DOI:
10.1039/D4QO02038C
(Review Article)
Org. Chem. Front., 2025, Advance Article
Recent advances in the photocatalytic cleavage of C–C and C–N bonds associated with amines and their derivatives
Received
31st October 2024
, Accepted 20th November 2024
First published on 22nd November 2024
Abstract
The cleavage of C–C and C–N bonds in ubiquitous amines and their derivatives serves as an important and useful protocol for the construction of valuable organic molecules. Recently, the visible-light-driven photocatalytic strategy for C–C and C–N bond scissions has received much attention because such a strategy has characteristic advantages of mild reaction conditions, unique selectivity and environmentally benign operation in comparison with the traditional thermal reaction protocols. In this review, the recent advances in the visible-light-mediated photocatalytic cleavage of C–C and C–N bonds associated with amines and their derivatives are summarized. At the end of this review, the limitations and challenges in this field are pointed out, and the future research direction of this field is also discussed.
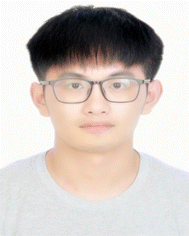 Qibo Ni | Qibo Ni was born in Zhejiang Province (China) in 1999. He received his bachelor's degree from Huzhou University in 2021. Then, he started his graduate research at the Zhejiang University of Technology under the supervision of Prof. Yunkui Liu. His research interests focus on P/N-heteroleptic Cu(I)-photosensitizer-catalyzed radical reactions. |
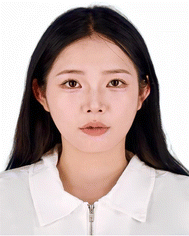 Yan Zhou | Yan Zhou was born in Zhejiang Province (China) in 2000. In 2022, she received her bachelor's degree from Hangzhou Normal University. She continued to pursue her master's degree at Zhejiang Technology University under the supervision of Prof. Yunkui Liu. Her main research interest focuses on P/N-heteroleptic Cu(I)-photosensitizer-catalyzed radical reactions. |
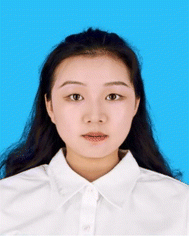 Lang Chen | Lang Chen received her bachelor's degree from the Hunan University of Arts and Science in 2022. She is currently pursuing her master's degree at the Zhejiang University of Technology under the supervision of Prof. Yunkui Liu. Her main research interest focuses on late-transition-metal-catalyzed reactions including P/N-heteroleptic Cu(I)-photosensitizer-catalyzed reactions. |
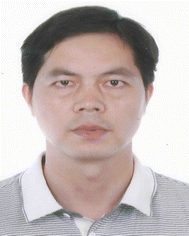 Yunkui Liu | Yunkui Liu is a professor at the Zhejiang University of Technology. He received his PhD degree (2002) in organic chemistry from Zhejiang University (China). He then studied at Hokkaido University (Japan) as a JSPS/JST postdoctoral fellow (2002–2005) supervised by professor Yasushi Tsuji. He was a visiting scholar at the University of California at Santa Barbara (USA) (2017–2018). His current research interests focus on late-transition-metal-catalyzed highly selective reactions, including P/N-heteroleptic Cu(I)-photosensitizer-, homogeneous Au(I)-, and other late-transition-metal-catalyzed reactions. |
1. Introduction
Amines (primary, secondary, and tertiary) are widely found in natural products, pharmaceuticals, biomolecules, and functional materials.1 They are easily available from natural sources as well as artificial synthesis.1,2 Thanks to the versatile synthetic platform based on amines, they can be easily converted to a wide range of functionally and/or synthetically valuable N-containing compounds, such as imines, amides, quaternary ammonium compounds, Katritzky salts, azo- and diazo compounds, isonitriles, N-heterocycles, etc. Both amines and their derivatives play an extraordinarily significant role in academia and industry.
The cleavage and formation of chemical bonds has been the core of discussion since the beginning of organic chemistry. Compared with the cleavage of chemical bonds with low dissociation energies (such as the C–Br/I bond),3 the cleavage of C–C4 and C–N5 bonds, which have shorter bond lengths (also have higher dissociation energies) and are not easily polarized, is a more challenging task. Therefore, chemists are committed to achieving the cleavage of C–C and C–N bonds through various means. To date, the cleavage of C–C bonds has been mainly achieved by three traditional thermochemical strategies: (a) activation of C–C bonds through transition metal-catalyzed oxidative addition processes,4b,6 (b) β-carbon elimination,7 and (c) the metal-mediated retro-allylation process.8 As for the cleavage of C–N bonds, it is often necessary to pre-activate the bonds, such as by conversion into iminium,9 diazonium,10 quaternary ammonium11 and pyridinium salts (Katritzky salts).12 Despite significant progress being made in the field of C–C and C–N bond scissions, it can be seen that the cleavage of C–C4,6–8 and C–N5,9–12 bonds under thermal reaction conditions often requires relatively harsh reaction conditions, complicated pre-treatments, and/or expensive and toxic transition metal catalysts. With a growing emphasis on resource and energy conservation and environmental concerns,13 the development of novel and efficient methods that allow for the cleavage of C–C and C–N bonds under mild and environmentally friendly reaction conditions has become a desirable synthetic target to achieve in synthetic chemistry.
In the past two decades, with the renaissance of radical chemistry, visible-light-mediated photocatalysis has emerged as an efficient and powerful tool for the construction of molecular complexity because of its unique activation mode via the combination of photosensitizers and visible light.14 Such an activation mode facilitates various sustainable free-radical-mediated processes wherein the cleavage and formation of chemical bonds can be achieved under relatively mild reaction conditions, with unique selectivity and broad functional group tolerance. As a result, recently, more and more elegant photocatalytic reactions involving the cleavage of inert chemical bonds,11d,12d,e,15 certainly including the cleavage of C–C15a–d,k,l and C–N11d,12d,e bonds associated with amines and their derivatives, have been documented in the literature.
Actually, the reactivity of amines has long been a hot research topic in visible-light-mediated photocatalysis. Amines can play different roles in photocatalytic reactions according to their different reactivities. For example, tertiary amines (mostly Et3N and iPr2NEt) have been proved to be faithful partners of excited photocatalysts for serving as electron sacrificial agents, especially in those photocatalytic reactions via the reductive quenching mechanism.14e,16 In these cases, amine additives are merely used as quenching agents for the completion of a photocatalytic cycle wherein they themselves are not incorporated into the final products (Scheme 1a). In addition, some tertiary amines can be utilized as useful HAT (hydrogen atom transfer)17 or XAT (halogen atom transfer)18 agents upon conversion into radical species under visible-light-driven photoredox reaction conditions. In these cases, amines also do not engage in the reassembly process with substrates (Scheme 1b). Moreover, amines can be utilized as reductants for quenching photocatalysts as well as precursors for the generation of nucleophilic α-aminoalkyl radicals that are capable of reacting with various electrophilic reagents.19 Alternatively, they can be used as two-electron donors to reduce photocatalysts as well as precursors for the generation of electrophilic iminium cations that are capable of reacting with various nucleophilic reagents.20 Under these two reactivity scenarios, amines participate in the reassembly process with substrates, but no occurrence of C–C and/or C–N bond scission events associated with them is involved (Scheme 1c). Finally, upon treatment under visible-light photoredox reaction conditions, amines can also undergo C–C and/or C–N bond scission reactions, thereby enabling the generation of various reactive species (Scheme 1d).11d,12d,e,15a–d Compared with the traditional thermal reactions of C–C4 or C–N5 bond scission, such photocatalytic variants via free-radical-induced fragmentation can generally proceed under mild conditions and have therefore become a promising and attractive alternative strategy for the cleavage of C–C or C–N bonds. In recent years, great progress has been made in this field. Under this context, we would like to contribute a review on the recent advances in the visible-light-mediated photocatalytic cleavage of C–C or C–N bonds associated with amines and their derivatives. Since the photocatalytic cleavage of C–C bonds associated with cycloalkylamines (cyclopropylamines and cyclobutylamines)15a,d and Hantzsch esters21 has been previously summarized in several reviews, only the representative and very recent examples associated with these two types of compounds will be discussed in the present review. Besides, since several reviews have previously highlighted the photocatalytic cleavage of C–N bonds associated with Katritzky salts12d,e and quaternary ammonium compounds,11d the photocatalytic reactions concerning these two types of amine derivatives are not covered in this review. We hope that this review could provide a quick overview of the state-of-the-art advances in this flourishing field to related researchers, help them better understand the future development trend, and facilitate the design of innovative photocatalytic reactions.
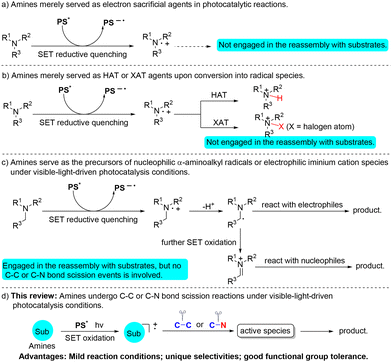 |
| Scheme 1 Various reactivities of amines under visible-light-driven photocatalysis conditions. | |
To compile this review more concisely, the content is first introduced according to two different main categories of chemical bond scission: C–C bond cleavage and C–N bond cleavage. Then each category is further divided into several subcategories according to different bond-scission types which are illustrated in Schemes 2 and 3, respectively. Furthermore, under each subcategory, the detailed progress associated with different classes of amines and their derivatives will be discussed.
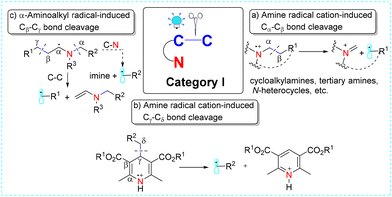 |
| Scheme 2 Different types of C–C bond scissions associated with amines and their derivatives under photoredox conditions. | |
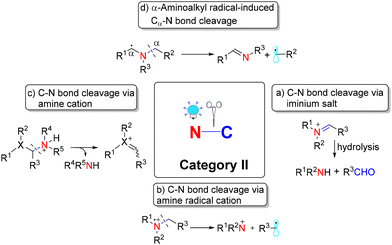 |
| Scheme 3 Different types of C–N bond scissions associated with amines and their derivatives under photoredox conditions. | |
2. Photocatalytic cleavage of C–C bonds
2.1. Amino radical cation species-induced cleavage of Cα–Cβ bonds
Under photoredox catalytic conditions, the lone-pair electrons of N-atoms in amines and their derivatives may easily undergo a single electron transfer (SET) process with excited photocatalysts, leading to the formation of amino radical cation species. Such an amino radical cation species is prone to inducing the cleavage of the Cα–Cβ bond, especially if there is a highly strained alicyclic ring or a leaving group at the β-position to the N-atom, resulting in the formation of a cationic species along with a radical species (or a distonic radical cation in the case of cycloalkylamines) (Scheme 2a, vide supra). Both the resulting cationic species and the radical intermediate may serve as reactive intermediates for various chemical transformations. In this section, we will introduce some representative examples associated with different types of amine derivatives, including cycloalkylamines (cyclopropylamines and cyclobutylamines), tertiary amines, and N-heterocycles.
2.1.1 Amino radical cation species-induced cleavage of Cα–Cβ bonds in cycloalkylamines. Cyclopropylamines and cyclobutylamines belong to small ring compounds, and they are prone to undergoing irreversible opening of their ring via cleavage of the Cα–Cβ bonds upon SET oxidation to the nitrogen-centered radical cation species under visible-light photoredox conditions, leading to the formation of active β-carbon radical iminium ions and γ-carbon radical iminium ions, respectively.15a,d The resulting distonic radical cations are versatile building blocks for a variety of dipolar cycloaddition reactions.22 For instance, in 2012, the Zheng group for the first time reported a Ru(bpz)3(PF6)2-catalyzed intermolecular [3 + 2] cycloaddition of cyclopropylamines 1 and olefins 2 to access aminocyclopentane derivatives 3 with high regioselectivity under visible-light-driven photocatalysis conditions (Scheme 4).23 Regarding the mechanism, first, the reaction starts with the generation of amine radical cation 4 through the SET oxidation of cyclopropylamines 1 by the excited Ru(II)-photocatalyst. A key radical-induced β-fragmentation leads to the three-membered ring opening and the formation of β-carbon radical iminium ion 5. Then the [3 + 2] cycloaddition of 5 with styrene 2 delivers an N-centered radical cation 6. Finally, an SET reduction of 6 by the reductive Ru(I) species affords the target product 3 and regenerates the Ru(II)-photocatalyst. The reaction exhibits excellent regioselectivity and broad functional group tolerance under mild reaction conditions. In addition, Zheng et al. found that the intermolecular [3 + 2] cycloaddition of bicyclic tertiary cyclopropylamines 7 and olefins 8 could also proceed smoothly to give 5,5-fused bicyclic heterocycles 9 with high diastereoselectivity and good substrate compatibility.
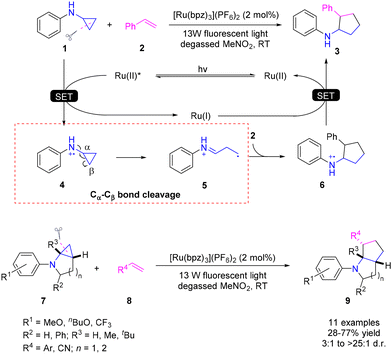 |
| Scheme 4 Visible-light-driven cleavage of the Cα–Cβ bond in cyclopropylamines and a related [3 + 2] cycloaddition reaction with olefins. | |
Similarly, cyclobutylamines can also undergo the cleavage of the Cα–Cβ bonds upon conversion to the nitrogen-centered radical cation species, although in this case, Ir(ppy)2(dtbbpy)PF6 is a more effective photocatalyst than Ru(bpz)3(PF6)2 and more strong irradiation conditions are required.24 The resulting γ-carbon radical iminium ions serve as versatile 1,4-dipolar synthons to react with various π-unsaturated molecules for the convenient construction of synthetically valuable six-membered ring compounds (Scheme 5).
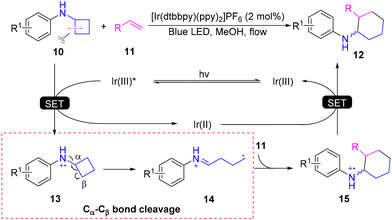 |
| Scheme 5 Visible-light-driven cleavage of the Cα–Cβ bond in cyclobutylamines and a related [4 + 2] cycloaddition reaction with olefins. | |
Despite having regioselectivity problems in the annulation of cyclopropylamines with internal π-unsaturated systems,15d in 2022, the Liu group reported a P/N-heteroleptic Cu(I)-photosensitizer-catalyzed regiospecific [3 + 2] annulation of cyclopropylamines with various functionalized alkynes, leading to the divergent construction of useful functionalized 3-aminocyclopentene derivatives including methylsulfonyl-, arylsulfonyl-, chloro-, ester- and trifluoromethyl-functionalized ones (Scheme 6).25 The authors disclosed that this cycloaddition reaction proceeds through the reductive quenching catalytic cycle rather than the oxidative quenching catalytic cycle based on preliminary mechanistic experiments and DFT calculations.
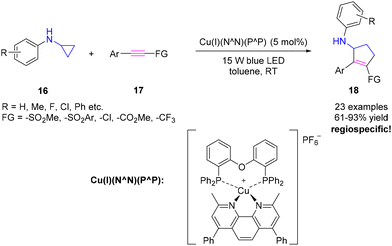 |
| Scheme 6 Visible-light-driven P/N-heteroleptic-Cu(I)-photosensitizer-catalyzed regiospecific [3 + 2] cycloaddition of cyclopropylamines with functionalized aryl alkynes. | |
Since Zheng's pioneering research work on the photocatalytic intermolecular [3 + 2] cycloaddition of cyclopropylamines with olefins to access pharmaceutically valuable cyclopentylamine derivatives,23 recently, several asymmetric variants for the synthesis of chiral cyclopentylamine derivatives have also been successfully achieved by using different platforms of photocatalysts and chiral catalysts (Scheme 7).26 For instance, in 2020, Ooi and co-workers for the first time reported a highly diastereo- and enantioselective [3 + 2] cycloaddition of N-cyclopropylureas with electron-neutral olefins enabled by a chiral ion-pair photocatalyst which comprises a polypyridyl Ir(III) cation and a weakly coordinating chiral borate anion (Scheme 7a).26a The key to inducing chirality relies on the use of urea as a redox-active anion-recognizable directing group and the iridium-chiral borate ion-pair [Ir(III)][B] as a photocatalyst. Later, the Ooi group further expanded this strategy for the asymmetric synthesis of five-membered alicyclic α-quaternary β-amino acids via the chiral [3 + 2] cycloaddition between cyclopropylureas and electron-deficient α-substituted acrylates (Scheme 7a).26b In 2020, the Huang and Jiang group realized an asymmetric [3 + 2] cycloaddition of cyclopropylamines with α-branched 2-vinylazaarenes to furnish chiral azaarene-substituted cyclopentanes enabled by cooperative DPZ photoredox catalysis and chiral Brϕnsted acid catalysis (Scheme 7b).26c The use of rigid and confined C2-symmetric imidodiphosphoric acid catalysts plays a key role in the achievement of high enantio- and diastereoselectivities in the transformations. Using similar cooperative photoredox and asymmetric H-bonding catalytic systems, in 2022, Jiang et al. disclosed a highly enantioselective [3 + 2] cycloaddition of N-arylcyclopropylamines with electron-rich or electron-neutral olefins to access several kinds of biologically important cyclopentylamine derivatives in high yields, ees and drs (Scheme 7c).26d Very recently, Zhao and Jiang et al. have also applied such cooperative photoredox and chiral H-bonding catalysis in the asymmetric [3 + 2] photocycloaddition of cyclopropylamines with electron-deficient olefins including 2-aryl acrylates/ketones and cyclic ketone-derived terminal olefins, leading to the synthesis of a range of pharmaceutically and biologically valuable chiral cyclopentylamine derivatives (Scheme 7d).26e In 2022, Fraile and Aleman et al. described an asymmetric synthesis of cyclic β-amino carbonyl derivatives by a formal [3 + 2] photocycloaddition between cyclopropylamine derivatives and coordinated α,β-unsaturated acyl imidazoles enabled by Meggers’ chiral Rh(III)-photocatalyst (Scheme 7e).26f The in situ formation of the substrate–catalyst complex accounts for both the photoredox process and the enantiocontrol task.
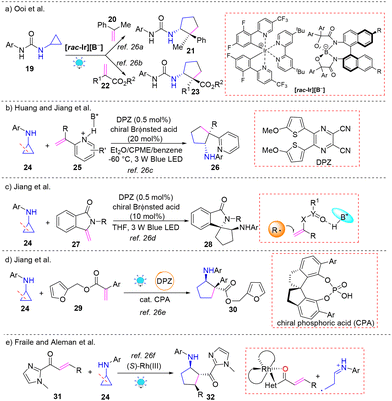 |
| Scheme 7 Several visible-light-mediated asymmetric variants of [3 + 2] cycloaddition of cyclopropylamine derivatives with olefins. | |
Despite much progress being made in the intermolecular [3 + 2] cycloadditions of cyclopropylamines with olefins,15a,d,23b,c,26 in sharp contrast, the intramolecular [3 + 2] cycloaddition variants have been underexplored. In 2019, Stephenson and co-workers reported a rare example of [Ir(dF(CF3)ppy)2(dtbbpy)]PF6-catalyzed intramolecular [3 + 2] photocycloaddition involving alkene-tethered aminocyclopropanes for the efficient and convenient synthesis of 1-aminonorbornanes, which can serve as useful aniline bioisosteres (Scheme 8).27 Mechanistically, the homolytic C–C bond cleavage in the amine radical cation intermediate triggers the consecutive 6-exo-trig and 5-exo-trig radical cyclizations to obtain the norbornane core structure. This strategy is one of the most effective and flexible methods for the preparation of 1-aminonorbornanes, which has advantages of excellent functional group tolerance and gram-scalable continuous-flow synthesis.
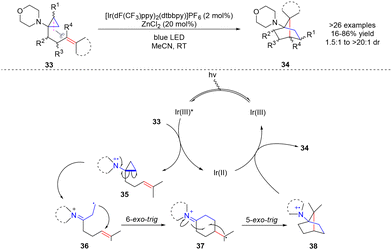 |
| Scheme 8 Synthesis of 1-aminonorborneol via photocatalytic intramolecular [3 + 2] annulation of alkene-tethered aminocyclopropanes. | |
In 2022, Stephenson's group further explored an intramolecular [3 + 2] photocycloaddition involving alkene-tethered cyclopropylsulfonamide derivatives for the convenient construction of a 2-azanorbornane scaffold enabled by an acridinium organophotocatalyst (Scheme 9).28 Similar to the above-mentioned protocol for the synthesis of 1-aminonorbornanes, this cycloaddition also initiates from the homolytic Cα–Cβ bond cleavage in the cyclopropylamine radical cation intermediate followed by an intramolecular 6-exo-trig radical cyclization to give the target products. Notably, the added Lewis acid plays an important role in facilitating the ring-opening of the cyclopropylamines.
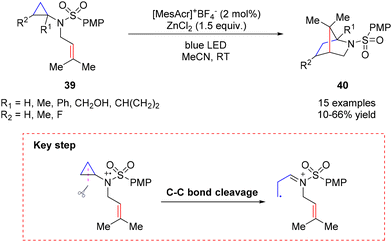 |
| Scheme 9 Synthesis of 2-azanorbornanes via photocatalytic intramolecular [3 + 2] annulation of alkene-tethered cyclopropylsulfonamides. | |
Besides being susceptible to [3 + 2] cycloaddition with π-type dipole systems, cyclopropylamines also allow for cycloaddition with σ-type dipole partners. In 2022, Molander et al. reported a photoinduced [3σ + 2σ] cycloaddition of cyclopropylamines with bicyclo[1.1.0]butanes to efficiently access trisubstituted bicyclo[2.1.1]heptanes, which may serve as useful meta-substituted arene bioisosteres, in a sustainable and straightforward manner (Scheme 10).29 In the radical cyclization process, the selective homolytic cleavage of the C1–C3 σ-bond in bicyclo[1.1.0]butanes is the key factor to guarantee the chemoselective formation of bicyclo[2.1.1]heptanes.
 |
| Scheme 10 The construction of aminobicyclo[3.1.1]heptanes via photocatalytic intermolecular [3σ + 2σ] cycloaddition of cyclopropylamines with bicyclo[1.1.0]butanes. | |
While extensive studies on the [3 + 2] photocycloaddition of cyclopropylamines with various 1,2-dipole partners to deliver five-membered cycles have been well documented, other manifolds of photocycloaddition to access six-membered cycles have been less achieved.30 In 2023, Yao and co-workers reported an Ir(III)-photocatalyst-induced cycloaddition of cyclopropylanilines with 2-alkenylarylisocyanides to access 3-aminotetrahydro-1H-carbazoles with high atom and step economy (Scheme 11a).30a In the transformation, 2-alkenylarylisocyanides serve as efficient radical acceptor platforms for the concomitant construction of both five- and six-membered rings. In the same year, Li and co-workers for the first time explored a radical-based [3 + 3] photocycloaddition of cyclopropylamines with N,N′-cyclic azomethine imines to access dinitrogen-fused six-membered cyclic compounds with high cis-diastereoselectivity by using Ir(dF(CF3)ppy)2(dtppy)PF6 as a photocatalyst (Scheme 11b).30b Mechanistically, the transformation undergoes a tandem process comprising the addition of β-carbon radical iminium ions to azomethine imines and sequential intramolecular 6-exo-trig cyclization. Very recently, Zhou and Li reported a 4CzIPN-catalyzed [3 + 3] annulation of cyclopropylamines and vinyldiazo compounds to deliver a diverse array of cyclohexenes bearing adjacent amino and carbonyl groups with broad functional group tolerance (Scheme 11c).30c In the process of cycloaddition, vinyldiazo compounds act as active 1,3-dipole partners.
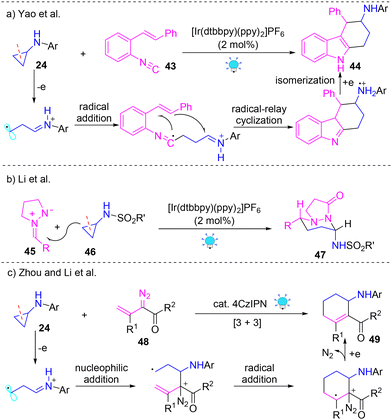 |
| Scheme 11 Photocatalytic intermolecular [3 + 3] annulations involving cyclopropylamines. | |
2.1.2 Amino radical cation species-induced cleavage of the Cα–Cβ bond in tertiary amines. N,N,N′,N′-Tetramethylethylenediamine (TMEDA) is a representative bidentate tertiary amine, which is widely used both as a ligand to coordinate with metal ions and as a catalyst in organic polymerizations.31 Upon SET oxidation by excited photocatalysts, TMEDA can also be easily converted into an amine radical cation. But unlike cyclopropylamines’ generation of distonic radical cations, the TMEDA radical cation is susceptible to splitting itself into a separated α-aminoalkyl radical and an iminium ion (Scheme 12). Both species are reactive intermediates and may undergo C–C or C–X (X = heteroatom) bond formation reactions. For instance, in 2012, the Li and Wang group designed a concept for the fragmentation of TMEDA 50 into iminium ion 52 and α-aminoalkyl radical 53 through a visible-light-driven C–C bond cleavage strategy.32 The resultant iminium ion and α-aminoalkyl radical could engage in aza-Henry condensations with nitroalkanes and radical photopolymerizations, respectively (Scheme 12a & b).
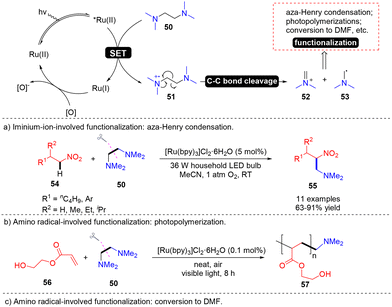 |
| Scheme 12 Visible light-mediated C–C bond cleavage in TMEDA. | |
In 2017, Opatz et al. reported a rare example of visible light-induced C–C-σ-bond metathesis reaction (Scheme 13).33 The transformation involves a complicated tandem process comprising the SET oxidation of tetrahydroisoquinoline derivatives 58 to yield amine radical cation intermediates 62, the generation of radical anions 65 from cyanopyridines 59 via the light-induced SET processes, and the final C–C-σ-bond metathesis between intermediates 62 and 65 to deliver α-aminonitriles 60 and pyridine derivatives 61. In the reaction, both the reactive intermediates arising from the amine radical cation 62, namely benzyl radical 64 and iminium ion 63, participate in the C–C-σ-bond metathesis processes.
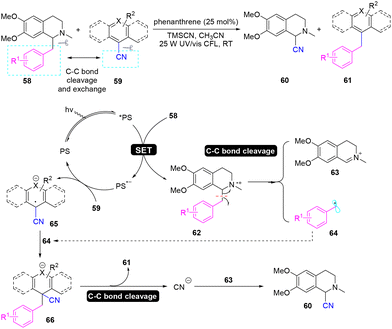 |
| Scheme 13 Visible light-mediated C–C-σ-bond metathesis reaction between tetrahydroisoquinolines and cyanopyridines. | |
In 2022, Koenigs, Patureau and co-workers reported a photocatalytic synthesis of gem-difluoroalkenes from aryl sulfonium salts 67 and α-(trifluoromethyl)styrenes 68 enabled by a [Ru(bpy)3](PF6)2/TMEDA catalytic system (Scheme 14).34 Mechanistically, TMEDA plays an important role in the whole reductive quenching catalytic cycle: first, TMEDA serves as an electron donor to quench the excited [Ru(bpy)3](PF6)2, resulting in the formation of a reductive Ru(I) species and an amine radical cation. Second, the α-aminoalkyl radical arising from the TMEDA radical cation is a key reductive agent for the conversion of radical intermediate 71 into anion intermediate 72. Finally, the iminium ion arising from the TMEDA radical cation acts as a fluorine acceptor to assist the conversion of 72 into the target products 69 via the release of the fluorine anion.
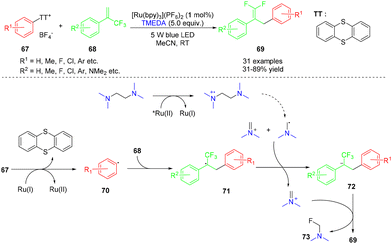 |
| Scheme 14 Photocatalytic synthesis of gem-difluoroalkenes from aryl sulfonium salts and α-(trifluoromethyl)styrenes under the catalysis of a [Ru(bpy)3](PF6)2/TMEDA system. | |
Since tertiary amine motifs are frequently encountered in N-containing compounds including natural products, it is reasonable to expect that the strategy of amine radical cation-induced C–C bond cleavage may have great potential for the construction of complex molecules. In 2014, Stephenson et al. showcased an example of the synthesis of valuable natural products including (–)-pseudotabersonine 78, (–)-pseudovincadifformine 79, and (+)-coronaridine 80 from commercially available catharanthine 74 through the visible-light photoredox-promoted C–C bond fragmentation of catharanthine radical cation 76 (Scheme 15).35 Compared with traditional thermal reactions for the C–C bond fragmentation of catharanthine, the photocatalysis approach has advantages of high yields, high chemoselectivity and mild reaction conditions.
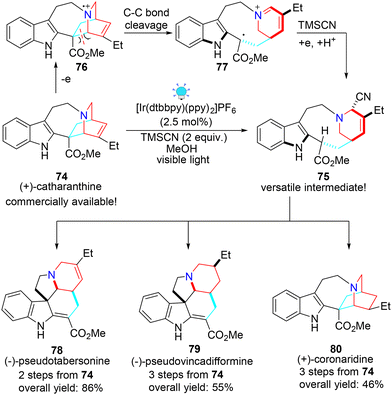 |
| Scheme 15 Photoredox catalysis enabling the synthesis of intermediate 75 from (+)-catharanthine and further conversion to alkaloids 78–80. | |
In 2021, Wang's group reported an interesting method for the diastereoselective construction of tetracyclic eight-membered lactams through a dearomative Heck reaction and a light-driven alkylative ring-opening of stable spiroindolines via C–C bond cleavage (Scheme 16).36 Mechanistic experiments showed that the spiroindolines can act as autocatalysts in the transformations under light irradiation. Mechanistically, the photoexcited spiroindoline 84 reduces perfluorobutyl iodide via a SET process to deliver perfluorobutyl radical 86 and spiroindoline radical cation 85. Subsequently, the addition of radical 86 to spiroindoline 81 may give radical intermediate 87. An SET oxidation of 87 by the previously generated spiroindoline radical cation 85 leads to the formation of cation intermediate 88 along with the regeneration of spiroindoline 81. 88 undergoes C–C bond cleavage via β-scission to give iminium ion 89, which is subsequently hydrolyzed by water to afford the desired products 83 (path a). Alternatively, although the authors did not mention it in their article, we speculate that the formation of a distonic radical iminium ion 91 (or 90) from spiroindoline radical cation 85 via a radical-induced β-fragmentation is also possible. The direct radical coupling between 91 and 86 may also deliver intermediate 89 (path b).
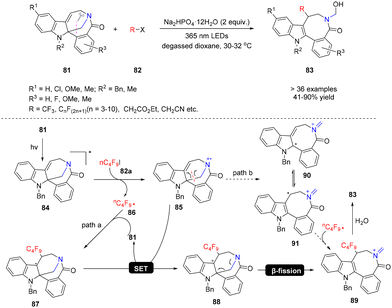 |
| Scheme 16 Photocatalytically diastereoselective synthesis of tetracyclic eight-membered lactams involving C–C bond cleavage. | |
Very recently, Dell'Amico and co-workers realized a novel photocatalytic [3 + 2] dipolar cycloaddition reaction of aziridines with various types of dipolarophiles to access pyrrolidines and dihydropyrroles with high chemo-, regio- and diastereoselectivities (Scheme 17).37 In this transformation, aziridine radical cation-induced Cα–Cβ bond cleavage and the subsequential photoreduction of the resultant distonic radical cation 98 play essential roles in the formation of the key azomethine ylide 99.
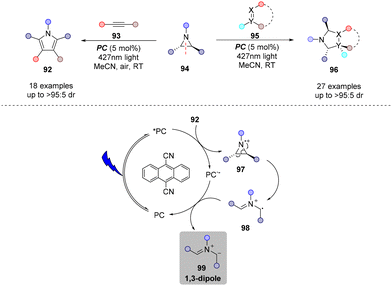 |
| Scheme 17 Photocatalytic [3 + 2] cycloaddition of aziridines and dipolarophiles involving C–C bond cleavage. | |
2.1.3 Amino radical cation species-induced cleavage of the Cα–Cβ bond in N-containing heterocycles. In 2022, the Protti and Fagnoni group reported a convenient method for the generation of alkyl radicals from 2-substituted oxazolidines via C–C bond cleavage under mild photocatalytic conditions (Scheme 18).38 A series of alkyl radicals including tertiary, α-oxy and α-amido radicals can be readily generated upon SET oxidation of 2-substituted oxazolidines to the amine radical cations by the excited [Acr-Mes]+[BF4]− photocatalyst. The resulting alkyl radicals can be easily trapped by electron-poor olefins or vinyl (hetero)aromatics.
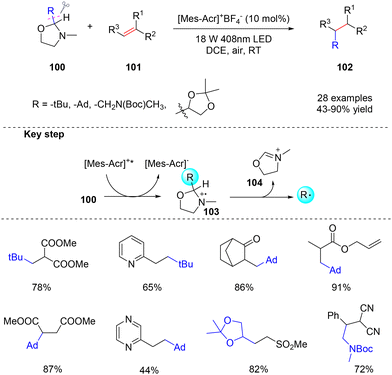 |
| Scheme 18 Visible light-mediated C–C bond cleavage in 2-substituted oxazolidines and subsequent application in alkylation reactions. | |
In the same year, Martin and co-workers described an elegant protocol for the generation of open-shell alkyl radical intermediates from dihydroquinazolinones through C–C bond cleavage initiated from the β-fragmentation of the amine radical cations enabled by 4CzIPN under photoredox catalysis conditions (Scheme 19).39 The resulting alkyl radicals can engage in cross-coupling reactions with aryl or alkyl bromides to efficiently forge (sp2)C–(sp3)C or (sp3)C–(sp3)C bonds enabled by a photoredox/nickel dual catalysis under mild and environmentally-friendly reaction conditions.
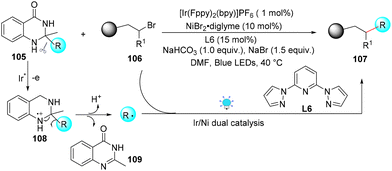 |
| Scheme 19 Photocatalytic synthesis of (sp2)C–(sp3)C or (sp3)C–(sp3)C bonds enabled by photoredox/nickel dual catalysis using dihydroquinazolinones as alkyl radical precursors. | |
2.2. Amino radical cation species-induced cleavage of Cγ–Cδ bonds
As well-designed alkyl radical precursors, alkyl-substituted Hantzsch esters are easy to undergo N-γ-fission under photocatalytic conditions to release alkyl radicals and stable pyridine derivatives.21 Since Tang's group first employed alkyl-substituted Hantzsch esters in the formation of C–C bonds that are difficult to achieve by other conventional methods (Scheme 20a),40 these amine derivatives have been widely applied in photocatalytic free-radical functionalization reactions. To date, a variety of C–C or C–X (X = heteroatom) formation reactions have been explored using alkyl-substituted Hantzsch esters as alkyl radical precursors under photocatalytic reaction conditions, including free radical substitution reactions,41 free radical addition reactions,42 photoredox/nickel dual catalyzed cross-coupling reactions,43 etc. (Scheme 20b). In 2017 and 2019, Cheng21a and Xiao21b well summarized the progress in the utilization of Hantzsch esters as radical alkylating reagents in photocatalytic reactions, respectively. After the publication of these reviews, the research studies on this domain were even more frequently documented in the literature.43c,44 Regarding the Hantzsch ester-involved photocatalytic alkylation reactions, the production of a stable aromatic pyridine ring acts as the driving force for facilitating the cleavage of the Cγ–Cδ bonds in Hantzsch ester radical cations in the presence of photocatalysts (Scheme 20b-I).21 It should be noted that in some reactions, Hantzsch esters can also directly absorb the energy of light and convert themselves into the excited state. The excited Hantzsch esters then undergo the homolytic cleavage of the Cγ–Cδ bonds and can also release alkyl free radicals even in the absence of a photocatalyst (Scheme 20b-II).45
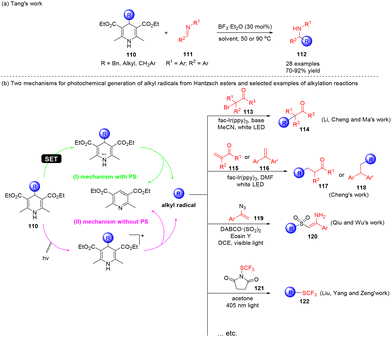 |
| Scheme 20 Pioneering and selected photocatalytic C–C bond formation reactions involving N-γ-fission of Hantzsch esters and two related mechanisms for the N-γ-fission. | |
Despite much advance in the utilization of alkyl-substituted Hantzsch esters in photocatalytic reactions, the asymmetric photocatalytic reactions involving Hantzsch esters as alkyl radical precursors have been underdeveloped.41c,44a–d In 2022, the Xiao group achieved a dynamic kinetic asymmetric transformation of racemic heterobiaryls to the corresponding axial chirality frameworks through an asymmetric radical trapping/cross-coupling reaction enabled by an asymmetric Ir/Ni metallaphotoredox dual catalysis system under visible light conditions (Scheme 21a).44a In the transformation, alkyl-substituted Hantzsch esters were used as the alkyl radical precursors. In 2023, Jiang and co-workers reported a novel and efficient method for the asymmetric synthesis of azarene-functionalized tertiary amines and α-amino acid derivatives via the enantioselective reductive coupling of azarene-substituted E/Z-ketimine mixtures with 1,4-hydropyridines enabled by the cooperative photoredox and chiral Brϕnsted acid catalysis (Scheme 21b).44b Very recently, Lu's group realized an enantioconvergent cross-coupling reaction of 1,4-dihydropyridine derivatives with aryl/alkenyl halides via a photoredox/nickel dual catalysis (Scheme 21c).44c The reaction has advantages of good efficiency, high enantioselectivity and mild reaction conditions. This strategy can not only be used for the synthesis and modifications of drug molecules and natural products, but also for the one-pot construction of molecules with multiple stereocenters. In the same year, the You group realized a dual photoredox/Rh-catalyzed asymmetric allylic substitution reaction between 4-alkyl-1,4-dihydropyridines and allyl acetates under visible light conditions, resulting in the formation of alkylation products in moderate to good yields with good to excellent enantioselectivity (Scheme 21d).44d
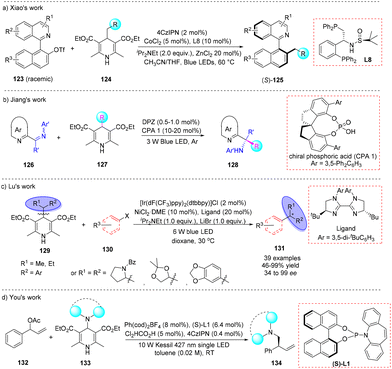 |
| Scheme 21 Photocatalytic asymmetric transformations involving alkyl-substituted Hantzsch esters as alkyl radical precursors. | |
Similarly, carbonyl-substituted Hantzsch esters,46 as analogues of alkyl-substituted Hantzsch esters, have been explored and serve as promising carbonyl radical precursors upon their Cγ–Cδ bond cleavage under photoredox catalytic conditions (Scheme 22). Thus, a variety of carbonyl-functionalization-related photocatalytic reactions have been achieved by harnessing carbonyl-substituted Hantzsch esters as carbonyl radical equivalents in recent years.
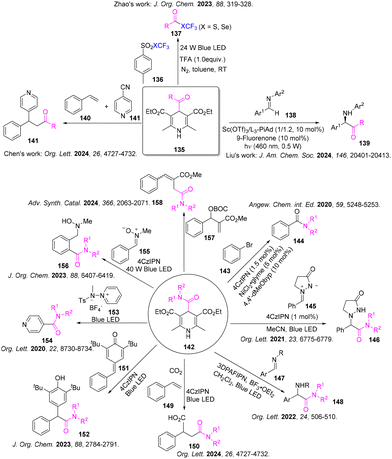 |
| Scheme 22 Photocatalytic transformations involving carbonyl-substituted Hantzsch esters as carbonyl radical precursors. | |
2.3. α-Aminoalkyl radical species-induced cleavage of Cβ–Cγ bonds
Upon SET oxidation by excited photocatalysts, tertiary amines are susceptible to conversion into α-aminoalkyl radicals via elimination of a proton from amine radical cations.19 The resultant α-aminoalkyl radicals can either undergo radical-induced Cα–N bond cleavage to form an alkyl radical and an imine species (Scheme 3d, vide supra; also see section 3.3, vide infra) or undergo radical-induced Cβ–Cγ bond cleavage to generate an alkyl radical and an enamine species (Scheme 2c, vide supra). While the former scenario of β-fragmentation leading to the Cα–N bond scission has been reported for many years,47,60–62 the latter scenario of β-fragmentation resulting in the Cβ–Cγ bond cleavage has not been discovered until recently.48 In 2024, almost at the same time, Meng's group48a and Yu's group48b for the first time independently discovered such a reactivity associated with an in situ generated α-aminoalkyl radical intermediate which is the key factor for the tertiary amine-assisted deconstructive carboxylation of alkenes with carbon dioxide under visible light photoredox conditions (Scheme 23). In this transformation, the tertiary amine N,N-dicyclohexylmethylamine is first oxidized into an α-aminoalkyl radical by the excited photocatalyst 4DPAIPN (1,3-dicyano-2,4,5,6-tetrakis(diphenylamino)-benzene) (or 3DPA2FBN (2,4,6-tris(diphenylamino)-3,5-difluorobenzonitrile) using Yu's procedure) via an SET oxidation process followed by the release of a proton. The addition of the α-aminoalkyl radical 162 across alkenes generates radical intermediate 163. An SET reduction of 163 by the reductive species PS˙− followed by carbonylation with CO2 and protonation affords γ-amino acid 165. Upon SET oxidation, tertiary amine 165 may generate another type of α-aminoalkyl radical 166. The radical-induced β-scission of the Cβ–Cγ bond in 166 results in the formation of radical intermediate 168 and enamine species 167. The SET reduction of 168 by the reductive species PS˙− followed by protonation gives the final carboxylic acid 160. It is worth noting that the present destructive carboxylation of carbon–carbon double bonds provides a unique approach for accessing carboxylic acids, which has advantages of easily available starting materials, a broad substrate scope, convenient application in the late-stage carboxylation of bioactive molecule derivatives, and mild reaction conditions.
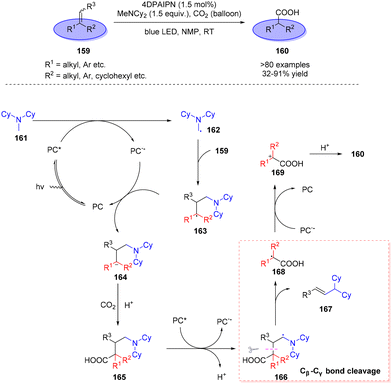 |
| Scheme 23 Photocatalytic deconstructive carboxylation of activated olefins involving α-amino radical-induced C C bond cleavage. | |
In the same year, by adopting a similar “α-aminoalkyl radical-induced site-selective cleavage of the Cβ–Cγ bond” strategy, the Liu group achieved an interesting demethylenative en-yne radical cyclization of 1,7-enynes to chemoselectively afford 3,4-dihydroquinolin-2-ones or quinolin-2-ones through a conceptually novel olefin-radical metathesis-type reaction enabled by P/N-heteroleptic Cu(I)-photocatalysts under photoredox catalysis conditions (Scheme 24).48c To realize the olefin-radical metathesis process and the demethylenative en-yne cyclization, they proposed a protocol termed the “radical-lending/methylene-returning” strategy by harnessing an α-aminoalkyl radical as a suitable radical exchange partner with olefin. The detailed mechanism of this transformation is illustrated in Scheme 24. First, an α-aminoalkyl radical 174 can be in situ generated from Et3N upon SET oxidation by the excited Cu(I)-photocatalyst. The addition of 174 across the terminal olefin unit of 1,7-enyne 170a is followed by intramolecular radical cyclization to deliver radical intermediate 176 (the radical lending process). Then 176 undergoes a 1,5-HAT process to generate a new α-aminoalkyl radical 177. After that, 177 undergoes a site-selective cleavage of the original (sp2)C–(sp2)C bond of the terminal olefin moiety to generate radical 179 (or 180) and release a molecule of enamine 178 (the methylene returning process). At this stage, as a net result, the formal olefin-α-aminoalkyl radical metathesis process is successfully achieved. Notably, the produced enamine species could be successfully detected and confirmed by high-resolution mass spectrometry (HRMS), supporting the above-mentioned olefin-radical exchange process. As for subsequent transformations, 179 (or 180) is reduced by the reductive Cu(0) species to give anion intermediate 181 (or 182) and regenerate the Cu(I)-photocatalyst. Finally, either the protonation and the subsequent 1,3-allylic isomerization of 181 or the direct protonation of 182 affords quinolin-2-one-type product 171a. Interestingly, under the catalysis of Cu1 having a more strongly reductive capability than Cu2, 171a can be further converted to 172a through a PCET-involved reductive pathway. Later, the same group further disclosed that an in situ generated α-aminoalkyl radical 188 from propionaldehyde 185 and dipropylamine 186 in the presence of Hantzsch ester 187 and Cu(I)-photocatalyst Cu1 can also efficiently act as a traceless initiator for the demethylenative cyclization of 1,7-enynes to access quinoline-2-ones under mild conditions.48d It is worth noting that the use of different α-aminoalkyl radicals in these two protocols leads to a big difference in the substrate scope. Thus, in the case of α-aminoalkyl radical 174 as the reaction initiator, the 1,7-enyne scope will be limited to those having no substituents at the α-position to the carbonyl group;48c whereas in the case of α-aminoalkyl radical 188 as the reaction initiator, either 2-substituted- or 2-substituent-free-1,7-enynes are viable for the demethylenative en-yne cyclizations.48d
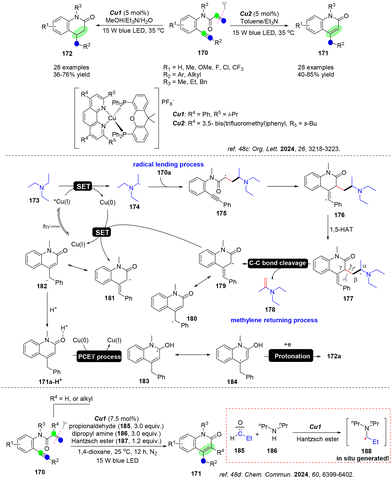 |
| Scheme 24 Cu(I)-photosensitizer-controlled chemoselective olefin-α-aminoalkyl radical metathesis/demethylenative cyclization of 1,7-enynes. | |
Shortly after the Liu group published the above findings, Singh's group48e and Li's group48f reported similar research studies on the photocatalytic, α-aminoalkyl radical-triggered, demethylenative en-yne cyclizations (Scheme 25). In Singh's research work, Ru(bpy)3Cl2·6H2O was employed as the photocatalyst and the α-aminoalkyl radical 188 derived from N,N-dimethylaniline 187 was used as the initiator for the ring-closing transformations. In addition, they further expanded their protocol for the demethylenative cyclization of o-cyano acrylamides to access carbostyril derivatives in good yields (Scheme 25a). In Li's research findings, they achieved divergent demethylenative ring-closing of 1,7-enynes under photoredox catalysis conditions depending on different α-aminoalkyl radical promoters and different structural variants of 1,7-enynes (Scheme 25b). Thus, when the α-aminoalkyl radical derived from dicyclohexylmethylamine (MeCy2) is used as the promoter under the catalysis of an Ir(III)-photocatalyst, type-I and type-II 1,7-enynes afford nonspirocyclic 192 and spirotricyclic quinolin-2-one derivatives 193 as the major products, respectively. Besides, when the α-aminoalkyl radical derived from TMEDA is used, type-III 1,7-enynes deliver spirotetracyclic quinoline-2-one derivatives 194 as the predominant products.
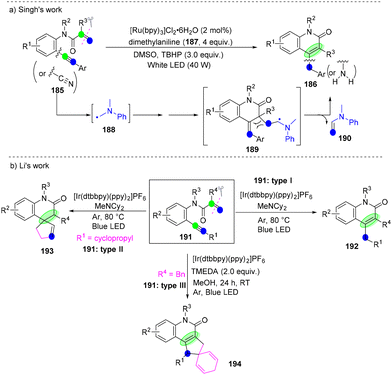 |
| Scheme 25 Photocatalytic deconstructive cyclizations of 1,7-enynes and/or o-cyano acrylamides using α-aminoalkyl radicals as traceless initiators. | |
2.4 α-Aminoalkyl radical species-induced cleavage of Cα–Cβ bonds in the presence of O2
In 2017, the Wang group reported a visible-light-induced oxidative formylation of N-alkyl-N-(prop-2-yn-1-yl)anilines with O2 involving C–C bond cleavage (Scheme 26a).49 Mechanistic studies disclosed that the in situ generated peroxide intermediate from α-aminoalkyl radical species and O2 plays a key role in achieving the Cα–Cβ bond cleavage. In the same year, they also realized oxidative formylation of N-methyl substituted anilines with O2 to access formamides under the irradiation of visible light (Scheme 26b).
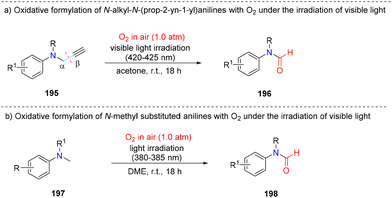 |
| Scheme 26 Visible-light-induced oxidative formylation of N-alkyl-N-(prop-2-yn-1-yl)anilines and N-methyl substituted anilines. | |
3. Photocatalytic cleavage of C–N bonds
The C–N bond is ubiquitous in organic molecules. A large number of C–N bond-containing compounds show great potential for serving as nitrogen and/or carbon sources in organic synthesis upon cleavage of C–N bonds. Nevertheless, the cleavage of C–N bonds5 is not an easy task because their dissociation energies are very high and they are less polarized than C–X (X = Cl, Br, I etc.) bonds.3 In the past few decades, despite the great progress that has been made in the transition-metal-catalyzed cleavage of the C–N single bond under thermal conditions,5 yet there remain huge challenges in this domain and there is a need to search for novel and highly efficient strategies for pursuing these tasks. With the flourishing of visible light-driven organic photochemical synthesis in recent years,14 the utilization of visible light-induced photocatalytic strategies for the cleavage of C–N bonds has received increasing attention because such photocatalytic transformations have special advantages of mild reaction conditions, unique selectivity and sustainable processes. Herein, we introduce the recent advances in the cleavage of unactivated C–N bonds associated with amines and their derivatives via visible-light-driven photocatalytic pathways.
3.1. Cleavage of C–N bonds in tertiary amines via iminium salts
Tertiary amines represent a class of important and useful feedstocks as well as synthetic intermediates in organic synthesis. Upon C–N bond cleavage, they can be fragmented into two parts, namely the carbon-linked fragment and the nitrogen-linked fragment, both of which may serve as useful synthetic building blocks in organic synthesis. In the past few decades, methods involving the transition-metal-catalyzed oxidative cleavage of C–N bonds in tertiary amines via iminium ions have received much attention in organic synthesis due to the convenient generation of secondary amines and carbonyl synthons via the hydrolysis of iminium ions.9 Nevertheless, under thermal reaction conditions, such procedures generally suffered from harsh reaction conditions. With the rapid development of visible-light-mediated photocatalysis, photoinduced two electron oxidation of tertiary amines into iminium ions has evolved into a powerful and sustainable strategy for the fragmentation of tertiary amines into useful amine and/or carbonyl synthons.
In 2014, Li and co-workers reported a Rose bengal-promoted indole C-3 formylation reaction under aerobic conditions using TMEDA as the one-carbon source through C–N bond cleavage (Scheme 27a).50 In the tandem processes for the formation of 3-indolyl formaldehydes, the photoredox-induced successive generation of iminium ion species 201 and 203 plays a key role in the whole transformation. In 2015, Cho's group developed a photocatalytic method for the oxidative C–N bond cleavage in amines to access carbonyl compounds along with NH3 or amines as byproducts under the catalysis of Ru(byp)3Cl2 (Scheme 27b).51 However, rather than an iminium ion-involved mechanism, in their proposed one, the nucleophilic addition of water to the photoredox-generated imine species followed by an elimination of the amine byproduct accounts for the formation of carbonyl compounds. Notably, this protocol also permits the removal of a p-methoxybenzyl (PMB) group from N-PMB protected amides 207 via the photocatalytic C–N bond cleavage.
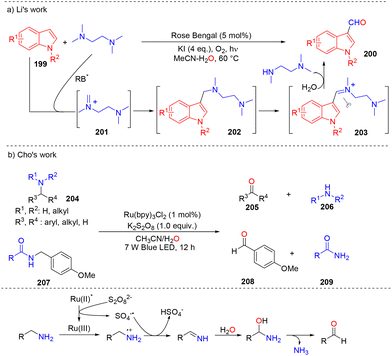 |
| Scheme 27 Photocatalytic deconstructive cyclizations of 1,7-enynes and/or o-cyano acrylamides using α-aminoalkyl radicals as traceless initiators. | |
In 2022, Xie's group achieved a visible-light-mediated amidation reaction between carboxylic acids and tertiary amines via C–N bond cleavage in the presence of pentafluoropyridine under the catalysis of [Ir(dF(CF3)ppy)2(dtbbpy)]PF6 (Scheme 28).52 In this transformation, pentafluoropyridine acts as an activating agent for the generation of acyl radical intermediate 215 from carboxylic acids 210, and tertiary amines 211 serve as the source of secondary amines 218 through the hydrolysis of self-derived iminium ions 217 under photoredox catalysis conditions. The nucleophilic addition of secondary amines 218 to acyl radicals 215 followed by a SET oxidation process delivers the target products tertiary amides 212. The present protocol exhibits a broad substrate scope of carboxylic acids and good functional group tolerance.
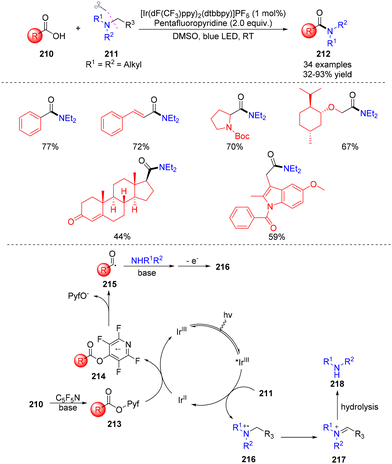 |
| Scheme 28 Visible-light-mediated photocatalytic amidation from carboxylic acids and tertiary amines involving C–N bond cleavage. | |
In 2023, Rovis and co-workers reported a photoinduced nickel-catalyzed N-demethylation of tertiary amines with high chemoselectivity by harnessing C(sp2)–bromides as HAT reagents (Scheme 29).53 Mechanistic studies disclosed that after the Ni(0) oxidative addition to the C(sp2)–Br bond, a photoinduced homolysis of the C(sp2)–Ni bond (224) occurs to produce aryl radical intermediate 225. A hydrogen-atom transfer (HAT) process between 225 and tertiary amines 219 proceeds to deliver α-aminomethyl radical 226. 226 can be intercepted by Ni(I)-OPiv to obtain an Ni(II) intermediate 227, which is in equilibrium with the iminium ion 228. Finally, the cleavage of the C–N bond occurs upon the hydrolysis of the iminium ion 228, leading to the formation of N-demethylation product 221 and formaldehyde. The advantages of this photoinduced nickel-catalyzed N-demethylation protocol lie in chemoselective demethylation, excellent functional group compatibility, and mild reaction conditions.
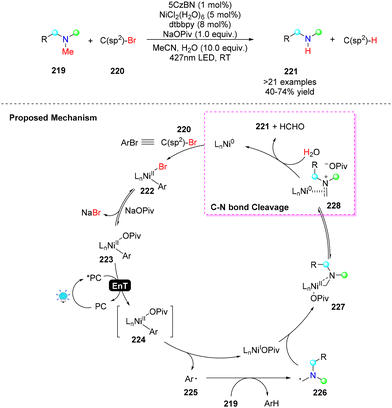 |
| Scheme 29 Selective N-demethylation of trialkylamines using C(sp2)–bromides as HAT reagents enabled by photoredox/nickel dual catalysis. | |
Apart from the precursors of secondary amines, tertiary amines can also serve as the precursors of carbonyl synthons upon C–N bond cleavage via iminium ions. In 2024, Senadi and Arumugam reported a three-component approach for the construction of 2,4-difunctionalized quinazolines from o-acylanilines, trialkylamines and ammonium chloride catalyzed by eosin Y under visible-light photoredox catalysis conditions, wherein tertiary amines serve as the source of alkyl aldehydes for the condensation with o-acylanilines (Scheme 30).54 Notably, the practicability of this method has been further used in the synthesis of 2-hydroxymethyl quinazolines by using easily available triethanolamine as a C2-OH source.
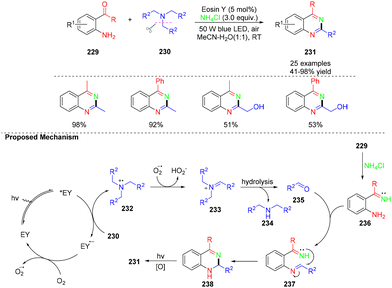 |
| Scheme 30 Photocatalytic synthesis of quinazolines from tertiary amines via C–N bond cleavage. | |
3.2. Cleavage of C–N bonds via amine radical cation species
In 2021, Sekine and Kuninobu reported a photoinduced deaminative borylation of inactive aniline derivatives with bis(pinacolato)diboron (B2Pin2) in the presence of CO2 under the catalysis of pyrene (Scheme 31a).55 According to the proposed mechanism, the reaction proceeds through a tandem process including the formation of a complex of the arylamino radical cation with B2Pin2 (243), the concerted C–N/B–B bond metathesis of 243 leading to the formation of borylated products 241 and aminoboryl radical cations 244, and the final formation of aminoborane byproduct 242 via an SET reduction event. Later, Zhang's group reported a different strategy to activate the C–N bond of non-reactive aniline derivatives and achieved a photoinduced aerobic borylation of various tertiary and secondary anilines (Scheme 31b).56 Mechanistic studies showed that the active singlet oxygen 1O2 reacts rapidly with aniline 245 to obtain an aniline cation radical 248 and O2˙−. Then the cation radical 248 reacts with B2pin2 via C–N/B–B bond metathesis to obtain aryl boronate ester product 246 along with aminoboronate ester cation radical 249. Finally, a single electron transfer from O2˙− to 249 delivers aminoboronate ester 247.
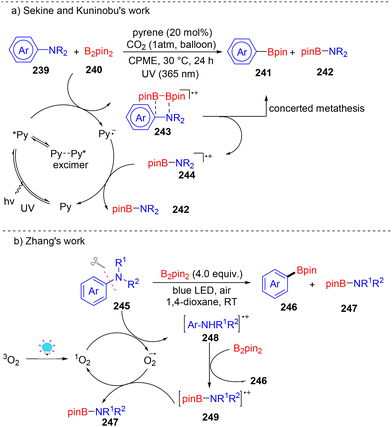 |
| Scheme 31 Photocatalytic C–N/B–B bond metathesis reactions for the construction of aryl boronate esters: Sekine and Kuninobu's work vs. Zhang's work. | |
In 2023, Zou et al. reported a photochemical reductive deamination of α-amino aryl alkyl ketones to access the corresponding ketone products (Scheme 32).57 Preliminary mechanistic studies disclosed that the reaction possibly proceeds through a key amino radical cation-induced C–N bond cleavage pathway, leading to the formation of ketone products and hydroxyamine byproducts (path a). Alternatively, a pathway involving an α-aminoalkyl radical intermediate-induced β-fragmentation to liberate a molecule of imine is also possible (path b).
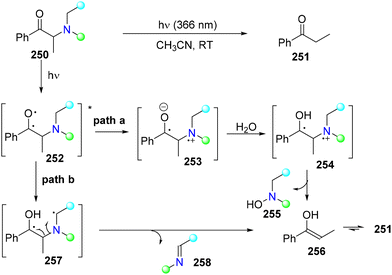 |
| Scheme 32 Photoinduced reductive deamination of α-amino aryl alkyl ketones. | |
3.3. Cleavage of C–N bonds via amine cation species
In 2021, Yang et al. developed a facile and practical protocol for the direct methylation of N-heteroarenes by using TMEDA as a methyl source through a photoredox-catalyzed deamination process (Scheme 33a).58 The mechanism studies revealed that an amine cation species-induced amine-elimination event is the key step to enable TMEDA as a C1 synthon for the C–H bond methylation. In 2024, Fan et al. reported a method for the photocatalytic synthesis of 2,3-diamines from anilines and DIPEA in the presence of aromatic aldehydes through C–N bond cleavage and C–C bond formation (Scheme 33b).59 They subtly employed DIPEA as a C2 synthon to participate in the reassembly with anilines to access 2,3-diamines. Mechanistically, the photo-oxidation of DIPEA in conjunction with the aromatic aldehyde-assisted PCET (proton-coupled electron transfer) process generates iminium ion species 272. The nucleophilic addition of anilines 265 to iminium 272 affords amine cation tautomers 273 and 274, which may undergo a C–N bond cleavage event via the elimination of a molecule of diisopropylamine, leading to the formation of another type of iminium ion 275. Finally, photoreduction of 275 followed by the dimerization of the resultant α-aminoalkyl radical 276 gives the final products 267. It should be noted that under the photoredox catalysis conditions, aromatic aldehydes can be converted into 1,2-diol products 271.
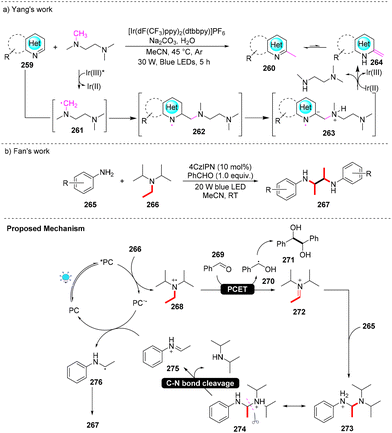 |
| Scheme 33 Visible light-induced C–N bond cleavage from amine cation species: Yang's work and Fan's work. | |
In the same year, Gnaprakasam et al. successfully utilized tertiary amines as alkyl synthons via C–N bond cleavage to synthesize biologically active tetraketones under visible-light photocatalytic conditions (Scheme 34).60 Similar to Fan's protocol,59 the key to the implementation of this strategy lies in the formation of iminium ion 280 and the deamination process of intermediate 281.
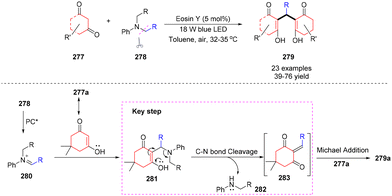 |
| Scheme 34 Tertiary amines acting as carbon sources for the intermolecular coupling with 1,3-diketones enabled by Eosin Y under photoredox catalysis conditions. | |
3.4. Cleavage of C–N bonds via α- or β-aminoalkyl radical species
Upon the single-electron oxidation by photocatalysts, tertiary amines are easily converted into amine radical cations which subsequently undergo deprotonation of the C–H bond adjacent to the N-atom to generate α-aminoalkyl radicals and release a proton.19 The generated α-aminoalkyl radicals serve as useful reactive intermediates in a broad range of photocatalytic reactions, typically acting as nucleophilic radical species in radical addition, substitution, and cross-coupling reactions.19 In most cases, α-aminoalkyl radicals do not undergo a C–N bond cleavage event. Yet in recent several years, photocatalytic transformations that involve a C–N bond cleavage event associated with reactive α-aminoalkyl radical intermediates have been gradually achieved. In 2020, the Liu group reported a P/N heteroleptic Cu(I)-photosensitizer-catalyzed deoxygenative radical alkylation of aryl alkynes with alkyl aldehydes to access allylarenes by using dipropylamine as a traceless linker agent (Scheme 35).61 In the tandem radical process, an α-aminoalkyl radical 290 is first in situ generated from alkyl aldehyde and dipropylamine in the presence of a P/N-heteroleptic Cu(I)-photocatalyst and Hantzsch ester. Then the regioselective addition of α-aminoalkyl radical 290 across aryl alkyne 284 yields radical intermediate 291. 291 undergoes 1,5-HAT to generate another α-aminoalkyl radical-type intermediate 292, which subsequently undergoes a radical-induced β-fragmentation of the N–Cα bond to deliver allylic radical 294 and imine 293. Finally, 1,3-allylic isomerization of 294 to 295 followed by a HAT process from dipropylamine radical cation 297 and/or water delivers the final products 288. As a net result, this protocol provides a unique way to utilize commercially available alkyl aldehydes as deoxygenative-type alkyl radical equivalents without the occurrence of decarbonylation. In the whole transformation, dipropylamine serves as a traceless linker agent for the cross-coupling of aryl alkynes and alkyl aldehydes.
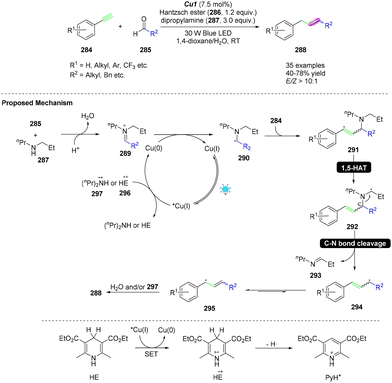 |
| Scheme 35 Alkyl aldehydes acting as deoxygenative alkyl radical equivalents for the coupling with aryl alkynes using secondary amines as traceless linker agents enabled by P/N–Cu(I)-photosensitizers under visible-light photoredox catalysis conditions. | |
On the basis of the above mechanistic profile and the fact that α-aminoalkyl radicals can be directly generated from tertiary amines through SET oxidation processes, Liu's group subsequently realized an efficient method for the synthesis of allylarenes directly from tertiary amines and aryl alkynes enabled by a similar P/N-heteroleptic Cu(I)-photosensitizer (Scheme 36).62 In these transformations, tertiary amines play dual roles as quenching agents for the excited Cu(I)-photocatalyst and alkylating equivalents for the cross-coupling with alkynes. This is the first endeavour to employ tertiary amines as deaminative alkyl radical equivalents in photocatalytic reactions.
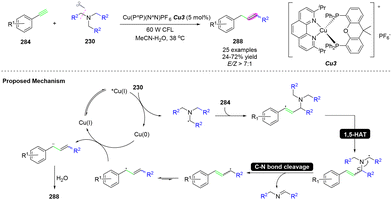 |
| Scheme 36 Tertiary amines acting as deaminative alkyl radical equivalents for the alkylation of aryl alkynes enabled by P/N-heteroleptic Cu(I)-photosensitizers. | |
After successfully using non-chiral alkyl aldehydes as deoxygenative alkyl radical equivalents in the alkylation with aryl alkynes,61 in 2023, the Liu group further expanded their protocol to harness α-chiral alkyl aldehydes as deoxygenative chiral alkyl radical equivalents as showcased in a 4CzIPN-catalyzed deoxygenative alkylation/cyclization of 2-biphenylisonitriles to access chiral 6-alkyl-substituted phenanthridines by using secondary amines as traceless linker agents (Scheme 37).63 Notably, in most cases, the ee values of the employed chiral α-amino aldehydes remain at high levels of retention. Mechanistically, the in situ generated α-amine radical 302 undergoes radical addition across 2-biphenylisonitriles 296 to obtain imine radical intermediate 303 which undergoes intramolecular homolytic aromatic substitution (HAS) to yield radical species 304. An SET oxidation of 304 followed by the release of a proton delivers phenanthridinyl-tethered tertiary amine 305. Upon SET oxidation and release of a proton, 305 may be converted into an α-amino radical 307, which further undergoes β-fragmentation of the N–Cα bond to deliver radical intermediate 309 along with imine species 308. Finally, 309 abstracts a hydride from HE˙+ to afford the target products 300a.
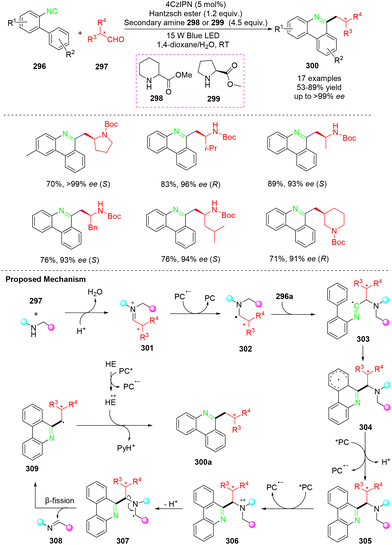 |
| Scheme 37 α-Chiral alkyl aldehydes acting as deoxygenative chiral alkyl radical equivalents for the alkylation/cyclization of 2-biphenylisonitriles enabled by 4CzIPN using secondary amines as traceless linker agents. | |
In the same year, Kang and co-workers also realized an α-aminoalkyl radical-involved deaminative alkylation/cyclization of 2-biphenylisonitriles to access 6-alkyl-substituted phenanthridines under photoredox catalysis conditions (Scheme 38).64 In Kang's protocol, tertiary amines were employed as the sources of α-aminoalkyl radicals and a super organophotoreductant CBZ6 was used as the photocatalyst. Based on preliminary mechanistic experiments, the authors proposed a possible mechanism for the deaminative alkylation/cyclization reaction. According to their proposed mechanism, the key step for the C–N bond cleavage does not involve an event comprising an α-aminoalkyl radical intermediate and the liberation of imine, as reported in Liu's protocol,63 but involves an event comprising a β-aminoalkyl radical intermediate (or an α-amino anion intermediate) and the release of a secondary amine.
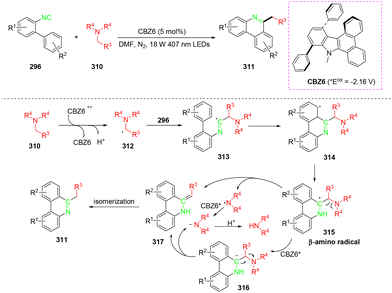 |
| Scheme 38 Photocatalytic alkylation/cyclization of 2-biphenylisonitriles by tertiary amines via C–N bond cleavage enabled by the super organophotoreductant CBZ6. | |
Very recently, the Ota and Yamaguchi group achieved a site-selective C–N bond cleavage in unstrained pyrrolidines enabled by a combination of Lewis acid and photoredox catalysis (Scheme 39).65 In this photocatalytic transformation, photocatalytic SET reduction of the Lewis acid-activated amide produces an α-amino radical intermediate which undergoes the key Cα–N bond cleavage.
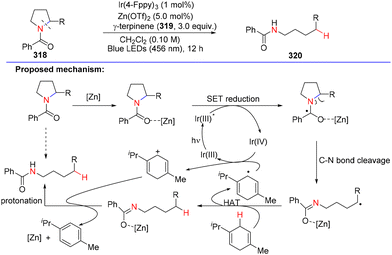 |
| Scheme 39 Site-selective C–N bond cleavage in unstrained pyrrolidines enabled by the combination of Lewis acid and photoredox catalysis. | |
In 2018, the Stephenson group reported a visible-light-mediated 1,4-aryl migration reaction of haloalkylamides to access aminoalkylated heteroarenes involving a homolytic C–N bond cleavage event (Scheme 40).66 According to their proposed mechanism, the photocatalytically generated spiro-β-amino radical intermediate 325 undergoes the key C–N bond cleavage process to accomplish 1,4-aryl migration.
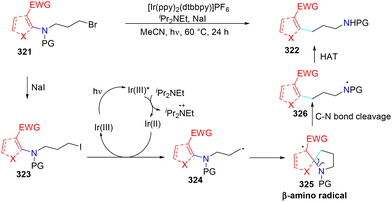 |
| Scheme 40 Photocatalytic C–N bond cleavage enabling 1,4-aryl migration in heteroarylamines. | |
3.5. Cleavage of C–N bonds via miscellaneous other ways
3.5.1 Cleavage of C–N bonds in redox-active imines. Primary amines play an important role in organic synthesis due to their easy availability and versatile reactivity. Among various strategies for using primary amines as valuable synthons, the conversion of primary amines into Katritzky salts10 is an elegant protocol that enables them to act as useful alkyl radical equivalents via C–N bond cleavage under suitable reductive conditions, certainly including visible-light photoredox catalysis conditions. Despite much advance in the application of Katritzky salts in organic synthesis, their synthesis is limited to α-1° and α-2° amines, whereas α-3° primary amines are not viable for their conversion into the corresponding Katritzky salts due to their steric hindrance. On the other hand, the harnessing of Katritzky salts as alkyl radical sources generally requires a reductive event. To circumvent these problems, in 2020, Rovis and co-workers proposed an elegant synthetic platform that can enable α-3° primary amines to act as alkyl radical equivalents through the photochemical oxidation of redox-active imines (RAIs) arising from the condensation of α-3° primary amines with trimethoxybenzaldehyde (Scheme 41).67 Mechanistically, the single-electron oxidation of the electron-rich phenyl ring in RAIs, followed by a deprotonation of imidoyl-H and a spin-centered-shift (SCS) event, leads to the formation of a key imidoyl radical species 333. The subsequent β-scission of imidoyl radical 333 leads to the C–N bond cleavage and the release of a nucleophilic alkyl radical 334, which can further be trapped by electron-deficient olefins. Rovis’ protocol provides an ideal solution for the employment of α-3° primary amines as alkyl radical equivalents, something that is not feasible with Katritzky salts, which will arouse great interest in the field of organic synthesis.
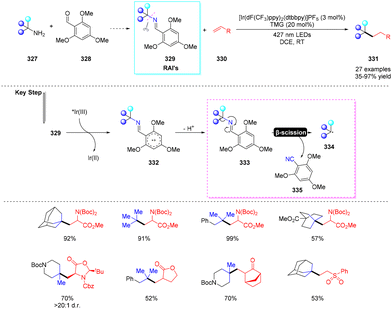 |
| Scheme 41 RAIs as deaminative 3°-alkyl radical equivalents enabled by visible light photoredox catalysis. | |
In 2021, Rovis and coworkers further expanded their protocol for the achievement of a dual nickel/photoredox-catalyzed deaminative cross-coupling of sterically encumbered primary amines with aryl halides to forge quaternary center-containing (sp3)C–(sp2)C bonds through the conversion of primary amines into RAIs (Scheme 42).68 The reaction exhibits broad substrate compatibility for both amines and aryl halides and can also be applicable in the construction of medicinally relevant scaffolds, e.g., for the synthesis of ALK2 inhibitors.
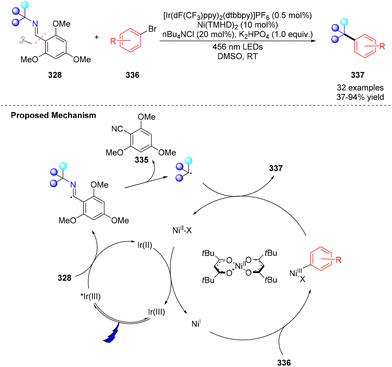 |
| Scheme 42 Deaminative cross-coupling of RAIs with aryl halides enabled by a nickel/photoredox dual catalysis. | |
Very recently, the same group described a method for the efficient generation of tertiary carbanions from primary amines via a photoredox-catalyzed deaminative radical-polar crossover strategy.69 The resultant tertiary carbanions are versatile nucleophiles that can be trapped by a series of electrophiles including aldehydes, ketones, imines, Michael acceptors, and H2O/D2O. As a net result, the redox-active imines (RAIs) derived from primary amines and trimethoxybenzaldehyde can be regarded as alkyl carbanion equivalents under the reported photoredox catalysis conditions.
Inspired by Rovis’ research studies, in 2022, Huang and Rueping achieved a regiodivergent cross-coupling alkylation of aryl halides with Rovis’ redox-active imines enabled by a photoredox/nickel dual catalysis.70 It is worth noting that in their protocol, an organophotocatalyst 4CzIPN exhibits better catalytic efficiency than the Ir(III)-based photocatalysts used in Rovis’ protocols.67–69 In the same year, Liu's group achieved a photocatalytic deaminative alkylation of DNA-tagged alkenes with tertiary amine-derived imines as radical precursors;71 the Liang group reported a visible-light-induced deaminative alkylation of trifluoromethyl-substituted alkenes to access gem-difluoroalkenes by using Rovis’ alkylation protocol.72 In 2023, Chen and co-workers described a similar photocatalytic transformation to Liang's work for the synthesis of gem-difluoroalkenes, although by using 4CzIPN as a photocatalyst.73
3.5.2 Cleavage of C–N bonds in isonitriles. As mentioned in 3.5.1, the conversion of α-1° and α-2° primary amines into Katritzky salts can enable them to act as deaminative alkyl radical equivalents,10 whereas the transformation of α-3° primary amines into RAIs can allow them to serve as deaminative tertiary alkyl radical equivalents.67–73 Despite much progress being made in both strategies, a general strategy that can permit all types of primary amines to serve as deaminative alkyl radical precursors is still rare. In 2024, Tortosa and co-workers reported a photochemical hydrodeamination reaction of isonitriles wherein various types of amine (α-1°, α-2° and α-3°)-derived isonitriles have been successfully used as alkyl radical precursors through C–N bond cleavage under visible-light irradiation (Scheme 43).74 The visible-light-mediated reaction can proceed with or without the addition of a photocatalyst, but the addition of an organophotocatalyst 4CzIPN causes significant rate acceleration for the reaction. On the basis of preliminary mechanistic studies, the authors proposed a possible mechanism for the hydrodeamination reaction of isonitriles. The oxidative quenching of the excited 4CzIPN (PC*) by isonitriles 338 results in the generation of radical anion species 341 and PC˙+. The occurrence of C–N bond cleavage in 341 leads to the generation of alkyl radical species 342 along with the release of CN−. The alkyl radical 342 may abstract a proton from silane 339 to deliver the target hydrodeamination products 340 and silyl radical 343. The SET oxidation of 343 by PC˙+ delivers silyl cationic species 344 and regenerates the ground state 4CzIPN (PC). Finally, 344 is trapped by CN− to give (TMS)3SiCN 345.
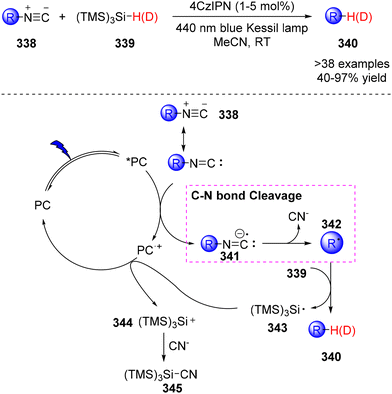 |
| Scheme 43 Isonitriles as deaminative alkyl radical equivalents for the hydro(deutero)deamination reactions under visible light photocatalytic reaction conditions. | |
3.5.3 Cleavage of C–N bonds in benzyl-protected amine derivatives. Very recently, the Xia group reported an interesting reductive deprotection of benzyl-derived nitrogen groups via the photochemical mesolytic cleavage of C–N bonds enabled by an excited phenolate-type photocatalyst (Scheme 44).75 This protocol is also applicable in the deprotection of benzyl-derived oxygen-related groups, such as ether, ester and carbamate groups. Mechanistically, the excited phenolate-type photocatalyst's reducing benzyl ring into a radical anion 348 and the subsequent mesolytic cleavage process play key roles in the achievement of C–N or C–O bond cleavage.
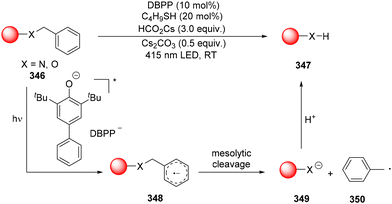 |
| Scheme 44 Reductive mesolytic debenzylation via C–N (or C–O) bond cleavage under visible light photocatalytic conditions. | |
3.5.4 Energy-transfer photocatalytic cleavage of C–N bonds in 1,2-dialkyldiazenes in situ generated from primary amines. In 2023, the Lambert group developed a method for the deaminative C(sp3)–C(sp3) cross-coupling of primary amines with O-nosylhydroxylamines enabled by an energy-transfer-mediated photocatalysis using [Ir(dF(CF3)ppy)2(dtbbpy)]PF6 (Scheme 45).76 Mechanistically, the reaction initiates from the in situ generation of 1,2-dialkyldiazenes 347 from primary amines 344 and O-nosylhydroxylamines 345 in the presence of atmospheric oxygen. Then energy transfer from the triplet state 3[Ir]* to the diazenes can generate the triplet excited state of the diazenes. Finally, the occurrence of homolytic C–N bond cleavage in diazenes 354 gives rise to two alkyl radical fragments (355 and 356) that can recombine to forge (sp3)C–(sp3)C bonds, along with the extrusion of a molecule of N2. The present protocol provides a novel approach for utilizing readily available amines as building blocks for the construction of complex carbon frameworks.
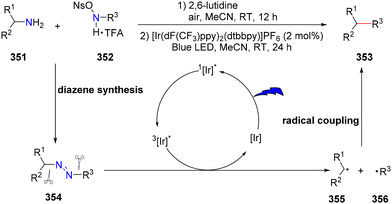 |
| Scheme 45 Deaminative C(sp3)–C(sp3) cross-coupling of primary amines with O-nosylhydroxylamines via denitrogenation of in situ generated diazenes enabled by energy-transfer photocatalysis. | |
3.5.5 C–N/C–C σ-bond metathesis via homolytic cleavage under visible light irradiation conditions. Very recently, the Li and Zeng group described an interesting C–N/C–C σ-bond metathesis reaction between tertiary amines and α-diketones to access aromatic amides and aryl alkyl ketones in the absence of photosensitizers under the irradiation of visible light (Scheme 46).77 Mechanistic studies disclosed that the simultaneous cleavage and recombination of C–N and C–C bonds in intermediates via homolytic fragmentation plays a key role in the formation of the target metathesis products.
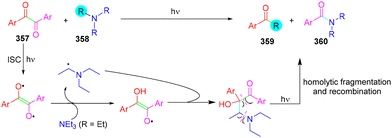 |
| Scheme 46 Visible-light irradiation enabling an intermolecular C–N/C–C σ-bond metathesis reaction between tertiary amines and α-diketones. | |
4. Summary and outlook
In summary, in this review, we have overviewed the recent state of the art of visible-light-driven photocatalytic C–C and C–N bond cleavage reactions associated with amines and their derivatives, including cycloalkylamines, tertiary amines, N-heterocycles, alkyl- or carbonyl-substituted Hantzsch esters, redox-active imines (RAIs), isonitriles, benzyl-protected amine derivatives, in situ generated diazenes, etc. All these photocatalytic reactions for the cleavage of C–C or C–N bonds showed relatively mild reaction conditions, more environmentally benign processes, higher selectivity, and broader functional group tolerance in comparison with the traditional thermal reactions. Despite much advance being made in this field, several big challenges still exist and need to be overcome: (1) since the C–C and C–N bond scission may break relevant amines and their derivatives into two fragments, both of which can be used as potential synthons from the viewpoint of atom economy, making full use of these synthons in organic synthesis has become a big challenge. Currently, most of the reported studies suffer from the utilization of one fragment only. (2) The research studies on the photocatalytic asymmetric synthesis involving C–C and/or C–N bond cleavage in amines and their derivatives have still been underdeveloped. The challenges lie in the following several factors. Firstly, the elusive radical species is difficult to control during the formation of chiral centers; secondly, the design and screening of suitable asymmetric ligands is a time-consuming task; thirdly, the precise cooperation between photocatalytic systems and asymmetric catalytic systems to create highly enantioselective centers is also a difficult task to deal with; finally, since the process of C–C or C–N bond cleavage may produce several reactive species, avoiding side reactions is a challenging task. Thus, the design of efficient photocatalytic and asymmetric catalytic systems for the synthesis of valuable chiral compounds is still highly desirable. (3) Currently, most of the reported photocatalytic systems for the cleavage of C–C and/or C–N bonds are limited to homogeneous catalytic systems which have the disadvantages of high catalytic loadings and inconvenient recycling. How to develop heterogeneous photocatalytic systems for the cleavage of C–C and/or C–N bonds remains a challenging task.
With the rapid development of visible-light photocatalytic organic synthesis and with even more amines and their derivatives being synthesized or isolated from natural sources, it is reasonable to expect that the photocatalytic strategies for the cleavage of C–C and/or C–N bonds in amines and their derivatives will find even broader applicability for the synthesis of complicated and valuable organic molecules.
Author contributions
Conceptualization, Y.L. and Q.N.; writing – original draft, Y.L. and Q.N.; writing – review and editing, Q.N., Y.L., Y.Z. and L.C.; funding acquisition, Y.L. All authors have given approval to the final version of the manuscript.
Data availability
No primary research results, software or code have been included and no new data were generated or analysed as part of this review.
Conflicts of interest
There are no conflicts to declare.
Acknowledgements
We are grateful to the Natural Science Foundation of China (No. 22171242) and the Natural Science Foundation of Zhejiang Province (No. LY20B020013) for financial support.
References
-
(a) C. C. Marvin, Synthesis of Amines and Ammonium Salts, Compr. Org. Synth., 2014, 34–99 CrossRef CAS;
(b) A. Ricci, Amine Group Chemistry: From Synthesis to the Life Science, Wiley, Weinheim, 2008 Search PubMed;
(c) J. T. Njardarson, Transition-Metal-Catalyzed Rearrangements of Small Cycloalkanes: Regioselectivity Trends in β-Carbon Elimination Reactions, J. Chem. Educ., 2010, 87, 1348–1349 CrossRef;
(d) O. I. Afanasyev, E. Kuchuk, D. L. Usanov and D. Chusov, Reductive Amination in the Synthesis of Pharmaceuticals, Chem. Rev., 2019, 119, 11857–11911 CrossRef CAS PubMed.
-
(a) P. Ruiz-Castillo and S. L. Buchwald, Applications of Palladium-Catalyzed C-N Cross-Coupling Reactions, Chem. Rev., 2016, 116, 12564–12649 CrossRef CAS PubMed;
(b) A. Trowbridge, S. M. Walton and M. J. Gaunt, New Strategies for the Transition-Metal Catalyzed Synthesis of Aliphatic Amines, Chem. Rev., 2020, 120, 2613–2692 CrossRef CAS;
(c) A. Cabre, X. Verdaguer and A. Riera, Recent Advances in the Enantioselective Synthesis of Chiral Amines via Transition Metal-Catalyzed Asymmetric Hydrogenation, Chem. Rev., 2022, 122, 269–339 CrossRef CAS PubMed.
-
(a) A. Biffis, D. Centomo, A. Del Zotto and M. Zecca, Pd Metal Catalysts for Cross-Couplings and Related Reactions in the 21st Century: A Critical Review, Chem. Rev., 2018, 118, 2249–2295 CrossRef CAS PubMed;
(b) L.-J. Cheng and N. P. Mankad, C-C and C-X coupling reactions of unactivated alkyl electrophiles using copper catalysis, Chem. Soc. Rev., 2020, 49, 8036–8064 RSC;
(c) W. Xue, X. Jia, X. Wang, X. Tao, Z. Yin and H. Gong, Nickel-catalyzed formation of quaternary carbon centers using tertiary alkyl electrophiles, Chem. Soc. Rev., 2021, 50, 4162–4184 CAS.
-
(a) Cleavage of Carbon-Carbon Single Bonds by Transition Metals, ed. M. Murakami and N. Chatani, Wiley-VCH, Weinheim, 2015, pp. 1–278 Search PubMed;
(b) Y.-F. Liang, M. Bilal, L.-Y. Tang, T.-Z. Liang, M. Bilal, L.-Y. Tang, T.-Z. Wang, Y.-Q. Guan, Z. Cheng, M. Zhu, J. Wei and N. Jiao, Carbon-Carbon Bond Cleavage for Late-Stage Functionalization, Chem. Rev., 2023, 123, 12313–12370 CAS , and references cited therein.
-
(a) K. Ouyang, W. Hao, W.-X. Zhang and Z. Xi, Transition-Metal-Catalyzed Cleavage of C-N Single Bonds, Chem. Rev., 2015, 115, 12045–12090 CAS;
(b) O. Wang, Y. Su, L. Li and H. Huang, Transition-metal catalysed C-N bond activation, Chem. Soc. Rev., 2016, 45, 1257–1272 Search PubMed.
- M. D. R. Lutz, S. Roediger, M. A. Rivero-Crespo and B. Morandi, Mechanistic Investigation of the Rhodium-Catalyzed Transfer Hydroarylation Reaction Involving Reversible C-C Bond Activation, J. Am. Chem. Soc., 2023, 145, 26657–26666 CAS.
- C. Aïssa, Transition-Metal-Catalyzed Rearrangements of Small Cycloalkanes: Regioselectivity Trends in β-Carbon Elimination Reactions, Synthesis, 2011, 3389–3407 CrossRef.
- H. Yorimitsu and K. Oshima, Metal-mediated retro-allylation of homoallyl alcohols for highly selective organic synthesis, Bull. Chem. Soc. Jpn., 2009, 82, 778–792 CrossRef CAS.
- J. B. Brazier and N. C. O. Tomkinson, Secondary and primary amine catalysts for iminium catalysis, Top. Curr. Chem., 2010, 291, 281–347 CrossRef CAS PubMed.
- A. Roglans, A. Pla-Quintana and M. Moreno-Manas, Diazonium Salts as Substrates in Palladium-Catalyzed Cross-Coupling Reactions, Chem. Rev., 2006, 106, 4622–4643 CrossRef CAS.
-
(a) J. García-cárceles, K. A. Bahou and J. Bower, Recent Methodologies That Exploit Oxidative Addition of C-N Bonds to Transition Metals, ACS Catal., 2020, 10, 12738–12759 CrossRef;
(b) E. Wenkert, A.-L. Han and C.-J. Jenny, Nickel-induced conversion of carbon–nitrogen into carbon–carbon bonds. One-step transformations
of aryl, quaternary ammonium salts into alkylarenes and biaryls, J. Chem. Soc., Chem. Commun., 1988, 975–976 RSC;
(c) Q. Zeng, F. Li and X. Yin, Transition metal-catalyzed construction of C-X bonds via cleavage of C-N bond of quaternary ammonium salts, AIMS Mol. Sci., 2023, 10, 153–170 CAS;
(d) X. Chen, N.-Z. Wang, Y.-M. Cheng, X. Kong and Z.-Y. Cao, Recent Advances in Electro- or Photochemical Driven Transformations via Cleavage of the C-N Bond of Quaternary Ammonium Salts, Synthesis, 2023, 2833–2842 CAS.
-
(a) A. R. Katritzky, G. De Ville and R. C. Patel, Hydrogenation of alkynes with a palladium anchored polystyrene catalyst, Tetrahedron, 1981, 37, 25–30 CrossRef;
(b) J. B. Bapat, R. J. Blade, A. J. Boulton, J. Epsztajn, A. R. Katritzky, J. Lewis, P. Molina-Buendia, P.-L. Nie and C. A. Ramsden, Pyridines as leaving groups in synthetic transformations: Nucleophilic displacements of amino groups, and novel preparations of nitriles and isocyanates, Tetrahedron Lett., 1976, 17, 2691–2694 CrossRef;
(c) F. He, S. Ye and J. Wu, Recent Advances in Pyridinium Salts as Radical Reservoirs in Organic Synthesis, ACS Catal., 2019, 9, 8943–8960 CrossRef CAS;
(d) J. T. M. Correia, V. A. Fernandes, B. T. Matsuo, J. A. C. Delgado, W. C. de Souza and M. W. Paixao, Photoinduced deaminative strategies: Katritzky salts as alkyl radical precursors, Chem. Commun., 2020, 56, 503–514 RSC;
(e) F. J. R. Klauck, M. J. James and F. Glorius, Deaminative Strategy for the Visible-Light-Mediated Generation of Alkyl Radicals, Angew. Chem., Int. Ed., 2017, 56, 12336–12339 CrossRef CAS.
-
(a) P. T. Anastas and M. M. Kirchhoff, Origins, current status, and future challenges of green chemistry, Acc. Chem. Res., 2002, 35, 686–693 CrossRef CAS;
(b) R. Sheldon, I. Arends and U. Hanefeld, Green Chemistry and Catalysis, Wiley-VCH, Weinheim, 2007, pp. 1–5 CrossRef;
(c) P. T. Anasta and J. C. Waner, Green Chemistry: Theory and Practice, Oxford University Press, Oxford, 1998 Search PubMed.
-
(a) C. R. J. Stephenson, T. P. Yoon and D. W. C. MacMillan, Visible Light Photocatalysis in Organic Chemistry, Wiley-VCH, Weinheim, Germany, 2018 CrossRef;
(b) J. M. R. Narayanam and C. R. J. Stephenson, Visible light photoredox catalysis: applications in organic synthesis, Chem. Soc. Rev., 2011, 40, 102–113 RSC;
(c) M. H. Shaw, J. Twilton and D. W. C. MacMillan, Photoredox Catalysis in Organic Chemistry, J. Org. Chem., 2016, 81, 6898–6926 CrossRef CAS PubMed;
(d) T. P. Yoon, M. A. Ischay and J. Du, Visible light photocatalysis as a greener approach to photochemical synthesis, Nat. Chem., 2010, 2, 527–532 CrossRef CAS;
(e) C. K. Prier, D. A. Rankic and D. W. C. MacMillan, Visible Light Photoredox Catalysis with Transition Metal Complexes: Applications in Organic Synthesis, Chem. Rev., 2013, 113, 5322–5363 CrossRef CAS PubMed;
(f) Y.-Q. Zou, J.-R. Chen and W.-J. Xiao, Homogeneous Visible-Light Photoredox Catalysis, Angew. Chem., Int. Ed., 2013, 52, 11701–11703 CrossRef CAS;
(g) L. Marzo, S. Pagire, O. Reiser and B. König, Visible-Light Photocatalysis: Does It Make a Difference in Organic Synthesis?, Angew. Chem., Int. Ed., 2018, 57, 10034–10072 CrossRef CAS;
(h) N. Romero and D. A. Nicewicz, Organic Photoredox Catalysis, Chem. Rev., 2016, 116, 10075–10166 CrossRef CAS.
-
(a) X.-Y. Yu, J.-R. Chen and W.-J. Xiao, Visible Light-Driven Radical-Mediated C-C Bond Cleavage/Functionalization in Organic Synthesis, Chem. Rev., 2021, 121, 506–561 CrossRef CAS PubMed;
(b) W. Yin and X. Wang, Recent advances in iminyl radical-mediated catalytic cyclizations and ring-opening reactions, New J. Chem., 2019, 43, 3254–3264 RSC;
(c) X. Wu and C. Zhu, Recent advances in radical-mediated C-C bond fragmentation of non-strained molecules, Chin. J. Chem., 2019, 37, 171–182 CrossRef CAS;
(d) S. A. Morris, J. Wang and N. Zheng, The Prowess of Photogenerated Amine Radical Cations in Cascade Reactions: From Carbocycles to Heterocycles, Acc. Chem. Res., 2016, 49, 1957–1968 CrossRef CAS;
(e) J. Xuan, Z.-G. Zhang and W.-J. Xiao, Visible-Light-Induced Decarboxylative Functionalization of Carboxylic Acids and Their Derivatives, Angew. Chem., Int. Ed., 2015, 54, 15632–15641 CrossRef CAS PubMed;
(f) D. M. Kitcatt, S. Nicolle and A.-L. Lee, Direct decarboxylative Giese reactions, Chem. Soc. Rev., 2022, 51, 1415–1453 RSC;
(g) L. McMurray, T. M. McGuire and R. L. Howells, Recent Advances in Photocatalytic Decarboxylative Coupling Reactions in Medicinal Chemistry, Synthesis, 2020, 1719–1737 CrossRef CAS;
(h) Y. Xu, H. Chen, L. Yu, X. Peng, J. Zhang, Z. Xing, Y. Bao, A. Liu, Y. Zhao, C. Tian, Y. Liang and X. Huang, A light-driven enzymatic enantioselective radical acylation, Nature, 2024, 625, 74–78 CrossRef CAS PubMed;
(i) K. Jia and Y. Chen, Visible-light-induced alkoxyl radical generation for inert chemical bond cleavage/functionalization, Chem. Commun., 2018, 54, 6105–6112 RSC;
(j) Z. Zuo, Photocatalytic alkoxy radical-mediated transformations, Tetrahedron Lett., 2018, 59, 2103–2111 CrossRef;
(k) H.-H. Li, J.-Q. Li, X. Zheng and P.-Q. Huang, Photoredox-Catalyzed Decarboxylative Cross-Coupling of α-Amino Acids with Nitrones, Org. Lett., 2021, 23, 876–880 CAS;
(l) G.-Q. Li, F.-R. Meng, W.-J. Xiao and J.-R. Chen, Photoinduced copper-catalyzed asymmetric radical three-component cross-coupling of 1,3-enynes with oxime esters and carboxylic acid, Org. Chem. Front., 2023, 10, 2773–2781 RSC;
(m) S. Yang, L. Wang, L. Wang and H. Li, Visible-Light Photoredox-Catalyzed Regioselective Sulfonylation of Alkenes Assisted by Oximes via [1,5]-H Migration, J. Org. Chem., 2020, 85, 564–573 CrossRef CAS.
-
(a) M. A. Ischay, M. E. Anzovino, J. Du and T. P. Yoon, Efficient Visible Light Photocatalysis of [2+2] Enone Cycloadditions, J. Am. Chem. Soc., 2008, 130, 12886–12887 CrossRef CAS PubMed;
(b) J. M. R. Narayanam, J. W. Tucker and C. R. J. Stephenson, Electron-Transfer Photoredox Catalysis: Development of a Tin-Free Reductive Dehalogenation Reaction, J. Am. Chem. Soc., 2009, 131, 8756–8757 CrossRef CAS PubMed;
(c) S.-P. Luo, E. Mejía, A. Friedrich, A. Pazidis, H. Junge, A. E. Surkus, R. Jackstell, S. Denurra, S. Gladiali, S. Lochbrunner and M. Beller, Photocatalytic Water Reduction with Copper-Based Photosensitizers: A Noble-Metal-Free System, Angew. Chem., Int. Ed., 2013, 52, 419–423 CrossRef CAS.
-
(a) L. Capaldo, D. Ravelli and M. Fagnoni, Direct Photocatalyzed Hydrogen Atom Transfer (HAT) for Aliphatic C-H Bonds Elaboration, Chem. Rev., 2022, 122, 1875–1924 CrossRef CAS PubMed;
(b) J. D. Bell and J. A. Murphy, Recent advances in visible light-activated radical coupling reactions triggered by (i) ruthenium,(II) iridium and (iii) organic photoredox agents, Chem. Soc. Rev., 2021, 50, 9540–9685 RSC.
-
(a) B. Górski, A.-L. Barthelemy, J. J. Douglas, F. Juliá and D. Leonori, Copper-catalysed amination of alkyl iodides enabled by halogen-atom transfer, Nat. Catal., 2021, 4, 623–630 CrossRef;
(b) T. Constantin, F. Juliá, N. S. Sheikh and D. Leonori, A case of chain propagation: α-aminoalkyl radicals as initiators for aryl radical chemistry, Chem. Sci., 2020, 11, 12822–12828 RSC;
(c) T. Constantin, M. Zanini, A. Regni, N. S. Shelikh, F. Juliá and D. Leonori, Aminoalkyl radicals as halogen-atom transfer agents for activation of alkyl and aryl halides, Science, 2020, 367, 1021–1026 CrossRef CAS PubMed;
(d) R. K. Neff, Y.-L. Su, S. Liu, M. Rosado, X. Zhang and M. P. Doyle, Generation of Halomethyl Radicals by Halogen Atom Abstraction and Their Addition Reactions with Alkenes, J. Am. Chem. Soc., 2019, 141, 16643–16650 CrossRef CAS PubMed;
(e) Y.-L. Su, L. Tram, D. Wherritt, H. Arman, W. P. Griffith and M. P. Doyle, α-Amino Radical-Mediated Diverse Difunctionalization of Alkenes: Construction of C-C, C-N, and C-S Bonds, ACS Catal., 2020, 10, 13682–13687 CAS.
-
(a) K. Nakajima, Y. Miyake and Y. Nishibayashi, Synthetic Utilization of α-Aminoalkyl Radicals and Related Species in Visible Light Photoredox Catalysis, Acc. Chem. Res., 2016, 49, 1946–1956 CAS;
(b) S. Zhu, A. Das, L. Bui, H. Zhou, D. P. Curran and M. Rueping, Oxygen Switch in Visible-Light Photoredox Catalysis: Radical Additions and Cyclizations and Unexpected C-C-Bond Cleavage Reactions, J. Am. Chem. Soc., 2013, 135, 1823–1829 CrossRef CAS;
(c) S. M. Thullen and T. Rovis, A Mild Hydroaminoalkylation of Conjugated Dienes Using a Unified Cobalt and Photoredox Catalytic System, J. Am. Chem. Soc., 2017, 139, 15504–15508 CrossRef CAS.
-
(a) Y. Zou and W. Xiao, Visible Light Mediated α-Amino C-H Functionalization Reactions, Wiley-VCH, Berlin, 2018, ch 4, pp. 93–128 Search PubMed;
(b) J. Xuan and W. Xiao, Visible-Light Photoredox Catalysis, Angew. Chem., Int. Ed., 2012, 51, 6828–6838 CrossRef CAS;
(c) J. M. R. Narayanam and C. R. J. Stephenson, Visible light photoredox catalysis: applications in organic synthesis, Chem. Soc. Rev., 2011, 40, 102–113 RSC;
(d) L. Shi and W. Xia, Photoredox functionalization of C-H bonds adjacent to a nitrogen atom, Chem. Soc. Rev., 2012, 41, 7687–7697 RSC;
(e) A. G. Condie, G. J. Conzález-Gómez and C. R. J. Stephenson, Visible-Light Photoredox Catalysis: Aza-Henry Reactions via C-H Functionalization, J. Am. Chem. Soc., 2010, 132, 1464–1465 CrossRef CAS.
-
(a) W. Huang and X. Cheng, Hantzsch Esters as Multifunctional Reagents in Visible-Light Photoredox Catalysis, Synlett, 2017, 148–158 CAS;
(b) P.-Z. Wang, J.-R. Chen and W.-J. Xiao, Hantzsch esters: an emerging versatile class of reagents in photoredox catalyzed organic synthesis, Org. Biomol. Chem., 2019, 17, 6936–6951 RSC.
- O. O. Sokolova and J. F. Bower, Selective Carbon-Carbon Bond Cleavage of Cyclopropylamine Derivatives, Chem. Rev., 2021, 121, 80–109 CrossRef CAS PubMed.
-
(a) S. Maity, M. Zhu, R. S. Shinabery and N. Zheng, Intermolecular [3+2] Cycloaddition of Cyclopropylamines with Olefins by Visible-Light Photocatalysis, Angew. Chem., Int. Ed., 2012, 51, 222–226 CrossRef CAS PubMed;
(b) T. H. Ngugen, S. Maity and N. Zheng, Visible light mediated intermolecular [3 + 2] annulation of cyclopropylanilines with alkynes, Beilstein J. Org. Chem., 2014, 10, 975–980 CrossRef;
(c) T. H. Nguyen, S. A. Morris and N. Zheng, Intermolecular [3+2] Annulation of Cyclopropylanilines with Alkynes, Enynes, and Diynes via Visible Light Photocatalysis, Adv. Synth. Catal., 2014, 356, 2831–2837 CrossRef CAS PubMed.
-
(a) J. Wang and N. Zheng, The cleavage of a C-C Bond in cyclobutylanilines by visible-light photoredox catalysis: Development of a [4+2] annulation method, Angew. Chem., Int. Ed., 2015, 54, 11424–11427 CrossRef CAS;
(b) J. Wang, T. H. Nguyen and N. Zheng, Photoredox-catalyzed [4+2] annulation of cyclobutylanilines with alkenes, alkynes, and diynes in continuous flow, Sci. China: Chem., 2016, 59, 180–183 CrossRef CAS.
- L. Chen, Y. Li, M. Han, Y. Peng, X. Chen, S. Xiang, H. Gao, T. Lu, S. P. Luo, B. Zhou, H. Wu, Y. F. Yang and Y. Liu, P/N-Heteroleptic Cu(I)-Photosensitizer-Catalyzed [3 + 2] Regiospecific Annulation of Aminocyclopropanes and Functionalized Alkynes, J. Org. Chem., 2022, 87, 15571–15581 CrossRef CAS PubMed.
-
(a) D. Uraguchi, Y. Kimura, F. Ueoka and T. Ooi, Urea as a Redox-Active Directing Group under Asymmetric Photocatalysis of Iridium-Chiral Borate Ion Pairs, J. Am. Chem. Soc., 2020, 142, 19462–19467 CrossRef CAS PubMed;
(b) Y. Kimura, D. Uraguchi and T. Ooi, Catalytic asymmetric synthesis of 5-membered alicyclic α-quaternary β-amino acids via [3 + 2]-photocycloaddition of α-substituted acrylates, Org. Biomol. Chem., 2021, 19, 1744–1747 RSC;
(c) Y. Yin, Y. Li, T. P. Gonçalves, Q. Zhan, G. Wang, X. Zhao, B. Qiao, K.-W. Huang and Z. Jiang, All-Carbon Quaternary Stereocenters α to Azaarenes via Radical-Based Asymmetric Olefin Difunctionalization, J. Am. Chem. Soc., 2020, 142, 19451–19456 CrossRef CAS;
(d) Y. Dai, S. Liang, G. Zeng, H. Huang, X. Zhao, S. Cao and Z. Jiang, Asymmetric [3 + 2] photocycloadditions of cyclopropylamines with electron-rich and electron-neutral olefins, Chem. Sci., 2022, 13, 3787–3795 RSC;
(e) Y. Dai, H. Huang, S. Liang, Y. Yin, X. Ban, X. Zhao and Z. Jiang, Catalytic Asymmetric Intermolecular [3 + 2] Photocycloaddition of Cyclopropylamines with Electron-Deficient Olefins, Org. Lett., 2003, 25, 4551–4555 CrossRef PubMed;
(f) L. Mollari, M. A. Valle-Anores, A. M. Martínez-Gualda, L. Marzo, A. Fraile and J. Aleman, Asymmetric synthesis of cyclic β-amino carbonyl derivatives by a formal [3+2] photocycloaddition, Chem. Commun., 2002, 58, 1334–1337 RSC.
- D. Staveness, T. M. Sodano, K. Li, E. A. Burnham, K. D. Jackson and C. R. J. Stephenson, Providing a New Aniline Bioisostere through the Photochemical Production of 1-Aminonorbornanes, Chem, 2019, 5, 215–226 CAS.
- A. R. Allen, A. E. Tharp and C. R. J. Stephenson, A formal (3+2) cycloaddition strategy toward 2-azanorbornanes, ChemRxiv, 2022, preprint, DOI:10.26434/chemrxiv-2022-42h36.
- Y. Zheng, W. Huang, R. K. Dhungana, A. Granados, S. Keess, M. Makvandi and G. A. Molander, Photochemical Intermolecular [3σ + 2σ]-Cycloaddition for the Construction of Aminobicyclo[3.1.1]heptanes, J. Am. Chem. Soc., 2022, 144, 23685–23690 CrossRef CAS PubMed.
-
(a) X. Zhang, Y. Wang, J. Liu, C. Tian, X. Li, P. Xie, Z. Zhu and T. Yao, Synthesis of 3-aminotetrahydro-1H-carbazols by visible-light photocatalyzed cycloaddition of cyclopropylanilines with 2-alkenylarylisocyanides, Chem. Commun., 2023, 59, 14423–14426 RSC;
(b) J. Qiu, W. Li, X. Li, Y. Cao, C.-X. Pan and H. Li, Radical-Based cis-Selective Annulations of N,N’-Cyclic Azomethine Imines with N-Sulfonyl Cyclopropylamines, Org. Lett., 2023, 25, 8000–8004 CrossRef CAS PubMed;
(c) S. Li and L. Zhou, Photocatalytic (3 + 3) Annnulation of Vinyldiazo Compounds and Aminocyclopropanes, Org. Lett., 2024, 26, 3294–3298 CrossRef CAS PubMed.
-
(a) M. Wakioka, H. Morita, N. Ichihara, M. Saito, I. Osaka and F. Ozawa, Mixed-Ligand Approach to Palladium-Catalyzed Direct Arylation Polymerization: Synthesis of Donor-Acceptor Polymers Containing Unsubstituted Bithiophene Units, Macromolecules, 2020, 53, 158–164 CAS;
(b) E. D. Silva, O. A. L. Alves, R. T. Ribeiro, R. C. R. Chagas, J. A. F. P. Villar and J. L. Princival, Homogeneous CuCl2/TMEDA/TEMPO-Catalyzed chemoselective base- and halogen-free aerobic oxidation of primary alcohols in mild conditions, Appl. Catal., A, 2021, 623, 118289 CAS;
(c) K. Verebelyi and B. Ivan, Cationic polymerization of styrene by the TiCl4/N,N,N′,N’-tetramethylethylenediamine(TMEDA) catalyst system in benzotrifluoride, an environmentally benign solvent, at room temperature, Polymer, 2012, 53, 3426–3431 CrossRef CAS;
(d) D. Baskaran, A. H. E. Muller and S. Sivaram, The effect of TMEDA on the kinetics of the anionic polymerization of methyl methacrylate in tetrahydrofuran using lithium as counterion, Macromol. Chem. Phys., 2000, 201, 1901–1911 CrossRef CAS.
- S. Cai, X. Zhao, X. Wang, Q. Liu, Z. Li and D. Z. Wang, Visible-Light-Promoted C-C Bond Cleavage: Photocatalytic Generation of Iminium Ions and Amino Radicals, Angew. Chem., Int. Ed., 2012, 51, 8050–8053 CrossRef CAS.
- B. Lipp, A. Lipp, H. Detert and T. Opatz, Light-Induced Alkylation of (Hetero)aromatic Nitriles in a TransitionMetal-Free C-C-Bond Metathesis, Org. Lett., 2017, 19, 2054–2057 CrossRef CAS PubMed.
- Y. Zhao, C. Empel, W. Liang, R. M. Koenigs and F. W. Patureau, Gem-Difluoroallylation of Aryl Sulfonium Salts, Org. Lett., 2022, 24, 8753–8758 CrossRef CAS.
- J. B. Beatty and C. R. J. Stephenson, Synthesis of (-)-Pseudotabersonine, (-)-Pseudovincadifformine, and (+)-Coronaridine Enabled by Photoredox Catalysis in Flow, J. Am. Chem. Soc., 2014, 136, 10270–10273 CrossRef CAS PubMed.
- J. Qiao, W. Zhao, Y. Liang, Z.-J. Yao and S. Wang, Diastereoselective Access to Tetracyclic Eight-Membered Lactams through a Dearomative Heck Reaction and an Alkylative Ring-Opening Driven by Photoexcited Spiroindolines, Chem. – Eur. J., 2021, 27, 6308–6314 CrossRef CAS.
- D. Mazzarella, T. Bortolato, G. Pelosi and L. Dell'Amico, Photocatalytic (3 + 2) dipolar cycloadditions of aziridines driven by visible-light, Chem. Sci., 2023, 15, 271–277 RSC.
- A. L. Ruiz, M. L. Mantia, D. Merli, S. Protti and M. Fagnoni, Alkyl Radical Generation via C-C Bond Cleavage in 2-Substituted Oxazolidines, ACS Catal., 2022, 12, 12469–12476 CrossRef PubMed.
- X.-Y. Lv, A. Abrams and R. Martin, Dihydroquinazolinones as adaptative C(sp3) handles in arylations and alkylations via dual catalytic C-C bond-functionalization, Nat. Commun., 2022, 13, 2394–2401 CrossRef CAS PubMed.
- G. Li, R. Chen, L. Wu, Q. Fu, X. Zhang and Z. Tang, Alkyl Transfer from C-C Cleavage, Angew. Chem., Int. Ed., 2013, 52, 8432–8436 CrossRef CAS PubMed.
-
(a) W. Chen, Z. Liu, J. Tian, J. Li, J. Ma, X. Cheng and G. Li, Building Congested Ketone: Substituted Hantzsch Ester and Nitrile as Alkylation Reagents in Photoredox Catalysis, J. Am. Chem. Soc., 2016, 138, 12312–12315 CrossRef CAS PubMed;
(b) K. Nakajima, S. Nojima, K. Sakata and Y. Nishibayashi, Visible-Light-Mediated Aromatic Substitution Reactions of Cyanoarenes with 4-Alkyl-1,4-dihydropyridines through Double Carbon-Carbon Bond Cleavage, ChemCatChem, 2016, 8, 1028–1032 CrossRef CAS;
(c) E. Gandolfo, X. Tang, S. R. Roy and P. Melchiorre, Photochemical Asymmetric Nickel-Catalyzed Acyl Cross-Coupling, Angew. Chem., Int. Ed., 2019, 58, 16854–16858 CrossRef CAS PubMed.
- F. Gu, W. Huang, X. Liu, W. Chen and X. Cheng, Substituted Hantzsch Esters as Versatile Radical Reservoirs in Photoredox Reactions, Adv. Synth. Catal., 2017, 360, 925–931 CrossRef.
-
(a) K. Nakajima, S. Nojima and Y. Nishibayashi, Nickel- and Photoredox-Catalyzed Cross-Coupling Reactions of Aryl Halides with 4-Alkyl-1,4-dihydropyridines as Formal Nucleophilic Alkylation Reagents, Angew. Chem., Int. Ed., 2016, 55, 14106–14110 CrossRef CAS;
(b) X. Wang, H. Li, G. Qiu and J. Wu, Substituted Hantzsch esters as radical reservoirs with the insertion of sulfur dioxide under photoredox catalysis, Chem. Commun., 2019, 55, 2062–2065 RSC;
(c) Y. Liu, Z. Zhang, Z. Wei, Z. Zhong, S. Liu, Y. Yang and X. Zeng, Catalyst-free photochemical reactions of alkyl dihydropyridines via modulation of chromophores and light wavelength, New J. Chem., 2023, 47, 7369–7374 RSC.
-
(a) X. Jiang, W. Xiong, S. Deng, F.-D. Lu, Y. Jia, Q. Yang, L.-Y. Xue, X. Qi, J. A. Tunge, L.-Q. Lu and W.-J. Xiao, Construction of axial chirality via asymmetric radical trapping by cobalt under visible light, Nat. Catal., 2022, 5, 788–797 CrossRef CAS;
(b) X. Song, Y. Zhang, Y. Li, X. Zhao, Y. Yin, X. Ban and Z. Jiang, Catalytic asymmetric synthesis of azaarene-functionalized tertiary amines and α-amino acid derivatives from E/Z-ketimine mixtures via enantioselective radical coupling, ACS Catal., 2023, 13, 6396–6402 CrossRef CAS;
(c) T. Li, L. Luo, X. Cheng and Z. Lu, Enantioconvergent Cross-Coupling Reaction with 1,4-Dihydropyridine Derivatives via Photoinduced Nickel Catalysis, ACS Catal., 2024, 14, 3278–3286 CrossRef CAS;
(d) R.-X. Wang, P. Yang, Y.-Z. Cheng and S.-L. You, Rh-Catalyzed Asymmetric Allylic Substitution with Photo-Generated Alkyl Radical Species, ACS Catal., 2024, 14, 12403–12409 CrossRef CAS;
(e) J. L. Shwarz, H.-M. Huang, T. O. Paulisch and F. Glorius, Stereoretentive Catalytic [3+2] Cycloaddition/Rearrangement/Decarboxylation Reactions of Indoles with Non-Racemic Donor-Acceptor Cyclopropanes, ACS Catal., 2020, 10, 1621–1627 CrossRef;
(f) R. A. Angnes, C. Potnis, S. Liang, C. R. D. Correia and G. B. Hammond, Photoredox-Catalyzed Synthesis of Alkylaryldiazenes: Formal Deformylative C-N Bond Formation with Alkyl Radicals, J. Org. Chem., 2020, 85, 4153–4164 CrossRef CAS PubMed;
(g) H.-W. Du, J. Sun, Q.-S. Gao, J.-Y. Wang, H. Wang, Z. Xu and M.-D. Zhou, Synthesis of Monofluoroalkenes through Visible-Light-Promoted Defluorinative Alkylation of gem-Difluoroalkenes with 4-Alkyl-1,4-dihydropyridines, Org. Lett., 2020, 22, 1542–1546 CrossRef CAS PubMed;
(h) A. V. Bay, K. P. Fitzpatrick, R. C. Betori and K. A. Scheidt, Combined Photoredox and Carbene Catalysis for the Synthesis of Ketones from Carboxylic Acids, Angew. Chem., Int. Ed., 2020, 59, 9143–9148 CrossRef CAS PubMed;
(i) X.-K. He, J. Lu, A.-J. Zhang, Q.-Q. Zhang, G.-Y. Xu and J. Xuan, BI-OAc-Accelerated C3-H Alkylation of Quinoxalin-2(1H)-ones under Visible-Light Irradiation, Org. Lett., 2020, 22, 5984–5989 CrossRef CAS PubMed;
(j) Y. Zhang, Y. Tanabe, S. Kuriyama and Y. Nishibayashi, Cooperative Photoredox- and Nickel-Catalyzed Alkylative Cyclization Reactions of Alkynes with 4-Alkyl-1,4-dihydropyridines, J. Org. Chem., 2021, 86, 12577–12590 CrossRef CAS PubMed;
(k) X. Wang, R. Liu, Q. Ding, W. Xiao and J. Wu, Synergistic photoredox and tertiary amine catalysis: generation of allylic sulfones from Morita-Baylis-Hillman acetates and sulfur dioxide, Org. Chem. Front., 2021, 8, 3308–3313 RSC;
(l) Y. Liu, W. Luo, T. Xia, Y. Fang, C. Du, X. Jin, Y. Li, L. Zhang, W. Lei and H. Wu, Merging radical-polar crossover/cycloisomerization processes: access to polyfunctional furans enabled by metallaphotoredox catalysis, Org. Chem. Front., 2021, 8, 1732–1738 RSC;
(m) S.-C. Ren, W.-X. Lv, X. Yang, J.-L. Yan, J. Xu, F.-X. Wang, L. Hao, H. Chai, Z. Jin and Y. R. Chi, Carbene-Catalyzed Alkylation of Carboxylic Esters via Direct Photoexcitation of Acyl Azolium Intermediates, ACS Catal., 2021, 11, 2925–2934 CrossRef CAS;
(n) A. V. Bay, K. P. Fitzpatrick, G. A. Gonzalez-Montiel, A. O. Farah, P. H.-Y. Cheong and K. A. Scheidt, Light-Driven Carbene Catalysis for the Synthesis of Aliphatic and α-Amino
Ketones, Angew. Chem., Int. Ed., 2021, 60, 17925–17931 CrossRef CAS PubMed;
(o) Z. Yao, J. Yang, Z. Luo, H. Wang, X. Zhang, J. Ye, L. Xu and Q. Shi, Photo-driven metal-free multicomponent reaction between aldehydes, anilines and 4-substituted-DHPs for the synthesis of secondary amines, Green Chem., 2022, 24, 7968–7973 RSC;
(p) X. Wang, Y. Tang, S. Ye, J. Zhang, Y. Kuang and J. Wu, Access to Sulfoxides under NHC/Photocatalysis via a Radical Pathway, Org. Lett., 2022, 24, 2059–2063 CrossRef CAS;
(q) L. Li, S.-Q. Zhang, Y. Chen, X. Cui, G. Zhao, Z. Tang and G.-X. Li, Photoredox Alkylation of Sulfinylamine Enables the Synthesis of Highly Functionalized Sulfinamides and S(VI) Derivatives, ACS Catal., 2022, 12, 15334–15340 CrossRef CAS;
(r) P. Ghosh, N. Y. Kwon, Y. Byun, N. K. Mishra, J. S. Park and I. S. Kim, Cobalt(II)-Catalyzed C-H Alkylation of N-Heterocycles with 1,4-Dihydropyridines, ACS Catal., 2022, 12, 15707–15714 Search PubMed;
(s) K. B. Pal, E. M. D. Tommaso, A. K. Inge and B. Olofsson, Stereospecific Photoredox-Catalyzed Vinylation to Functionalized Alkenes and C-Glycosides, Angew. Chem., Int. Ed., 2023, 62, e202301368 CrossRef PubMed;
(t) J. Liu, W. Zhang, X. Tao, Q. Wang, X. Wang, Y. Pan, J. Ma, L. Yan and Y. Wang, Photoredox microfluidic synthesis of trifluoromethylated amino acids, Org. Lett., 2023, 25, 3083–3088 CrossRef CAS PubMed;
(u) L. L. Yan, T.-Z. Zhang, Z.-Z. Lv and M.-C. Fu, Photoinduced defluorinative alkylation of trifluoromethyl alkenes with carbonyl derivatives by C-C bond scission, Org. Chem. Front., 2023, 10, 6205–6211 RSC;
(v) H. Li, S. Li, H. Hu, R. Sun, M. Liu, A. Ding, X. Liu, W. Luo, Z. Fu, S. Guo and H. Cai, Visible-light-induced C(sp3)-C(sp3) bond formation via radical/radical cross-coupling, Chem. Commun., 2023, 59, 1205–1208 RSC;
(w) S. Patel, A. Chakrabort and I. Chatterjee, C(sp3)-C(sp3) Radical-Cross-Coupling Reaction via Photoexcitation, Org. Lett., 2023, 25, 8246–8251 CrossRef CAS;
(x) Q. Li, L.-C. Wang, Z.-P. Bao and X.-F. Wu, Photoredox-catalyzed carbonylative acylation of styrenes with Hantzsch esters, Chem. Commun., 2024, 60, 4656–4658 RSC.
- F. Gu, W. Huang, X. Liu, W. Chen and X. Cheng, Substituted Hantzsch Esters as Versatile Radical Reservoirs in Photoredox Reactions, Adv. Synth. Catal., 2018, 360, 925–931 CrossRef CAS.
-
(a) L. Marchini, J. A. C. Velez, E. Elias, J. T. M. Correia, S. Moura and M. W. Paixão, Organophotocatalytic Carbamoylation of Morita-Baylis-Hillman Carbonates, Adv. Synth. Catal., 2024, 366, 2063–2071 CrossRef CAS;
(b) Z. Zhong, H. Wu, X. Chen, Y. Luo, L. Yang, X. Feng and X. Liu, Visible Light-Promoted Enantioselective Acylation and Alkylation of Aldimines Enabled by 9-Fluorenone Electron-Shuttle Catalysis, J. Am. Chem. Soc., 2024, 146, 20401–20413 CrossRef CAS;
(c) K. B. Vega, A. L. C. de Oliveira, B. Konig and M. W. Paixão, Visible-Light-Induced Synthesis of 1,2-Dicarboxyl Compounds from Carbon Dioxide, Carbamoyl-dihydropyridine, and Styrene, Org. Lett., 2024, 26, 860–865 CrossRef CAS PubMed;
(d) Z. Zhang, J. Wang, C. Yu, J. Tan, H. Du and N. Chen, Visible-Light-Induced Acylative Pyridylation of Styrenes, Org. Lett., 2024, 26, 4727–4732 CrossRef CAS PubMed;
(e) P. H. R. Oliveira, E. Tordato, J. A. C. Velez, P. S. Carneiro and M. W. Paixão, Visible-Light Mediated Carbamoylation of Nitrones under a Continuous Flow Regime, J. Org. Chem., 2023, 88, 6407–6419 CrossRef CAS PubMed;
(f) T. Singh, G. C. Upreti, A. Arora, H. Chauhan and A. Singh, Visible Light-Mediated Carbamoylation of para-Quinone Methides, J. Org. Chem., 2023, 88, 2784–2791 CrossRef CAS;
(g) X. Shan, X. Wang, E. Chen, J. Liu, K. Lu and X. Zhao, Visible-Light-Promoted Trifluoromethylthiolation and Trifluoromethylselenolation of 1,4-Dihydropyridines, J. Org. Chem., 2023, 88, 319–328 CrossRef CAS;
(h) L. Cardinale, M.-O. W. S. Schmotz, M. O. Konev and A. J. von Wangelin, Photoredox-Catalyzed Synthesis of α-Amino Acid Amides by Imine Carbamoylation, Org. Lett., 2022, 24, 506–510 CrossRef CAS;
(i) B. T. Mastuo, P. H. R. Oliveira, J. T. M. Correia and M. W. Paixão, Carbamoylation of Azomethine Imines via Visible-Light Photoredox Catalysis, Org. Lett., 2021, 23, 6775–6779 CrossRef;
(j) L. Cardinale, M. O. Konev and A. J. von Wangelin, Photoredox-Catalyzed Addition of Carbamoyl Radicals to Olefins: A 1,4-Dihydropyridine Approach, Chem. – Eur. J., 2020, 26, 8239–8243 CrossRef CAS PubMed;
(k) N. Alandini, L. Buzzetti, G. Favi, T. Schulte, L. Candish, K. D. Collins and P. Melchiorre, Amide Synthesis by Nickel/Photoredox-Catalyzed Direct Carbamoylation of (Hetero)Aryl Bromides, Angew. Chem., Int. Ed., 2020, 59, 5248–5253 CrossRef CAS.
-
(a) F. Tureček and R. R. Julian, Peptide Radicals and Cation Radicals in the Gas Phase, Chem. Rev., 2013, 113, 6691–6733 Search PubMed;
(b) R. A. Zubarev, N. L. Kelleher and F. W. Mclafferty, Electron Capture Dissociation of Multiply Charged Protein Cations. A Nonergodic Process, J. Am. Chem. Soc., 1998, 120, 3265–3266 CAS;
(c) N. Neerathilingam, M. B. Reddy and R. Anandhan, Regioselective Synthesis of 2° Amides Using Visible-Light-Induced Photoredox-Catalyzed Nonaqueous Oxidative C-N Cleavage of N,N-Dibenzylanilines, J. Org. Chem., 2021, 86, 15117–15127 CrossRef CAS PubMed.
-
(a) P. F. Yuan, Z. Yang, S. S. Zhang, C. M. Zhu, X. L. Yang and Q. Y. Meng, Deconstructive Carboxylation of Activated Alkenes with Carbon Dioxide, Angew. Chem., Int. Ed., 2024, 63, e202313030 CrossRef CAS;
(b) K.-G. Cao, T.-Y. Gao, L.-L. Liao, C.-K. Ran, Y.-X. Jiang, W. Zhang, Q. Zhou, J.-H. Ye, Y. Lan and D.-G. Yu, Photocatalytic carboxylation of styrenes with CO2 via C=C double bond cleavage, Chin. J. Catal., 2024, 56, 74–80 CrossRef CAS;
(c) Y. Peng, H. Bao, L. Zheng, Y. Zhou, Q. Ni, X. Chen, Y. Li, P. Yan, Y.-F. Yang and Y. Liu, Cu(I)-Photosensitizer-Catalyzed Olefin-α-Amino Radical Metathesis/Demethylenative Cyclization of 1,7-Enynes, Org. Lett., 2024, 26, 3218–3223 CrossRef CAS PubMed;
(d) Q. Jiang, H. Bao, Y. Peng, Y. Zhou, L. Chen and Y. Liu, Demethylenative cyclization of 1,7-enynes using a-amino radicals as a traceless initiator enabled by Cu(I)-photosensitizers, Chem. Commun., 2024, 60, 6399–6402 RSC;
(e) G. C. Upreti, T. Singh, K. Khanna, D. Sahoo and A. Singh, Photocatalytic, α-Aminoalkyl Radical-Mediated, MethyleneExtrusive Ring-Closing Transformation of o-Alkynyl and o-Cyano Acrylamides, Org. Lett., 2024, 26, 3652–3656 CrossRef CAS PubMed;
(f) W.-T. Li, Z.-X. Zhang, J. Huang, H.-M. Jiang, Z.-W. Luo, J.-H. Li and X.-H. Ouyang, Photochemical Divergent Ring-Closing Metathesis of 1,7-Enynes: Efficient Synthesis of Spirocyclic Quinolin-2-ones, Org. Lett., 2024, 26, 6664–6669 CrossRef CAS PubMed.
-
(a) W. Ji, P. Li, S. Yang and L. Wang, Visible-light-induced oxidative formylation of N-alkyl-N-(prop-e-yn-1-yl)anilines with molecular oxygen in the absence of an external photosenistizer, Chem. Commun., 2017, 53, 8482–8485 RSC;
(b) S. Yang, P. Li, Z. Wang and L. Wang, Photoinduced oxidative formylation of N,N-dimethylanilines with molecular oxygen without external photocatalyst, Org. Lett., 2017, 19, 3386–3389 CrossRef CAS PubMed.
- X. Li, X. Gu, Y. Li and P. Li, Aerobic Transition-Metal-Free Visible-Light Photoredox Indole C-3 Formylation Reaction, ACS Catal., 2104, 4, 1897–1900 CrossRef.
- N. Iqbal and E. J. Cho, Formation of Carbonyl Compounds from Amines through Oxidative C-N Bond Cleavage using Visible Light Photocatalysis and Applications to N-PMB-Amide Deprotection, Adv. Synth. Catal., 2015, 357, 2187–2192 CrossRef CAS.
- C. Gu, S. Wang, Q. Zhang and J. Xie, Visible-light-mediated amidation from carboxylic acids and tertiary amines via C–N cleavage, Chem. Commun., 2022, 58, 5873–5876 RSC.
- X. Zhang, Y. Shen and T. Rovis, Photoinduced Nickel-Catalyzed Selective N-Demethylation of Trialkylamines Using C(sp2)-Bromides as HAT Reagents, J. Am. Chem. Soc., 2023, 145, 3294–3300 CrossRef CAS.
- A. Arumugam and G. C. Senadi, Visible-light photocatalyzed C–N bond activation of tertiary amines: a three-component approach to synthesize quinazolines, Org. Biomol. Chem., 2024, 22, 1245–1253 RSC.
- A. Shiozuka, K. Sekine and Y. Kuninobu, Photoinduced Deaminative Borylation of Unreactive Aromatic Amines Enhanced by CO2, Org. Lett., 2021, 23, 4774–4778 CrossRef CAS.
- S. Ji, S. Qin, C. Yin, L. Luo and H. Zhang, Unreactive C-N Bond Activation of Anilines via Photoinduced Aerobic Borylation, Org. Lett., 2022, 24, 64–68 CrossRef CAS PubMed.
- J.-Y. Liang, Y.-W. Sun and Y.-Q. Zou, Photochemical reductive deamination of alpha-amino aryl alkyl ketones, Chem. Commun., 2023, 59, 14685–14688 RSC.
- F. Liu, Z.-P. Ye, Y.-Z. Hu, J. Gao, L. Zheng, K. Chen, H.-Y. Xiang, X.-Q. Chen and H. Yang, N,N,N′,N′-Tetramethylethylene-diamine-Enabled PhotoredoxCatalyzed C-H Methylation of N-Heteroarenes, J. Org. Chem., 2021, 86, 11905–11914 CrossRef CAS.
- Y. Meng, C. Pan, N. Liu, H. Li, Z. Liu, Y. Deng, Z. Wei, J. Xu and B. Fan, Photocatalytic synthesis of 2,3-diamines from anilines and DIPEA via C-N bond cleavage and C-C bond formation, Green Chem., 2024, 26, 300–305 RSC.
- S. Mondal, A. M. Pandey and B. Gnanaprakasam, Visible Light Mediated Organophotoredox Catalyzed Synthesis of Tetraketones Using Tertiary Amines as Alkyl Synthons, J. Org. Chem., 2024, 89, 3769–3780 CAS.
- H. Bao, B. Zhou, S.-P. Luo, Z. Xu, H. Jin and Y. Liu, P/N Heteroleptic Cu(I)-Photosensitizer-Catalyzed Deoxygenative Radical Alkylation of Aromatic Alkynes with Alkyl Aldehydes Using Dipropylamine as a Traceless Linker Agent, ACS Catal., 2020, 10, 7563–7572 Search PubMed.
- L. Zheng, Q. Jiang, H. Bao, B. Zhou, S. P. Luo, H. Jin, H. Wu and Y. Liu, Tertiary Amines Acting as Alkyl Radical Equivalents Enabled by a P/N Heteroleptic Cu(I) Photosensitizer, Org. Lett., 2020, 22, 8888–8893 Search PubMed.
- H. Bao, L. Zheng, Q. Liu, M. Han, Y. Li, M. Bao, Y. Li, P. Yan and Y. Liu, A photocatalytic traceless C–N bond formation/cleavage strategy enabling the use of (α-chiral) alkyl aldehydes as deoxygenative (chiral) alkyl radical equivalents, Org. Chem. Front., 2023, 10, 5551–5558 CAS.
- Y. Z. Chen, Y. M. Chen, Y. Hu, J. P. Qu and Y. B. Kang, Transalkylation via C-N Bond Cleavage of Amines Catalyzed by Super Organophotoreductant CBZ6, Org. Lett., 2023, 25, 7518–7522 Search PubMed.
- K. Aida, M. Hirao, T. Saitoh, T. Yamamoto, Y. Einaga, E. Ota and J. Yamaguchi, Selective C–N Bond Cleavage in Unstrained Pyrrolidines Enabled by Lewis Acid and Photoredox Catalysis, J. Am. Chem. Soc., 2024, 146, 30698–30707 CrossRef CAS.
- D. Alpers, K. P. Cole and C. R. J. Stephenson, Visible Light Mediated Aryl Migration by Homolytic C-N Cleavage of Aryl Amines, Angew. Chem., Int. Ed., 2018, 57, 12167–12170 CrossRef CAS PubMed.
- M. A. Ashley and T. Rovis, Photoredox-Catalyzed Deaminative Alkylation via C-N Bond Activation of Primary Amines, J. Am. Chem. Soc., 2020, 142, 18310–18316 CrossRef CAS PubMed.
- J. R. Dorsheimer, M. A. Ashley and T. Rovis, Dual Nickel/Photoredox-Catalyzed Deaminative Cross-Coupling of Sterically Hindered Primary Amines, J. Am. Chem. Soc., 2021, 143, 19294–19299 CrossRef CAS PubMed.
- A. D. Marchese, J. R. Dorsheimer and T. Rovis, Photoredox-Catalyzed Generation of Tertiary Anions from Primary Amines via a Radical Polar Crossover, Angew. Chem., Int. Ed., 2024, 63, e202317563 CrossRef CAS.
- L. Huang, R. Kancherla and M. Rueping, Nickel Catalyzed Regiodivergent Cross-Coupling Alkylation of Aryl Halides with Redox-Active Imines, ACS Catal., 2022, 12, 11563–11572 CrossRef CAS.
- Y. Shen, G. Yang, W. Huang, A. Shaginian, Q. Lin, J. Wan, J. Li, Y. Deng and G. Liu, Photoredox Deaminative Alkylation in DNA-Encoded Library Synthesis, Org. Lett., 2022, 24, 2650–2654 CrossRef CAS PubMed.
- B. Wang, C.-T. Wang, X.-S. Li, X.-Y. Liu and Y.-M. Liang, Visible-Light-Induced C-F and C-N Bond Cleavage for the Synthesis of gem-Difluoroalkenes, Org. Lett., 2022, 24, 6566–6560 CrossRef CAS.
- G. Zhang, L. Wang, L. Cui, P. Gao and F. Chen, Deaminative defluoroalkylation of α-trifluoromethylalkenes enabled by photoredox catalysis, Org. Biomol. Chem., 2023, 21, 294–299 RSC.
- I. Quiros, M. Martin, M. Gomez-Mendoza, M. J. Cabrera-Afonso, M. Liras, I. Fernandez, L. Novoa and M. Tortosa, Isonitriles as Alkyl Radical Precursors in Visible Light Mediated Hydro- and Deuterodeamination Reactions, Angew. Chem., Int. Ed., 2024, 63, e202317683 CrossRef CAS PubMed.
- K. Liang, X. Li, D. Wei, C. Jin, C. Liu and C. Xia, Deprotection of benzyl-derived groups via photochemically mesolytic cleavage of C–N and C–O bonds, Chem, 2023, 9, 511–522 CAS.
- K. A. Steiniger, M. C. Lamb and T. H. Lambert, Cross-Coupling of Amines via Photocatalytic Denitrogenation of In Situ Generated Diazenes, J. Am. Chem. Soc., 2023, 145, 11524–11529 CrossRef CAS PubMed.
- R. Li, R. Zhan, Y. Lang, C.-J. Li and H. Zeng, Intermolecular C−C/C−N σ-bond metathesis enabled by visible light, Chem. Sci., 2024, 15, 12900–12905 RSC.
|
This journal is © the Partner Organisations 2025 |
Click here to see how this site uses Cookies. View our privacy policy here.