hernandez, S. Levine, C. Chen, F. Yu, A. K. Sheaffer, G. Zhai, D. Barry, J. O. Knipe, Y.-H. Han, R. Schartman, M. Donoso, K. Mosure, M. W. Sinz, T. Zvyaga, A. C. Good, R. Rajamani, K. Kish, J. Tredup, H. E. Klei, Q. Gao, L. Mueller, R. J. Colonno, D. M. Grasela, S. P. Adams, J. Loy, P. C. Levesque, H. Sun, H. Shi, L. Sun, W. Warner, D. Li, J. Zhu, N. A. Meanwell and F. McPhee, J. Med. Chem., 2014, 57, 1730–1752 CrossRef CAS
.
-
(a) H. M. L. Davies and R. E. J. Beckwith, Chem. Rev., 2003, 103, 2861–2904 CrossRef CAS
;
(b) M. P. Doyle, R. Duffy, M. Ratnikov and L. Zhou, Chem. Rev., 2010, 110, 704 CrossRef CAS
;
(c) H. M. L. Davies and D. Morton, Chem. Soc. Rev., 2011, 40, 1857 RSC
.
-
(a) M. Astada and S. Hashimoto, Tetrahedron Lett., 1998, 39, 79–82 CrossRef
;
(b) T. Takahashi, H. Tsutsui, M. Tamura, S. Kitagaki, M. Nakajima and S. Hashimoto, Chem. Commun., 2001, 17, 1604–1605 RSC
;
(c) H. Saito, H. Oishi, S. Kitagaki, S. Nakamura, M. Anada and S. Hashimoto, Org. Lett., 2002, 4, 3887–3890 CrossRef CAS
;
(d) K. Minami, H. Saito, H. Tsutsui, H. Nambu, M. Anada and S. Hashimoto, Adv. Synth. Catal., 2005, 347, 1483–1487 CrossRef CAS
.
-
(a) P. Müller, Y. Allenbach and E. Robert, Tetrahedron: Asymmetry, 2003, 14, 779–785 CrossRef
;
(b) C. Liang, F. Robert-Peillard, C. Fruit, P. Müller, R. H. Dodd and P. Dauban, Angew. Chem., Int. Ed., 2006, 45, 4641–4644 CrossRef CAS
.
-
(a) R. P. Reddy, G. H. Lee and H. M. L. Davies, Org. Lett., 2006, 8, 3437–3440 CrossRef CAS
;
(b) K. Liao, T. C. Pickel, V. Boyarskikh, J. Bacsa, D. G. Musaev and H. M. L. Davies, Nature, 2017, 551, 609–613 CrossRef CAS
.
-
(a) Y.-S. Jang, M. Dieckmann and N. Cramer, Angew. Chem., Int. Ed., 2017, 56, 15088–15092 CrossRef CAS
;
(b) Y.-S. Jang, Ł. Woźniak, J. Pedroni and N. Cramer, Angew. Chem., Int. Ed., 2018, 57, 12901–12905 CrossRef CAS
;
(c) Y. Sun and N. Cramer, Angew. Chem., Int. Ed., 2018, 57, 15539–15543 CrossRef CAS
.
-
(a) S. Fukagawa, Y. Kato, R. Tanaka, M. Kojima, T. Yoshino and S. Matsunaga, Angew. Chem., Int. Ed., 2018, 131, 1165–1169 CrossRef
;
(b) W. Wang, M. M. Lorion, J. Shah, A. R. Kapdi and L. Ackermann, Angew. Chem., Int. Ed., 2018, 57, 14700–14717 CrossRef CAS
.
- For early reports on the use of mono-protected amino acids as chiral ligands in C–H activation, see:
(a) B.-F. Shi, N. Maugel, Y.-H. Zhang and J.-Q. Yu, Angew. Chem., Int. Ed., 2008, 47, 4882–4886 CrossRef CAS
;
(b) B.-F. Shi, Y.-H. Zhang, J. K. Lam, D.-H. Wang and J.-Q. Yu, J. Am. Chem. Soc., 2010, 132, 460–461 CrossRef CAS
;
(c) D.-W. Gao, Y.-C. Shi, Q. Gu, Z.-L. Zhao and S.-L. You, J. Am. Chem. Soc., 2013, 135, 86–89 CrossRef CAS
;
(d) D.-W. Gao, Q. Yin, Q. Gu and S.-L. You, J. Am. Chem. Soc., 2014, 136, 4841 CrossRef CAS
.
-
(a) G. He, B. Wang, W. A. Nack and G. Chen, Acc. Chem. Res., 2016, 49, 635–645 CrossRef CAS
;
(b) X. Lu, B. Xiao, R. Shang and L. Liu, Chin. Chem. Lett., 2016, 27, 305–311 CrossRef CAS
.
- For selected reviews on C(sp3)–H bond activation:
(a) T. W. Lyons and M. S. Sanford, Chem. Rev., 2010, 110, 1147–1169 CrossRef CAS
;
(b) O. Baudoin, Chem. Soc. Rev., 2011, 40, 4902 RSC
;
(c) G. Rouquet and N. Chatani, Angew. Chem., Int. Ed., 2013, 52, 11726–11743 CrossRef CAS
;
(d) O. Daugulis, J. Roane and L. D. Tran, Acc. Chem. Res., 2015, 4, 1053–1064 CrossRef
;
(e) J. He, M. Wasa, K. S. L. Chan, Q. Shao and J.-Q. Yu, Chem. Rev., 2017, 117, 8754–8786 CrossRef CAS
;
(f) J. C. K. Chu and T. Rovis, Angew. Chem., Int. Ed., 2017, 57, 62–101 CrossRef
;
(g) Q. Zhang and B.-F. Shi, Chin. J. Chem., 2019, 48, 5094 Search PubMed
;
(h) C. He, W. G. Whitehurst and M. J. Guant, Chem, 2019, 5, 1031–1058 CrossRef CAS
.
-
(a) K. M. Engle, T.-S. Mei, M. Wasa and J.-Q. Yu, Acc. Chem. Res., 2012, 45, 788–802 CrossRef CAS
;
(b) G.-F. Shi and Y.-H. Zhang, Adv. Synth. Catal., 2014, 356, 1419–1442 CrossRef CAS
;
(c) M. P. Drapeau and L. J. Gooßen, Chem.–Eur. J., 2016, 22, 18654–18677 CrossRef
.
-
(a) R. Giri, N. Maugel, J.-J. Li, D.-H. Wang, S. P. Breazzano, L. B. Saunders and J.-Q. Yu, J. Am. Chem. Soc., 2007, 129, 3510–3511 CrossRef CAS
;
(b) G. Chen, Z. Zhuang, G.-C. Li, T. G. Saint-Denis, Y. Hsiao, C. L. Joe and J.-Q. Yu, Angew. Chem., Int. Ed., 2017, 129, 1506–1509 CrossRef PubMed
;
(c) L. Hu, P.-X. Shen, Q. Shao, K. Hong, J. X. Qiao and J.-Q. Yu, Angew. Chem., Int. Ed., 2019, 58, 2134–2138 CrossRef CAS PubMed
.
-
(a) K. K. Ghosh and M. van Gemmeren, Chem.–Eur. J., 2017, 23, 17697–17700 CrossRef CAS
;
(b) Y. Zhu, X. Chen, C. Yuan, G. Li, J. Zhang and Y. Zhao, Nat. Commun., 2017, 8, 14904 CrossRef CAS
.
- During the submission of this manuscript, elegant work on γ-C(sp3)–H arylation of free aliphatic acid was reported; however, this protocol was incompatible with Tle and peptides. P. Dolui, J. Das, H. B. Chandrashekar, S. S. Anjana and D. Maiti, Angew. Chem., Int. Ed., 2019, 58, 13773–13777 CrossRef CAS PubMed
.
-
(a) W. Gong, G. Zhang, T. Liu, R. Giri and J.-Q. Yu, J. Am. Chem. Soc., 2014, 136, 16940–16946 CrossRef CAS
;
(b) T. Liu, J. X. Qiao, M. A. Poss and J.-Q. Yu, Angew. Chem., Int. Ed., 2017, 56, 10924–10927 CrossRef CAS
;
(c) A. F. M. Noisier, J. García, I. A. Ionuţ and F. Albericio, Angew. Chem., 2016, 129, 320–324 CrossRef
;
(d) J. Tang, Y. He, H. Chen, W. Sheng and H. Wang, Chem. Sci., 2017, 8, 4565–4570 RSC
.
-
(a) K. M. Engle and J.-Q. Yu, J. Org. Chem., 2013, 78, 8927–8955 CrossRef CAS
;
(b) K. M. Engle, Pure Appl. Chem., 2016, 88, 119–138 CAS
.
- K. L. Bay, Y.-F. Yang and K. N. Houk, J. Organomet. Chem., 2018, 864, 19–25 CrossRef CAS
.
-
(a) W. Wang, M. M. Lorion, O. Martinazzoli and L. Ackermann, Angew. Chem., Int. Ed., 2018, 57, 10554–10558 CrossRef CAS
;
(b) K. Chen and B.-F. Shi, Sci. Bull., 2018, 63, 1238–1240 CrossRef CAS
;
(c) A. F. M. Noisier and M. A. Brimble, Chem. Rev., 2014, 114, 8775–8806 CrossRef CAS
.
-
(a) E. Hernando, J. Villalva, Á. M. Martínez, I. Alonso, N. Rodríguez, R. Gómez Arrayás and J. C. Carretero, ACS Catal., 2016, 6, 6868–6882 CrossRef CAS
;
(b) W. Wang, M. M. Lorion, O. Martinazzoli and L. Ackermann, Angew. Chem., Int. Ed., 2018, 57, 10554–10558 CrossRef CAS
;
(c) X. Zhang, G. Lu, M. Sun, M. Mahankali, Y. Ma, M. Zhang, W. Hua, Y. Hu, Q. Wang, J. Chen, G. He, X. Qi, W. Shen, P. Liu and G. Chen, Nat. Chem., 2018, 10, 540–548 CrossRef CAS
;
(d) B.-B. Zhan, Y. Li, J.-W. Xu, X.-L. Nie, J. Fan, L. Jin and B.-F. Shi, Angew. Chem., Int. Ed., 2018, 57, 5858–5862 CrossRef CAS
.
Footnote |
† Electronic supplementary information (ESI) available. See DOI: 10.1039/c9sc04482e |
|
This journal is © The Royal Society of Chemistry 2020 |
Click here to see how this site uses Cookies. View our privacy policy here.