DOI:
10.1039/B210286B
(Communication)
Chem. Commun., 2003, 126-127
Nitrogen atom exchange between molybdenum, tungsten and carbon. A convenient method for N-15 labeling†
Received
(in Purdue, IN, USA)
18th October 2002
, Accepted 5th November 2002
First published on 26th November 2002
Modern computational methods aid in identifying new modes of reactivity based upon their predictions of thermodynamic preference and reaction pathways. We are currently examining the reactions shown in eqn. (1) by experimental procedures and | L3M ML3 + X Y ⇌ L6M2XY | (1a) |
| L6M2XY ⇌ L3M X + L3M Y | (1b) |
computational methods based on model compounds (M = Mo or W; L = alkoxide, thiolate, amide, alkyl; X = Y = N or CR, X = N, Y = CR).1–5
The reaction 1b involves the reversible formation and rupture of X–Y and M–M bonds and was the subject of a recent communication in this journal dealing with the coupling of benzylidine ligands when M = W and L = 2-MeC6H4S.5 During this work we noted that the calculated barrier to X–Y and M–M bond formation was higher than that for X, Y group transfer via L3M(μ-X)(μ-Y)ML3 intermediates. This prompted us to probe for the existence of such reactions. We describe herein the isotopically labeled reactions shown in eqn. (2), (3) and (4), where L = OBut that confirm the predictions based on the computations.6
| L3W 13CMe + L3Mo CPri
⇌ L3W CPri + L3Mo 13CMe | (2) |
| L3W 13CMe + L3Mo N ⇌ L3W N + L3Mo 13CMe | (3) |
| L3W 15N + L3Mo N ⇌ L3W N + L3Mo 15N | (4) |
Evidence for X, Y group exchange in reactions (2)–(4) was seen by NMR spectroscopy6 (1H, 13C or 15N) and by mass spectrometry. The calculations based on model compounds L = OH or CH3, X = Y = N or CH predict that the thermodynamic products involving the reactants present in eqn. (4) are N
N and L3Mo
WL3 but these are not observed. However, L3Mo
WL3 (where L = OBut) is seen in reaction (5) although the L3Mo
WL3 compound reacts further with L3Mo
N7 to give Mo2L6 and L3W
N.6,8
| L3Mo N + L3W WL3
→ L3W N + L3Mo WL3 | (5) |
The facility of nitrogen atom exchange in reactions (3)–(5) led us to examine the potential for nitrogen atom exchange between molybdenum, tungsten and carbon.9 The compound (ButO)3W
N8 was shown to exchange its nitrogen atom with that of acetonitrile, eqn. (6) and furthermore catalyze 15N
| (ButO)3W 15N + MeC N ⇌ (ButO)3W N + MeC 15N | (6) |
scrambling with
benzonitrile,
eqn. (7). See
Fig. 1.
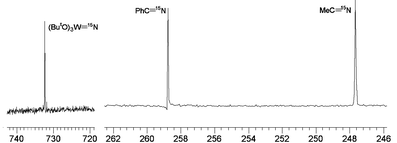 |
| Fig. 1
15N NMR spectrum of the reaction between labelled MeC 15N and PhC N (natural abundance) in the presence of (ButO)3W N recorded in d8-THF at 298 K, 50.6 MHz, showing the 15N for 14N atom exchange. | |
The 15N/14N scrambling in reactions (6) and (7) has been monitored by 15N NMR spectroscopy and mass spectrometry.9 Rather interestingly the closely related compound (ButO)3Mo
N7 does not exhibit similar nitrogen atom exchange with MeC
15N or catalyze scrambling with PhC
N, at room temperature in d8-THF. However, upon addition of a trace amount of (ButO)3W
N, scrambling of nitrogen atoms occurs leading to formation of PhC
15N and (ButO)3Mo
15N.
In order to establish that the 15N labeling exchange observed in reaction 7 occurs exclusively as a result of nitrogen atom exchange and not cyano group exchange, we followed the reaction between PhC
N, Me13C
N and MeC
15N in d8-THF in the presence of (ButO)3W
N. By NMR spectroscopy the appearance of Me13C
15N and PhC
15N could be detected but no 13C enrichment of the benzonitrile cyano group carbon was detected. See Fig. 2.
|  | (7) |
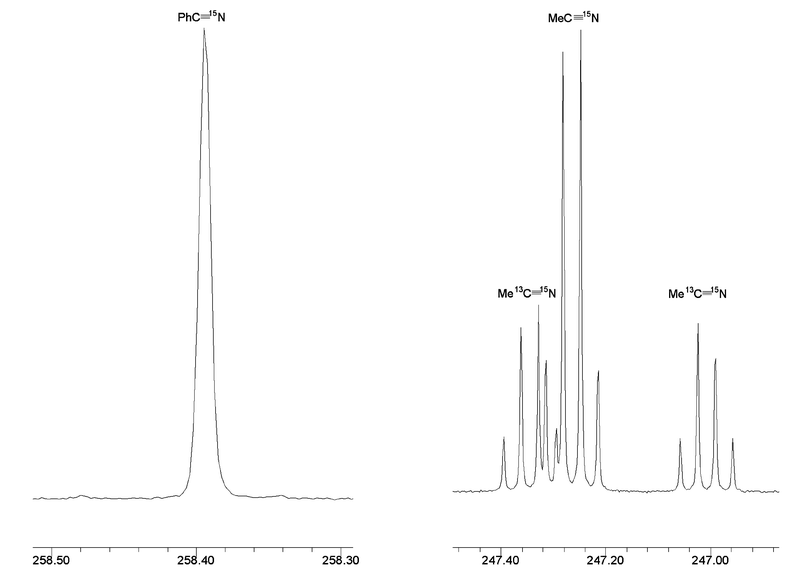 |
| Fig. 2
15N NMR spectrum of the reaction mixture between Me13C N, MeC 15N and PhC N in the presence of a trace of (ButO)3W N recorded in d8-THF at 298 K, 50.6 MHz. The PhC 15N signal shows enhancement due to 15N atom exchange and appears as a singlet due to lack of coupling to 1H or 13C whereas the MeC N 15N signal shows coupling to 1H, 3J1H–15N = 1.7 Hz and for Me13C 15N coupling to 13C, 1J13C–15N = 17 Hz. The signal thus appears as a central 1∶3∶3∶1 quartet flanked by 13C satellites. The unsymmetrical nature of the 13C satellites arises from 12C/13C isotopic chemical shift perturbation. | |
The seemingly most plausible pathway leading to nitrogen atom exchange involves the formation of a 2 + 2 cycloaddition reactive intermediate as represented schematically by eqn. (8).
|
| (8) |
This bears analogy to the reaction pathway of alkyne metathesis by (ButO)3W
CR complexes.10–12 Calculations employing density functional theory13 on the model reactants (HO)3W
N and MeC
N predict that formation of the five-coordinate intermediate shown in Fig. 3 to be enthalpically higher in energy than the starting materials by 18 kcal mol−1. In contrast, formation of the related molybdenum 2 + 2 cycloaddition product is enthalpically disfavored by an additional 10 kcal mol−1.
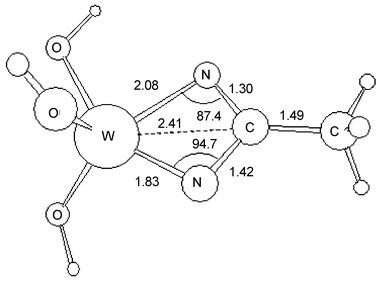 |
| Fig. 3 A drawing of the calculated geometry for the minimum energy of a hypothetical reactive intermediate (HO)3W(η2-N2CMe) showing the asymmetric nature of the metallacycle. | |
Based on these preliminary results we believe that these and related nitrogen atom exchange reactions hold considerable promise for 15N isotope labeling studies in a wide variety of chemical systems. Furthermore, it should be possible to link nitrogen atom exchange reactions to dinitrogen cleavage via a reactive mononuclear L3M fragment of the type pioneered by Cummins.14–16
We thank the National Science Foundation for support.
Notes and references
- R. R. Schrock, M. L. Listemann and L. G. Sturgeoff, J. Am. Chem. Soc., 1982, 104, 4291 CrossRef CAS.
- H. Strutz and R. R. Schrock, Organometallics, 1984, 3, 1600 CrossRef CAS.
- M. H. Chisholm, Chem. Record, 2001, 1, 12 CrossRef CAS.
- M. H. Chisholm, E. R. Davidson, J. C. Huffman and K. B. Quinlan, J. Am. Chem. Soc., 2001, 123, 9652 CrossRef CAS.
- M. H. Chisholm, E. R. Davidson, M. Pink and K. B. Qunilan, Chem. Commun., 2002, 2770 RSC.
- These reactions were followed by 1H, 13C{H} and 15N NMR spectroscopy and the labeled compounds L3W
13CMe and L3W
15N were prepared by the ‘chop–chop’ reaction1 between L3W
WL3 and Me13C
N and MeC
15N respectively (L = OBut). All spectra were aquired at room temperature over a 12–16 h period after which time a statistical isotopic distribution of 15N was observed. Selected 13C NMR data (d6-benzene, 125.8 MHz): 13C{H}: L3W
13CMe, δ 254.1, J(183W–13C) 306.5 Hz; L3Mo
13CMe, δ 279.6; L3W
13CPri, δ 268.3; L3Mo
13CPri, δ 292.7. 15N NMR data (d8-THF, 50.6 MHz): L3W
15N, δ 731.8, J(183W–15N) 54; L3Mo
15N, δ 828.8 (relative to NH3). The compound L3W
MoL3 shows two singlets in the 1H NMR spectrum (d6-benzene, 298 K, 400 MHz), δ 1.58 and 1.60 and a molecular ion with the anticipated isotope pattern for MoWL6+ in the mass spectrum..
- D. M. T. Chan, M. H. Chisholm, K. Folting, J. C. Huffman and N. S. Marchant, Inorg. Chem., 1986, 25, 4170 CrossRef CAS.
- M. H. Chisholm, D. M. Hoffman and J. C. Huffman, Inorg. Chem., 1983, 22, 2903 CrossRef CAS.
- All 15NMR experiments where performed at room temperature over a 12–16 h period using a 5 mm J. Young NMR tube with dried and degassed d8-THF. Organic nitriles were used as received and in some cases stored over activated molecular sieves. Reagents were carefully weighed out in the drybox and dissolved in d8-THF. All 15N data were collected using a Bruker 500 MHz spectrometer operating at 50.6 MHz.
- J. H. Wengrovius, J. Sancho and R. R. Schrock, J. Am. Chem. Soc., 1981, 103, 3932 CrossRef CAS.
- J. H. Freudenberger, R. R. Schrock, M. R. Churchill, A. L. Rheingold and J. W. Ziller, Organometallics, 1984, 3, 1563 CrossRef CAS.
- R. R. Schrock, J. Chem. Soc., Dalton Trans., 2001, 2541 RSC.
-
J. A. Pople, et al., Gaussian Inc., Pittsburgh, PA. 1998 Gaussian 98, Revision A.6.
- C. C. Cummins, Chem. Commun., 1998, 1777 RSC.
- C. E. Laplaza, M. J. A. Johnson, J. Peters, A. L. Odom, E. Kim, C. C. Cummins, G. N. George and I. J. Pickering, J. Am. Chem. Soc., 1996, 118, 8623 CrossRef CAS.
- C. E. Laplaza, A. R. Johnson and C. C. Cummins, J. Am. Chem. Soc., 1996, 118, 709 CrossRef CAS.
Footnote |
† Dedicated to Professor Dr. G. Huttner on the occasion of his 65th birthday. |
|
This journal is © The Royal Society of Chemistry 2003 |
Click here to see how this site uses Cookies. View our privacy policy here.